Highly sequence specific RNA terminal labeling by DNA photoligation†
Received
30th October 2006
, Accepted 10th November 2006
First published on 28th November 2006
Abstract
We report the nonenzymatic terminal labeling of oligoribonucleotide (ORN) by using template-directed photoligation through 5-carboxyvinyl-2′-deoxyuridine (CVU) with high selectivity.
Introduction
Terminal labeling of RNA by using ligation methods is an important technique in both basic and applied sciences. Template-directed ligation has potential applications in RNA-based biotechnologies,1–3 particularly as tools for preparing the chimeric RNA–DNA strands, and for studying RNA structural and functional analyses. For example, detection of bacterial RNAs with single nucleotide resolution was performed by template-directed ligation.4 Thus, terminal labeling of RNA has become a useful method for monitoring and investigating RNA. Although DNA ligases are useful for the ligation of DNA, enzymatic ligation methods for terminal labeling of RNA face limitations. For example, DNA ligases show low activity with RNAs.5,6 In addition, ligase methods are not likely to be useful in intact cellular or tissue preparations, because it would be difficult to deliver the ligase into cells. Synthesis of chimeric RNA–DNA strands such as lariat RNAs have been reported; nevertheless, the methodologies require the use of cyanogen bromide which is a highly toxic and irritating reagent.7 In our previously work, we reported on the template-directed reversible photoligation with 5-vinyl-2′-deoxyuridine (VU).8 The advantage of using photons as reagents for initiating photoligation is that no additional chemical reagents need to be introduced into the system. A recent photoligation method by using the RNA template showed higher activity than the corresponding DNA template.9 Here, we report on a terminal labeling of ORN by using template-directed photoligation through CVU. We also describe the high selectivity of terminal labeling of ORN by using solid-state assays.
Results and discussion
An oligodeoxynucleotide (ODN) containing CVU, ODN(CVU) (5′-d(CVUGCGTG)-3′), was synthesized by using the cyanoethylphosphoramidite of CVU according to conventional DNA synthesis (Fig. 1a).8 ODN(CVU) was characterized by nucleoside composition and MALDI-TOF-MS (calcd 1878.27 for [M + H]+; found 1878.13). We determined the feasibility of terminal labeling of RNA through ODN(CVU). When ODN(CVU) and ORN(U) (5′-r(UGUGCU)-3′) were irradiated at 366 nm for 32 min in the presence of template ODN(A) (5′-d(CACGCAAGCACA)-3′; Fig. 1b), HPLC showed the appearance of a peak relating to ORN(U)^ODN(CVU) in 96% yield along with the disappearance of the ODN(CVU) and ORN(U) peaks (Fig. 2). MALDI-TOF-MS indicates that the isolated ORN(U)^ODN(CVU) obtained from HPLC purification was a photoligated product of ODN(CVU) and ORN(U) (calcd 3731.43 for [M + H]+; found 3731.15). Enzymatic digestion of isolated ORN(U)^ODN(CVU) showed the formation of rC, rU + dC, rG, dG, and dT in a ratio of 1 : 3 : 2 : 3 : 1 together with rU–CVU photoadduct, which was confirmed by MALDI-TOF-MS (calcd 542.1734 for [M + H]+; found 542.1682). As shown in Fig. 3, the CD spectrum of ORN(U)^ODN(CVU) and ODN(A) showed a spectrum intermediate between B-form duplex and A-form duplex.10 On the other hand, when ODN(CVU) and ORN(C) (5′-r(UGUGCC)-3′) were irradiated at 366 nm for 32 min in the presence of template ODN(G) (5′-d(CACGCAGGCACA)-3′), we observed a peak corresponding to ORN(C)^ODN(CVU), as determined by HPLC.11 As shown in Fig. 4, ORN containing a pyrimidine base at the 3′ terminal site reacted with photoexcited CVU to produce a terminal modified product, but the photoligation rates by using ORN(C) were slower than the corresponding ORN(U). Therefore, template-directed photoligation methods were successful at modifying the 3′-terminal site of RNA.
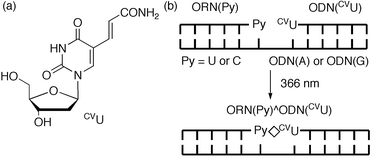 |
| Fig. 1 (a) Structure of 5-carboxyvinyl-2′-deoxyuridine, CVU. (b) Schematic illustration of DNA photoligation at RNA terminal. | |
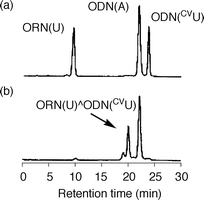 |
| Fig. 2 HPLC analysis of the irradiated ODN(CVU) and ORN(U) in the presence of template ODN(A): (a) before irradiation; (b) after irradiation at 366 nm for 32 min, 96% yield. | |
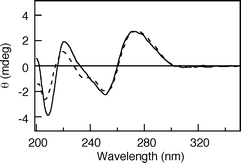 |
| Fig. 3 CD spectra of ORN(U)^ODN(CVU), and template ODN(A) (solid line), and ORN(C)^ODN(CVU), and template ODN(G) (broken line). | |
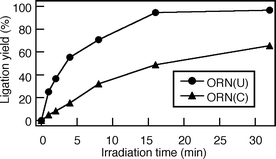 |
| Fig. 4 Time course of photochemical terminal ligation of ORN(U) or ORN(C). | |
We investigated the thermal stability of the RNA–DNA/DNA duplexes containing terminal modified ORN(U)^ODN(CVU) by monitoring the melting temperature (Tm). In Tm measurements of the duplex, sigmoidal curves on the change of A260 were obtained, and the Tm value was calculated from the first part of the curve (Table 1). The Tm value (21.7 °C) of terminal modified ORN(U)^ODN(CVU) and ODN(A) was lower than that of the dodecamer duplex ORN(U)pODN(T) (5′-r(UGUGCU)d(TGCGTG)-3′) and ODN(A) (50.9 °C), whereas the duplex was 7.0 °C more thermally stable than a mixture of ORN(U), ODN(CVU), and the template ODN(A) (14.7 °C).
Table 1
T
m values (°C) for duplexesa
|
T
m/°C |
All Tm values of the duplexes (2.5 µM) were measured in 50 mM sodium cacodylate buffer (pH 7.0) and 100 mM sodium chloride. ORN(C)pODN(T) = 5′-r(UGUGCC)d(TGTGCT)-3′.
|
ODN(CVU), ORN(U)/ODN(A) |
14.7 |
ORN(U)^ODN(CVU)/ODN(A) |
21.7 |
ORN(U)pODN(T)/ODN(A) |
50.9 |
ODN(CVU), ORN(C)/ODN(G) |
22.0 |
ORN(C)^ODN(CVU)/ODN(G) |
40.6 |
ORN(C)pODN(T)/ODN(G) |
58.5 |
To investigate the selectivity of terminal labeling of ORN, we constructed the DNA chip by attaching amino-labeled ODN containing CVU, amino-ODN(CVU) (5′-d(CVUGCGTG)-SSSS-NH2-3′; here S corresponds to a hexa(ethylene glycol) linker fragment), onto the aldehyde-modified glass surface.8,9 A glass chip spotted with 2 µM template ODN(A) and biotin-labeled B-ORN(U) (5′-biotin-r(UGUGCU)-3′), was irradiated at 366 nm for 1 h in 50 mM sodium cacodylate buffer (pH 7.0) and 100 mM sodium chloride (Fig. 5). After the chip had been washed with deionized water at 98 °C for 5 min, a phosphate-buffered saline (PBS) solution of streptavidin-Cy3 conjugate was added to the surface, and the chip was washed twice in PBS. Fluorescence signals were detected on a microarray scanner. When amino-ODN(CVU) and B-ORN(U) were irradiated at 366 nm in the absence of template ODN(A), no terminal modified product was observed (Fig. 6, lane 2). On the other hand, we measured the strong fluorescence signal of the terminal modified product with the completely complementary case (Fig. 6, lane 3). Then we constructed a set of four closely related ORNs with a single variable base (A, U, G, or C) in the third position. The results show that a single nucleotide difference at the third ORN position yielded very little terminal modified product, with a measured fluorescence signal that was 32-fold lower than the completely complementary case (Table 2). Arylazide mediated photocrosslinking of RNA has been reported, but the photogenerated intermediates have broad, indiscriminant reactivity.12 Thus, the high selectivity of the terminal labeling of RNA would be extremely useful for investigating the specific RNA sequence.
Table 2 Normalized fluorescence intensity for the terminal modified product of amino-ODN(CVU), correctly base paired to four ORNs that differed in a single nucleotide position
|
ORNa |
Fluorescence intensityb |
Underlined characters indicate a mismatched base.
Each experiment was repeated at least three times.
|
B-ORN(U) |
5′-Biotin-r(UGUGCU)-3′ |
1.0 ± 0.20 |
B-ORN(A) |
5′-Biotin-r(UG GCU)-3′ |
0.014 ± 0.008 |
B-ORN(G) |
5′-Biotin-r(UG GCU)-3′ |
0.014 ± 0.012 |
B-ORN(C) |
5′-Biotin-r(UG GCU)-3′ |
0.031 ± 0.020 |
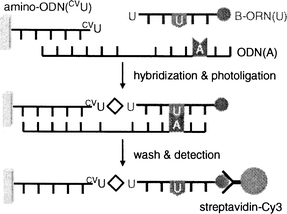 |
| Fig. 5 Schematic illustration for investigating the selectivity of terminal labeling of ORN. | |
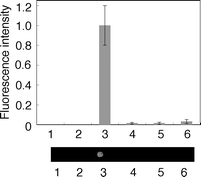 |
| Fig. 6 Fluorescence intensity (top) and images (bottom) acquired on a microarray scanner for the product of photoligation. Lane 1, ODN(A), irradiation at 366 nm; lane 2, B-ORN(U), irradiation at 366 nm; lane 3, B-ORN(U) + ODN(A), irradiation at 366 nm; lane 4, B-ORN(A) + ODN(A), irradiation at 366 nm; lane 5, B-ORN(G) + ODN(A), irradiation at 366 nm; lane 6, B-ORN(C) + ODN(A), irradiation at 366 nm. | |
Conclusions
In conclusion, we demonstrated the terminal labeling of RNA through ODN(CVU). When an ODN containing CVU at the 5′ terminal site was photoirradiated with an ORN containing a pyrimidine base at the 3′ terminal site in the presence of template DNA, efficient terminal labeling was observed. Furthermore, terminal labeling of RNA showed a high degree of single nucleotide specificity. Therefore, this system can be widely used for photochemical post-modification of the terminal site of RNA. This method is useful for incorporating functional units such as fluorophores and electrochemical species. This system is expected to allow the detection of noncoding RNAs and the investigation of RNA structural and functional analyses.
Experimental
General
1H NMR spectra were measured with a Varian Gemini 300 (300 MHz) spectrometer. Coupling constants (J value) are reported in hertz. The chemical shifts are expressed in ppm downfield from tetramethylsilane, using residual chloroform (δ = 7.24 in 1H NMR) and water (δ = 4.65 in 1H NMR) as an internal standard. Mass spectra were recorded on a Voyager-DE PRO-SF, Applied Biosystems. Irradiation was performed by a 25 W transilluminator (FUNAKOSHI, TFL-40, 366 nm, 5700 µW cm−2). HPLC was performed on a Cosmosil 5C18AR column (4.6 × 150 mm) with a JASCO PU-980, HG-980-31, DG-980-50 system equipped with a JASCO UV 970 detector at 260 nm. Kanto Chemical Silica Gel 60 N was used for silica gel column chromatography. Pre-coated TLC plates (Merck silica gel 60 F254) were used for monitoring reactions. The reagents for the DNA synthesizer such as A, G, C, T-β-cyanoethyl phosphoramidite, and CPG support were purchased from Glen Research. Calf intestine alkaline phosphatase (AP) was purchased from Promega. Nuclease P1 was purchased from Yamasa.
Preparation of ODN
ODN sequences were synthesized by the conventional phosphoramidite method by using an Applied Biosystems 3400 DNA synthesizer. The coupling efficiency was monitored with a trityl monitor. The coupling efficiency of cyanoethylphosphoramidite of CVU was 97% yield. The coupling time of cyanoethylphosphoramidite of CVU was 999 sec. They were deprotected by incubation with 28% ammonia for 8 h at 55 °C and were purified on a Chemcobond 5-ODS-H column (10 × 150 mm) by reverse phase HPLC; elution was with 0.05 M ammonium formate containing 3–20% CH3CN, linear gradient (30 min) at a flow rate of 3.0 mL min−1. Preparation of ODNs was confirmed by MALDI-TOF-MS analysis.
Photoligation of ODNs as monitored by HPLC
The reaction mixture (total volume 60 µL) containing ODN(CVC) and ORN(U) (each 20 µM, strand concn) in the presence of template ODN(A) (24 µM, strand concn) in 50 mM sodium cacodylate buffer (pH 7.0) and 100 mM sodium chloride was irradiated with a 25 W transilluminator (366 nm) at 0 °C for 32 min. After irradiation, the progress of the photoreaction was monitored by HPLC on a Cosmosil 5C18AR column (4.6 × 150 mm, elution with a solvent mixture of 50 mM ammonium formate, pH 7.0, linear gradient over 30 min from 4% to 10% acetonitrile at a flow rate of 0.6 mL min−1). The yield was calculated based on ORN(U).
Spectroscopic measurements
The absorbance of the hybrid duplexes was monitored at 260 nm from 4.0 to 80 °C with a heating rate of 1.0 °C min−1 using a JASCO V-550 UV–VIS spectrophotometer or a BECKMAN COULTER DU 800 UV–Visible spectrophotometer. The CD spectra were measured from 200 to 350 nm in a 0.1 cm path length cuvette. All spectra of the duplexes (25 µM, strand concn) were measured at 4.0 °C in a buffer containing 50 mM sodium cacodylate and 100 mM sodium chloride, pH 7.0. CD spectra were recorded on a JASCO J-720W spectrometer.
Immobilization of amino-labeled ODN
The amino-labeled ODN probe containing CVU was diluted to a concentration of 20 µM in 100 mM sodium cacodylate buffer (pH 7.0). Spotting was accomplished by using 4 µL aliquots from a standard micropipette. Binding of the amino-labeled ODN probe containing CVU to the surface was performed over a period of 12 h at room temperature inside a desiccator. After probe immobilization, the glass surface was rinsed with 0.1% SDS and deionized water. The surface was deactivated with a solution made of NaBH4 (3.75 mg), PBS (1.5 mL) and ethanol (375 µL) over a period of 5 min. The surface was subsequently washed with deionized water, and dried.
Terminal labeling of RNA on a DNA chip
A glass chip spotted with a solution (4 µL) made of 2 µM ODN(A) and biotin-labeled ORN(U) in 50 mM sodium cacodylate buffer (pH 7.0) and 100 mM sodium chloride was irradiated at 366 nm for 1 h. After the chip had been washed with deionized water at 98 °C for 5 min, a PBS solution of streptavidin-Cy3 conjugate (20 µg mL−1) was added to the surface, and the chip was washed twice in PBS. Fluorescence measurements were performed on a microarray scanner CRBIO II e (Hitachi), which was equipped with a laser with an excitation wavelength of 532 nm.
Acknowledgements
This article is dedicated to Professor Isao Saito on the occasion of his 65th birthday. This work was supported by a Precursory Research for Embryonic Science and Technology (PRESTO) grant from the Japan Science and Technology Agency (JST). Partial support by a Grant-in-Aid for Scientific Research from the Ministry of Education, Culture, Sports, Science, and Technology, Japan is also acknowledged.
References
- A. P. Silverman and E. T. Kool, Trends Biotechnol., 2005, 23, 225 CrossRef CAS; A. P. Silverman and E. T. Kool, Chem. Rev., 2006, 106, 3775 CrossRef CAS.
- M. M. Mhlanga, D. Y. Vargas, C. W. Fung, F. R. Kramer and S. Tyagi, Nucleic Acids Res., 2005, 33, 1902 CrossRef CAS.
- R. Kawai, M. Kimoto, S. Ikeda, T. Mitsui, M. Endo, S. Yokoyama and I. Hirao, J. Am. Chem. Soc., 2005, 127, 17286 CrossRef CAS; K. Moriyama, M. Kimoto, T. Mitsui, S. Yokoyama and I. Hirao, Nucleic Acids Res., 2005, 33, e129 CrossRef.
- S. Sando and E. T. Kool, J. Am. Chem. Soc., 2002, 124, 9686 CrossRef CAS; S. Sando, H. Abe and E. T. Kool, J. Am. Chem. Soc., 2004, 126, 1081 CrossRef CAS.
- J. Sekiguchi and S. Shuman, Biochemistry, 1997, 36, 9073 CrossRef CAS.
- M. Nilsson, G. Barbany, D. Antson, K. Gertow and U. Landegren, Nat. Biotechnol., 2000, 18, 791 CrossRef CAS.
- S. Carriero and M. J. Damha, J. Org. Chem., 2003, 68, 8328 CrossRef CAS; S. Carriero and M. J. Damha, Nucleic Acids Res., 2003, 31, 6157 CrossRef CAS.
- K. Fujimoto, S. Matsuda, N. Takahashi and I. Saito, J. Am. Chem. Soc., 2000, 122, 5646 CrossRef CAS; I. Saito, Y. Miyauchi, Y. Saito and K. Fujimoto, Tetrahedron Lett., 2005, 46, 97 CrossRef CAS; Y. Yoshimura, Y. Ito and K. Fujimoto, Bioorg. Med. Chem. Lett., 2005, 15, 1299 CrossRef CAS; K. Fujimoto, Y. Yoshimura, T. Ikemoto, A. Nakazawa, M. Hayashi and I. Saito, Chem. Commun., 2005, 3177 RSC; M. Ogino, Y. Yoshimura, A. Nakazawa, I. Saito and K. Fujimoto, Org. Lett., 2005, 7, 2853 CrossRef CAS; S. Ogasawara and K. Fujimoto, ChemBioChem, 2005, 6, 1756 CrossRef CAS; S. Ogasawara and K. Fujimoto, Angew. Chem., Int. Ed., 2006, 45, 4512 CrossRef CAS; K. Fujimoto, S. Matsuda, Y. Yoshimura, T. Matsumura, M. Hayashi and I. Saito, Chem. Commun., 2006, 3223 RSC; Y. Yoshimura, D. Okamura, M. Ogino and K. Fujimoto, Org. Lett., 2006, 8, 5049 CrossRef CAS.
- Y. Yoshimura, Y. Noguchi, H. Sato and K. Fujimoto, ChemBioChem, 2006, 7, 598 CrossRef CAS.
- S. Nakano, T. Kanzaki and N. Sugimoto, J. Am. Chem. Soc., 2004, 126, 1088 CrossRef CAS.
- MALDI-TOF-MS: calcd 3730.34 for ORN(C)^ODN(CVU) [(M + H)+], found 3730.51. The yield was calculated based on ORN(C).
- K. L. Buchmueller, B. T. Hill, M. S. Platz and K. M. Weeks, J. Am. Chem. Soc., 2003, 125, 10850 CrossRef CAS; K. L. Buchmueller and K. M. Weeks, Biochemistry, 2003, 42, 13869 CrossRef CAS.
Footnote |
† Electronic supplementary information (ESI) available: Experimental details. See DOI: 10.1039/b615715g |
|
This journal is © The Royal Society of Chemistry 2007 |