DOI:
10.1039/B705520J
(Paper)
Green Chem., 2008,
10, 67-72
Influence of anions on the toxic effects of ionic liquids to a phytoplankton Selenastrum capricornutum
Received 12th April 2007, Accepted 8th October 2007
First published on 26th October 2007
Abstract
Investigations on the influence of anions of ionic liquids, with respect to toxicity and ecotoxicity, have been reported; however, no consistent conclusion has been drawn. The aim of this study was to elucidate the influence of the anionic component, using the alga Selenastrum capricornutum, by assessing the toxicity of various anions associated with imidazolium-based ionic liquids as well as alkali salts. Additionally, the hydrolysis of fluoride-containing ionic liquids, i.e., 1-butyl-3-methylimidazolium [BMIM], incorporated with tetrafluoroborate, hexafluorophosphate and hexafluoroantimonate, was also estimated. The results obtained revealed that imidazolium-based ionic liquids were more toxic than their corresponding alkali salts. In both cases, SbF6– and PF6– were identified as the most toxic anions. In general, Selenastrum capricornutum was sensitive to the anion moieties in the order: SbF6– > PF6– > BF4– > CF3SO3– > C8H17OSO3– > Br– ≈ Cl–. With regard to the hydrolytic effect, [BMIM][SbF6] generated a greater amount of fluoride compared with [BMIM][BF4], but no fluoride formation occurred with the hexafluorophosphate.
Introduction
Room temperature ionic liquids are a class of non-molecular ionic solvents with low melting points. They are usually composed of asymmetrically substituted nitrogen-containing cations (e.g., imidazole, pyridine, pyrrolidine and ammonium) or a phosphorus-containing cation (e.g., phosphonium) with organic or inorganic anions. The physicochemical properties of ionic liquids (ILs) include non-volatility, non-flammability, high thermal, chemical and electrochemical stability, and the ability to be easily recycled, with favourable solvation behaviour (many organic, organometallic and inorganic compounds can be dissolved in ILs).1–4 Furthermore, it is possible to tune the physical and chemical properties of ILs by varying the nature of the anions and cations;5 in this way, they can be made task-specific.Recently, ILs have gained considerable attention in several branches of the chemical industry as potential “green” substitutes for conventional organic solvents.6,7 The “green” aspect of ILs is mainly derived from their undetectable vapour pressure, as this decreases the risk of exposure and loss of solvent by evaporation, thereby reducing air pollution. However, these green credentials are currently unproven in aquatic environments, since the water solubility of many ILs is not negligible.8 As their popularity with industrial chemists increases, the probability that ILs will find their way into watercourses through effluent discharges or accidental spills will also increase and they may affect the aquatic environment for a long time because of their poor biodegradability. According to Jastorff et al.,9 the toxicity of ILs is roughly driven by the head group, the side chain, and the anion. Currently, the biological effects of ILs have attracted considerable attention, resulting in increasing reports which have dealt mainly with the influence of the alkyl side chain length of various ILs head groups. Pernak et al. pointed out that their antimicrobial activity increased with increasing alkyl chain length on pyridinium, imidazolium and quaternary ammonium salts.10–14 Similar phenomena were also observed for enzyme acetylcholinesterase,15 the marine bacterium Vibrio fischeri,16,17 mammalian cells,16 human cell HeLa18 and higher organisms, including the soil nematode Caenorhabditis elegans,19 as well as the freshwater snail Physa acuta.20 However, with respect to the influence of anions, no consistent conclusion has been obtained from previous investigations. Some studies have reported that varying the anion had minimal effects on the toxicities of several pyridinium and imidazolium compounds, and indicated that the toxicity of IL was largely driven by the alkyl chain branching and hydrophobicity of the cation.12,16,21,22 In the meantime, others have suggested that the counter-anion contributed significantly to their toxicity.18,23,24 In particular, some ILs with fluorine-containing anions were suggested to be relatively toxic because the anions were hydrolysed to fluoride in the aqueous solution and the fluoride had a toxic effect.18,23 However, in-depth studies on this hydrolysis of fluorine-containing anions and their effect on the toxicity were insufficient to explain the inhibitory effect of ILs.
The toxicity of ILs towards aquatic organisms, such as fish and invertebrates, has been extensively studied,9,21,24,25 but the influence of ILs on freshwater green algae has rarely been investigated and remains largely unknown. As algae are primary producers, either directly or indirectly, of organic matter required by small consumers in freshwater food chains, their ecology is crucial in providing the energy for sustaining other higher trophic levels. The widespread distribution of algae makes these organisms ideal for toxicological studies and, because they have a short life cycle, they can respond quickly to environmental change.26,27 In addition, algal assays are relatively simple, quick and inexpensive compared with bioassays using other organisms.28 Thus, test procedures using algae are of advantage when used as biological assay tools for environmental impact studies.
In this paper, the influence of anions on the toxicity of ILs towards the freshwater green alga Selenastrum capricornutum (recently changed to Pseudokirchneriella subcapitata) was investigated based on the US Environmental Protection Agency (US EPA) standard29 and Organization for Economic Cooperation and Development (OECD)30 protocols. The hydrolytic effects of typical anions, BF4–, PF6– and SbF6–, were also assessed using ion chromatography.
Results and discussion
Influence of anions on toxicity of ionic liquid entities
The influence of ILs on the growth of S. capricornutum was estimated in relation to the toxic effect, with the relative activity defined as the ratio of the cell concentration of the treatment sample to that of the control. Fig. 1 demonstrates the growth inhibition of S. capricornutum during 96 h exposure to different concentrations of ILs added to the media. The pattern of algal cell inhibition was similar for all the ILs tested, regardless of the compound used. As is summarized in Table 1, the EC50 values obtained ranged from 135 to 2884 µM, indicating that the most toxic compound was [BMIM][SbF6]. Fig. 1 reveals that the inhibitory effect on the algal growth rate became stronger with increasing IL concentration. Additionally, the entire dose-response curve of [BMIM][SbF6] was observed to shift to the left compared with those of the other ILs. Therefore, the imidazolium-based ILs, with SbF6– as the counter-anion, obviously drastically reduced the algal cell density, resulting in low EC50 values. In addition to [BMIM][SbF6], [BMIM][PF6] also exhibited slightly enhanced inhibition of the algal cell proliferation at an effective concentration of 1318 µM. With respect to the other ILs, the concentration-response curves showed no remarkable differences, but for those associated with chloride, bromide and hexafluorophosphate, at low concentrations, the S. capricornutum culture was recovered to attain a final density close to, or even higher than, the initial value. The aforementioned hormetic effect (i.e., stimulatory effects occurring in response to low levels of exposure to agents harmful at high levels of exposure) depends both on the nature of the organism and the duration of exposure. Similar phenomena were also observed by Ranke et al. and Stepnowski et al.16,18 during their investigations on the toxicity of ILs towards IPC-81 leukaemia cells and HeLa cells, respectively. Considering [BMIM][CF3SO3] and [BMIM][C8H17OSO3], their effective concentrations were identical and not significantly different from those of ILs combined with bromide, chloride as well as tetrafluoroborate.![Influence of anions with respect to 1-butyl-3-methylimidazolium-based ionic liquids on the alga Selenastrum capricornutum. [BMIM][BF4] (-●-), [BMIM][Cl] (-○-), [BMIM][C8H17OSO3] (-▼-), [BMIM][PF6] (-▽-), [BMIM][CF4SO3] (-■-), [BMIM][Br] (-□-) and [BMIM][SbF6] (-◆-). Error bars correspond to standard deviations of the experimental data.](/image/article/2008/GC/b705520j/b705520j-f1.gif) |
| Fig. 1 Influence of anions with respect to 1-butyl-3-methylimidazolium-based ionic liquids on the alga Selenastrum capricornutum. [BMIM][BF4] (-●-), [BMIM][Cl] (-○-), [BMIM][C8H17OSO3] (-▼-), [BMIM][PF6] (-▽-), [BMIM][CF4SO3] (-■-), [BMIM][Br] (-□-) and [BMIM][SbF6] (-◆-). Error bars correspond to standard deviations of the experimental data. | |
Table 1 The effective concentrations of various ionic liquids and alkali salts to the alga, Selenastrum capricornutum, in 96-h chronic toxicity tests
Chemicals | EC50/µM | Standard deviation/µM |
---|
[BMIM][Br] | 2137 | 1621–2818 |
[BMIM][Cl] | 2884 | 2529–3289 |
[BMIM][BF4] | 2512 | 2018–3126 |
[BMIM][PF6] | 1318 | 954–1819 |
[BMIM][CF3SO3] | 2188 | 1828–2618 |
[BMIM][C8H17SO4] | 2239 | 1914–2618 |
[BMIM][SbF6] | 135 | 102–178 |
[NaCl] | 125 892 | 84 723–187 068 |
[NaBr] | 91 201 | 51 168–162 555 |
[NaBF4] | 4466 | 4037–4943 |
[NaPF6] | 1698 | 1189–2427 |
[KSbF6] | 162 | 88.3–297.9 |
[KF] | 40 738 | 35 810–46 345 |
[NaCF3SO3] | 5248 | 1047–26 303 |
[NaC8H17OSO3] | 10 889 | 9016–13 152 |
Methanol | 707 945 | 588 843–851 138 |
DMF | 23 442 | 17 378–31 622 |
2-Propanol | 194 984 | 147 253–269 153 |
In the toxicity experiment using imidazolium-based ILs the toxicity of the ILs containing halide anions was not remarkable, except for the cases of [BMIM][SbF6] and [BMIM][PF6]. This also accords with the earlier observation by Garcia et al., and Zhao et al.,31,32 which showed that the nature of the inorganic anion has only a little effect. The toxicity of common organic solvents (methanol, dimethylformamide and 2-propanol) that have been widely used was explored for comparison with that of ILs on same alga. The toxicities of these solvents were estimated to be two to five orders of magnitude less than those of ILs (Table 1). Of the tested solvents, dimethyl formamide was the most toxic compound with an effective concentration ranging between 17.4 and 31.6 mM, which was approximately 8 times higher compared with that of the least toxic IL ([BMIM][Cl]) in this study.
Influence of anions on toxicity of alkali salts
In order to explore the influence of the anionic compartment of the ILs, a reference group of sodium and potassium salts, with various anions, was also subjected to the toxicity assay, the results of which are presented in Table 1. As is shown in Fig. 2, the influence of anions was apparently easier to recognize compared with that obtained for ILs. From the results, SbF6– and PF6– were observed to be responsible for the strongest inhibition to alga growth, with effective concentrations of 162 and 1698 µM, respectively. These findings were in line with the IL data mentioned above, where SbF6– and PF6– as the counter-anions evidently caused serious algal growth inhibition after 96 h of exposure. With respect to BF4– and CF3SO3–, their EC50 values were not significantly different in respect of the inhibitory effects towards the algal cell density. However, as can be deduced from the graph pattern, BF4– dramatically inhibited the algal growth at concentrations between 2511 and 5011 µM, while the toxic influence of CF3SO3– gradually increased with increasing concentration and remained unchanged at concentrations above 10 mM. In the case of typical halogen atoms, Br– was found to affect the algal growth at concentrations between 1 and 10 mM. However, both Br– and Cl– exhibited similar toxic effects, with EC50 values around 0.1 M. Additionally, Br– and Cl– were identified as the least toxic of the anions of the compounds tested on the growth rate of S. capricornutum. Especially in our study on Na+ or K+-based salts, the results demonstrated that C8H17SO4– exhibited higher toxicity towards freshwater green algae than the corrosive F– and Cl–. This was contrary to results found by Wasserscheid et al.,33 who suggested that the imidazolium cation incorporated with octyl sulfate was “greener” than typical ILs consisting of halogen containing anions, as the presence of halogen atoms may be the cause of serious concerns. In detail, it was approximately 10 times more toxic than the halide anions, Br– and Cl–, but less toxic than the other anions.![Influence of anions with respect to sodium and potassium salts on the alga Selenastrum capricornutum. [NaBF4] (-●-), [NaPF6] (-○-), [NaCl] (-▼-), [NaBr] (-▽-), [KF] (-■-), [NaCF3SO3] (-□-), [NaC8H17OSO3] (-◇-) and [KSbF6] (-◆-). Error bars correspond to standard deviations of the experimental data.](/image/article/2008/GC/b705520j/b705520j-f2.gif) |
| Fig. 2 Influence of anions with respect to sodium and potassium salts on the alga Selenastrum capricornutum. [NaBF4] (-●-), [NaPF6] (-○-), [NaCl] (-▼-), [NaBr] (-▽-), [KF] (-■-), [NaCF3SO3] (-□-), [NaC8H17OSO3] (-◇-) and [KSbF6] (-◆-). Error bars correspond to standard deviations of the experimental data. | |
Hydrolysis of SbF6–, BF4– and PF6– associated with [BMIM] cations and fluoride effect on algal growth
In order to assess the hydrolysis of ILs leading to fluoride formation, ion chromatography analysis was performed on imidazolium salts combined with fluoride-containing anions, such as BF4–, PF6– and SbF6–. The experiments were conducted continuously for a 9-day period. Each IL compound was prepared by dissolving in a sufficient amount of distilled water to yield a concentration of 7 mM; the experimental data obtained are shown in Fig. 3. Additionally, [KF] was also tested to find the toxic effect of fluoride on algal growth. As is illustrated in Fig. 3, fluoride was rapidly generated from [BMIM] [SbF6] during the first 2 days, but the formation was retarded during the following days. This was in accordance with results found by Griffiths and Walrafen34, who reported that the first step of SbF6– hydrolysis was virtually instantaneous. In principle, the extent of the hydrolysis reaction can be followed as a function of time by determining the fluoride ions formed in the reaction outlined in eqn. (1): | SbF6– + 2nH2O → [SbF6–n(OH)n]– + nF– + nH3O+ | (1) |
![Time variation of the formation of fluoride ions during the hydrolysis of [BMIM][SbF6] (-▼-), [BMIM][BF4] (-○-), and [BMIM][PF6] (-●-) in water.](/image/article/2008/GC/b705520j/b705520j-f3.gif) |
| Fig. 3 Time variation of the formation of fluoride ions during the hydrolysis of [BMIM][SbF6] (-▼-), [BMIM][BF4] (-○-), and [BMIM][PF6] (-●-) in water. | |
With regard to hexafluorophosphate, several researchers18,35,36 have dealt with the instability of PF6– containing ILs. In this study, however, an equivocal outcome was observed for the fluoride formation during the hydrolysis of PF6– moiety at ambient temperature, which was totally in agreement with results found by Villagrán et al.37 However, considerable care should be taken when hexafluorophosphate-containing compounds are applied to industrial processes, as significant potential problems might occur due to their decomposition at high temperature.38 In the case of [BMIM][BF4], the formation of fluoride was easy to observe, but the reaction rate as well as the amount of fluoride generated were less than those with [BMIM][SbF6]. Considering the biological impact of fluoride ions, it was evident that F–, with an effective concentration of 40.7 mM (Table 1), was a more toxic halide ion than the other halides examined.
In order to investigate the effect of the generated fluoride, the amount of fluoride formed by the hydrolysis of [BMIM][SbF6] after 96 h was compared with the EC50 value of [KF]. The fluoride concentration formed from the EC50 concentration of [BMIM][SbF6], and that proportionally calculated for [KSbF6], were less than the EC50 value of [KF]. Therefore, the fluoride formed by hydrolysis of [BMIM][BF4] and [BMIM][SbF6] might not affect the algal proliferation for 96 hours. However, because with increasing time, the formation of fluoride was observed to steadily increase, a re-experiment on the toxicity of [BMIM][BF4] was performed on stock solution prepared 6 months before. The re-experiment was performed on 6-month old stock solution, not simply the exact same experiment re-done six months later. As a result, the toxicity was observed to have further increased, as shown in Fig. 4, with a log10EC50 value of [BMIM][BF4] of 2.11 ± 0.042, and a hormetic effect also occurred. The estimated EC50 value using the incubated stock solution of [BMIM][BF4] prepared 6 months earlier was similar with that of [BMIM][SbF6].
![Toxic effect of [BMIM][BF4] according to storage time. Stock solution freshly prepared at the onset of testing experiment (-○-), Stock solution prepared 6 months earlier (-●-). Error bars correspond to standard deviations of the experimental data.](/image/article/2008/GC/b705520j/b705520j-f4.gif) |
| Fig. 4 Toxic effect of [BMIM][BF4] according to storage time. Stock solution freshly prepared at the onset of testing experiment (-○-), Stock solution prepared 6 months earlier (-●-). Error bars correspond to standard deviations of the experimental data. | |
Conclusions
This study has demonstrated the effects of several typical anions on the toxicity of imidazolium-based ILs toward S. capricornutum, which lives in surface waters, using a testing method based on algal growth. The present study identified that imidazolium-based ILs were more toxic to algae than Na+ or K+-based compounds. Of the imidazolium-based ILs, [BMIM][SbF6] and [BMIM][PF6] affected the algal growth rate much more than remaining compounds and the difference of EC50 values of these compounds was not significant. Conversely, in the toxicity experiment on the anions combined with Na+ or K+, the influence of anions was clearly observed.Also, in order to examine the hydrolysis of fluoride-containing anions, fluoride was detected using ion chromatography. Of the ILs analysed, fluoride ions were formed from [BMIM][SbF6] and [BMIM][BF4], but not from [BMIM][PF6]. When only small amounts of fluoride ions were formed from [BMIM][SbF6] and [BMIM][BF4] within 96 h, the fluoride ion formed did not affect the algal growth rate. Nevertheless, the fluoride ion formation from [BMIM][BF4] increased with incubating time of the stock solution, thus resulting in slightly higher toxicity of the 6 month old stock.
Anions are important, as they can decide the properties of ILs for their suitable use in several industrial applications. At the same time, an anionic component can, in part, determine the toxicity of ILs. Thus, further study should be performed to better understand the influence of anions on the toxicity and environmental fate of ILs.
Experimental
Ionic liquids and other chemicals
1-Butyl-3-methylimidazolium tetrafluoroborate [BMIM][BF4] (purity > 98%), 1-butyl-3-methylimidazolium hexafluorophosphate [BMIM][PF6] (purity > 98%), 1-butyl-3-methylimidazolium bromide [BMIM][Br] (purity > 98%) and 1-butyl-3-methylimidazolium hexafluoroantimonate [BMIM][SbF6] (purity > 98%) were purchased from C-TRI, Korea. 1-Butyl-3-methylimidazolium chloride [BMIM][Cl] (purity > 98%), 1-butyl-3-methylimidazolium octylsulfate [BMIM][C8H17SO4] (purity > 95%) and 1-butyl-3-methylimidazolium trifluoromethanesulfonate [BMIM][CF3SO3] (purity > 95%) were purchased from Sigma–Aldrich. Sodium tetrafluoroborate [NaBF4] (purity > 98%), sodium hexafluorophosphate [NaPF6] (purity > 98%), sodium chloride [NaCl] (purity > 99.5%), sodium bromide [NaBr] (purity > 99%), sodium trifluoromethanesulfonate [NaCF3SO3] (purity > 98%), potassium fluoride [KF] (purity > 99%) and potassium hexafluoroantimonate [KSbF6] (purity > 99%) were purchased from Sigma–Aldrich, and sodium octylsulfate [NaC8H17SO4] (purity > 99%) from Lancaster. 2-Propanol (purity > 99.5%) and dimethylformamide (purity > 99.5%) were purchased from Sigma–Aldrich and methanol (purity > 99.5%) from Samchun Pure Chemical Co. (Korea).Microalgal strain and cultivation
The green microalga, Selenastrum capricornutum ATCC-22662, was obtained from the National Institute Environmental Research (Korea), and used as the model algal strain in this study. The stock alga was cultivated in a 250 ml Erlenmeyer flask containing 200 ml of sterilized nitrate-enriched BBM medium, which was made from triple distilled water to avoid nitrogen limitation in the high-density culture.39 The culture flask was continuously agitated on a rotary shaker at 170 rpm, with air bubbling (1 volume of air/volume of liquid/min) but without a sparger. Continuous illumination of 30 ± 5 µE m–2 s–1 was provided by overhead warm-white fluorescent tubes (Korea General Electric, Korea), with the temperature controlled at 25 ± 2 °C.Toxicity tests
The ILs toxicity tests were conducted using the methods recommended in the EPA29 and the OECD guidelines.30 Experiments were conducted in a 250 ml Erlenmeyer flask, containing 55 ml of sterilized culture medium, inoculated with 5 ml of 7-day cultured algal samples. Subsequently, a known concentration of the ILs was added to each flask, placed on a shaking incubator maintained at 170 rpm and 25 °C, and with 24 h illumination from warm-white fluorescent tubes, which provided an average illumination of 30 ± 5 µE m–2 s–1. At each determined exposure period date, the optical density of the algal biomass was estimated at 438 nm, using a spectrophotometer (UV mini-1240, Shimadzu, Kyoto, Japan). The dry cell weight corresponding to the optical density was determined through the linear relation Dry cell weight = 0.1329 × optical density, and expressed in g l–1. In this study, two replicates were set up for each treatment, whereas there were three replicates for controls, because the control is the basis for evaluation of toxicity, and if the control is not precise the EC50 may contain a large error.Analytical methods
Ion chromatography was used to determine the fluoride ions generated during the hydrolysis of imidazolium-based ILs incorporating BF4–, PF6– and SbF6– as the counter-anions. All chromatographic analyses37 were performed at room temperature using a Metrohm Model 792 Basic IC (Metrohm, Herisau, Switzerland), with a suppressor module, and equipped with a Metrosep A Supp 5 column (150 × 170 mm). The anions were detected using a conductivity detector. The eluent was comprised of a 3.2 mM Na2CO3 and 1.0 mM NaHCO3 mixture, with H2SO3 used as the suppressor regenerating solution. Prior to preparing the eluent mixture and regenerating solution, the deionized water was filtered through a 0.45 µm filter.Data treatment
The protocol used for the growth inhibition bioassay was based on the standard procedures in the OECD guidelines.30 The average specific growth rate, µ, for a specific period was calculated as the logarithmic increase in biomass: | 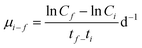 | (2) |
where µi–f is the average specific growth rate from the initial time, i, to the final time, f; Ci and Cf are the initial and final biomass concentrations (mg l–1), respectively; and ti and tf the initial and final times (d), respectively.The proportion of cell growth (I) for each treatment replicate can be calculated from:
|  | (3) |
where
µC and
µT are the specific
growth rates in the control and treatment (d
–1), respectively.
Where feasible, the concentration–response curves were fitted, using the nonlinear least-squares method, to the multinomial data employing the logistic model for the relationship of the cell viability and inhibition to the decadic logarithm of the examined concentrations, which can be written as follows:
|  | (4) |
where
x is the substance concentration to which the
cells were exposed,
I the proportion of
cell growth, normalized with the negative controls (
i.e., controls consisting of the same
nutrient medium conditions, procedures, and algal seeds from the same culture, except that none of the test substance (ILs) is added) to intervals from 1 (
x = 0) to 0 (negative control);
x0 represents the EC50, and
b the slope of the function on a logit-log scale. Calculations were carried out using the Sigma Plot program (Sigma-Plot 8.02).
References
- J. D. Holbrey and K. R. Seddon, Clean Prod. Process., 1999, 1, 223–236 CrossRef.
- H. Olivier-Bourbigou and L. Magna, J. Mol. Catal. A: Chem., 2002, 182–183, 419–437 CrossRef CAS.
- K. N. Marsh, J. A. Boxall and R. Lichtenthaler, Fluid Phase Equilibr., 2004, 219, 93–98 CrossRef CAS.
- J. Fuler, R. T. Carlin and R. A. Osteryoung, J. Electrochem. Soc., 1997, 144, 3881–3886 CAS.
- T. Welton, Chem. Rev., 1999, 99, 2071–2083 CrossRef CAS.
- R. Sheldon, Chem. Commun., 2001, 2399–2407 RSC.
- J. Dupont, R. F. De Souza and P. A. Z. Suazez, Chem. Rev., 2002, 102, 3667–3691 CrossRef CAS.
- D. S. H. Wong, J. P. Chen, J. M. Chang and C. H. Chou, Fluid Phase Equilibr., 2002, 194–197, 1089–1095 CrossRef CAS.
- B. Jastorff, K. Mölter, P. Behrend, U. Bottin-Weber, J. Filser, A. Heimersm, B. Ondruschka, J. Ranke, M. Schaefer, H. Schröder, A. Stark, P. Stepnowski, F. Stock, R. Störmann, S. Stolte, U. Welz-Biermann, S. Ziegert and J. Thöming, Green Chem., 2005, 7, 362–372 RSC.
- J. Pernak, J. Rogoża and I. Mirska, Eur. J. Med. Chem., 2001, 36, 313–320 CrossRef CAS.
- J. Pernak, J. Kalewska, H. Ksycińska and J. Cybulski, Eur. J. Med. Chem., 2001, 36, 899–907 CrossRef CAS.
- J. Pernak, K. Sobaszkiewicz and I. Mirska, Green Chem., 2003, 5, 52–56 RSC.
- J. Pernak and P. Chwała, Eur. J. Med. Chem., 2003, 38, 1035–1042 CrossRef CAS.
- J. Pernak, I. Goc and I. Mirska, Green Chem., 2004, 6, 323–329 RSC.
- F. Stock, J. Hoffmann, J. Ranke, R. Störmann, B. Ondruschka and B. Jastorff, Green Chem., 2004, 6, 286–290 RSC.
- J. Ranke, K. Mölter, F. Stock, U. Bottin-Weber, J. Poczobutt, J. Hoffmann, B. Ondruschka, J. Filser and B. Jastorff, Ecotoxicol. Environ. Saf., 2004, 58, 396–404 CrossRef CAS.
- K. M. Docherty and C. F. Kulpa, Jr., Green Chem., 2005, 7, 185–189 RSC.
- P. Stepnowski, A. C. Składanowski, A. Ludwiczak and E. Łaczyńska, Hum. Exp. Toxicol., 2004, 23, 513–517 CrossRef CAS.
- R. P. Swatloski, J. D. Hobrey, S. B. Memon, G. A. Caldwell, K. A. Caldwell and R. D. Rogers, Chem. Commun., 2004, 668–669 RSC.
- R. J. Bernot, E. E. Kennedy and G. A. Lamberti, Environ. Toxicol. Chem., 2005, 24(7), 1759–1765 CrossRef CAS.
- R. J. Bernot, M. A. Brueseke, M. A. Evans-White and G. A. Lamberti, Environ. Toxicol. Chem., 2005, 24(1), 87–92 CrossRef.
- S.-M. Lee, W.-J. Chang, A.-R. Choi and Y.-M. Koo, Korean J. Chem. Eng., 2005, 22(5), 687–690 Search PubMed.
- S. Stole, J. Arning, U. Bottin-Weber, M. Matzke, F. Stock, K. Thiele, M. Uerdingen, U. Welz-Biermann, B. Jastorff and J. Ranke, Green Chem., 2006, 8, 621–629 RSC.
- A. S. Wells and V. T. Coombe, Org. Process Res. Dev., 2006, 10, 794–798 Search PubMed.
- C. Pretti, C. Chiappe, D. Pieraccini, M. Gregori, F. Abramo, G. Monni and L. Intorre, Green Chem., 2006, 8, 238–240 RSC.
- C. R. Blaise, in Ecotoxicology Monitoring, ed. M. L. Richardson, VCH, Weinheim, 1993, pp. 83–108 Search PubMed.
- M. A. Lewis, in Fundamentals of aquatic toxicology: Effects, environment fate, and risk assessment, ed. G. M. Rand, Taylor and Francis, Washington, DC, USA, 2nd edn., 1995, pp. 135–170 Search PubMed.
- A. Latała, P. Stepnowski, M. Nędzi and W. Mrozik, Aquat. Toxicol., 2005, 73(1), 91–98 CrossRef CAS.
- U.S. Environmental Protection Agency, Ecological Effect Test Guidelines, OPPTs 850.5400, Algal Toxicity, Tiers I and II, 1996.
- Organization for Economic Cooperation and Development, Guidelines for the testing of chemicals, Proposal for updating guideline 201, Freshwater alga and cyanobacteria, Growth Inhibition Test, 2002.
- D. Zhao, Y. Liao and Z. Zhang, Clean, 2007, 35, 42–48 Search PubMed.
- M. T. Garcia, N. Gathergood and P. J. Scammells, Green Chem., 2005, 7, 9–14 RSC.
- P. Wasserscheid, R. van Hal and A. Boesmann, Green Chem., 2002, 4, 401–404 Search PubMed.
- J. E. Griffiths and G. E. Walrafen, Inorg. Chem., 1972, 2, 427–429 CrossRef.
- A. E. Visser, R. P. Swatloski, W. M. Reichert, S. T. Griffin and R. D. Rogers, Ind. Eng. Chem. Res., 2000, 39, 3596–3604 CrossRef CAS.
- R. P. Swatloski, J. D. Hobrey and R. D. Rogers, Green Chem., 2003, 5, 361–363 RSC.
- C. Villagrán, M. Deetlefs, W. R. Piter and C. Hardacre, Anal. Chem., 2004, 76, 2118–2123 CrossRef CAS.
- G. A. Baker and S. N. Baker, Aust. J. Chem., 2005, 58, 172–177.
- Y.-S. Yun and J. M. Park, Korean J. Chem. Eng., 1997, 14, 1297–1300 Search PubMed.
|
This journal is © The Royal Society of Chemistry 2008 |
Click here to see how this site uses Cookies. View our privacy policy here.