Estrogen receptor-beta signaling protects epidermal cytokine expression and immune function from UVB-induced impairment in mice
Received
29th June 2007
, Accepted 22nd October 2007
First published on 2nd November 2007
Abstract
A previous study in the hairless mouse, in which the photoimmune protective properties of a topical phytoestrogen or 17-β-estradiol were abrogated by the estrogen receptor antagonist ICI 182,780, revealed that estrogen receptor (Er) signaling is involved in the regulation of the suppression of immune function by UVB (290–320 nm) radiation. Here we identify the expression of Er-β but not Er-α mRNA in hairless mouse skin, whereas Er-α and Er-β mRNA were present in normal haired mouse skin. This suggests that the non-classical estrogen target Er-β is involved in the photoimmune modulation, and is consistent with Er-α being more closely associated with hair growth control, as indicated by other studies. In mice with a null mutation for Er-β, there was a significant exacerbation of the solar simulated UV (290–400 nm)-induced suppression of contact hypersensitivity. Immunohistochemical analysis revealed that the Er-β deficiency inhibited the normally immunoprotective upregulation by the UVA (320–400 nm) waveband of the epidermal expression of the cytokines IFN-γ and IL-12. Er-β deficiency also significantly increased the UVB-induced expression of the immunosuppressive cytokine IL-10. Thus Er signallingvia the Er-β is evidently a major regulator of the UVA and UVB waveband interactions that determine the skin's immune functional status, and achieves this by normalization of the cutaneous cytokine array in the UV-irradiated skin.
Introduction
We have recently made the observation in the Skh:hr-1 hairless mouse, that estrogen receptor signaling plays a protectively regulating role in the immunological response of the skin to UV radiation.1 Topical application of the complete estrogen receptor (Er) antagonist ICI 182,780 significantly exacerbated the suppression of contact hypersensitivity induced in these mice by solar simulated UV radiation (SSUV; 290–400 nm). On the other hand, topically applied estradiol, or the phytoestrogen equol, dose-dependently inhibited the photoimmune suppression, and this protective effect was antagonized by Er blockade by ICI 182,780.
The immune protection by topically applied estradiol or equol was shown to be mediated by their inactivation of the epidermal photoproduct that mediates UVB (290–320 nm)-induced immunosuppression, cis-urocanic acid,1,2 thereby suggesting that estrogen-dependent pathways in the skin regulate the immune response to the UVB waveband. It is well understood that photoimmune suppression is induced by this UVB waveband, and is associated with the development of a defective cytokine pattern in the skin, characterized by a relative overexpression of the Th-2-associated immune suppressive cytokine IL-10 and a deficiency of the Th-1-associated immune potentiating IL-12 and IFN-γ in the epidermis.3,4 However, the immune modulating activity of the longer wavelength UVA (320–400 nm) remains controversial.5 In spite of the controversy, we have previously reported consistent evidence in the mouse, that environmentally relevant suberythemogenic exposure to UVA radiation alone does not alter immune function, but at the same time provides significant dose-dependent protection against the immune suppressive properties of UVB radiation.6,7 Such UVA exposure induced IL-12 and IFN-γ expression in the mouse epidermis, and when combined with the immunosuppressive UVB irradiation or topical treatment with cis-urocanic acid, markedly reduced the suppression of immune function, and inhibited the upregulated epidermal IL-10 expression.7,8
These observations have led to the suggestion that the photoimmune protection by UVA radiation and by normal endogenous estrogen-dependent mechanisms, might be linked. It has been reported that estrogen receptors are expressed in the haired mouse skin, not only the classical estrogen target Er-α, but also the more recently recognized Er-β.9 In this study we identify Er-β gene activity in the skin of both hairless and normal haired mice. Using the Er-β gene knockout mouse (GKO; targeted mutation for Er-β), evidence is presented supporting the photoimmune protective effect of signalingvia this receptor, both in protecting against functional photoimmune suppression demonstrated by the contact hypersensitivity reaction, and in controlling the UVA- or UVB-modulated expression of the relevant epidermal cytokines IL-10, IL-12 and IFN-γ demonstrated immunohistochemically.
Materials and methods
Mice
Inbred female Skh:hr-1 hairless albino mice and normal haired C57BL/6 mice 8 to 12 weeks of age were obtained from the Veterinary Science breeding colonies. A breeding nucleus of the Er-β targeted mutation strain (B6.129P2-Esr2tm1Unc/J) was obtained from the Jackson Laboratory (Bar Harbor, Maine). Homozygous Er-β GKO males (–/–) were mated with heterozygous GKO females (+/–), and the genotype of the offspring (+/– or –/–) was identified by reverse transcriptase polymerase chain reaction (RT-PCR) from a tail sample.
The mice were maintained in a ventilated isolator cageing system on compressed paper bedding (Fibrecycle Pty. Ltd., Mudgeeraba, Queensland, Australia) and were fed stock rodent pellets (Gordons Specialty Stockfeeds, Yanderra, New South Wales, Australia) and acidified tap waterad lib. The room temperature was 25 °C, and the ambient lighting was from GEC F40 GO fluorescent tubes that do not emit UV radiation. The dorsal fur was removed from the haired mice with electric clippers (Oster clippers, #42 fine blade; Oster Manufacturing, Milwaukee, WI) 24 h before UV radiation exposure. All procedures were approved by the University of Sydney Animal Ethics Committee and comply with the current New South Wales Animal Welfare Act.
UV radiation
The solar simulated UV radiation (SSUV) was provided by a fluorescent tube source comprising a bank of 6 × 120 cm UVA tubes (Hitachi 40 W F40T 10/BL, Tokyo, Japan) flanking a single 120 cm UVB tube (Philips TL40W 12/RS, Eindhoven, The Netherlands) held in a reflective batten and filtered through a 0.125 mm sheet of cellulose acetate (Grafix Plastics, Cleveland, OH) to remove wavelengths below 290 nm. The UVB (290–320 nm) and UVA (320–400 nm) components of this source were isolated by switching off the unwanted tubes. The UVB was filtered through the cellulose acetate, and the UVA was filtered through a 6 mm window glass filter that is opaque to wavelengths below 320 nm. Irradiance was measured using an International Light (Newburyport, MA) IL1500 radiometer with UVA and UVB detectors calibrated to the relevant emission spectra.7Groups of mice were irradiated with timed exposures on the dorsum, unrestrained in their boxes with the wire lids removed. Temperature under the lights was stabilized by using curtains and an electric fan. The minimal edematous dose (MEdD) of SSUV in the Skh:hr-1 hairless mouse, which is equivalent to a minimal erythemal dose but more amenable to quantitation by non-invasively measuring the mid-dorsal skinfold thickness with a spring micrometer (Interrapid, Zurich, Switzerland), has been previously established as 1.33 kJ m–2 of UVB and 21.3 kJ m–2 of UVA,7 and is approximately twice this dose for normal Oster clipper-shaved haired mice.
Expression of Er-α, Er-β by RT-PCR
To establish the methodology, RNA was extracted from two classical estrogen target tissues of the normal control C57BL/6 mouse, the uterus and ovary, and then from the dorsal skin of groups of 3 C57BL/6, Skh:hr-1, Er-β+/– and Er-β–/– mice. Total RNA was extracted and first strand cDNA synthesized as previously described,10 and amplified and analysed by RT-PCR using primers as described by Ohnemus et al.9 The housekeeping gene used was β-actin.
Induction of contact hypersensitivity
For the measurement of contact hypersensitivity, groups of 5 shaved mice of the C57BL/6, Er-β+/– and Er-β–/– genotypes were irradiated (or remained non-irradiated) with a daily dose of SSUV equivalent to 1 MEdD on 3 consecutive days. This resulted in a mild to moderate but non-burning erythema in all three mouse strains. On days 8 and 9, the mice were sensitized on the unirradiated abdominal skin with 0.1 mL 2% oxazolone (Sigma Aldrich Corp., Castle Hill, New South Wales, Australia) in ethanol, in order to reveal systemic changes to the immune responsiveness, and were challenged on day 15 with 5 µL 2% oxazolone–ethanol applied to each surface of the pinnae. The negative control treatment was ear challenge only (no sensitization). Ear thickness was measured with the spring micrometer before and repeatedly between 16–24 h post-challenge, to establish the peak ear swelling in the unirradiated normal C57BL/6 mice. The group average ear swelling at this time point, and the % suppression of the reaction by the SSUV, were calculated for the three mouse genotypes.11 Statistical significance of the differences between treatment groups was obtained using Student's t test.
Immunohistochemistry
Previous studies in the Skh:hr-1 mouse have determined the time course for immunohistochemically detectable epidermal cytokine expression following SSUV, UVB and UVA exposure,7,8 and this was found to be similar for the shaved C57BL/6 mouse (unpublished). Therefore groups of 3 shaved Er-β+/–, Er-β–/– and the normal C57BL/6 mice, were exposed (or remained unexposed) to a single dose equivalent to 3 × MEdD of UVB alone (7.98 kJ m–2) known to be immunosuppressive in the C57BL/6 mouse, or to a dose of 400 kJ m–2 of glass-filtered UVA known to be sub-edematous but also of immunological relevance.8 Mice were euthanized and the dorsal skin removed to examine the effect of the UVA or UVB irradiation on specific cytokines at the peak of their expression in the epidermis. Thus IFN-γ and IL-12 were examined at 24 h and 72 h post-UVA exposure respectively, and IL-10 was examined at 72 h post-UVB exposure. Dorsal skin samples were fixed for 6 h in Histochoice (Amresco, Solon, OH), processed in an automated ethanol–formalin system, wax-embedded, and sections of 4 µm were cut for immunohistochemical staining.
As previously described in greater detail,7,8 the cytokines were identified by staining with the primary antibodies goat anti-mouse IFN-γ, IL-10 or IL-12 antibody (R&D Systems, Minneapolis, MN, USA), omitting this antibody for the negative control section. After quenching the endogenous peroxidases, the sections were incubated with the secondary antibody (horse radish peroxidase-labelled anti-goat IgG(H + L), Vector Laboratories Inc, Burlingame, CA, USA), followed by the chromogen 3,3′-diaminobenzidine (DAB; Kirkegaard & Perry Laboratories Inc, Gaithersburg, Maryland, USA), then counter-stained with hematoxylin. The sections were examined for positive brown staining under light microscopy, and images were captured digitally using a Sony HyperHAD color video camera (Sony, Melbourne, Australia) connected to a Leica Q500MC computer (Leica Microsystems, Sydney, Australia). Semi-quantitation of the positively stained area of the epidermis was obtained using the Leica image processing and analysis system (Leica QWin version V01.00, Cambridge, UK) and averaged for 16 sequential fields across the skin section from each of 3 mice per treatment group. Statistical significance of the differences between the mouse strains was obtained using Student's t test.
Results
Detection of the Er mRNAs in mouse skin
The C57BL/6 mouse uterus and ovary provided positive controls for the identification of the Er mRNAs, using the technique and primers described by Ohnemus et al.9 Analysis of the RNA extracted from the skin of Skh:hr-1 and C57BL/6 mice demonstrated that Er expression at the mRNA level differed between hairless and normal haired mice. Whereas both Er-α and Er-β were present in the haired mouse skin, Er-β predominated in hairless mouse skin in which Er-α was not detectable (Fig. 1). It was also confirmed that the Er-β–/– mouse expressed only Er-α, whereas both Er-α and Er-β could be detected in the heterozygous Er-β+/– mouse skin.
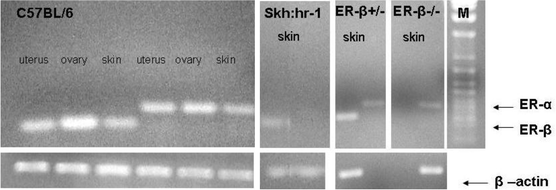 |
| Fig. 1 Expression of Er-α and Er-β mRNAs in extracts of uterus, ovary and dorsal skin from C57BL/6 mice, and in dorsal skin of Er-β+/–, Er-β–/– and hairless Skh:hr-1 mice. Housekeeping gene was β-actin. M = M.Wt. marker. | |
Contact hypersensitivity
The normal contact hypersensitivity reaction is measurable as an approximate doubling of the ear thickness following oxazolone challenge, shown in the C57BL/6 mice as a group average ear swelling of 33.7 ± 0.8 mm × 0.01 (Fig. 2). In the SSUV-irradiated C57BL/6 mice, the ear swelling was markedly (P < 0.001) reduced to 23.0 ± 0.8 mm × 0.01, indicating a 32% suppression of this immune function. The normal contact hypersensitivity reaction in both the Er-β+/– and Er-β–/– mice was moderately reduced (P < 0.01) to 26.7 ± 0.8 and 29.6 ± 1.0 mm × 0.01 in comparison with the C57BL/6, with no significant difference detected between Er-β+/– and Er-β–/–. However there was a significant exacerbation of the SSUV-suppression of contact hypersensitivity compared with the 32% suppression in C57BL/6 mice, such that the Er-β+/– ear swelling was reduced by 35% to 17.5 ± 1.4 mm × 0.01 (P < 0.05), and the Er-β–/– ear swelling by 53% to only 13.8 ± 2.1 mm × 0.01 (P < 0.001). The severe immunosuppression in the Er-β–/– mice was highly significantly exacerbated compared with both the partially compromised heterozygous Er-β+/– and the normal C57BL/6 mice (P < 0.001). Thus deletion of the Er-β activity enhanced the immunosuppressive effect of SSUV radiation.
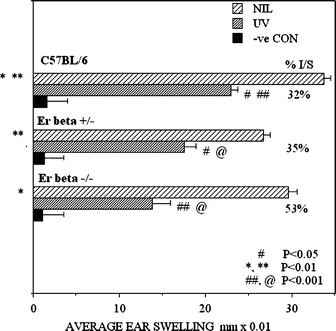 |
| Fig. 2 Comparison of the contact hypersensitivity response to oxazolone, measured as average post-challenge ear swelling, in mice of three genotypes, C57BL/6, Er-β+/– and Er-β–/–. Bars indicate SEM; n = 5. Pairs of symbols indicate significantly different responses. The significance of the differences in the severity of the UV-suppressed responses is compared against the response of the non-irradiated mice of the same genotype. | |
Cytokine
response to
UVA radiation
Immunopositive IFN-γ was detected in the epidermis of each of the mouse genotypes (Fig. 3). The cytokine was expressed intracellularly in the basal layer cells and also in the lining epidermal cells of the hair follicles in the dermis. A reduced proportion of the basal layer cells of Er-β–/– appeared to be expressing this cytokine, but this was not statistically significant by image analysis (Fig. 4). At 24 h following UVA irradiation, IFN-γ was observed as diffuse staining throughout the epidermal layer, suggesting that UVA radiation facilitated the release of IFN-γ from the basal cells. The intensity of the staining was strongest in the C57BL/6 epidermis, but was weaker in the Er deficient mice, with a statistically significant decrease in expression in the Er-β–/– epidermis (P < 0.05; Fig. 4). Hair follicle staining was reduced in the Er-β+/– and was not apparent in the Er-β–/– follicles. Thus the Er deficient mice maintained normal levels of IFN-γ in the epidermis, but showed a compromised capacity for upregulation of IFN-γ in response to UVA radiation.
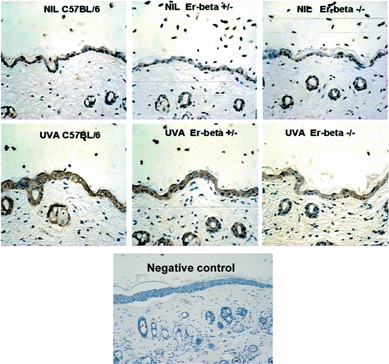 |
| Fig. 3 Immunohistochemical staining (brown) for IFN-γ in mid-dorsal skin sections of C57BL/6, Er-β+/– and Er-β–/– mice, before and at 24 h post-UVA irradiation. Primary antibody was omitted in negative control section. | |
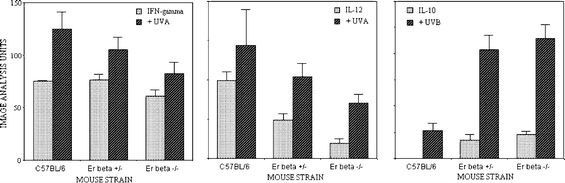 |
| Fig. 4 Semi-quantitation by image analysis of the immunohistochemically detected epidermal expression of the cytokines IFN-γ, IL-12 and IL-10 following irradiation with either UVB or UVA, in normal C57BL/6 mice and Er-β+/– and Er-β–/– mice. | |
The expression of IL-12 in normal skin differed, being diffuse throughout the epidermis in the C57BL/6 mice, and was also apparent in the epidermal lining of the hair follicles (Fig. 5). Untreated skin of the Er deficient mice showed significantly (P < 0.001; Fig. 4) decreased staining intensity in both the epidermis and the follicular lining cells, with IL-12 expression in the untreated Er-β–/–epidermis and follicles even weaker than the Er-β+/– mouse (P < 0.01; Fig. 4). UVA irradiation induced IL-12 at 72 h in all the mouse strains. Staining was the most intense in the C57BL/6 epidermis and hair follicle linings, with significantly reduced expression of IL-12 in both the UVA-irradiated Er-β+/– (P < 0.01; Fig. 4) and Er-β–/– (P < 0.001; Fig. 4) epidermis and hair follicles. Thus the Er deficient mice retained the capacity for IL-12 induction in response to UVA irradiation, but the upregulated epidermal level of IL-12 remained similar to, or less than the IL-12 level in the untreated C57BL/6 epidermis.
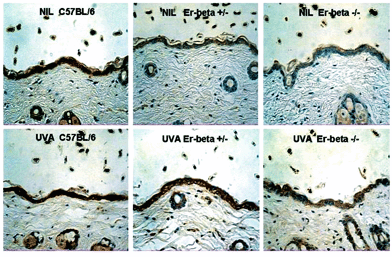 |
| Fig. 5 Immunohistochemical staining (brown) for IL-12 in mid-dorsal skin sections of C57BL/6, Er-β+/– and Er-β–/– mice, before and at 72 h post-UVA irradiation. | |
Cytokine
response to
UVB radiation
As expected, immunopositive IL-10 was almost undetectable in the normal C57BL/6 epidermis (Fig. 6). However, untreated Er-β-deficient epidermis did express significant levels of IL-10 in the epidermis and hair follicle lining cells (P < 0.01; Fig. 4). At 72 h following UVB irradiation, the hyperplastic response of the epidermis is apparent. Epidermal thickness was maximal in the Er-β–/– skin, which had increased to 5–6 cell layers thick compared with C57BL/6 which was 3–4 cell layers thick. There was increased IL-10 immunopositivity diffusely throughout the epidermis of all mouse strains as well as in the hair follicle lining cells. Discrete dermal cells were also IL-10 immunopositive. The most intense expression was found in the Er-β deficient skin, where not only cellular but also diffuse IL-10 staining was also seen in the dermal layer. Thus Er deficiency resulted in abnormal IL-10 expression in untreated skin, and an exacerbated upregulation of IL-10 expression in response to UVB radiation.
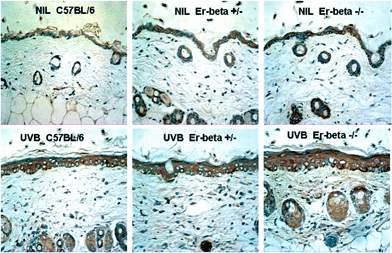 |
| Fig. 6 Immunohistochemical staining (brown) for IL-10 in mid-dorsal skin sections of C57BL/6, Er-β+/– and Er-β–/– mice, before and at 72 h post-UVB irradiation. | |
Discussion
Until relatively recently, only Er-α had been detected in murine skin in association with the hair growth cycle,12,13 although Er-β was identified in human skin, and reported as the predominating Er in human scalp skin.14–16 In human skin Er-α expression has been described as restricted to sebocytes of the sebaceous gland,14,16 with the stronger presence of Er-β localized in the outer root sheath, epithelial matrix, dermal papilla and cells of the bulge region. This localization was suggestive of a regulatory function by Er-β for both hair growth and epidermal basal cell proliferation. More recently, Er-β expression at both mRNA and protein level has been demonstrated in the skin of C57BL/6 mice,9 with Er-β expression constant and unaffected by the hair growth cycle, in contrast to Er-α for which the expression varied in a hair growth-dependent manner. Although we have not observed gross changes in the Er-β–/– pelage, reported evidence of abnormalities of the hair growth cycle in Er-β–/– mice suggests a minor regulatory role for Er-β through interaction with Er-α for hair growth control.9
In agreement with Ohnemus et al.,9 we found that the normal haired C57BL/6 mouse skin expressed both Er-α and Er-β mRNA. The detection here of Er-β mRNA as the predominant Er in the hairless Skh:hr-1 mouse skin, where Er-α was not detected, is not surprising considering the disrupted hair growth function in this mouse, and is consistent with functions other than hair growth control for the Er-β. As expected, the Er-β–/– mouse did not express Er-β mRNA. Both receptors were detected in the Er-β+/– mouse, but whether the level of Er-β was reduced in this mouse was not ascertained.
We found that the constitutive contact hypersensitivity response was slightly but significantly depressed in both the Er-β+/– and –/– mice, but as there was no difference in the depression between these two deficient strains, the biological significance is unclear. On the other hand, there was a distinct exacerbation of the SSUV-induced photoimmune suppression that was dependent on the Er-β deficiency, as the Er-β–/– mice displayed the greatest suppression, and the Er-β+/– mice had an intermediate response. This implies that Er-β has a natural photoimmune protective role in the skin, and identified that it is this non-classical Er in mouse skin that normally attenuates the severity of photoimmune suppression.
Immunohistochemical detection of the cytokines involved in photoimmune suppression supported the immune functional status measured as contact hypersensitivity in the three strains of mice. Constitutive expression of the suppressive IL-10, absent in the normal C57BL/6 mice, was observed in the Er deficient epidermis, and this observation may explain the slight but significant suppression of the constitutive contact hypersensitivity response in these mice. However following UVB irradiation, there was markedly stronger IL-10 expression in both Er deficient strains, not only in the epidermis, but also diffusely in the dermis and in dermal cell populations that appeared to be inflammatory infiltrating cells. Consistent with this, the Er-β–/– mice also developed the greatest epidermal hyperplasia, indicating the most severe photodamage. Expression of UVB-induced IL-10 strongly in the dermal inflammatory infiltrate in Er-β deficiency is consistent with the reported inhibition of IL-10 release from inflammatory peripheral blood monocytes by an Er-β agonist (diarylpropionitrile, DPN), although both Er-α and Er-β appeared to control IL-10 release from splenic or alveolar macrophages, suggestive of tissue compartment-specificity for these receptor functions.17,18
In contrast with this UVB response, the responses to UVA radiation were consistent with a down-regulation of the immune potentiating cytokines IFN-γ and IL-12 in Er-β deficiency. Although reduced IFN-γ expression in untreated Er-β–/– epidermis was not statistically significant by image analysis, there was significantly reduced expression of IL-12 in untreated epidermis of both Er deficient strains, and significantly reduced upregulation of these cytokines in response to UVA radiation.
Conclusions
We conclude from these cytokine responses that signalingvia the Er-β in the skin regulates the epidermal cytokine responses to SSUV, protecting the Th-1-associated IFN-γ and IL-12 from depletion by UVB, and restricting the release of immunosuppressive Th-2-associated IL-10 by UVB. These effects have previously also been attributed to the UVA waveband,7,8 and therefore our hypothesis that UVA photoimmune protection might be mediated via the Er-β appears to hold. The UVA waveband interacts with the skin by secondary oxidative reactions, and its photoimmune protective effect has been related to the UVA-inducibility of cutaneous heme oxygenase-1,19 an important redox-regulated stress enzyme found in most tissues. There is evidence of a relationship between estrogen protection against oxidative stress-induced damage in neurological or intestinal tissues and the induction of heme oxygenase-1,20,21 or its relevant downstream target, guanylyl cyclase.22,23 Interestingly, the protective estrogen-activated upregulation of heme oxygenase-1 in intestinal shock in the rat was found to be Er-β-mediated.21 In addition, specific Er-β signaling has also been reported to induce various other antioxidant enzymes such as quinone reductase,24 glutathione-S-transferase and γ-glutamylcysteine synthase25 in other tissues, consistent with a universal protective role for Er-β signaling against oxidative stress.
In summary, this study provides evidence that the photoimmune protective role of endogenous estrogen, both for maintaining normal T cell mediated immunity, measured as the contact hypersensitivity reaction, and for normalizing the array of immune regulatory cytokines released in the UV-irradiated epidermis, is mediated via the Er-β in the mouse. We speculate that this protection is achieved by the induction of the endogenous antioxidant defence molecules in the skin, similar to the skin response to UVA irradiation, and will confirm these pathways in future studies. If this role of the Er-β can be substantiated in human skin, the opportunity to develop new photoprotective strategies using receptor-specific agonists might provide better skin protection from solar UV radiation-induced damage in the more susceptible human populations, and particularly in males.
Acknowledgements
We acknowledge the University of Sydney Sesqui Grants for financial support of this work.
References
- S. Widyarini, D. Domanski, N. Painter and V. E. Reeve, Estrogen signalling protects against immune suppression by UV radiation exposure, Proc. Natl. Acad. Sci. USA, 2006, 103, 12837–12842 CrossRef CAS.
- S. Widyarini, N. Spinks, A. J. Husband and V. E. Reeve, Isoflavonoid compounds from red clover (Trifolium pratense) protect from inflammation and immune suppression induced by UV radiation, Photochem. Photobiol., 2001, 74, 465–470 CrossRef CAS.
- S. E. Ullrich, Review. Mechanisms underlying UV-induced immune suppression, Mutat. Res., 2005, 571, 185–205 CrossRef CAS.
- M. Norval, Review. The mechanisms and consequences of ultraviolet-induced immunosuppression, Progr. Biophys. Mol. Biol., 2006, 92, 108–118 CrossRef CAS.
- R. M. Tyrrell and V. E. Reeve, Potential protection of skin by acute UVA irradiation-From cellular to animal models, Progr. Biophys. Mol. Biol., 2006, 92, 86–91 CrossRef CAS.
- V. E. Reeve, M. Bosnic, C. Boehm-Wilcox, N. Nishimura and R. D. Ley, Ultraviolet A radiation (320–400 nm) protects hairless mice from immunosuppression induced by ultraviolet B radiation (280–320 nm) or cis-urocanic acid, Int. Arch. Allergy Immunol., 1998, 115, 316–322 Search PubMed.
- V. E. Reeve, D. Domanski and M. Slater, Radiation sources providing increased UVA/UVB ratios induce UVA dose-dependently increased photoprotection in hairless mice, Photochem. Photobiol., 2006, 82, 406–411 CrossRef CAS.
- J. Shen, S. Bao and V. E. Reeve, Modulation of IL-10, IL-12, and IFN-γ in the epidermis of hairless mice by UVA (320–400 nm) and UVB (280–320 nm) radiation, J. Invest. Dermatol., 1999, 113, 1059–1064 CrossRef CAS.
- U. Ohnemus, M. Uenalan, F. Conrad, B. Handjiski, L. Mecklemburg, M. Nakamura, J. Inzunza, J.-A. Gustafsson and R. Paus, Hair cycle control by estrogens: Catagen induction via estrogen receptor (ER)-α is checked by ERβ signalling, Endocrinology, 2005, 146, 1214–1225 CAS.
- M. Allanson and V. E. Reeve, Immunoprotective UVA (320–400 nm) irradiation upregulates heme oxygenase in the dermis and epidermis of hairless mouse skin, J. Invest. Dermatol., 2004, 122, 1030–1036 CrossRef CAS.
- V. E. Reeve, Ultraviolet radiation and the contact hypersensitivity reaction in mice, Methods, 2002, 28, 20–40 CrossRef CAS.
- H. S. Oh and R. C. Smart, An estrogen receptor pathway regulates the telogen-anagen hair follicle transition and influences epidermal cell proliferation, Proc. Natl. Acad. Sci. USA, 1996, 93, 12525–12530 CrossRef CAS.
- S. Chanda, C. L. Robinette, J. F. Couse and R. C. Smart, 17β-estradiol and ICI-182780 regulate the hair follicle cycle in mice through an estrogen receptor-α pathway, Am. J. Physiol. Endocrin. Metab., 200, 278, E202–210 Search PubMed.
- M. J. Thornton, A. H. Taylor, K. Mulligan, F. All-Azzawi, C. C. Lyon, J. O'Driscoll and A. G. Messenger, Oestrogen receptor beta is the predominant oestrogen receptor in human scalp, Exp. Dermatol., 2003, 12, 181–190 CrossRef CAS.
- M. J. Thornton, A. H. Taylor, K. Mulligan, F. All-Azzawi, C. C. Lyon, J. O'Driscoll and A. G. Messenger, The distribution of estrogen receptor beta is distinct to that of estrogen receptor alpha and the androgen receptor in human skin, J. Invest. Dermatol. Symp. Proc., 2003, 8, 100–103 Search PubMed.
- G. Pelletier and L. Ren, Localization of sex steroid receptors in human skin (Review), Histol. Histopath., 2004, 19, 629–636 Search PubMed.
- F. Hildebrand, W. J. Hubbard, M. A. Choudry, B. M. Thobe, H. C. Pape and I. H. Chaudry, Are the protective effects of 17beta-estradiol on splenic macrophages and splenocytes after trauma-hemorrhage mediated via estrogen-receptor (ER)-alpha or ER-beta?, J. Leukocyte Biol., 2006, 79, 1173–1180 Search PubMed.
- T. Suzuki, T. Shimizu, H. P. Yu, Y. C. Hsieh, M. A. Chaudry, M. G. Schwacha and I. H. Chaudry, Tissue compartment-specific role of estrogen receptor subtypes in immune cell cytokine production following trauma-hemorrhage, J. Appl. Physiol., 2007, 102, 163–168 CAS.
- V. E. Reeve and R. M. Tyrrell, Heme oxygenase induction mediates the photoimmunoprotective activity of UVA radiation in the mouse, Proc. Natl. Acad. Sci. USA, 1999, 96, 9317–9321 CrossRef CAS.
- T. Nakamura, G. Xi, R. F. Keep, M. Wang, S. Nagao, J. T. Hoff and Y. Hua, Effects of endogenous and exogenous estrogen on intracerebral hemorrhage-induced brain damage in rats, Acta Neurochirurgica-Suppl., 2006, 96, 218–221 Search PubMed.
- H. P. Yu, T. Shimizu, Y. C. Hsieh, T. Suzuki, M. A. Choudry, M. G. Schwacha and I. H. Chaudry, Mechanism of the salutary effects of flutamide on intestinal myeloperoxidase activity following trauma-hemorrhage: upregulation of estrogen receptor-beta-dependent HO-1, J. Leukocyte Biol., 2006, 79, 963–970 Search PubMed.
- M. Allanson, D. Domanski and V. E. Reeve, Photoimmunoprotection by UVA (320–400 nm) radiation is determined by UVA dose and is associated with cutaneous cyclic guanosine monophosphate, J. Invest. Dermatol., 2006, 126, 191–197 CrossRef CAS.
- S. Y. Lee, T. Andoh, D. L. Murphy and C. C. Chiueh, 17beta-estradiol activates ICI 182,780-sensitive estrogen receptors and cyclic GMP-dependent thioredoxin expression for neuroprotection, FASEB J., 2003, 17, 947–948 CAS.
- M. M. Montano and B. S. Katzenellenbogen, The quinone reductase gene: a unique estrogen receptor-regulated gene that is activated by antiestrogens, Proc. Natl. Acad. Sci. USA, 1997, 94, 2581–2586 CrossRef CAS.
- M. M. Montano, H. Deng, M. Liu, X. Sun and R. Singal, Transcriptional regulation by the estrogen receptor of antioxidative stress enzymes and its functional implications, Oncogene, 2004, 23, 2442–2453 CrossRef CAS.
|
This journal is © The Royal Society of Chemistry and Owner Societies 2008 |
Click here to see how this site uses Cookies. View our privacy policy here.