Aryl triflates: useful coupling partners for the direct arylation of heteroaryl derivatives via Pd-catalyzed C–H activation–functionalization†
Received
3rd October 2007
, Accepted 31st October 2007
First published on 21st November 2007
Abstract
Aryl triflates were found to be suitable partners for the palladium catalyzed direct arylation of heteroaromatics via C–H activation–functionalization. The reaction conditions and the catalyst have a determining influence on the yields. The system combining PPh3 and Pd(OAc)2 using KOAc as base and DMF as solvent promotes the selective 2- or 5-arylations in moderate to high yields. Several heteroaromatics such as furan, thiophene, thiazole or oxazole derivatives have been employed successfully. The electronic properties of the aryl triflates also have a decisive influence on the yields of the coupling products. Electron-rich aryl triflates gave satisfactory results, whereas the electron-poor ones led to the formation of phenols .
Introduction
Heterobiaryls are important building blocks in organic synthesis due to their biological properties. The coupling reaction of aryl triflates with heteroaryl halides provides an efficient method for the preparation of these compounds.1 One of the classical methods to perform this coupling reaction is to employ an aryl triflate with an organometallic heteroaryl derivative using a palladium catalyst (Scheme 1). However, these reactions require the preparation of organometallic derivatives such as ArZnX,2,3 ArSnR34–6 or ArB(OR)27–9 and provide either an organometallic or a salt (MX) as by-product.
 |
| Scheme 1 | |
Since the discovery by Ohta and co-workers of the direct coupling of aryl halides with heteroaryl derivatives via a C–H bond activation,10 very exciting results for the coupling of a variety of heteroaryl derivatives with aryl iodides, bromides and even chlorides have been reported by several groups.11 On the other hand, the direct coupling of aromatics12,13 and especially heteroaromatics14,15 with aryl triflates via C–H activation–functionalization has attracted less attention. Such coupling reactions would provide an attractive procedure for the preparation of these compounds due to the very wide availability, low cost and protection ability of phenols and also to the common use of protected phenols in total synthesis. Moreover, the phenolic group can be used as a means to introduce the desired functionality in the aromatic ring and then be converted into a carbon–carbon bond via the corresponding triflate.
To our knowledge, very few examples of couplings of heteroaromatics with aryl triflates have been reported so far. This reaction was described in 1995 by Proudfoot et al. using Pd(PPh3)4 as catalyst.14a The coupling reaction of a functionalized aryl triflate with oxazole gave the 5-arylated oxazole in 34% yield. A few years later, Miura and co-workers reported the 3-arylation of 2-thiophene carboxamide with phenyl triflate in 71% yield using P(o-biphenyl)(t-Bu)2–Pd(OAc)2 as catalyst and Cs2CO3 as base.14b The 5-arylation of 4-methylthiazole using phenyl triflate in the presence of Pd(OAc)2 has also been described.14c Finally, an intramolecular version of this reaction has been reported.15
Although a few examples of Pd catalyzed arylation of heteroaromatics using aryl triflates in moderate to high yields have been described, to the best of our knowledge, the influence of the reaction conditions, palladium source and ligand and also the effect of the nature of the heteroaromatic derivative and of the substituents on aryl triflates have not been reported. Therefore, the discovery of an effective and selective procedure allowing high yields of arylation products for the coupling of a variety of these challenging substrates is still subject to significant improvement.
Results and discussion
Our first goal was to determine the most suitable reaction conditions for the coupling of phenyl triflate with 2-n-butylfuran (Scheme 3,Table 1). We observed that the reaction conditions and nature of the catalyst have a determining influence on the selectivity of the reaction and yield of 5-phenyl-2-n-butylfuran1. In the course of this reaction, the formation of three products was observed: phenol, biphenyl and 5-phenyl-2-n-butylfuran1. The polarity of the solvent has a decisive influence on the yields. The relatively non-polar solvent ethylbenzene gave no coupling product, whereas NMP or DMF led to complete conversion of phenyl triflate with high selectivities in favor of the formation of 1 (Table 1 entries 1–3). On the other hand, DMAc or 2-methoxyethyl ether led to 1 in low conversions and low to moderate selectivities (Table 1, entries 4 and 5). Then, we examined the influence of the base for this reaction. K2CO3 or Cs2CO3 led to the formation of phenol in 83 and 76% selectivities (Table 1, entries 7 and 8). With these bases, the expected product 1 was obtained only in trace amounts. The most suitable base appears to be KOAc with a selectivity of 90% in 1, whereas NaOAc which is less soluble in DMF gave 1 only as traces (Table 1, entries 3 and 6). The influence of the base might be explained by the abstraction of triflate from the arylpalladium triflate intermediate in the presence of potassium acetate to form an arylpalladium acetate (Scheme 2). This complex would be more reactive in the subsequent steps of the catalytic cycle.
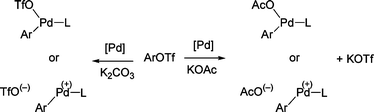 |
| Scheme 2 | |
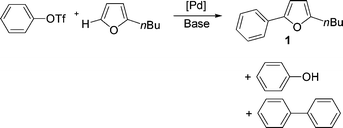 |
| Scheme 3 | |
Entry |
Catalyst |
Solvent |
Base |
Temp./°C |
Ratio phenol : biphenyl : 1 |
Conversion of 2-nbutylfuran |
Isolated yield of 1 (%) |
Conditions: catalyst: Pd(OAc)2 or PdCl(C3H5)DPPB 0.05 mmol or [PdCl(C3H5)]2 0.025 mmol, PPh3, P(oTol)3 or P(2-furyl)3: 0.1 mmol, DPPM, DPPE or DPPB: 0.05 mmol, aryl bromide: 1 mmol, 2-nbutylfuran: 2 mmol, base: 2 mmol, 20 h, ratio phenol : biphenyl : 1 determined by GC and NMR, conversion of the phenyl triflate into phenol, biphenyl and 1. |
1 |
Pd(OAc)2–PPh3 |
Ethylbenzene
|
KOAc
|
150 |
— |
0 |
|
2 |
Pd(OAc)2–PPh3 |
NMP
|
KOAc
|
150 |
7 : 11 : 82 |
100 |
|
3 |
Pd(OAc)2–PPh3 |
DMF
|
KOAc
|
150 |
6 : 4 : 90 |
100 |
51 |
4 |
Pd(OAc)2–PPh3 |
DMAc |
KOAc
|
150 |
25 : 12 : 6 |
43 |
|
5 |
Pd(OAc)2–PPh3 |
2-Methoxyethyl ether
|
KOAc
|
150 |
0 : 11 : 38 |
49 |
27 |
6 |
Pd(OAc)2–PPh3 |
DMF
|
NaOAc |
150 |
25 : 13 : 5 |
43 |
|
7 |
Pd(OAc)2–PPh3 |
DMF
|
K2CO3
|
150 |
83 : 14 : 3 |
100 |
|
8 |
Pd(OAc)2–PPh3 |
DMF
|
Cs2CO3 |
150 |
76 : 12 : 12 |
100 |
|
9 |
Pd(OAc)2–PPh3 |
DMF
|
KOAc
|
120 |
5 : 5 : 75 |
85 |
|
10 |
Pd(OAc)2–PPh3 |
DMF
|
KOAc
|
100 |
3 : 3 : 12 |
18 |
|
11 |
Pd(OAc)2 |
DMF
|
KOAc
|
150 |
37 : 17 : 2 |
56 |
|
12 |
Pd(OAc)2–P(oTol)3 |
DMF
|
KOAc
|
150 |
94 : 0 : 4 |
98 |
|
13 |
Pd(OAc)2–P(2-furyl)3 |
DMF
|
KOAc
|
150 |
96 : 0 : 2 |
98 |
|
14 |
Pd(OAc)2–DPPM |
DMF
|
KOAc
|
150 |
92 : 5 : 2 |
99 |
|
15 |
Pd(OAc)2–DPPE |
DMF
|
KOAc
|
150 |
8 : 5 : 87 |
100 |
22 |
16 |
Pd(OAc)2–DPPB |
DMF
|
KOAc
|
150 |
25 : 2 : 2 |
29 |
|
17 |
[PdCl(C3H5)]2–PPh3 |
DMF
|
KOAc
|
150 |
2 : 13 : 85 |
100 |
19 |
18 |
[PdCl(C3H5)]2–DPPE |
DMF
|
KOAc
|
150 |
8 : 20 : 72 |
100 |
28 |
19 |
PdCl(C3H5)DPPB16 |
DMF
|
KOAc
|
150 |
25 : 12 : 6 |
43 |
|
Then, we examined the influence of the catalyst precursor on this reaction using several mono- or diphosphine ligands. Initially we performed the reaction with Pd(OAc)2 without added ligand, but the reaction gave a very low yield of 1: 2% (Table 1, entry 11). Three monophosphines were employed, PPh3, P(o-Tol)3 and P(2-furyl)3. The system combining Pd(OAc)2 and PPh3 is a particularly effective catalyst for this arylation reaction and gave a high selectivity in favor of 1, whereas the two other phosphines led almost exclusively to the formation of phenol (Table 1, entries 3, 12 and 13). This is probably due to the steric and electronic properties of these ligands which could led to a slower oxidative addition of the aryl triflate to palladium. P(2-furyl)3 possess a weaker σ-donicity compared to PPh3 and P(o-Tol)3 is more congested. The use of the bidentate ligands DPPM or DPPB did not lead to better results with, again, a predominant formation of phenol (Table 1, entries 14 and 16). On the other hand, DPPE associated to Pd(OAc)2 gave a high selectivity in favor of 1, but a low isolated yield (Table 1, entry 15). The palladium source has also an influence on the selectivity and the yield of the reaction. Although relatively high selectivities in favor of the formation of 1 were observed using [PdCl(C3H5)]2 associated to PPh3 or DPPE, relatively low isolated yields were obtained (Table 1, entries 17–19).
The direct arylation of 2-n-butylfuran using aryl triflates appears to be much more sensitive to the reaction conditions and catalyst precursor than using aryl bromides.11f This could be the result both of a lower stability of aryl triflates in the reaction conditions (formation of phenol) and also of a lower reactivity or stability of the ArPd+ OTf− intermediates.
Several mechanisms have been suggested for the C–H activation–functionalization of heteroarenes .11b With furans, thiophenes or thiazoles we believe that the reaction might proceed via a classical oxidative addition of the aryl triflate to a palladium(0) complex followed by a Heck type insertion of the heteroaromatic derivative. On this intermediate the Pd on carbon 4 and the H on carbon 5 of furan, thiophene or thiazole are anti. As anti β-eliminations are unfavored, we assume that a base-assisted elimination of the proton on carbon 5 followed by an aromatization and elimination of Pd occurs. Then, a reductive elimination assisted by the base regenerates the Pd(0). In the presence of benzoxazole, an electrophilic aromatic substitution type mechanism seems more likely.
Then, we tried to evaluate the scope and limitations of this procedure using several heteroaryl derivatives and aryl triflates (Scheme 4). A survey of these couplings is provided in Table 2. A variety of heteroarenes are tolerated. Using the substituted furan derivative 2-furylacetone with phenyl triflate, the coupling product 2 was obtained in a modest 28% yield (Table 2, entry 1). Thiophenes are suitable reactants for such couplings. 2-n-Butylthiophene or thiophene 2-carbonitrile gave the 5-phenylated thiophenes 3 and 4 in 69 and 64% yields (Table 2, entries 2 and 3). Then, we examined the reactivity of two thiazole derivatives. Using 2-n-propylthiazole or 2-ethyl-4-methylthiazole, the 5-arylated products 5 and 6 were obtained in 78 and 37% yields, respectively (Table 2, entries 4 and 5). In the presence of benzoxazole, slightly different reaction conditions had to be employed in order to obtain a high yield of coupling product. With this substrate, the reaction performed with KOAc as base led to a low yield of 7, whereas the use of the more soluble base Cs2CO3 led to 7 in a very high yield of 81% (Table 2, entry 6). The presence of this base might favor a deprotonation of benzoxazole.
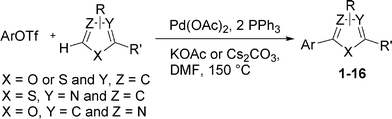 |
| Scheme 4 | |
Entry |
Aryl triflate |
Heteroarene
|
Product |
Yield (%) |
Conditions: Pd(OAc)2: 0.05 mmol, PPh3: 0.1 mmol, aryl triflate: 1 mmol, heteroarene : 2 mmol, KOAc: 2 mmol, DMF, 20 h, 150 °C, isolated yields. Cs2CO3: 2 mmol instead of KOAc as base. |
1 |
Phenyl triflate
|
2-Furylacetone
|
|
28 |
2 |
Phenyl triflate
|
2-n-Butylthiophene
|
|
69 |
3 |
Phenyl triflate
|
Thiophene 2-carbonitrile
|
|
64 |
4 |
Phenyl triflate
|
2-n-Propylthiazole
|
|
78 |
5 |
Phenyl triflate
|
2-Ethyl-4-methylthiazole
|
|
37 |
6 |
Phenyl triflate
|
Benzoxazole
|
|
81a |
7 |
2-Naphthyl triflate
|
2-n-Butylfuran
|
|
53 |
8 |
2-Naphthyl triflate
|
2-n-Propylthiazole
|
|
62 |
9 |
2-Naphthyl triflate
|
Benzoxazole
|
|
86a |
10 |
4-Methoxyphenyl triflate
|
Benzoxazole
|
|
78a |
11 |
3-N,N-Dimethylaminophenyl triflate
|
2-n-Propylthiazole
|
|
69 |
12 |
3-N,N-Dimethylaminophenyl triflate
|
Benzoxazole
|
|
57a |
13 |
4-tert-Butylphenyl triflate
|
2-n-Propylthiazole
|
|
58 |
14 |
4-tert-Butylphenyl triflate
|
Thiophene 2-carbonitrile
|
|
64 |
15 |
3,5-Dimethoxyphenyl triflate
|
2-n-Propylthiazole
|
|
61 |
16 |
4-Nitrophenyl triflate
|
Benzoxazole
|
— |
0a |
17 |
4-Benzoylphenyl triflate
|
Benzoxazole
|
— |
0a |
Although several heteroaromatics are tolerated and gave the biaryls in moderate to high yields, the influence of the substituents on the aryl triflate is more important. First, we studied the reactivity of electron-deficient aryl triflates such as 4-nitrophenyl triflate or 4-benzoylphenyl triflate. However, with these substrates, the expected coupling products were not observed, and a complete conversion of the aryl triflates into the corresponding phenols was observed (Table 2, entries 15 and 16). On the other hand, using 2-naphthyl triflate or the electron-rich 4-methoxyphenyl-, 3,5-dimethoxyphenyl-, 4-tert-butylphenyl- and 3-N,N-dimethylaminophenyl triflates, the expected coupling products 8–16 were obtained in good yields (Table 2, entries 7–15). For example, the reaction of 2-naphthyl triflate with 2-n-butylfuran, 2-n-propylthiazole or benzoxazole gave the arylated products in 53–86% yields. It should be noted that, for these reactions, the phenylated products 1, 4, 5 and 7 were also observed as side-products. The formation of these products comes from the cleavage of the P–Ph bond of triphenylphosphine assisted by the Pd complex. Such behaviour is already known in other organic reactions where the catalytic system comprises palladium and phosphine ligands.17
Conclusion
In conclusion, electron-rich aryl triflates are useful reactants in palladium-catalyzed direct arylation of heteroaromatics. However, the reaction is extremely sensitive to the reaction conditions, catalyst precursor and ligand. The best catalytic system appears to be Pd(OAc)2–PPh3 with KOAc as base in most cases and DMF as solvent. The electronic properties of the aryl triflate have a determining influence on the reaction selectivities and yields. If the electron-donating substituents support the reactions, on the other hand, electron-withdrawing substituents are unfavorable. A wide range of heteroaromatics such as furan, thiophene, thiazole or oxazole derivatives have been successfully employed. Moreover, this procedure is very simple, economically attractive due to the easy access to several heteroaromatics and phenols , and employs commercially available ligand and catalyst precursors.
Experimental
General
All reactions were run under argon using vacuum lines and oven-dried Schlenk tubes. Commercial heteroarenes and phenyl triflate were employed. The other aryl triflates were prepared by stirring of the phenol derivative (1 eq.) and triflic anhydride (1 eq.) in dry dichloromethane at 0 °C during 3 hours followed by hydrolysis, extraction with dichloromethane, separation, drying and purification on silica gel. The reactions were followed by GC. 1H (200 or 300 MHz), 13C (50 MHz) spectra were recorded for CDCl3 solutions. Chemical shift (δ) are reported in ppm relative to CDCl3 (1H: 7.25 and 13C: 77.0). Flash chromatography was performed on silica gel (230–400 mesh).
Representative procedure for palladium-catalysed couplings of heteroaryls with aryl triflates
The aryl triflate (1 mmol), heteroaryl derivative (2 mmol), KOAc (0.196 mg, 2 mmol) (Cs2CO3, 0.651 mg, 2 mmol, instead of KOAc for the reactions with benzoxazole), Pd(OAc)2 (0.011 mg, 0.05 mmol) and PPh3 (0.026 mg, 0.1 mmol) were dissolved in DMF (3 mL) under an argon atmosphere. The reaction mixture was stirred at 150 °C for 20 h. The solution was diluted with H2O (10 mL) (KOH–H2O solution for the reactions with benzoxazole), then the product was extracted with CH2Cl2. The combined organic layer was dried over MgSO4 and the solvent was removed in vacuo. The crude product was purified by silica gel column chromatography .
This compound was prepared according to the representative procedure with phenyl triflate (0.226 g, 1 mmol), 2-n-butylfuran (0.248 g, 2 mmol) and the Pd complex (0.05 mmol) in 102 mg, 51% yield as an oil.
This compound was prepared according to the representative procedure with phenyl triflate (0.226 g, 1 mmol), 2-furylacetone (0.248 g, 2 mmol) and the Pd complex (0.05 mmol) in 56 mg, 28% yield as an oil.
This compound was prepared according to the representative procedure with phenyl triflate (0.226 g, 1 mmol), 2-n-butylthiophene (0.280 g, 2 mmol) and the Pd complex (0.05 mmol) in 149 mg, 69% yield as an oil.
1H (200 MHz) NMR spectra: δ = 0.99 (t, J = 7.5 Hz, 3 H), 1.48 (sext., J = 7.5 Hz, 2 H), 1.73 (quint., J = 7.5 Hz, 2 H), 2.86 (t, J = 7.5 Hz, 2 H), 6.79 (d, J = 3.6 Hz, 1 H), 7.17 (d, J = 3.6 Hz, 1 H), 7.30 (t, J = 7.5 Hz, 1 H), 7.42 (t, J = 7.5 Hz, 2 H), 7.71 (d, J = 8.5 Hz, 2 H).
13C (50 MHz) NMR spectra: δ = 13.8, 22.2, 29.9, 33.7, 122.6, 125.0, 125.5, 126.9, 128.8, 134.8, 141.6, 145.7.
Anal. Calcd. for C14H16S: C, 77.72; H, 7.45. Found: C, 77.81; H, 7.19%.
5-Phenylthiophene-2-carbonitrile (4)19
This compound was prepared according to the representative procedure with phenyl triflate (0.226 g, 1 mmol), thiophene-2-carbonitrile (0.218 g, 2 mmol) and the Pd complex (0.05 mmol) in 119 mg, 64% yield as a white solid.
5-Phenyl-2-n-propylthiazole (5)
This compound was prepared according to the representative procedure with phenyl triflate (0.226 g, 1 mmol), 2-n-propylthiazole (0.254 g, 2 mmol) and the Pd complex (0.05 mmol) in 159 mg, 78% yield as an oil.
1H (200 MHz) NMR spectra: δ = 1.06 (t, J = 7.5 Hz, 3 H), 1.88 (sext., J = 7.5 Hz, 2 H), 3.01 (t, J = 7.5 Hz, 2 H), 7.20–7.40 (m, 3 H), 7.47 (d, J = 7.8 Hz, 2 H), 7.80 (s, 1 H).
13C (50 MHz) NMR spectra: δ = 14.0, 23.7, 35.9, 132.8, 147.3, 126.9, 128.3, 129.6, 132.0, 170.8.
Anal. Calcd. for C12H13NS: C, 70.89; H, 6.45. Found: C, 71.04; H, 6.49%.
2-Ethyl-4-methyl-5-phenylthiazole (6)
This compound was prepared according to the representative procedure with phenyl triflate (0.226 g, 1 mmol), 2-ethyl-4-methylthiazole (0.254 g, 2 mmol) and the Pd complex (0.05 mmol) in 75 mg, 37% yield as an oil.
1H (200 MHz) NMR spectra: δ = 1.42 (t, J = 7.5 Hz, 3 H), 2.49 (s, 3 H), 3.02 (q, J = 7.5 Hz, 2 H), 7.34–7.44 (m, 5 H).
13C (50 MHz) NMR spectra: δ = 14.7, 16.5, 27.3, 127.9, 129.0, 129.6, 129.9, 132.8, 147.3, 170.7.
Anal. Calcd. for C12H13NS: C, 70.89; H, 6.45. Found: C, 70.71; H, 6.58%.
This compound was prepared according to the representative procedure with phenyl triflate (0.226 g, 1 mmol), benzoxazole (0.238 g, 2 mmol) and the Pd complex (0.05 mmol) in 158 mg, 81% yield as a white solid.
2-n-Butyl-5-naphthalen-2-yl-furan (8)
This compound was prepared according to the representative procedure with 2-naphthyl triflate (0.276 g, 1 mmol), 2-n-butylfuran (0.248 g, 2 mmol) and the Pd complex (0.05 mmol) in 133 mg, 53% yield as an oil.
1H (200 MHz) NMR spectra: δ = 0.96 (t, J = 7.5 Hz, 3 H), 1.29 (sext., J = 7.5 Hz, 2 H), 1.64 (quint., J = 7.5 Hz, 2 H), 2.74 (t, J = 7.5 Hz, 2 H), 6.14 (d, J = 3.0 Hz, 1 H), 6.70 (d, J = 3.0 Hz, 1 H), 7.28–7.90 (m, 6 H), 8.08 (s, 1 H).
13C (50 MHz) NMR spectra: δ = 14.4, 22.9, 30.7, 32.5, 107.0, 107.6, 121.8, 122.7, 126.9, 127.9, 128.3, 128.6, 129.1, 129.4, 132.8, 133.0, 134.2, 147.3.
Anal. Calcd. for C18H18O: C, 86.36; H, 7.25. Found: C, 86.54; H, 7.41%.
4-Naphthalen-2-yl-2-n-propylthiazole (9)
This compound was prepared according to the representative procedure with 2-naphthyl triflate (0.276 g, 1 mmol), 2-n-propylthiazole (0.254 g, 2 mmol) and the Pd complex (0.05 mmol) in 157 mg, 62% yield as an oil.
1H (200 MHz) NMR spectra: δ = 1.09 (t, J = 7.5 Hz, 3 H), 1.90 (sext., J = 7.5 Hz, 2 H), 3.04 (t, J = 7.5 Hz, 2 H), 7.28 (m, 2 H), 7.52 (d, J = 7.5 Hz, 1 H), 7.70–7.88 (m, 3 H), 7.98 (s, 2 H).
13C (50 MHz) NMR spectra: δ = 14.1, 23.8, 36.0, 123.1, 124.9, 125.7, 126.7, 127.2, 128.2, 129.2, 130.8, 133.3, 134.0, 138.3, 148.3, 171.3.
Anal. Calcd. for C16H15NS: C, 75.85; H, 5.97. Found: C, 75.70; H, 6.09%.
This compound was prepared according to the representative procedure with 2-naphthyl triflate (0.276 g, 1 mmol), benzoxazole (0.238 g, 2 mmol) and the Pd complex (0.05 mmol) in 211 mg, 86% yield as an oil.
This compound was prepared according to the representative procedure with 4-methoxyphenyl triflate (0.254 g, 1 mmol), benzoxazole (0.238 g, 2 mmol) and the Pd complex (0.05 mmol) in 176 mg, 78% yield as a white solid.
5-(3-N,N-Dimethylaminophenyl)-2-n-propylthiazole (12)
This compound was prepared according to the representative procedure with 3-N,N-dimethylaminophenyl triflate (0.269 g, 1 mmol), 2-n-propylthiazole (0.254 g, 2 mmol) and the Pd complex (0.05 mmol) in 170 mg, 69% yield as an oil.
1H (200 MHz) NMR spectra: δ = 1.03 (t, J = 7.6 Hz, 3 H), 1.94 (sext., J = 7.6 Hz, 2 H), 2.95 (t, J = 7.6 Hz, 2 H), 2.96 (s, 6 H), 6.71 (dd, J = 8.0, 2.3 Hz, 1 H), 6.86 (s, 1 H), 6.91 (d, J = 7.7 Hz, 1 H), 7.26 (t, J = 8.0 Hz, 1 H), 7.83 (s, 1 H).
13C (50 MHz) NMR spectra: δ = 14.2, 23.8, 36.0, 41.0, 110.9, 112.7, 115.5, 130.1, 132.7, 137.7, 139.8, 151.3, 170.7.
Anal. Calcd. for C14H18N2S: C, 68.25; H, 7.36. Found: C, 68.41; H, 7.44%.
[3-(Benzoxazol-2-yl)phenyl]dimethylamine (13)
This compound was prepared according to the representative procedure with 3-N,N-dimethylaminophenyl triflate (0.269 g, 1 mmol), benzoxazole (0.238 g, 2 mmol) and the Pd complex (0.05 mmol) in 136 mg, 57% yield as an oil.
1H (200 MHz) NMR spectra: δ = 3.08 (s, 6 H), 6.88 (dd, J = 8.0, 2.3 Hz, 1 H), 7.30–7.45 (m, 3 H), 7.50–7.75 (m, 3 H), 7.80 (m, 1 H).
13C (50 MHz) NMR spectra: δ = 41.0, 110.9, 111.4, 116.0, 116.2, 120.3, 124.9, 125.3, 128.1, 130.0, 142.5, 151.1, 164.3.
Anal. Calcd. for C15H14N2O: C, 75.61; H, 5.92. Found: C, 75.78; H, 5.87%.
5-(4-tert-Butylphenyl)-2-n-propylthiazole (14)
This compound was prepared according to the representative procedure with 4-tert-butylphenyl triflate (0.282 g, 1 mmol), 2-n-propylthiazole (0.254 g, 2 mmol) and the Pd complex (0.05 mmol) in 151 mg, 58% yield as an oil.
1H (200 MHz) NMR spectra: δ = 1.03 (t, J = 7.6 Hz, 3 H), 1.32 (s, 9 H), 1.92 (sext., J = 7.6 Hz, 2 H), 2.96 (t, J = 7.6 Hz, 2 H), 7.40 (d, J = 7.8 Hz, 2 H), 7.45 (d, J = 7.8 Hz, 2 H), 7.80 (s, 1 H).
13C (50 MHz) NMR spectra: δ = 13.6, 23.2, 31.1, 34.5, 35.5, 125.8, 126.2, 128.7, 137.1, 138.2, 151.0, 170.1.
Anal. Calcd. for C16H21NS: C, 74.08; H, 8.16. Found: C, 73.99; H, 8.27%.
5-(4-tert-Butylphenyl)thiophene-2-carbonitrile (15)
This compound was prepared according to representative procedure with 4-tert-butylphenyl triflate (0.282 g, 1 mmol), thiophene-2-carbonitrile (0.218 g, 2 mmol) and the Pd complex (0.05 mmol) in 154 mg, 64% yield as an oil.
1H (200 MHz) NMR spectra: δ = 1.30 (s, 9 H), 7.22 (d, J = 4.0 Hz, 1 H), 7.43 (d, J = 8.2 Hz, 2 H), 7.53 (d, J = 8.2 Hz, 2 H), 7.57 (d, J = 4.0 Hz, 1 H).
13C (50 MHz) NMR spectra: δ = 31.1, 34.8, 107.7, 114.5, 122.8, 126.1, 126.2, 129.5, 138.3, 152.0, 152.9.
Anal. Calcd. for C15H15NS: C, 74.65; H, 6.26. Found: C, 74.89; H, 6.07%.
5-(3,5-Dimethoxyphenyl)-2-n-propylthiazole (16)
This compound was prepared according to representative procedure with 3,5-dimethoxyphenyl triflate (0.286 g, 1 mmol), 2-n-propylthiazole (0.254 g, 2 mmol) and the Pd complex (0.05 mmol) in 161 mg, 61% yield as an oil.
1H (200 MHz) NMR spectra: δ = 1.03 (t, J = 7.6 Hz, 3 H), 1.92 (sext., J = 7.6 Hz, 2 H), 2.96 (t, J = 7.6 Hz, 2 H), 3.82 (s, 6 H), 6.41 (s, 1 H), 6.62 (s, 2 H), 7.78 (s, 1 H).
13C (50 MHz) NMR spectra: δ = 14.1, 23.8, 35.9, 55.8, 100.3, 105.3, 122.5, 133.7, 138.2, 161.5.
Anal. Calcd. for C14H17NO2S: C, 63.85; H, 6.51. Found: C, 63.70; H, 6.57%.
References
- For examples of palladium cross-coupling with heteroaromatic compounds: J. J. Li and G. W. Gribble, Palladium in Heterocyclic Chemistry, Pergamon, Amsterdam, 2000 Search PubMed.
- For selected examples of arylations of furan, thiophene or thiazole derivatives via Negishi coupling using aryl triflates:
(a) A. Arcadi, A. Burini, S. Cacchi, M. Delmastro, F. Marinelli and B. Pietroni, Synlett, 1990, 47 CrossRef CAS;
(b) G. S. Cockerill, H. J. Easterfield, J. M. Percy and S. Pintat, J. Chem. Soc., Perkin Trans. 1, 2000, 2591 RSC.
- For selected examples of arylations of oxazole derivatives via Negishi coupling using aryl triflates: B. A. Anderson and N. K. Harn, Synthesis, 1996, 583 Search PubMed.
- For selected examples of 2-arylations of furan derivatives via Stille coupling using aryl triflates:
(a) I. S. Mann, D. A. Widdowson and J. M. Clough, Tetrahedron, 1991, 47, 7991 CrossRef CAS;
(b) D. P. Curran and M. Hoshino, J. Org. Chem., 1996, 61, 6480 CrossRef CAS;
(c) G. A. DeBoos, J. J. Fullbrook and J. M. Percy, Org. Lett., 2001, 3, 2859 CrossRef CAS.
- For selected examples of 2-arylations of thiophene derivatives via Stille coupling using aryl triflates:
(a) G. T. Crisp and S. Papadopoulos, Aust. J. Chem., 1988, 41, 1711 CAS;
(b) A. Busch, V. Gautheron Chapoulaud, J. Audoux, N. Ple and A. Turck, Tetrahedron, 2004, 60, 5373 CrossRef CAS;
(c) M. Torrado, C. F. Masaguer and E. Ravina, Tetrahedron Lett., 2007, 48, 323 CrossRef CAS.
- For selected examples of 2-arylations of thiazole derivatives via Stille coupling using aryl triflates: S. G. Cockerill, H. J. Easterfield and J. M. Percy, Tetrahedron Lett., 1999, 40, 2601 Search PubMed.
- For selected examples of 2-arylations of furan derivatives via Suzuki coupling using aryl triflates:
(a) A. Huth, I. Beetz and I. Schumann, Tetrahedron, 1989, 45, 6679 CrossRef CAS;
(b) W. C. Shieh and J. A. Carlson, J. Org. Chem., 1992, 57, 379 CrossRef CAS;
(c) A. G. Chittiboyina, C. R. Reddy, E. B. Watkins and M. A. Avery, Tetrahedron Lett., 2004, 45, 1689 CrossRef CAS;
(d) C. Pain, S. Celanire, G. Guillaumet and B. Joseph, Tetrahedron, 2003, 59, 9627 CrossRef CAS;
(e) Y. Okada, M. Yokozawa and J. Nishimura, Synlett, 2005, 352 CrossRef CAS.
- For selected examples of arylations of thiophene derivatives via Suzuki coupling using aryl triflates:
(a) C. Coudret and V. Mazenc, Tetrahedron Lett., 1997, 38, 5293 CrossRef CAS;
(b) G. McCort, O. Duclos, C. Cadilhac and E. Guilpain, Tetrahedron Lett., 1999, 40, 6211 CrossRef CAS;
(c) M. Frigoli, C. Moustrou, A. Samat and R. Guglielmetti, Eur. J. Org. Chem., 2003, 2799 CrossRef CAS;
(d) B. Appel, N. N. R. Saleh and P. Langer, Chem.–Eur. J., 2006, 12, 1221 CrossRef CAS;
(e) J. E. Gautrot, P. Hodge, D. Cupertino and M. Helliwell, New J. Chem., 2006, 30, 1801 RSC.
- For an example of 5-arylation of oxazole via Suzuki coupling using an aryl triflate: E. F. Flegeau, M. E. Popkin and M. F. Greaney, Org. Lett., 2006, 8, 2495 Search PubMed.
- A. Ohta, Y. Akita, T. Ohkuwa, M. Chiba, R. Fukunaga, A. Miyafuji, T. Nakata, N. Tani and Y. Aoyagi, Heterocycles, 1990, 31, 1951 CrossRef CAS.
- For reviews or recent results on Pd-catalyzed C–H activation–functionalization
of aryls see:
(a) C. Campeau and K. Fagnou, Chem. Commun., 2006, 1253 RSC;
(b) D. Alberico, M. E. Scott and M. Lautens, Chem. Rev., 2007, 107, 174 CrossRef CAS;
(c) T. Satoh and M. Miura, Chem. Lett., 2007, 36, 200 CrossRef CAS;
(d) J.-P. Leclerc and K. Fagnou, Angew. Chem., Int. Ed., 2006, 45, 7781 CrossRef CAS;
(e) N. R. Deprez, D. Kalyani, A. Krause and M. S. Sanford, J. Am. Chem. Soc., 2006, 128, 4972 CrossRef CAS;
(f) J.-Q. Yu, R. Giri and X. Chen, Org. Biomol. Chem., 2006, 4041 Search PubMed;
(g) A. Battace, M. Lemhadri, T. Zair, H. Doucet and M. Santelli, Organometallics, 2007, 26, 472 CrossRef CAS;
(h) A. Chiong and O. Daugulis, Org. Lett., 2007, 9, 1449 CrossRef CAS;
(i) A. L. Gottumukkala and H. Doucet, Eur. J. Inorg. Chem., 2007, 3629 CrossRef CAS;
(j) D. G. Hulcoop and M. Lautens, Org. Lett., 2007, 9, 1761 CrossRef CAS.
- For examples of Pd-catalyzed direct arylations of aromatic derivatives via C–H activation using aryl triflates:
(a) T. Hosoya, E. Takashiro, T. Matsumoto and K. Suzuki, J. Am. Chem. Soc., 1994, 116, 1004 CrossRef;
(b) G. Bringmann, A. Wuzik, J. Kraus, K. Peters and E.-M. Peters, Tetrahedron Lett., 1998, 39, 1545 CrossRef CAS;
(c) L. Wang and P. B. Shevlin, Tetrahedron Lett., 2000, 41, 285 CrossRef CAS;
(d) Y. Kametani, T. Satoh, M. Miura and M. Nomura, Tetrahedron Lett., 2000, 41, 2655 CrossRef CAS;
(e) H. Nishioka, Y. Shoujiguchi, H. Abe, Y. Takeuchi and T. Harayama, Heterocycles, 2004, 64, 463 CrossRef CAS.
- L. Ackermann, A. Althammer and R. Born, Angew. Chem., Int. Ed., 2006, 45, 2619 CrossRef CAS.
-
(a) J. R. Proudfoot, K. D. Hargrave, S. R. Kapadia, U. R. Patel, K. G. Grozinger, D. W. McNeil, E. Cullen, M. Cardozo, L. Tong, T. A. Kelly, J. Rose, E. David, S. C. Mauldin, V. U. Fuchs, J. Vitous, M. Hoermann, J. M. Klunder, P. Raghavan, J. W. Skiles, P. Mui, D. D. Richman, J. L. Sullivan, C.-K. Shih, P. M. Grob and J. Adams, J. Med. Chem., 1995, 38, 4830 CrossRef CAS;
(b) T. Okazawa, T. Satoh, M. Miura and M. Nomura, J. Am. Chem. Soc., 2002, 124, 5286 CrossRef CAS;
(c) O. Hara, T. Nakamura, F. Sato, K. Makino and Y. Hamada, Heterocycles, 2006, 68, 1 CrossRef CAS.
- M. Brenner, G. Mayer, A. Terpin and W. Steglich, Chem.–Eur. J., 1997, 3, 70 CrossRef CAS.
- T. Cantat, E. Génin, C. Giroud, G. Meyer and A. Jutand, J. Organomet. Chem., 2003, 687, 365 CrossRef CAS.
-
(a) F. E. Goodson, T. I. Wallow and B. M. Novak, J. Am. Chem. Soc., 1997, 119, 12442;
(b) F. Churruca, R. SanMartin, M. Carril, I. Tellitu and E. Domínguez, Tetrahedron, 2004, 60, 2393 CrossRef CAS and references herein.
- C. W. Ong, C. M. Chen and S. S. Juang, J. Org. Chem., 1994, 59, 7915 CrossRef CAS.
- L. Lavenot, C. Gozzi, K. Ilg, I. Orlova, V. Penalva and M. Lemaire, J. Organomet. Chem., 1998, 567, 49 CrossRef CAS.
- J. C. Lewis, S. H. Wiedemann, R. G. Bergman and J. A. Ellman, Org. Lett., 2004, 6, 35 CrossRef CAS.
- C. S. Cho, D. T. Kim, J. Q. Zhang, S.-L. Ho, T.-J. Kim and S. C. Shim, J. Heterocycl. Chem., 2002, 39, 421 CrossRef CAS.
- G. Altenhoff and F. Glorius, Adv. Synth. Catal., 2004, 346, 1661 CrossRef CAS.
Footnote |
† Electronic supplementary information (ESI) available: Further experimental data. See DOI: 10.1039/b715235c |
|
This journal is © The Royal Society of Chemistry 2008 |
Click here to see how this site uses Cookies. View our privacy policy here.