DOI:
10.1039/B711273D
(Paper)
CrystEngComm, 2008,
10, 79-85
Novel two-dimensional uranyl–organic assemblages in the citrate and D(–)-citramalate families†
Received
23rd July 2007
, Accepted 29th August 2007
First published on 7th September 2007
Abstract
Uranyl nitrate reacts with D(–)-citramalic acid (H3citml) under mild hydrothermal conditions to give the two-dimensional polymer [UO2(Hcitml)] 1, in which each ligand chelates one metal atom through its hydroxyl and α-carboxylate groups and binds to three others in monodentate fashion. The resulting neutral layers display isolated uranyl pentagonal bipyramidal polyhedra. Whereas citric acid (H4cit) has been shown previously to give various three- and monodimensional uranyl–organic assemblages, complexation under hydrothermal conditions in the presence of either NaOH/NEt4Cl or pyridine yields the complexes [NEt4]2[(UO2)3(cit)2(H2O)2]·2H2O 2 and [Hpy]2[(UO2)3(cit)(Hcit)(OH)] 3, respectively, which both crystallize as two-dimensional frameworks. The layers are either planar and separated by the counter ions in 2 or corrugated and hydrogen bonded to one another in 3. In both 2 and 3, [UO2(cit)]24– dimeric subunits with edge-sharing pentagonal bipyramidal uranium coordination polyhedra are present but, in both cases and in contrast with previous structures containing [UO2(Hcit)]22– dimers, the carboxylate group not involved in the dimer formation is coordinated to another uranyl unit, which is part of either a centrosymmetric hexagonal bipyramidal bis-aquated group or a different, [(UO2)2(Hcit)(OH)] dimer. These examples of two-dimensional assemblages further illustrate the variety of architectures which can be obtained with citric and related acids and the important structure-directing effects of the counter ions.
Introduction
The investigation of the uranyl citrate system has been restricted for long to solution studies, which provided a wealth of data on the complexes formed,1 with structural information, in particular, being obtained from EXAFS or energy-dispersive X-ray diffraction.2 However, considering the importance of this system in several areas related to nuclear technology such as treatment of nuclear wastes, soil decontamination3 and phytoremediation4 or studies of radionuclides mobility,5 the lack of crystallographic characterization of uranyl citrates appeared quite frustrating. Only very recently, the first crystal structures of uranyl complexes with citric and related (tricarballylic, D(–)-citramalic) acids were reported,6,7 which also evidenced the potential of these acids for the design of uranyl–organic frameworks (UOFs),8 as further demonstrated by the synthesis of a uranyl–copper hybrid compound.9 Whereas most of the solution studies indicate the presence of a dinuclear dimeric species, [UO2(Hcit)]22–, at low pH values (∼2–4), the crystal structures of the species grown under hydrothermal conditions and with different bases and counter ions constitute a varied set of assemblages. The first structure reported was that of [(UO2)3(Hcit)2(H2O)3]·2H2O (H4cit = citric acid, Scheme 1), obtained in the presence of NaOH, which crystallizes as a three-dimensional framework with two different uranyl environments and all three carboxylate groups coordinated.6 This form of uranyl citrate is quite ubiquitous since it is very easily obtained with a wide range of experimental conditions, but other forms based on the dimeric motif expected from solution studies resulted from the use of diamines as bases.7,9 In several cases, these dimers are assembled in polymeric chains through carboxylate bridges. Analogous dimer-based assemblages are obtained with the related D(–)-citramalic acid [D(–)-2-hydroxy-2-methylbutanedioic acid, H3citml], which lacks one of the –CH2COOH groups.7 Further exploration of different experimental conditions led to the first examples of two-dimensional uranyl–organic frameworks with these ligands, which are reported herein.
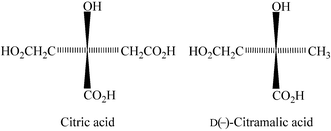 |
| Scheme 1 | |
Experimental
Synthesis
UO2(NO3)2·6H2O was purchased from Prolabo, citric and D(–)-citramalic acids from Fluka. All were used without further purification. Elemental analyses were performed by Analytische Laboratorien GmbH at Lindlar, Germany.
[UO2(Hcitml)] (1).
D(–)-Citramalic acid (44 mg, 0.297 mmol) and UO2(NO3)2·6H2O (150 mg, 0.299 mmol) were dissolved in demineralized water (2.4 mL) and heated at 180 °C under autogenous pressure (ca. 1.1 MPa) in a 15 mL tightly closed glass vessel. Light yellow crystals of complex 1 were obtained within five days. As in the following cases, the product was recovered after filtration and washed with water (30 mg, 24% yield). Anal. calcd. for C5H6O7U
∶
C, 14.43; H, 1.45. Found
∶
C, 14.23; H, 1.38%.
[NEt4]2[(UO2)3(cit)2(H2O)2]·2H2O (2).
Citric acid (55 mg, 0.286 mmol), UO2(NO3)2·6H2O (143 mg, 0.285 mmol), NEt4Cl (50 mg, 0.302 mmol), NaOH (12 mg, 0.300 mmol) and demineralized water (3.5 mL) were placed in a 15 mL tightly closed glass vessel and heated at 180 °C under autogenous pressure (ca. 1.1 MPa). Light yellow crystals of complex 2 were obtained within two days (26 mg, 18% yield on the basis of U). Anal. calcd. for C28H56N2O24U3
∶
C, 22.14; H, 3.72; N, 1.84. Found
∶
C, 21.98; H, 3.73; N, 1.74%.
[Hpy]2[(UO2)3(cit)(Hcit)(OH)] (3).
Citric acid (55 mg, 0.286 mmol), UO2(NO3)2·6H2O (143 mg, 0.285 mmol), pyridine (46 mg, 0.582 mmol) and demineralized water (2.3 mL) were placed in a 15 mL tightly closed glass vessel and heated at 180 °C under autogenous pressure (ca. 1.1 MPa). Light yellow crystals of complex 3 were obtained within two days (106 mg, 82% yield on the basis of U). Anal. calcd. for C22H22N2O21U3
∶
C, 19.37; H, 1.63; N, 2.05. Found
∶
C, 19.04; H, 1.60; N, 1.94%.
The data were collected at 100(2) K on a Nonius Kappa-CCD area detector diffractometer10 using graphite-monochromated Mo Kα radiation (λ = 0.71073 Å). The crystals were introduced in glass capillaries with a protecting “Paratone-N” oil (Hampton Research) coating. The unit cell parameters were determined from ten frames, then refined on all data. The data (combinations of ϕ- and ω-scans giving complete data sets up to θ = 25.7° and a minimum redundancy of 4 for 90% of the reflections) were processed with HKL2000.11 The structures were solved by direct methods with SHELXS-97 and subsequent Fourier-difference synthesis and refined by full-matrix least-squares on F2 with SHELXL-97.12 Absorption effects were corrected empirically with the program SCALEPACK.11 All non-hydrogen atoms were refined with anisotropic displacement parameters. The hydrogen atoms bound to oxygen and nitrogen atoms were found on Fourier-difference maps and all the others were introduced at calculated positions; all were treated as riding atoms with a displacement parameter equal to 1.2 (OH, NH, CH2) or 1.5 (CH3) times that of the parent atom. The absolute configuration of complex 1 as determined from the value of the Flack parameter13 matches which was expected.
Crystal data and structure refinement parameters are given in Table 1 and selected bond lengths and angles in Table 2. The molecular plots were drawn with SHELXTL14 and Balls and Sticks.15
|
1
|
2
|
3
|
Chemical formula |
C5H6O7U |
C28H56N2O24U3 |
C22H22N2O21U3 |
M/g mol–1 |
416.13 |
1518.84 |
1364.51 |
Crystal system |
Monoclinic |
Triclinic |
Monoclinic |
Space group |
P21 |
P![[1 with combining macron]](https://www.rsc.org/images/entities/char_0031_0304.gif) |
P21/n |
a/Å |
8.3067(9) |
8.4980(3) |
10.0669(5) |
b/Å |
9.7312(12) |
9.2992(4) |
29.4919(13) |
c/Å |
10.7272(13) |
13.2045(6) |
11.1076(6) |
α/° |
90 |
86.048(2) |
90 |
β/° |
106.181(8) |
87.745(3) |
107.873(3) |
γ/° |
90 |
88.381(3) |
90 |
V/Å3 |
832.77(17) |
1039.85(7) |
3138.6(3) |
Z
|
4 |
1 |
4 |
D
calc/g cm–3 |
3.319 |
2.425 |
2.888 |
µ (MoKα)/mm–1 |
19.496 |
11.740 |
15.534 |
F(000)
|
736 |
706 |
2448 |
Reflections collected |
24997 |
51509 |
77666 |
Independent reflections |
3159 |
3951 |
5939 |
Observed reflections [I > 2σ(I)] |
2971 |
3748 |
4923 |
R
int
|
0.079 |
0.048 |
0.038 |
Parameters refined |
238 |
263 |
433 |
R1 |
0.034 |
0.020 |
0.032 |
wR2 |
0.084 |
0.049 |
0.081 |
S
|
1.025 |
1.056 |
1.045 |
Flack parameter |
–0.002(19) |
— |
— |
Δρmin/e Å–3 |
–1.85 |
–1.66 |
–1.43 |
Δρmax/e Å–3 |
1.65 |
0.69 |
2.78 |
Table 2 Environment of the metal atoms in compounds 1–3
∶
selected bond lengths (Å) and angles (°)
Symmetry codes∶ 1 ′ = –x, ½ + y, 1 – z; ″ = x, 1 + y, z; # = 2 – x, –½ + y, 2 – z; * = x, –1 + y, z. 2 ′ = 1 – x, –y, 1 – z; ″ = 1 + x, y, z; # = –x, –y, 2 – z. 3 ′ = 1 – x, –y, –z; ″ = 2 – x, –y, 1 – z; # = –½ + x, ½ – y, –½ + z; * = ½ + x, ½ – y, ½ + z. |
1
|
U1–O1 |
1.760(9) |
U2–O3 |
1.755(9) |
|
|
U1–O2 |
1.755(10) |
U2–O4 |
1.754(10) |
|
|
U1–O8 |
2.370(9) |
U2–O9 |
2.292(8) |
|
|
U1–O13 |
2.326(9) |
U2–O14 |
2.392(10) |
|
|
U1–O5′ |
2.498(7) |
U2–O10# |
2.513(9) |
|
|
U1–O6′ |
2.405(9) |
U2–O11# |
2.400(10) |
|
|
U1–O7″ |
2.427(13) |
U2–O12* |
2.424(12) |
|
|
O1–U1–O2 |
178.6(8) |
O3–U2–O4 |
179.2(4) |
|
|
O8–U1–O13 |
83.5(3) |
O9–U2–O14 |
82.8(3) |
|
|
O13–U1–O7″ |
75.8(3) |
O14–U2–O11# |
67.3(3) |
|
|
O7″–U1–O5′ |
73.6(3) |
O11#–U2–O10# |
60.8(3) |
|
|
O5′–U1–O6′ |
60.0(3) |
O10#–U2–O12* |
74.0(3) |
|
|
O6′–U1–O8 |
67.3(3) |
O12*–U2–O9 |
75.3(3) |
|
|
2
|
U1–O1 |
2.377(2) |
U2–O4 |
2.500(2) |
|
|
U1–O2 |
2.373(2) |
U2–O5 |
2.472(2) |
|
|
U1–O1′ |
2.394(2) |
U2–O10 |
1.770(2) |
|
|
U1–O7′ |
2.345(2) |
U2–O11 |
2.449(3) |
|
|
U1–O6″ |
2.423(2) |
|
|
|
|
U1–O8 |
1.783(2) |
|
|
|
|
U1–O9 |
1.777(2) |
|
|
|
|
O1–U1–O2 |
66.51(8) |
O4–U2–O5 |
52.16(8) |
|
|
O2–U1–O6″ |
75.46(8) |
O4–U2–O11 |
63.92(8) |
|
|
O6″–U1–O7′ |
77.07(8) |
O11–U2–O5# |
64.88(8) |
|
|
O7′–U1–O1′ |
73.70(8) |
O10–U2–O10# |
180 |
|
|
O1′–U1–O1 |
67.24(8) |
|
|
|
|
O8–U1–O9 |
178.06(11) |
|
|
|
|
3
|
U1–O1 |
1.789(6) |
U2–O3 |
1.789(6) |
U3–O5 |
1.779(5) |
U1–O2 |
1.792(6) |
U2–O4 |
1.777(6) |
U3–O6 |
1.780(6) |
U1–O1A |
2.362(6) |
U2–O5A |
2.408(7) |
U3–O1B |
2.456(5) |
U1–O4A |
2.367(6) |
U2–O6A |
2.333(6) |
U3–O2B |
2.387(5) |
U1–O1A′ |
2.396(6) |
U2–O1B |
2.374(5) |
U3–O3B# |
2.430(5) |
U1–O2A′ |
2.360(6) |
U2–O4B |
2.387(5) |
U3–O7A* |
2.383(6) |
U1–O5B″ |
2.382(6) |
U2–O7 |
2.272(5) |
U3–O7 |
2.283(5) |
O1–U1–O2 |
177.7(3) |
O3–U2–O4 |
175.4(3) |
O5–U3–O6 |
176.4(3) |
O1A–U1–O4A |
72.40(19) |
O5A–U2–O6A |
75.3(3) |
O1B–U3–O2B |
64.70(17) |
O4A–U1–O5B″ |
73.9(2) |
O6A–U2–O7 |
74.1(2) |
O2B–U3–O7A* |
82.10(18) |
O5B″–U1–O2A′ |
75.1(2) |
O7–U2–O1B |
64.76(17) |
O7A*–U3–O3B# |
77.18(19) |
O2A′–U1–O1A′ |
68.9(2) |
O1B–U2–O4B |
74.53(18) |
O3B#–U3–O7 |
73.72(19) |
O1A′–U1–O1A |
69.9(2) |
O4B–U2–O5A |
72.6(2) |
O7–U3–O1B |
63.25(17) |
Results and discussion
The complex [UO2(Hcitml)] (1) was obtained from D(–)-citramalic acid and uranyl nitrate reacted under hydrothermal conditions in the absence of base. This compound crystallizes with no water molecule, either coordinated or included in the lattice, which, although uncommon in this family of compounds, was already observed in [H2DABCO][UO2(citml)]2 (DABCO = 1,4-diazabicyclo[2.2.2]octane).7 Two uranyl ions and two doubly deprotonated ligands are present in the asymmetric unit, both hydroxyl groups retaining their proton (Fig. 1). The absolute configuration experimentally determined indicates that no racemization of the ligand occurred. All the oxygen atoms of the D(–)-citramalate molecule are coordinated, the α-carboxylate and hydroxyl groups being chelating and the two carboxylate groups being bound to two metal atoms each, through only one oxygen atom in each case, which results in an overall µ4-1κ2O,O′
∶
2κO″
∶
3κO‴
∶
4κO″″ coordination mode for each ligand. The full coordination and assembling potential of the ligand is thus exploited, whereas in the two uranyl D(–)-citramalate complexes previously reported, i.e. the one-dimensional, dimer-based polymer {[H2DABCO][UO2(citml)]2}n and the dimeric [H2-4,4′-bipy][UO2(citml)(H2O)]2·H2O (4,4′-bipy = 4,4′-bipyridine),7 one or four oxygen atoms per dimer, respectively, were uncoordinated, some of them being involved in hydrogen bonds with the ammonium counter ions. In the latter forms, two edge-sharing uranium polyhedra are encompassed by two bis-chelating ligands with deprotonated, bridging hydroxyl groups, whereas the uranyl polyhedra are separated from each other and the ligand is simply chelating in the present case. The hydroxyl groups in 1 are further involved in hydrogen bonds with coordinated carboxylate groups (Table 3). Each uranyl ion is connected to four ligands, one in chelating and three in monodentate fashion, which gives the usual pentagonal bipyramidal uranium environment. The average U–O(hydroxyl) and U–O(carboxylate) bond lengths, 2.506(8) and 2.38(4) Å, respectively, match those in [(UO2)3(Hcit)2(H2O)3]·2H2O, in which the hydroxyl group retains also its proton,6 and, as in this compound and previous uranyl citramalate complexes, the average U–O(β-carboxylate) bond lengths in 1 seem to be slightly, but not quite significantly, smaller than the U–O(α-carboxylate) ones, with values of 2.35(4) and 2.414(12) Å, respectively. This coordination mode results in the formation of a two-dimensional framework consisting in flat layers parallel to (
0 2), with adjacent subunits comprising six metal atoms arranged in a somewhat elliptical shape centered on a C2 axis of pseudo-symmetry and held together by two ligands around a central narrow cavity. All the hydrogen bonds are intra-layer ones and the sheets are simply tightly packed with no solvent molecule included in the inter-layer space and no formation of extended channels.
![Top∶ View of the complex [UO2(Hcitml)] 1. Hydrogen bonds are shown as dashed lines. Displacement ellipsoids are drawn at the 50% probability level. Symmetry codes∶ see Table 2. Bottom∶ View of one layer showing the uranium coordination polyhedra; the other atoms are shown as spheres of arbitrary radii; hydrogen atoms are omitted for clarity.](/image/article/2008/CE/b711273d/b711273d-f1.gif) |
| Fig. 1 Top∶ View of the complex [UO2(Hcitml)] 1. Hydrogen bonds are shown as dashed lines. Displacement ellipsoids are drawn at the 50% probability level. Symmetry codes∶ see Table 2. Bottom∶ View of one layer showing the uranium coordination polyhedra; the other atoms are shown as spheres of arbitrary radii; hydrogen atoms are omitted for clarity. | |
Table 3
Hydrogen bonding geometry in compounds 1–3
|
|
D⋯A/Å |
D–H/Å |
H⋯A/Å |
D–H⋯A/° |
Symmetry codes∶ 1 ′ = –x, ½ + y, 1 – z; # = 2 – x, –½ + y, 2 – z. 2 ′ = 1 – x, –y, 2 – z. 3# = –½ + x, ½ – y, –½ + z; ″ = x, y, 1 + z. |
1
|
O5⋯O6′ |
2.529(12) |
0.99 |
1.56 |
165 |
O10⋯O11# |
2.562(14) |
0.94 |
1.66 |
161 |
2
|
O11⋯O12 |
2.645(4) |
0.92 |
1.79 |
154 |
O11⋯O2′ |
2.947(3) |
0.86 |
2.20 |
145 |
O12⋯O3 |
2.808(3) |
0.97 |
1.85 |
170 |
O12⋯O4′ |
2.740(4) |
0.84 |
1.90 |
177 |
3
|
N1⋯O3A |
2.840(11) |
0.88 |
1.97 |
167 |
N2⋯O5A |
3.021(11) |
0.95 |
2.11 |
159 |
O7⋯O2B# |
2.677(7) |
0.81 |
1.96 |
146 |
O7B⋯O3A″ |
2.713(10) |
0.96 |
1.77 |
166 |
The complex [NEt4]2[(UO2)3(cit)2(H2O)2]·2H2O (2) has been obtained in the presence of NaOH and NEt4Cl (the same compound is also obtained when NaOH is replaced by a twofold stoichiometric amount of pyridine), so as to provide an ammonium counter-ion unable to form hydrogen bonds, in contrast with the ammonium ions used previously which were involved in two divergent hydrogen bonds (H2DABCO, H2-4,4′-bipy) or a single one (H-2,2′-bipy) (with the exception of H2[2.2.2]cryptand which was protonated in the in-in form and thus unable to form intermolecular hydrogen bonds).7 The asymmetric unit in complex 2 comprises two independent uranyl ions (one of them on an inversion centre), one fully deprotonated citrate ligand, one tetraethylammonium counter ion and two water molecules (one coordinated and one free) (Fig. 2). One of the uranyl ions (U1) is part of a 2
∶
2 centrosymmetric dimer analogous to those previously described both in solution2 and in the solid state,7,9 but with a fully deprotonated ligand instead of one retaining a hydroxylic2 or a carboxylic7,9 proton and hence a different charge, i.e. [UO2(cit)]24– instead of [UO2(Hcit)]22–. The two citrate ligands encompassing the two uranyl ions are doubly chelating, with bridging alkoxide groups, and they define one five- and one six-membered chelate rings. The coordination environment of the uranium atom is completed by an oxygen atom from the β-carboxylate group of a neighbouring dimer and it assumes the usual pentagonal bipyramidal geometry with the two polyhedra in the dimer sharing the edge defined by the two alkoxide oxygen atoms. The average U1–O(alkoxide) and U1–O(carboxylate) bond lengths in the dimer, 2.386(8) and 2.359(14) Å, as well as the longer U1–O(carboxylate) bond length with the carboxylate from the neighbouring dimer, 2.423(2) Å, match their counterparts in the previous complexes. The U1⋯U1′ separation, 3.9728(3) Å, is also unexceptional. The five equatorial donor atoms define a mean plane with an r.m.s. deviation of 0.010 Å, the uranium atom being displaced by 0.0223(8) Å. Whereas the second β-carboxylic group of each ligand was uncoordinated in the previous dimeric complexes, and involved in hydrogen bonds with the ammonium counter ions or with carboxylate groups from neighbouring complexes, it is coordinated to the second uranyl unit (U2) in complex 2. The uranium atom U2, being located on an inversion center, is chelated by two such carboxylate groups in a trans arrangement and it is also bound to two water molecules, with a resulting hexagonal bipyramidal environment. The average U2–O(carboxylate) bond length, 2.486(14) Å, is larger than that for U1, in agreement with the increase in the equatorial coordination number, and it matches the value of 2.47(3) Å in six-coordinate uranyl tricarballylate.6 The U2–O(water) bond length, 2.449(3) Å, matches the average value from the Cambridge Structural Database (CSD, Version 5.27),16 which is 2.44(4) Å. The six equatorial donor atoms define a slightly puckered plane with an r.m.s. deviation of 0.140 Å. The overall citrate coordination mode corresponds to µ4-1κ2O,O′
∶
2κ2O,O″
∶
3κO‴
∶
4κ2O″″,O″‴. The two torsion angles defined by the carbon atoms from both β-carboxylate groups and the central carbon atom characterize the citrate conformation;17 their values are 42.0(4) and 53.0(4)° in 2, the corresponding twisted shape enabling coordination of the pendent group on each side of the dimer.
![Top∶ View of the complex [NEt4]2[(UO2)3(cit)2(H2O)2]·2H2O 2. Carbon-bound hydrogen atoms are omitted for clarity. Hydrogen bonds are shown as dashed lines. Displacement ellipsoids are drawn at the 50% probability level. Symmetry codes∶ see Table 2. Bottom∶ View of one layer showing the uranium coordination polyhedra (green∶ six-coordinate, yellow∶ five-coordinate uranyl ions); the other atoms are shown as spheres of arbitrary radii; the counter-ions, solvent molecules and hydrogen atoms are omitted for clarity.](/image/article/2008/CE/b711273d/b711273d-f2.gif) |
| Fig. 2 Top∶ View of the complex [NEt4]2[(UO2)3(cit)2(H2O)2]·2H2O 2. Carbon-bound hydrogen atoms are omitted for clarity. Hydrogen bonds are shown as dashed lines. Displacement ellipsoids are drawn at the 50% probability level. Symmetry codes∶ see Table 2. Bottom∶ View of one layer showing the uranium coordination polyhedra (green∶ six-coordinate, yellow∶ five-coordinate uranyl ions); the other atoms are shown as spheres of arbitrary radii; the counter-ions, solvent molecules and hydrogen atoms are omitted for clarity. | |
As previously observed in [H2-4,4′-bipy][UO2(Hcit)]2, the citrate dimers in 2 are assembled through β-carboxylate bridging to form linear polymeric chains directed along the a axis, with each motif related to its neighbours by translations. Such an arrangement was also found with citramalate ligands in [H2DABCO][UO2(citml)]2. In these two previous cases, the chains were further connected by diammonium ions hydrogen bonded to carboxylate oxygen atoms, whereas in 2, they are connected by the six-coordinate uranyl ions located on the inversion centres, which gives chains directed along the (1 0
) direction. A two-dimensional framework parallel to the ac plane is thus generated, with neighbouring layers related to one another by translations along the b axis. The tetraethylammonium counter ions are located in between whereas the hydrogen bonded solvent water molecules occupy the channels located between successive U2 complex units along the a axis. Compound 2 thus displays a lamellar structure with anionic polymeric layers and isolated cations in the inter-layer spaces.
Complex 3, [Hpy]2[(UO2)3(cit)(Hcit)(OH)], has been obtained in the presence of a twofold excess of pyridine; it is unusual in this series due to the presence of hydroxide bridges. The asymmetric unit comprises three uranyl ions, two citrate ligands in different degrees of deprotonation, one hydroxide ion and two pyridinium counter ions (Fig. 3). Atom U1 and its image by the inversion center are coordinated by two fully deprotonated citrates (molecule A and its image) to form a dimer analogous to that in complex 2 and with similar metal–ligand bond lengths. Its coordination sphere is completed by an oxygen atom from a third citrate moiety (molecule B). Ligand A chelates also a third uranyl group (U2) through both its β-carboxylate groups (and not through only one as in 2), each of these groups providing one oxygen atom. The ligand B chelates two uranium atoms (U2 and U3) by its bridging alkoxide group and its β- and α-carboxylate groups, respectively. The hydroxyl ion (O7) also bridges these two metal atoms. The coordination sphere of U3 is completed by one carboxylate oxygen atom from both molecules A and B. All the uranium atoms are thus in pentagonal bipyramidal environments. Atoms U2 and U3 are in a binuclear subunit close in geometry to the 2
∶
2 dimer, with edge-sharing uranium polyhedra, but with a 2
∶
1 metal
∶
ligand stoichiometry, the second citrate being replaced by a hydroxide bridge. The citrates A and B are thus bound to four metal atoms each (but one carboxylic group of molecule B is uncoordinated and retains its proton), with µ4-1κ2O,O′
∶
2κ2O,O″
∶
3κ2O‴,O″″
∶
4κO″‴ and µ4-1κ2O,O′
∶
2κ2O,O″
∶
3κO‴
∶
4κO″″ coordination modes, respectively, while U1 and U3 are bound to three and U2 to two citrate moieties. The peculiar tris-chelating coordination mode of molecule A is an occurrence never observed before in this family of compounds. The average U2,3–O(alkoxide) and U2,3–O(carboxylate) bond lengths are in agreement with those in the 2
∶
2 dimers, with values of 2.42(4) and 2.39(3) Å; the mean U2,3–O7 bond length, 2.278(6) Å and the U2–O7–U3 angle, 117.4(2)°, are comparable to the mean values for hydroxide bridges reported in the CSD, 2.33(2) Å and 111(3)°, respectively. The U2⋯U3 separation, 3.8924(4) Å, is identical to U1⋯U1′, 3.9001(7) Å, and they are both somewhat shorter than in the previous cases. The five equatorial donor atoms around U2 and U3 define mean planes with r.m.s. deviations of 0.152 and 0.123 Å, respectively, and the dihedral angle between these two planes is 26.2(2)°. The two torsion angles defining the citrate conformation are both gauche for molecule A [49(1) and 61(1)°] but one is anti in molecule B [53.6(9) and 172.7(7)°], which is associated to the uncoordinated pendent arm. The hydroxide, carboxylic and two pyridinium groups are involved in hydrogen bonds with carboxylate oxygen atoms (Table 3).
![Top∶ View of the complex [Hpy]2[(UO2)3(cit)(Hcit)(OH)] 3. Carbon-bound hydrogen atoms are omitted for clarity. Hydrogen bonds are shown as dashed lines. Displacement ellipsoids are drawn at the 30% probability level. Symmetry codes∶ see Table 2. Bottom∶ View of one layer showing the uranium coordination polyhedra; (U1,U1′) and (U2,U3) dimers are in green and yellow, respectively; the other atoms are shown as spheres of arbitrary radii; the counter-ions and hydrogen atoms are omitted for clarity.](/image/article/2008/CE/b711273d/b711273d-f3.gif) |
| Fig. 3 Top∶ View of the complex [Hpy]2[(UO2)3(cit)(Hcit)(OH)] 3. Carbon-bound hydrogen atoms are omitted for clarity. Hydrogen bonds are shown as dashed lines. Displacement ellipsoids are drawn at the 30% probability level. Symmetry codes∶ see Table 2. Bottom∶ View of one layer showing the uranium coordination polyhedra; (U1,U1′) and (U2,U3) dimers are in green and yellow, respectively; the other atoms are shown as spheres of arbitrary radii; the counter-ions and hydrogen atoms are omitted for clarity. | |
The resulting assemblage comprises corrugated layers parallel to (1 0
). The (U1,U1′) and the (U2,U3) dimers in each layer are both arranged in rows perpendicular to the b axis, but, since the latter are twice as numerous as the former, the corresponding rows are more tightly packed. Neighbouring and tilted (U2,U3) dimers are in fact bound to one another in the rows, whereas the parallel (U1,U1′) dimers are isolated from one another and connected through (U2,U3) ones. The layer's corrugation stems from the rows of (U2,U3) dimers being alternately above and below the mean plane defined by the (U1,U1′) dimers, a consequence of the centrosymmetric geometry of the latter and the location of one pendent, U2-coordinated carboxylate group on each side of the mean plane. The packing of the layers is such that the spaces between the (U1,U1′) dimers form channels parallel to the c axis, which are partly occupied by the pyridinium ions. The uncoordinated carboxylic groups are pointing outside on both sides of the layers and they are hydrogen bonded to carboxylate groups of the next layers, while the hydroxide–carboxylate hydrogen bonds are intra-layer ones.
Conclusion
The three crystal structures of uranyl–organic frameworks reported herein further illustrate the wide array of architectures which can be obtained with highly flexible ligands containing a large number (up to seven) of potentially coordinating atoms. The commonest form of uranyl citrate in the solid state appears to be [(UO2)3(Hcit)2(H2O)3]·2H2O, which is obtained in the presence of NaOH with different stoichiometries of the reactants and at temperatures of 140 or 180 °C, and also in the presence of KOH, CsOH or NEt3, or even by direct reaction of citric acid and UO3 in water at 180 °C. This form crystallizes also in the presence of the tetraalkylammonium ions NMe4+, NPr4+ and NBu4+, either alone or in the presence of a base such as NaOH, NEt3 or pyridine, and it is even isolated, instead of complex 2, when NEt4Cl is used without addition of NaOH.18 In this complex, the 3
∶
2 metal
∶
ligand stoichiometry is associated with two different uranyl environments. The hydroxyl group (not deprotonated) is non-bridging and the three carboxylate groups are coordinated. In contrast, the 2
∶
2 dimers and dimer-based polymeric chains isolated in the presence of DABCO, 4,4′- and 2,2′-bipyridine or [2.2.2]cryptand possess bridging alkoxides and one uncoordinated carboxylic acid group. In these respects, complexes 2 and 3 can be viewed as intermediate forms, with both a 3
∶
2 stoichiometry and the presence of dimers and bridging alkoxides, but they differ strongly in other particulars. In complex 2, all carboxylate groups are coordinated, whereas one citrate in complex 3 has a pendent carboxylic group available for hydrogen bond formation. The two-dimensional uranyl–organic frameworks displayed by compounds 1, 2 and 3 are the first to be obtained with these ligands (layers are however observed in uranyl sodium tricarballylate)6 and, moreover, they illustrate three different situations∶ neutral and planar layers in 1, polyanionic planar layers separated by counter ions in 2 and corrugated layers (containing both the polyanionic complex and the hydrogen bonded counter ions) hydrogen bonded to one another in 3. Together with the previous cases with diamines as divergent hydrogen bond linkers or simply as included counter ions, complexes 2 and 3 are examples of the template or structure-directing effects19 which are exercised by the counter ions in this family of compounds, and of the richness and variety of the resulting architectures.
References
-
(a) I. Feldman and W. F. Neuman, J. Am. Chem. Soc., 1951, 73, 2312 CrossRef CAS;
(b) I. Feldman, C. A. North and H. B. Hunter, J. Phys. Chem., 1960, 64, 1224 CrossRef;
(c) K. S. Rajan and A. E. Martell, Inorg. Chem., 1965, 4, 462 CrossRef CAS;
(d) G. Markovits, P. Klotz and L. Newman, Inorg. Chem., 1972, 11, 2405 CrossRef CAS;
(e) M. T. Nunes and V. M. S. Gil, Inorg. Chim. Acta, 1987, 129, 283 CrossRef CAS;
(f) M. Kakihana, T. Nagumo, M. Okamoto and H. Kakihana, J. Phys. Chem., 1987, 91, 6128 CrossRef CAS;
(g) J. J. Lenhart, S. E. Cabaniss, P. MacCarthy and B. D. Honeyman, Radiochim. Acta, 2000, 88, 345 CrossRef CAS;
(h) S. P. Pasilis and J. E. Pemberton, Inorg. Chem., 2003, 42, 6793 CrossRef CAS.
-
(a) P. G. Allen, D. K. Shuh, J. J. Bucher, N. M. Edelstein, T. Reich, M. A. Denecke and H. Nitsche, Inorg. Chem., 1996, 35, 784 CrossRef CAS;
(b) C. J. Dodge and A. J. Francis, Radiochim. Acta, 2003, 91, 525 CrossRef CAS;
(c) E. Vasca, G. Palladino, C. Manfredi, C. Fontanella, C. Sadun and R. Caminiti, Eur. J. Inorg. Chem., 2004, 2739 CrossRef CAS;
(d) E. H. Bailey, J. F. W. Mosselmans and P. F. Schofield, Chem. Geol., 2005, 216, 1 CrossRef CAS.
-
(a) C. J. Dodge and A. J. Francis, Environ. Sci. Technol., 1994, 28, 1300 CrossRef CAS;
(b) F. Y. C. Huang, P. V. Brady, E. R. Lindgren and P. Guerra, Environ. Sci. Technol., 1998, 32, 379 CrossRef CAS;
(c) A. J. Francis and C. J. Dodge, Environ. Sci. Technol., 1998, 32, 3993 CrossRef CAS;
(d) C. W. Francis, M. E. Timpson and J. H. Wilson, J. Hazard. Mater., 1999, 66, 67 CrossRef CAS;
(e) C. J. Dodge and A. J. Francis, Environ. Sci. Technol., 2002, 36, 2094 CrossRef CAS.
-
(a) J. W. Huang, M. J. Blaylock, Y. Kapulnik and B. D. Ensley, Environ. Sci. Technol., 1998, 32, 2004 CrossRef CAS;
(b) S. D. Ebbs, D. J. Brady and L. V. Kochian, J. Exp. Bot., 1998, 49, 1183 CrossRef CAS;
(c) H. Shahandeh and L. R. Hossner, Soil Sci., 2002, 167, 269 CrossRef CAS.
-
(a) A. J. Francis, C. J. Dodge and J. B. Gillow, Nature, 1992, 356, 140 CrossRef CAS;
(b) B. A. Logue, R. W. Smith and J. C. Westall, Environ. Sci. Technol., 2004, 38, 3752 CrossRef CAS.
- P. Thuéry, Chem. Commun., 2006, 853 RSC.
- P. Thuéry, Inorg. Chem., 2007, 46, 2307 CrossRef CAS.
- See, for example∶
(a) J. Y. Kim, A. J. Norquist and D. O'Hare, Chem. Mater., 2003, 15, 1970 CrossRef CAS;
(b) J. Y. Kim, A. J. Norquist and D. O'Hare, Dalton Trans., 2003, 2813 RSC;
(c) L. A. Borkowski and C. L. Cahill, Inorg. Chem., 2003, 42, 7041 CrossRef CAS;
(d) Z. T. Yu, G. H. Li, Y. S. Jiang, J. J. Xu and J. S. Chen, Dalton Trans., 2003, 4219 RSC;
(e) W. Chen, H. M. Yuan, J. Y. Wang, Z. Y. Liu, J. J. Xu, M. Yang and J. S. Chen, J. Am. Chem. Soc., 2003, 125, 9266 CrossRef CAS;
(f) Z. T. Yu, Z. L. Liao, Y. S. Jiang, G. H. Li, G. D. Li and J. S. Chen, Chem. Commun., 2004, 1814 RSC;
(g) M. Frisch and C. L. Cahill, Dalton Trans., 2005, 1518 RSC;
(h) Z. T. Yu, Z. L. Liao, Y. S. Jiang, G. H. Li and J. S. Chen, Chem.–Eur. J., 2005, 11, 2642 CrossRef CAS;
(i) Y. Z. Zheng, M. L. Tong and X. M. Chen, Eur. J. Inorg. Chem., 2005, 4109 CrossRef;
(j) Y. S. Jiang, Z. T. Yu, Z. L. Liao, G. H. Li and J. S. Chen, Polyhedron, 2006, 25, 1359 CrossRef CAS;
(k) W. Zhang and J. Zhao, Inorg. Chem. Commun., 2006, 9, 397 CrossRef CAS;
(l) Y. S. Jiang, G. H. Li, Y. Tian, Z. L. Liao and J. S. Chen, Inorg. Chem. Commun., 2006, 9, 595 CrossRef CAS;
(m) L. A. Borkowski and C. L. Cahill, Cryst. Growth Des., 2006, 6, 2248 CrossRef CAS;
(n) P. Thuéry, Eur. J. Inorg. Chem., 2006, 3646 CrossRef CAS;
(o) P. Thuéry, Polyhedron, 2007, 26, 101 CrossRef CAS;
(p)
C. L. Cahill and L. A. Borkowski, in Structural Chemistry of Inorganic Actinide Compounds, ed. S. V. Krivovichev, P. C. Burns and I. G. Tananaev, Elsevier, Amsterdam, Oxford, 2007, ch. 11 Search PubMed;
(q) C. L. Cahill, D. T. de Lill and M. Frisch, CrystEngComm, 2007, 9, 15 RSC.
- P. Thuéry, CrystEngComm, 2007, 9, 358 RSC.
-
Kappa-CCD Software, Nonius B.V., Delft, The Netherlands, 1998 Search PubMed.
- Z. Otwinowski and W. Minor, Meth. Enzymol., 1997, 276, 307 Search PubMed.
-
G. M. Sheldrick, SHELXS-97 and SHELXL-97, University of Göttingen, Germany, 1997.
- H. D. Flack, Acta Crystallogr., Sect. A: Found. Crystallogr., 1983, 39, 876 CrossRef.
-
G. M. Sheldrick, SHELXTL, Version 5.1, Bruker AXS Inc., Madison, WI, USA, 1999.
-
S. J. Kang and T. C. Ozawa, Balls & Sticks Search PubMed, available from∶ http://www.toycrate.org..
- F. H. Allen, Acta Crystallogr., Sect. B: Struct. Sci., 2002, 58, 380 CrossRef.
- J. P. Glusker, Acc. Chem. Res., 1980, 13, 345 CrossRef CAS.
-
P. Thuéry
, unpublished results.
- See, for example∶
(a) R. J. Francis, P. S. Halasyamani and D. O'Hare, Chem. Mater., 1998, 10, 3131 CrossRef;
(b) P. S. Halasyamani, R. J. Francis, S. M. Walker and D. O'Hare, Inorg. Chem., 1999, 38, 271 CrossRef CAS;
(c) S. M. Walker, P. S. Halasyamani, S. Allen and D. O'Hare, J. Am. Chem. Soc., 1999, 121, 10513 CrossRef CAS;
(d) P. M. Almond, L. Deakin, A. Mar and T. E. Albrecht-Schmitt, Inorg. Chem., 2001, 40, 886 CrossRef CAS;
(e) C. L. Cahill and P. C. Burns, Inorg. Chem., 2001, 40, 1347 CrossRef CAS;
(f) J. A. Danis, W. H. Runde, B. Scott, J. Fettinger and B. Eichhorn, Chem. Commun., 2001, 2378 RSC;
(g) A. J. Norquist, P. M. Thomas, M. B. Doran and D. O'Hare, Chem. Mater., 2002, 14, 5179 CrossRef CAS;
(h) Z. G. Sun, Y. P. Ren, L. S. Long, R. B. Huang and L. S. Zheng, Inorg. Chem. Commun., 2002, 5, 629 CrossRef CAS;
(i) A. J. Norquist, M. B. Doran, P. M. Thomas and D. O'Hare, Dalton Trans., 2003, 1168 RSC;
(j) D. T. de Lill, N. S. Gunning and C. L. Cahill, Inorg. Chem., 2005, 44, 258 CrossRef CAS.
|
This journal is © The Royal Society of Chemistry 2008 |
Click here to see how this site uses Cookies. View our privacy policy here.