Photodynamic effect in medulloblastoma: downregulation of matrix metalloproteinases and human telomerase reverse transcriptase expressions
Received 6th March 2007, Accepted 12th October 2007
First published on 2nd November 2007
Abstract
Tumor invasion and immortality are the most unfavorable drawbacks after cancer treatment. In this study, we focus on determining the photodynamic modulation of the proteolytic enzymes, matrix metalloproteinases (MMP); and a core catalytic subunit of telomerase, the human telomerase reverse transcriptase (hTERT) in medulloblastoma (MED) cell line (TE-671). Hexvix (ALA-H) mediated photodynamic therapy (PDT) demonstrated greater efficacy than 5-aminolevulinic acid (5-ALA) in terms of drug uptake and anti-proliferative effect. Both MMP-2 and hTERT expression are down-regulated quantitatively using ELISA and reverse-transcriptase-PCR (RT-PCR) respectively at post-treatment for this cell line. The MMP-9 expression remains unchanged after treatment. Further, there is a statistically significant inhibition of cell migration at 24 h post-ALA-H-PDT at LD50 (0.01 mM, 2 J cm–2; p < 0.001) in MED TE-671 cells. Evidently, MMP-2 and hTERT mRNA expressions can be the targets for the photodynamic intervention on tumor cell migration and immortality. Hence, PDT may be an alternate cancer regime for medulloblastoma.
1. Introduction
Medulloblastoma (MED) is a highly aggressive form of neoplasm in the central nervous system and is the most common malignant childhood brain tumor. Since 1989, MED has represented 20 to 25% of pediatric brain tumors occurring in children between the age of 5 and 10.1,2 According to the Hong Kong Cancer Registry, brain cancer is a common cancer in children in the age group between 0 and 14 years old.3 In the past decade, medulloblastoma (MED) was the source of relatively heated debates among neuropathologists because obstructive hydrocephalus and cerebellar dysfunction always appeared in children with MED, and the treatment for them remains in flux.4 The conventional treatments for MED include surgical resection, neuraxis irradiation, craniospinal radiation, and chemotherapy. The main limitation is lack of specificity and these treatments also have a cytotoxic effect. Half of affected children survive for about 5 years after treatment.2 However, they are at risk for significant sequelae, including tumor-related and treatment-related complications. Therefore, cures are rarely achieved after recurrence.5 New advances in the improvement of existing therapy and new strategies in targeting and understanding the biology and pathogenesis of MED are important for treatment development.Photodynamic therapy (PDT) is a new strategic focal treatment targeting tumor cells. It has lately been under investigation in clinical and experimental trials for tumors. Five-aminolevulinic acid (5-ALA), ALA-hexylester (ALA-H), and Photofrin II are photosensitizing agents approved by the Food and Drug Administration (FDA) for clinical applications in the United States, Europe, and Japan.6 Upon the photoactivation of the photosensitizer (PS) with a specific wavelength of light in the presence of oxygen, the reactive oxygen species (ROS) will be generated for tumor cell disruption. Five-aminolevulinic acid (5-ALA) and its derivatives are prospective prodrugs that have been under investigation over the past decade. Their efficacy is due to protoporphyrin IX (PpIX), a metabolite in the biosynthetic pathway of a heme.7 Accumulation of PpIX inside the tumor cells will result in cellular disruption upon specific wavelengths of light irradiation. The localization of the PS varies depending on its properties. It may localize on cellular organelles including the cell membrane, mitochondria, lyzosomes, or the nucleus, triggering different modes of cell death.8–10 PDT efficacy has been demonstrated in different clinical trials of tumor patients, such as patients with pancreatic, lung, brain, and head-and-neck tumors.11–14 Thus, monitoring PDT treatment in tumor prognosis is a growing concern.
Tumor invasion is a complex process present in the tumor microenvironment. One of the critical factors for tumor invasion is the large production of proteolytic enzymes called matrix metalloproteinases (MMPs). MMPs belong to a large family of zinc- and calcium-dependent enzymes that play a vital role in several physiological processes including cell proliferation, angiogenesis, and tumor metastasis.15,16 MMPs principally participate in the invasion process via the degradation of extracellular matrix (ECM) components with other substrates such as cytokines, growth factor receptors, and cell–cell adhesion molecules. Matrix metalloproteinase-2 (MMP-2) and -9 (MMP-9) are two prevalent type IV collagenases termed gelatinase A and gelatinase B respectively. They can degrade the type IV collagen of basal laminae and other nonhelical collagen domains such as laminin.17,18 There is increasing evidence supporting the theory that MMP-2 and MMP-9 expressions are associated with disease outcomes in different cancers such as ovarian and breast tumors.19,20 It is therefore timely to explore a new treatment regime to overcome tumor metastasis that may be induced by conventional therapies.
Telomerase is a ribonucleoprotein expressed in over 85% of advanced tumors but not in most normal proliferating cells. Its activity can compensate the erosion of telomeric DNA at telomeres during each cell division, thus stabilizing the immortal state.21–23 A human telomerase reverse transcriptase (hTERT), the core catalytic subunit of mammalian telomerase holoenzyme, is a rate-limiting component of telomerase. It is a reverse transcriptase adding hexameric DNA repeats (TTAGGG) and telomerase RNA components (hTR) to provide the template sequence. The telomerase activity is correlated with the expression of hTERT and hTR mRNA, especially in immortal tumor cells.24,25 The hTERT mRNA expression level can be one of the representing markers for monitoring tumor immortality.
In this study, PDT was applied to the medulloblastoma cell line, TE-671 (MED). The optimum drug uptake, protoporphyrin IX (PpIX) formation, and the photodynamic efficacy of the two porphyrin-precursors, 5-aminolevulinic acid (5-ALA), and its derivative, ALA hexylester (ALA-H), were compared. The effect of PDT on MMP-2 and -9 expressions as well as cell migration in MED tumor cells were determined. The evaluation of the catalytic subunit of telomerase, the human telomerase reverse transcriptase (hTERT), was quantitated at the transcriptional level at pre- and post-PDT treatment. These two novel markers were first established as potential indicators in tumor invasion and immortality for PDT in medulloblastoma.
2. Materials and methods
Cell cultivation and incubation
The medulloblastoma (MED)-derived cell line TE-671 was purchased from ATCC (American Type Culture Collection, Manassas, VA). Cells were routinely cultured in monolayer in 75 cm2 tissue culture flasks (Corning, USA) with RPMI supplemented with 10% fetal bovine serum (FBS; Invitrogen, Carlsbad, CA) and antibiotics PSN (50 IU mL–1 penicillin, 50 µg mL–1 streptomycin, and 100 µg mL–1 neomycin) at 37 °C in a humidified 5% CO2 incubator. When the cell growth reached 90% confluence, the cells were harvested for PDT treatment using 0.25% trypsin–EDTA (Invitrogen, Carlsbad, CA).The 5-aminolevulinic acid (5-ALA) was from Sigma (St. Louis, MO) and the ALA hexylester (ALA-H) was kindly provided by PhotoCure (Norway). Their stock and working solutions were prepared in PBS and freshly prepared with a serum-free RPMI culture medium respectively.
Cellular uptake
The cellular uptake of 5-ALA and ALA-H was measured by determining the protoporphyrin IX (PpIX) formation in TE671 cells by use of a spectrophotometer (Tecan's Spectrafluor Plus). MED cells (3 × 104 cells/well) were seeded in a 96-well flat-bottom microtiter plate. 5-ALA (1 mM) and ALA-H (0.005 mM) were freshly prepared and incubated at various time intervals (2, 4, 8, 12, and 15 h). The drugs were then removed and the cells were centrifuged and washed twice with PBS. Methyl sulfoxide dimethylsulfoxide (DMSO; Sigma, St. Louis, MO) was then applied for half an hour for cell lysis, and in this way PpIX was released from the cells. The fluorescence intensities of PpIX were measured with an excitation wavelength at 405 nm and an emission wavelength at 635 nm using a spectrophotometer. All results were presented as the mean ± standard deviation (SD) for at least three independent experiments.Cytotoxicity
MED cells (3 × 104 cells/well) were incubated with a range concentration of either 5-ALA (0.05–0.5 mM) or ALA-H (0.005–0.04 mM) for 15 h and 8 h respectively. The MED cells were then irradiated from a 400 w quartz-halogen lamp with a heat isolation filter and a 600 nm long-pass filter at 1 J cm–2-4 J cm–2. The drug was then removed and the cells were centrifuge washed with PBS and incubated in incomplete RPMI. The cytotoxicity of the MED cells was determined after 24 h by the tetrazolium colorimetric reduction assay (MTT assay). MTT (3-[4,5-dimethylthiazol-2-yl]-2,5-diphenyltetrazolium bromide; Sigma) solution was added and the cells were further incubated for 3 h. The cells were washed with PBS and 150 µL DMSO was added to lyse the cells and release the formazan crystal. Finally, the absorbance was measured by a spectrophotometer at a wavelength of 570 nm. All results were presented as the mean ± standard deviation (SD) in triplicate.Quantitation of matrix metalloproteinase-2 and -9 (MMP-2 and -9) expressions
The MED cells (2 × 104 cells) were seeded and treated with 5-ALA and ALA-H at LD10 and LD30. Twenty-four hours post-PDT treatment, the medium was collected for the determination of MMP-2 and MMP-9. Both MMP-2 and MMP-9 were quantitated by MMP-2 and MMP-9 ELISA assays as instructed (Oncogene Research Products, Boston, USA). These assays were based on a sandwich enzyme immunoassay principle with an antibody specific to either human MMP-2 or MMP-9 proteins coated onto the microtiter plate. The tested PDT controls and treated media and the conjugated antibody were added to the plate and incubated for 2 h. MMP-2 and MMP-9 proteins present in the treated cell media were captured by the detecting antibodies. Unbound proteins were washed away and horseradish peroxidase-conjugated streptavidin was added to bind the detecting antibodies. The color developed was detected by a spectrophotometer at specific dual wavelengths. The absorbance was proportional to the amount of MMP-2 or MMP-9 proteins present in the tested samples and the concentration (ng mL–1) of the MMP-2 and MMP-9 was expressed as per 2 × 104 cells per sample.Evaluation of hTERT mRNA expression by reverse transcription-PCR
A reverse transcriptase-polymerase chain reaction (RT-PCR) was applied for hTERT (GenBank accession no. AY007685.1) detection. MED tumor cells (1 × 106 cells) were first seeded in 35 mm culture dishes and incubated with 5-ALA and ALA-H until LD30 and LD50 were achieved after light irradiation. The treated cells and untreated controls were harvested at 24 h post-PDT and the total RNA of each sample was then extracted by an RNA extraction kit (Roche Diagnostics, Switzerland). The isolated RNA from the samples were quantified at an optimal density of 260 nm using a spectrophotometer (Perkin Elmer MBA 2000). Two µg of the extracted RNA from each treated and control cell were used to reverse transcribe into cDNA using RT-PCR. Random primer (Invitrogen, USA), Moloney Murine Leukemia Virus Reverse Transcriptase RNase, H Minus, point mutation (M-MLV RT(H–)) (Promega), and RNA template were added. The synthesized cDNA were further amplified with a specific hTERT forward primer (5′-TTT CTA CCG GAA GAG TGT CTG-3′) and a reverse primer (5′-CGT AGT CCA TGT TCA CAA TCG-3′) with a product size of 196 bp. A primer pair for GAPDH was added as a housekeeping gene of internal control (forward: 5′-CTC ATC ATC TCT GCC CCC CTC CTG-3′ and reverse: 5′-CGC CTG CTT CAC CAC CTT CTTG-3′) that could synthesize a product size of 450 bp. The final amplified products were fractionated on 1.5% agarose gel by electrophoresis. Ethidium bromide was used to stain the gel and the gel images were captured under UV excitation by a Lumi-imager F1 workstation (Roche Diagnostics, Switzerland). The density of the RT-PCR products was presented in terms of the density unit (BLU).Determination of cell migration at pre- and post-PDT
The cell migration was determined by wound-healing assay.26,27 The MED cells (3 × 104) were seeded in 96-well plates overnight at 37 °C with 5% CO2. After the cells had reached confluency, a wound was made with a 200 µl pipette tip. The detached cells and debris were removed and washed with PBS. The cells were then treated with 5-ALA and ALA-H at LD30 and LD50; control cells and dark controls were included. The closure of the wound by migrating cells was determined at 15 and 24 h post-PDT using a Leica inverted-phase contrast microscope (Leica DMI 4000B) coupled with a CCD camera and quantified by NIH Image Software. The dose-dependent effect of the PSs on wounded TE-671 cells was as a migration rate compared with the untreated cells as the control and the data were analyzed by two-way ANOVA.Statistical analysis
The data presented were analyzed using GraphPad Prism 4 (GraphPad Software, Inc., San Diego, CA) and were performed in triplicate independent experiments.3. Results
Cellular uptake and protoporphyrin IX (PpIX) formation
The uptake kinetics of 5-ALA (1 mM) and ALA-H (0.005 mM) on MED cells at various times from 2 to 15 h was determined by the amount of induced protoporphyrin IX (PpIX) formation. The fluorescence intensity after treatment with ALA-H and 5-ALA in MED cells is shown in Fig. 1A and 1B. The results show that ALA-H had a significantly higher formation of PpIX at 2 h when compared to the cells treated with 5-ALA. ALA-H (0.005 mM) was about 200 times more efficient in PpIX formation than 5-ALA (1 mM). Therefore, ALA-H is a more effective photosensitizer in generating PpIX than 5-ALA in the MED cells. The 15 h and 8 h were chosen as the incubation time for the cellular uptake of 5-ALA and ALA-H by MED cells respectively because LD80 was achieved for either photosensitizer.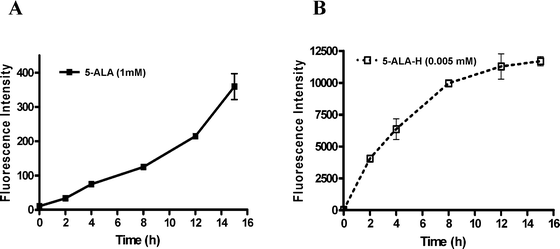 |
| Fig. 1 Cellular uptake of (A) 5-ALA (1 mM; p < 0.05) and (B) ALA-H (0.005 mM; p < 0.0004) mediated PpIX formations by MED cell lines at 2, 4, 8, 12, and 15 h incubation. The fluorescence intensity obtained by spectrophotometer was proportional to the PpIX formed in MED cells. Error bars (SD) obtained from three independent experiments. | |
Photodynamic efficacy
The results of the photodynamic effect of 5-ALA and ALA-H on MED cells at the uptake time (15 h and 8 h respectively) are presented in Fig. 2A and 2B. The dark toxicity was negligible for either drug. In Fig. 2A, as the 5-ALA concentration (0.05–0.5 mM) increased, the cytotoxicity of the MED cells also increased in all three sets of light–dose treatment (1, 2 and 4 J cm–2). In Fig. 2B, a similar cytotoxic pattern was obtained when ALA-H was applied. After 15 h incubation of 5-ALA, LD80 was obtained at 0.5 mM, 2 J cm–2. Similarly, after 8 h incubation of ALA-H, LD80 was achieved at 0.04 mM, 4 J cm–2. As a result, a shorter incubation time and lower drug concentration of ALA-H applied could achieve the same lethal dose in MED cells when compared with the longer incubation time and higher concentration of 5-ALA. The results show that the phototoxicity of both drugs was positively related to both drug concentrations and light doses in the MED cell line. It also implied that a much lower concentration of ALA-H could generate an ample amount of PpIX for MED cell destruction upon light irradiation.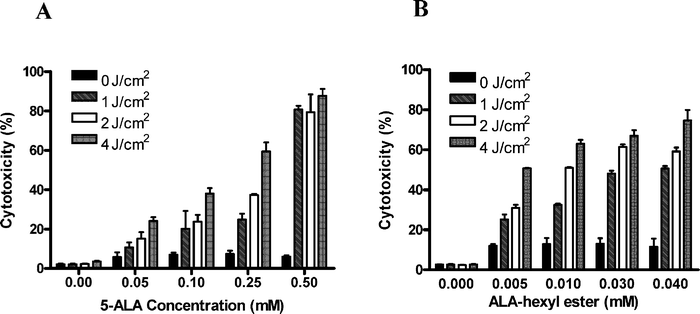 |
| Fig. 2 Photodynamic effect of (A) 5-ALA and (B) ALA-H in MED cells. MED cells were incubated with various concentrations of 5-ALA (0.05–0.5 mM) for 15 h or ALA-H (0.005–0.04 mM) for 8 h, then irradiated with different light doses (1–4 J cm–2). MTT was performed at 24 h after light irradiation to determine cell death. Error bars (SD) were obtained in triplicate independent experiments. | |
Downregulation of MMP-2 mediated by 5-ALA- and ALA-H-PDT
In order to investigate the anti-metastatic effect of 5-ALA- and ALA-H-mediated PDT in MED cells, MMP-2 and MMP-9 were applied as indicators. At LD10 and LD30 of 5-ALA and ALA-H, the MMP-2 expression of MED cells was significantly reduced, as quantitated by MMP-2 ELISA assay, when compared with the neat and dark controls (Fig. 3). The MMP-2 released by MED cells after either 5-ALA or ALA-H-PDT treatment was only reduced to a lesser extent, but the reduction was statistically significant when compared with the untreated control cells. Also, no MMP-9 was determined in the control and no MMP-9 expression induced in the MED cells after 5-ALA or ALA-H treatment (data not shown). The results suggest that the MMP-2 expression was lowered by the treatment of 5-ALA- and ALA-H-PDT in MED cells, but that the treatment had no effect on MMP-9 expression.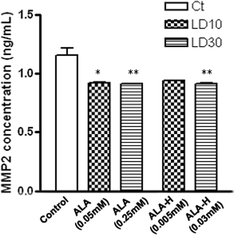 |
| Fig. 3 Downregulation of MMP-2 expression by PDT treatment of MED cells. The expression of MMP-2 in MED cells post-PDT treatment of 5-ALA or ALA-H at LD10 and LD30 were downregulated and the results were all statistically significant compared with control. All the data were presented in triplicate independent experiments. (A) 5-ALA loaded cells (*, p < 0.05; **, p < 0.01); (B) ALA-H loaded cells (**, p < 0.01). | |
Downregulation hTERT mRNA expression after PDT
MED cells were treated either with 5-ALA or ALA-H at the PDT dose of LD30 or LD50. Control cells (without drug or light treatment) were included. The gel images of 5-ALA- (data not shown) and ALA-H-(Fig. 4A) treated PCR products were visualized and presented. The mRNA PCR products of GAPDH and hTERT were 450 bp and 196 bp respectively. The primers of GAPDH acted as an internal control. When comparing the controls (Ctl) of GAPDH and hTERT products, the intensity of GAPDH products were found to be similar at LD30 and LD50; whereas the intensity of the amplified hTERT mRNA products with the same PDT doses gradually diminished in a dose-dependent manner for both 5-ALA and ALA-H (Fig. 4B). The percentage differences in the intensities of 5-ALA-treated hTERT mRNA products were 9.1% and 14% at LD30 and LD50 respectively. On the other hand, the percentage differences of the ALA-H-treated products were further downregulated to the greater extent of 16.5% and 29.8% at LD30 and LD50 respectively. The results indicated that ALA-H-PDT inhibited the hTERT mRNA expression in MED cells, implying that ALA-H-PDT possibly inhibits the migration ability of MED cells.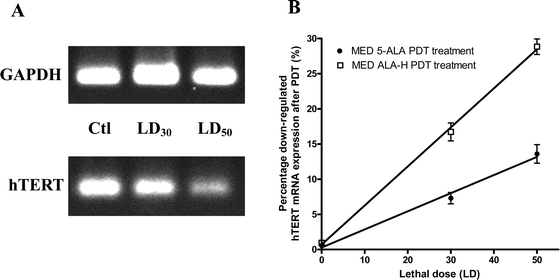 |
| Fig. 4 The hTERT mRNA expression was down regulated post-5-ALA and ALA-H PDT. The MED cells were treated with 5-ALA (data not shown) or ALA-H at dosages of LD30 and LD50. The hTERT and GAPDH mRNA PCR products were obtained from the treated cells. Since the housekeeping gene GAPDH acted as an internal control, its expression level was not affected under therapeutic conditions. When compared with the neat control (Ctl) and GAPDH from the gel image, the percentage of the intensity was decreased significantly. (A) Amplified PCR products from ALA-H-PDT treated cells. (B) The percentage differences in the intensity of the amplified hTERT products after ALA-H-PDT or 5-ALA-PDT at LD30 and LD50 were significantly downregulated in a dose-dependent manner. Results were obtained from four individual experiments. | |
Although there was only a slight downregulation of MMP-2 by both 5-ALA and ALA-H-PDT in MED cells and hTERT mRNA expression was reduced after PDT as well, further investigation of the modulation of cell migration after PDT in MED cells was evaluated. The typical inhibition in cell migration after ALA-H-PDT at LD30 and LD50 is shown in Fig. 5. In Table 1, for the MED cells treated with ALA-H at LD50, there was about 50% of inhibition in cell migration at 24 h post-ALA-H-PDT (p < 0.001). Only about 30% inhibition of cell migration was obtained at LD50 of 5-ALA-treated MED cells (p < 0.01). Our findings suggested that the cell migration was inhibited by both 5-ALA and ALA-H-PDT which correlated with the downregulation in MMP-2 and hTERT expression.
Table 1 Migration rate of MED cells treated with 5-ALA and ALA-H-PDTa
Samples | Migration area at different intervals/mm2 |
---|
0 h pretreatment | 24 h post-PDT | Migration rate (%) |
---|
Mean | SD | Mean | SD | |
---|
The cell migration rate was measured at pre- and post-PDT treatment by the NIH software. The migration area for each group of PS-treated cells was monitored at 24 h. Values are means with their standard deviation from three separate experiments. Migration rate of each treatment is expressed as a percentage of the control. The significant difference between means (two-way ANOVA): *, p < 0.01, **, p < 0.001 with regard to control values. |
---|
ALA-H |
Control | 86.5 | 20.3 | 164.1 | 13.5 | — |
LD30 | 74.5 | 13.2 | 134.1 | 12.1 | 76.8**b |
LD50 | 82.9 | 13.87 | 124.3 | 9.4 | 53.4**b |
|
5-ALA |
Control | 72.7 | 10.2 | 147.1 | 17.2 | — |
LD30 | 71.0 | 10.1 | 132.4 | 8.8 | 82.5 |
LD50 | 67.8 | 7.0 | 122.0 | 4.6 | 72.88*b |
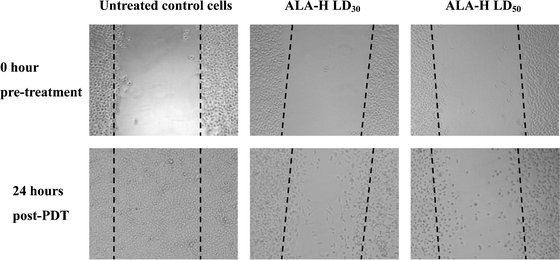 |
| Fig. 5 The typical inhibition of cell migration of MED cells treated with ALA-H-PDT. A wound was made to the confluent cells. Images were taken at pre- and post-PDT under phase-contrast microscopy. The images of the untreated control cells indicated the wound was healed normally after 24 h incubation. For the cells treated with ALA-H at LD30 and LD50, only a small amount of cell migrated from the wounded area. At 24 h post ALA-H-PDT, the cells treated at LD30 still strongly attached to the well plate; whereas most of the cells treated at LD50 became loosely attached and floating nucleus resulted in less invasive. | |
4. Discussion
In summary, our results reveal an effective and responsive PDT treatment of a medulloblastoma cell line (TE-671) with 5-ALA or ALA hexylester (ALA-H). Encouraging evidence is shown in this study that ALA hexylester is more powerful than 5-ALA in inhibiting the progression of MED (TE-671) cells in vitro.Studies have demonstrated that 5-ALA is effective for tumor cell disruption in, for example, bladder cancer, nasopharyngeal carcinoma and glioma cells.28–30 In order to improve the efficacy of 5-ALA, modified 5-ALA derivatives with improved efficiency were synthesized. They are of a long lipophilic chain and thus offer better penetration into cytoplasm. Extra exogenous 5-ALA or ALA esters can also enhance the formation and accumulation of PpIX in both in vivo and in vitro models.31,32 Studies comparing the efficacy of 5-ALA and its ester concluded that, at the same drug concentration, these 5-ALA esters are more powerful than 5-ALA, with higher PpIX formation in tumor cells.33,34 Lately, our group has also demonstrated that 5-ALA and ALA-H do not cause severe phototoxicity at low dose on human lymphocytes35 and ALA-H has a greater photo-killing ability with nasopharyngeal carcinoma cells.36 In this study, we have demonstrated that ALA-H generated a higher amount of PpIX than 5-ALA in MED cells.
Brain tumors are difficult to treat and treatment is highly invasive to nearby normal brain cells, especially after conventional treatment. Medulloblastoma (MED) is a prevalent childhood brain tumor, usually with a high recurrence rate after conventional treatment. Due to its location and invasion features, children with MED always present with obstructive hydrocephalus and cerebellar dysfunction.4,37
In recent studies, it is reported that tumor metastasis is directly related to the secretion of matrix metalloproteinases (MMPs) by tumor cells. MMPs are regulated at transcriptional levels by the modulation of zymogens and tissue inhibitors of metalloproteinases (TIMPs) under normal conditions.38,39 Therefore, an imbalance between the expression of MMPs and their inhibition by TIMPs can result in pathological abnormalities, particularly the degradation of the extracellular matrix (ECM) and the invasive potential of tumors.14,40 Hence, maintenance of the balance among them, especially in tumors, is essential. Among the MMPs, MMP-2 and MMP-9 are two metastatic proteins of gelatinases that can degrade the basement membrane of endothelial cells. When the degradation occurs, tumor cells can invade and metastasize to various sites.41 Studies have already reported that expressions of MMP-2 or MMP-9 or both are closely associated with tumor progression in several medulloblastoma cell lines in vitro.42,43 Forsyth et al. reported that the overexpression of MMP-2 and MMP-9 in glioblastoma cells correlated with tumor invasiveness.44 This implies that when the expression of MMP-2 or MMP-9 or both decreases after treatment, then the treatment can be considered effective in preventing tumor-cell metastasis and invasion. Recent reports also claimed that PDT mediated different MMP expressions. Karrer et al., demonstrated that 5-ALA-PDT induced MMP-1 and MMP-3 expressions in dermal fibroblasts for collagen degradation;45 whereas Takahashi et al. also revealed similar results, showing that 5-ALA-PDT mediated MMP-1 and MMP-3 expression, but not MMP-2 in fibroblasts from scleroderma patients.46 The treatment of 5-ALA seems to trigger the MMPs for collagen degradation. On the other hand, Au et al. have recently demonstrated that 5-ALA-PDT reduced the MMP expression of glioma cells and possibly diminished the invasive ability to degrade the ECM after PDT.30 The 5-ALA-PDT-mediated decrease in MMP-2 or MMP-9 expression, or both, in glioma cells prompted us to investigate the exogenous expression of these two matrix metalloproteinases, MMP-2 and MMP-9, versus the antimetastatic behavior after 5-ALA/ALA-H-mediated PDT in MED cells.
In order to evaluate the antimetastatic ability of 5-ALA- and ALA-H-mediated PDT on MED tumor cells, we have quantitated the amount of MMP-2 and MMP-9 expression at pre- and post-PDT treatment on MED (TE-671) cells. Results showed that the expression of MMP-2 was significantly downregulated after 5-ALA- or ALA-H-PDT treatment (Fig. 3) and this strongly suggested that PDT might diminish the invasive ability of MED cells. It was confirmed by the wound-healing assay that the migration rate of MED cells decreased after 5-ALA- and ALA-H-PDT (Fig. 5 and Table 1). There was only a 30% inhibition of the cell migration after 5-ALA-PDT in MED cells, which implied that MED cells have greater invasion ability than that in 5-ALA-PDT-treated glioma cells.30 On the other hand, ALA-H-PDT inhibited about 50% of cell migration in MED cells, indicating that ALA-H is a more potent photosensitizer than 5-ALA. Interestingly, there was no MMP-9 expression in MED (TE-671) cells at pre- and post-PDT (data not shown), although it was found to be expressed in various medulloblastoma cell lines.42
Human telomerase reverse transcriptase (hTERT)—the catalytic subunit of telomerase—is another therapeutic target that is involved in tumor cell immortalization, proliferation, and transformation.48–50 Particularly in malignant transformation, telomerase functions to synthesize telomeric sequences of telomere in order to protect the chromosomes from damage and degradation. Studies have demonstrated that hTERT transcriptional expression is closely related to the telomerase activity in tumors such as prostate and breast carcinomas.51,52 Until now, no scientific literatures have reported that PDT can modulate hTERT expression. We previously demonstrated that ALA-H-PDT mediated the reduction of MMP-2 and hTERT expression in nasopharyngeal carcinoma cells.47 Hence, we applied PDT to MED cells and investigated for the modulation of hTERT mRNA levels mediated by PDT. Our results demonstrated that there was a significant downregulation of hTERT mRNA expression after 5-ALA or ALA-H-PDT in MED cells (Fig. 4A and 4B). The diminished intensity strongly suggested that ALA-H-PDT in MED cells was more effective in inhibiting the expression of hTERT mRNA at transcriptional level than 5-ALA, and the decreased hTERT mRNA expression in PDT-treated MED cells indicated an inhibition of cell proliferation (Fig. 2A and 2B) and migration (Fig. 5). Thus, the inhibited cell proliferation and migration in turn reduces the telomerase activity and invasive ability. It is promising that hTERT transcriptional expression can be of significance as a target with therapeutic regimes for medulloblastoma patients, and perhaps other tumors as well.
In summary, this is the first report on the downregulated expressions of MMPs and hTERT for PDT efficacy in medulloblastoma cells. These imply that MMP-2 and hTERT might be essential indicators and potential targets for tumor cell migration and treatment monitoring. Thus, further clinical investigations concerning PDT in targeting and understanding the biology and pathogenesis of tumor in patients are deserved explorations.
5. Abbreviations
Acknowledgements
We would like to thank the Competitive Earmarked Research Grant (CERG) of Hong Kong for supporting this study (PolyU.5404/04 M).References
- D. S. Russell and L. J. Rubinstein, Pathology of tumours of the nervous system, Williams & Wilkins, Baltimore, 5th edn, 1989, pp. 83–448 Search PubMed.
- S. Marino, Medulloblastoma: developmental mechanisms out of control, Trends Mol. Med., 2005, 11, 17–22 CrossRef CAS.
- New Cases Registered of Childhood and Adolescent Cancer in 2003, Hong Kong Cancer Registry, Hong Kong Hospital Authority.
- H. T. Whelan, H. G. Krouwer, M. H. Schmidt, K. W. Reichert and E. H. Kovnar, Current therapy and new perspectives in the treatment of medulloblastoma, Pediatr. Neurol., 1998, 18, 103–115 CrossRef CAS.
- M. Chintagumpala, S. Berg and S. M. Blaney, Treatment controversies in medulloblastoma, Curr. Opin. Oncol., 2001, 13, 154–159 CrossRef CAS.
- C. H. Sibata, V. C. Colussi, N. L. Oleinick and T. J. Kinsella, Photodynamic therapy in oncology, Expert Opin. Pharmacother., 2001, 2, 917–927 Search PubMed.
- H. Fukuda, A. Casas and A. Batlle, Aminolevulinic acid: from its unique biological function to its star role in photodynamic therapy, Int. J. Biochem. Cell Biol., 2005, 37, 272–276 CrossRef CAS.
- C. M. N. Yow, N. K. Mak, S. Szeto, J. Y. Chen, Y. L. Lee, N. H. Cheung, D. P. Huang and A. W. N. Leung, Photocytotoxic and DNA damaging effect of temoporfin (mTHPC) and merocyanine 540 (MC540) on nasopharygeal carcinoma cell, Toxicol. Lett., 2000, 115, 53–61 CrossRef CAS.
- W. N. Leung, X. Sun, N. K. Mak and C. M. N. Yow, Photodynamic effects of mTHPC on human colon adenocarcinoma cells: photocytotoxicity, subcellular localization and apoptosis, Photochem. Photobiol., 2002, 75, 406–411 CrossRef CAS.
- F. Rancan, A. Wiehe, M. Nobel, M. O. Senge, S. A. Omari, F. Bohm, M. John and B. Roder, Influence of substitutions on asymmetric dihydroxychlorins with regard to intracellular uptake, subcellular localization and photosensitization of Jurkat cells, J. Photochem. Photobiol., B, 2005, 78, 17–28 CrossRef CAS.
- P. J. Lou, L. Jones and C. Hooper, Clinical outcomes of photodynamic therapy for head-and-neck cancer, Technol. Cancer Res. Treat., 2003, 2, 311–317 Search PubMed.
- H. Kato, M. Harada, S. Ichinose, J. Usuda, T. Tsuchida and T. Okunaka, Photodynamic therapy (PDT) of lung cancer: experience of the Tokyo Medical University, Photodiagn. Photodyn. Ther., 2004, 1, 49–55 Search PubMed.
- S. S. Stylli, M. Howes, L. MacGregor, P. Rajendra and A. H. Kaye, Photodynamic therapy of brain tumors: evaluation of porphyrin uptake versus clinical outcome, J. Clin. Neurosci., 2004, 11, 584–596 CrossRef CAS.
- L. Ayaru, S. G. Bown and S. P. Pereira, Photodynamic therapy for pancreatic and biliary tract carcinoma, Int. J. Gastrointest. Cancer, 2005, 35, 1–14 Search PubMed.
- Z. Kachra, E. Beaulieu, L. Delbecchi, N. Mousseau, F. Berthelet, R. Moumdjian, R. Del. Maestro and R. Beliveau, Expression of matrix metalloproteinases and their inhibitors in human brain tumors, Clin. Exp. Metastas., 1999, 17, 555–566 Search PubMed.
- D. Ribatti, G. Surico, A. Vacca, F. De Leonardis, G. Lastilla, P. G. Montaldo, N. Rigillo and M. Ponzoni, Angiogenesis extent and expression of matrix metalloproteinase-2 and -9 correlate with progression in human neuroblastoma, Life Sci., 2001, 68, 1161–1168 CrossRef CAS.
- A. F. Chambers and L. M. Matrisian, Changing views of the role of matrix metalloproteinases in metastasis, J. Natl. Cancer Inst., 1997, 89, 1260–1270 CrossRef CAS.
- X. Xu, Y. Wang, Z. Chen, M. D. Sternlicht, M. Hidalgo and B. Steffensen, Matrix metalloproteinase-2 contributes to cancer cell migration on collagen, Cancer Res., 2005, 65, 130–136 CAS.
- B. Davidson, I. Goldberg, W. H. Gotlieb, J. Kopolovic, G. Ben-Baruch, J. M. Nesland, A. Berner, M. Bryne and R. Reich, High levels of MMP-2, MMP-9, MT1-MMP and TIMP-2 mRNA correlate with poor survival in ovarian carcinoma, Clin. Exp. Metastas., 1999, 17, 799–808 Search PubMed.
- T. Yoneda, A. Sasaki, C. Dunstan, P. J. Williams, F. Bauss, Y. A. De Clerck and G. R. Mundy, Inhibition of osteolytic bone metastasis of breast cancer by combined treatment with the bisphosphonate ibandronate and tissue inhibitor of the matrix metalloproteinase-2, J. Clin. Invest., 1997, 99, 2509–2517 CrossRef CAS.
- N. W. Kim, M. A. Piatyszek, K. R. Prowse, C. B. Harley, M. D. West, P. L. Ho, G. M. Coviello, W. E. Wright, S. L. Weinrich and J. W. Shay, Specific association of human telomerase activity with immortal cells and cancer, Science, 1994, 266, 2011–2015 CrossRef CAS.
- M. N. Helder, G. B. A. Wisman and A. G. J. van der Zee, Telomerase and telomeres: from basic biology to cancer treatment, Cancer Invest., 2002, 20, 82–101 CrossRef CAS.
- Y. S. Cong, W. E. Wright and J. W. Shay, Human telomerase and its regulation, Microbiol. Mol. Biol. Rev., 2002, 66, 407–425 CrossRef CAS.
- W. Yasui, H. Tahara, E. Tahara, J. Fujimoto, J. Nakayama, F. Ishikawa, T. Ide and E. Tahara, Expression of telomerase catalytic component, telomerase reverse transcriptase, in human gastric carcinomas, Jpn. J. Cancer Res., 1998, 89, 1099–1103 CAS.
- K. Ohuchida, K. Mizumoto, Y. Ogura, N. Ishikawa, E. Nagai, K. Yamaguchi and M. Tanaka, Quantitative assessment of telomerase activity and human telomerase reverse transcriptase messenger RNA levels in pancreatic juice samples for the diagnosis of pancreatic cancer, Clin. Cancer Res., 2005, 11, 2285–2292 CrossRef CAS.
- Y. Li, L. Li, T. J. Brown and P. Heldin, Silencing of hyaluronan synthase 2 suppresses the malignant phenotype of invasive breast cancer cells, Int. J. Cancer, 2007, 120, 2557–2567 CrossRef CAS.
- L. Liu, L. Han, D. Y. Wong, P. Y. Yue, W. Y. Ha, Y. H. Hu, P. X. Wang and R. N. Wong, Effects of Si-Jun-Zi decoction polysaccharides on cell migration and gene expression in wounded rat intestinal epithelial cells, Br. J. Nutr., 2005, 93, 21–29 CrossRef CAS.
- P. Jichlinski and H. J. Leisinger, Photodynamic therapy in superficial bladder cancer: past, present and future, Urol. Res., 2001, 29, 396–405 CrossRef CAS.
- C. M. N. Yow, J. Y. Chen, N. K. Mak, N. H. Cheung and A. W. N. Leung, Cellular uptake, subcellular localization and photodamaging effect of temoporfin (mTHPC) in nasopharyngeal carcinoma cells: comparison with hematoporphyrin derivative (HPD), Cancer Lett., 2000, 157, 123–131 CrossRef CAS.
- C. M. Au, S. K. Luk, C. J. Jackson, H. K. Ng, C. M. N. Yow and T. S. S. To, Differential effects of photofrin, 5-aminolevulinic acid and calphostin C on glioma cells, J. Photochem. Photobiol., B, 2006, 85, 92–101 CrossRef CAS.
- A. Juzeniene, P. Juzenas, V. Iani and J. Moan, Topical application of 5-aminolevulinic acid and its methylester, hexylester and octylester derivatives: considerations for dosimetry in mouse skin model, Photochem. Photobiol., 2002, 76, 329–34 CrossRef CAS.
- S. L. Gibson, M. L. Nguyen, J. J. Havens, A. Barbarin and R. Hilf, Relationship of delta-aminolevulinic acid-induced protoporphyrin IX levels to mitochondrial content in neoplastic cells in vitro, Biochem. Biophys. Res. Commun., 1999, 265, 315–321 CrossRef CAS.
- H. Brunner, F. Hausmann, R. C. Krieg, E. Endlicher, J. Scholmerich, R. Knuechel and H. Messmann, The
effects of 5-aminolevulinic acid esters on protoprophyrin IX production in human adenocarcinoma cell lines, Photochem. Photobiol., 2001, 74, 617–623 CrossRef.
- R. F. Turchiello, F. C. Vena, P. Maillard, C. S. Souza, M. V. Bentley and A. C. Tedesco, Cubic phase gel as a drug delivery system for topical application of 5-ALA, its ester derivatives and m-THPC in photodynamic therapy (PDT), J. Photochem. Photobiol., B, 2003, 70, 1–6 CrossRef CAS.
- E. S. M. Chu, R. W. K. Wu, C. M. N. Yow, T. K. S. Wong and J. Y. Chen, The cytotoxic and genotoxic potential of 5-aminolevulinic acid on lymphocytes: a comet assay study, Cancer Chemother. Pharmacol., 2006, 58, 408–414 CrossRef CAS.
- R. W. K. Wu, E. S. M. Chu, C. M. N. Yow and J. Y. Chen, Photodynamic effects on nasopharyngeal carcinoma (NPC) cells with 5-aminolevulinic acid or its hexyl ester, Cancer Lett., 2006, 242, 112–119 CrossRef CAS.
- P. G. Fisher, P. C. Burger and C. G. Eberhart, Biological risk stratification of Medulloblastoma: the real time is now, J. Clin. Oncol., 2004, 22, 971–974 CrossRef CAS.
- H. K. Rooprai and D. McCormick, Proteinases and their inhibitors in human brain tumours: a review, Anticancer Res., 1997, 17, 4151–4162 CAS.
- M. D. Sternlicht and Z. Werb, How matrix metalloproteinases regulate cell behaviour, Annu. Rev. Cell Dev. Biol., 2001, 17, 463–516 CrossRef CAS.
- M. Nakada, D. Kita, K. Futami, J. Yamashita, N. Fujimoto, H. Sato and Y. Okada, Roles of membrane type 1 matrix metalloproteinase and tissue inhibitor of metalloproteinases-2 in invasion and dissemination of human malignant glioma, J. Neurosurg., 2001, 94, 464–473 CrossRef CAS.
- S. Curran and G. I. Murray, Matrix metalloproteinases in tumor invasion and metastasis, J. Pathol., 1999, 189, 300–308 CrossRef CAS.
- G. H. Vince, C. Herbold, R. Klein, J. Kuhl, T. Pietsch, S. Franz, K. Roosen and J. C. Tonn, Medulloblastoma displays distinct regional matrix metalloprotease expression, J. Neuro-Oncol., 2001, 53, 99–106 CrossRef CAS.
- O. Ozen, B. Krebs, B. Hemmerlein, A. Pekrun, H. Kretzschmar and J. Herms, Expression of matrix metalloproteinases and their inhibitors in medulloblastomas and their prognostic relevance, Clin. Cancer Res., 2004, 10, 4746–4753 CrossRef.
- P. A. Forsyth, H. Wong, T. D. Laing, N. B. Rewcastle, D. G. Morris, H. Muzik, K. J. Leco, R. N. Johnston, P. M. A. Brasher, G. Sutherland and D. R. Edward, Gelatinase-A (MMP-2), gelatinase-B (MMP-9) and membrane type matrix metalloproteinase-1 (MT-MMP) are involved in different aspects of the pathophysiology of malignant gliomas, Br. J. Cancer, 1999, 79, 1828–1835 CrossRef CAS.
- S. Karrer, A. K. Bosserhoff, P. Weiderer, M. Landthaler and R. M. Szeimies, Keratinocyte-derived cytokines after photodynamic therapy and their paracrine induction of matrix metalloproteinases in fibroblasts, Br. J. Dermatol., 2004, 151, 776–783 CrossRef CAS.
- H. Takahashi, S. Komatsu, M. Ibe, A. Ishida-Yamamoto, S. Nakajima, I. Sakata and H. Iizuka, ATX-S10(Na)-PDT shows more potent effect on collagen metabolism of human normal and scleroderma dermal fibroblasts than ALA-PDT, Arch. Dermatol. Res., 2006, 298, 257–263 CrossRef CAS.
- C. M. N. Yow, E. S. M. Chu and T. K. S. Wong, Photodynamic therapy (PDT)-regulation of human telomerase: reverse transcriptase (hTERT) and matrix metalloproteinase (MMP-2) in human cancer cells, Proc. Am. Assoc. Cancer Res., 2005, 46, 283 Search PubMed.
- L. Hayflick and P. S. Moorhead, The serial cultivation of human diploid cell strains, Exp. Cell Res., 1961, 25, 585–621 CrossRef.
- C. P. Chiu and C. B. Harley, Replicative senescence and cell immortality: the role of telomeres and telomerase, Proc. Soc. Exp. Biol. Med., 1997, 214, 99–106 Search PubMed.
- V. Urquidi, D. Tarin and S. Goodison, Role of telomerase in cell senescence and oncogenesis, Annu. Rev. Med., 2000, 51, 65–79 CrossRef CAS.
- A. Schindler, U. Fiedler, A. Meye, U. Schmidt, S. Fussel, C. Pilarsky, J. Herrmann and M. P. Wirth, Human telomerase reverse transcriptase antisense treatment down-regulates the viability of prostate cancer cells in vitro, Int. J. Oncol., 2001, 19, 25–30 CAS.
- M. M. Leon-Blanco, J. M. Guerrero, R. J. Reiter and D. Pozo, RNA expression of human telomerase subunits TR and TERT is differentially affected by melatonin receptor agonists in the MCF-7 tumor cell line, Cancer Lett., 2004, 216, 73–80 CrossRef CAS.
|
This journal is © The Royal Society of Chemistry and Owner Societies 2008 |