Cyclic sulfamidates as versatile lactam precursors. An evaluation of synthetic strategies towards (−)-aphanorphine
Received
16th October 2006
, Accepted 31st October 2006
First published on 16th November 2006
Abstract
A full account of studies which led to the efficient asymmetric synthesis of (−)-aphanorphine 1 is reported. Two routes to the key cyclic sulfamidate intermediate 5 are described, the first was based on a chiral auxiliary approach and the second utilised asymmetric hydrogenation methodology. A range of C(3)-substituted lactams (4, 22 and 25) were synthesised and evaluated as precursors for Pd(0) catalysed entries (based on (i) α-arylation of a lactam enolate and (ii) reductive Heck reaction) to the 3-benzazepine core of 1. These approaches were less effective than an aryl radical cyclisation which allowed the completion of a synthesis of 1 in 12 steps from anisaldehyde.
Introduction
(−)-Aphanorphine 1 is a tricyclic alkaloid isolated from the freshwater blue-green alga Aphanizomenon flos-aquae.1 The structural resemblance of 1 to benzomorphan analgesics2 such as pentazocine 2 and eptazocine 3 has led to widespread synthetic attention culminating in several approaches to both racemic and enantiopure 1.3
As part of a programme to develop cyclic sulfamidates as N-heterocycle building blocks, we have recently outlined versatile methodologies for the synthesis of a range of enantiopure 5- and 6-ring N-heterocyclic derivatives.4 In particular, the reactions of 1,2- and 1,3-cyclic sulfamidates with functionalised enolates offer a facile and modular entry to α-functionalised pyrrolidinones and piperidinones respectively (Scheme 1).4b,c We have investigated the incorporation of a variety of functionalities at C(3), including esters, phosphonates and sulfides, which then provide a handle for further manipulation and access to other highly functionalised lactam variants.
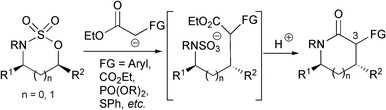 |
| Scheme 1 | |
In order to define further the scope and robustness of this cyclic sulfamidate based methodology we recently reported an efficient asymmetric entry to (−)-aphanorphine 1.5 In this paper we describe full studies directed towards this target molecule and in so doing also provide a further demonstration of the versatility of the heterocyclic strategy that we have developed.
Results and discussion
Our initial approach to (−)-aphanorphine is outlined in Scheme 2 and called for the formation of α-methylated lactam 4 as a precursor for the construction of the core ring system via a palladium-catalysed α-arylation reaction to form the C(1)–C(9a) bond associated with 1. Lactam 4 would in turn be synthesised from cyclic sulfamidate 5 which would be derived from amino acid derivative 6.
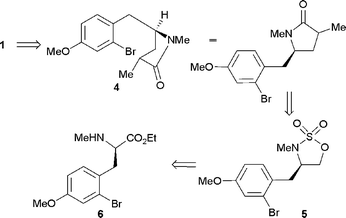 |
| Scheme 2 Retrosynthetic analysis of (−)-aphanorphine 1. | |
Our first generation approach to cyclic sulfamidate 5 is depicted in Scheme 3. Bromination of p-anisaldehyde was achieved in 62% yield in accordance with the procedure described by Durst which employs Comins' methodology for directed ortho-lithiation of aromatic aldehydes.6 A screen of commonly employed electrophilic brominating agents revealed CBr4 to be optimal (Table 1, entry 3).†
Table 1 Brominating agents screened for the formation of 7a
Entrya |
Brominating agent |
Yieldb |
All reactions were performed on a 1.00 mmol scale.
Isolated yield (SM = starting material).
7a and 7b were not separable by chromatography, distillation or crystallisation.
|
1 |
Br2 |
18% (+ 70% SM) |
2 |
BrCH2CH2Br |
6% (+ 81% SM) |
3 |
CBr4 |
62% (+ 22% SM) |
4 |
BrCCl2CCl2Br |
42% (+ 11% SM & 30% 7b)c |
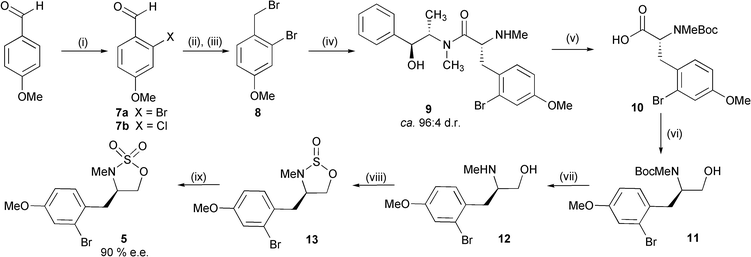 |
| Scheme 3
Reagents and conditions: i, n-BuLi, trimethylethylenediamine, THF, −20 °C, then n-BuLi, −20 °C, then CBr4 (62%) (see Table 1), −78 °C to r.t.; ii, NaBH4, MeOH, THF, r.t.; iii, PBr3, Et2O, 0 °C to r.t. (95% from 7a); iv, (S,S)-(+)-pseudoephedrine sarcosinamide, LDA (1.95 eq.), LiCl (6 eq.), THF, 0 °C, 1 h then bromide 8 (1.05 eq.), 0 °C, (77%); v, NaOH, MeOH, H2O, reflux, then Boc2O, NaHCO3, dioxane, 0 °C to r.t., (99%); vi, EtCO2Cl, Et3N, THF, 0 °C, then NaBH4, H2O; vii, TFA, CH2Cl2, (85% from 10); viii, SOCl2, imidazole, Et3N, CH2Cl2, 0 °C; ix, RuCl3 (0.15 mol%), NaIO4, H2O, EtOAc, 0 °C (81% from 12). | |
Aldehyde 7a was reduced (NaBH4) and brominated (PBr3) to afford benzyl bromide 8 in 95% yield over the two steps.‡ We initially chose to employ an auxiliary controlled protocol to install the key stereocentre present in sulfamidate 5; Myers has previously outlined the use of pseudoephedrine sarcosinamide as a precursor to N-methylated amino acid derivatives.7 Accordingly, alkylation of the dianion derived from (S,S)-(+)-pseudoephedrine sarcosinamide with bromide 8 generated adduct 9 in 77% yield and in approximately 92% d.e. (as estimated by HPLC analysis of peracetylated material).§ Direct hydrolytic cleavage (H2O, dioxane, reflux) of the auxiliary was possible but we were only able to isolate the corresponding amino acid in moderate (45%) yield. In addition, attempted reduction of this species with LiAlH4 to the requisite amino alcohol 12 was unsuccessful due to concomitant reductive debromination. We therefore chose to employ Myers’ alternative base-induced auxiliary cleavage protocol and, after requisite Boc protection, were able to isolate adduct 10 in excellent yield (99%). Reduction of this substrate (to 11) was successful under mixed anhydride conditions and, following Boc deprotection, amino alcohol 12 was isolated in 85% overall yield from 10. This material was converted to cyclic sulfamidate 5via the corresponding cyclic sulfamidite 13 in good overall yield (81%). Critically, the use of EtOAc–H2O8 as solvent for the oxidation step was absolutely essential.¶ When we employed a more commonly used MeCN–H2O solvent system, yields of 5 were significantly lower (14–55%) and irreproducible, presumably due to competing oxidation of the 4-methoxybromobenzyl substituent (Table 2). It therefore appears that the chemoselectivity and/or reactivity of the oxidation step can be tailored via judicious choice of reaction solvent. At this stage we were able to determine the enantiopurity of 5 as 90% e.e. by chiral HPLC (using the corresponding racemate as a standard); this reflects upon the diastereoselectivity of the alkylation step (8
→
9). The route outlined in Scheme 3 proceeds in 9 steps and in 29% overall yield.
Table 2 Optimisation of oxidation conditions for the synthesis of 5
Entrya |
Solvent |
NaIO4 eq. |
Scale |
Time |
Yieldb |
All reactions were performed at 0 °C using 0.15 mol% RuCl3.
Isolated yield.
|
1 |
1 : 1 MeCN–H2O |
1.5 |
0.78 mmol |
2.5 hrs |
14% |
2 |
1 : 1 MeCN–H2O |
1.0 |
0.26 mmol |
50 min |
55% |
3 |
1 : 1 MeCN–H2O |
1.0 |
1.14 mmol |
30 min |
18% |
4 |
2 : 1 MeCN–H2O |
1.0 |
0.98 mmol |
20 min |
40% |
5 |
1 : 1 EtOAc–H2O |
1.0 |
0.80 mmol |
10 min |
82% |
Given the level of enantiomeric excess (90%) associated with 5 and the length of the sequence, we chose to focus upon an alternative hydrogenation strategy to synthesise the key amino alcohol 12. Condensation of bromide 7a with ethyl isocyanoacetate afforded Knoevenagel adduct 14 in 41% yield and as a mixture of geometric isomers (1 : 1 E–Z) (Scheme 4). Unfortunately we were unable to achieve reduction of this substrate (to give 15) with a variety of RhL* systems.9|| Hydrogenation of an N-Boc variant 16 was, however, successful (Scheme 5).
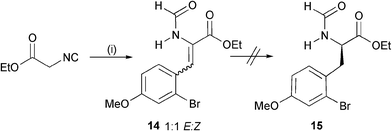 |
| Scheme 4
Reagents and conditions: i, 7a, NaH, THF, 0 °C to r.t. (41%). | |
![Reagents and conditions i, BocNHCH(PO(OMe2))CO2Me, N,N,N′,N′-tetramethylguanidine (TMG), CH2Cl2 (99%); ii, [((R,R)–Et-DuPHOS)Rh(COD)]BF4 (1.5 mol%), H2 (5 bar), MeOH (100%).](/image/article/2007/OB/b614999e/b614999e-s5.gif) |
| Scheme 5
Reagents and conditions i, BocNHCH(PO(OMe2))CO2Me, N,N,N′,N′-tetramethylguanidine (TMG), CH2Cl2 (99%); ii, [((R,R)–Et-DuPHOS)Rh(COD)]BF4 (1.5 mol%), H2 (5 bar), MeOH (100%). | |
Reaction of bromide 7a with Boc-α-phosphonoglycine trimethyl ester in the presence of TMG yielded Boc protected dehydroamino ester 16 in essentially quantitative yield and as a single geometric isomer (>99 : 1 Z–E) which was assigned on the basis of extensive literature precedent.10 Reduction of this species was possible and, of the catalysts evaluated (Table 3), a Rh-Et-DuPHOS system delivered the best conversion and asymmetric induction (>98% e.e. as determined by chiral HPLC) to afford amino acid derivative 17 in quantitative yield.11
Table 3 Hydrogenation catalysts screened for the conversion of 16
→
17
Entrya |
Catalyst |
e.e.b |
Yieldc |
Reaction conditions: catalyst (1.5 mol%), H2 (5 bar), r.t., MeOH, 36 h.
Determined by chiral HPLC using a racemic standard prepared with Wilkinson's catalyst.
Isolated yield.
|
1 |
((R,R)–Et-DuPHOS)Rh(COD)]BF4 |
>98% |
100% |
2 |
((S,S)–i-Pr-DuPHOS)Rh(COD)]BF4 |
n.d. |
8% (+ 92% 16) |
3 |
((R,R)–Me-BPE)Rh(COD)]BF4 |
55% |
100% |
Reduction of both the ester and N-Boc moieties of 17 directly to amino alcohol 12 with LiAlH4 was not possible, due again to concomitant debromination. We therefore examined the possibility of an N-methylation,12 Boc deprotection and chemoselective (0 °C) ester reduction sequence. Although we were able to obtain amino alcohol 12 in 77% yield over these three steps, it was evident upon conversion to the cyclic sulfamidate 5 (62% e.e.) and analysis by chiral HPLC that some epimerisation had occurred, presumably during the base-mediated N-methylation step (Scheme 6).
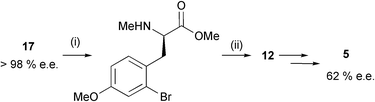 |
| Scheme 6
Reagents and conditions: i, NaH (1.2 eq.), MeI, DMF, r.t. then TFA (82%); ii, LiAlH4, THF, 0 °C, (93%). | |
An alternative strategy for the conversion of amino ester 17 to the target amino alcohol 12 was devised (Scheme 7). Chemoselective reduction of ester 17 with LiAlH4 (0 °C) proceeded smoothly to afford alcohol 18 in 96% yield with the Ar–Br bond (and N-Boc group) still intact. Treatment of this species with NaH effected cyclisation to cyclic carbamate 19. Once this step was complete, as judged by TLC, methyl iodide and further NaH were added to achieve methylation to afford 20.** This intermediate was cleaved hydrolytically and the resulting amino alcohol 12 was isolated in 92% overall yield. Conversion of 12 to cyclic sulfamidate 5 proceeded smoothly using our previously developed conditions and at this stage the enantiopurity of 5 was confirmed as >98% e.e. by chiral HPLC. This sequence allowed us to synthesise the key intermediate 5 in 48% overall yield over 7 steps from p-anisaldehyde (compare against Scheme 3).
 |
| Scheme 7
Reagents and conditions: i, LiAlH4, THF, 0 °C (96%); ii, NaH, THF; iii, NaH, MeI, THF; iv, NaOH, MeOH, reflux (92% from 18). | |
Attention now turned to the synthesis of α-methyl lactam 4. Although we have previously reported reactions of structurally representative cyclic sulfamidates with stabilised enolates as a route to C(3)-substituted lactams, the direct introduction of α-alkyl groups, via simple alkyl ester enolates, has been unsuccessful.4b Sulfamidate 5 did, however, react efficiently with the sodium enolate of α-methyl diethyl malonate to deliver lactam 21 after hydrolysis (to cleave the intermediate N-sulfate) and lactamisation (Scheme 8). Hydrolysis and subsequent decarboxylation afforded lactam 4 as a 2 : 1 mixture of diastereomers in 65% yield from cyclic sulfamidate 5; this sequence was conducted efficiently without purification of intermediate 21. Attempted cyclisation by exposure of lactam 4 to a variety of Pd-catalysed α-arylation protocols was unsuccessful.13 At lower temperatures (<100 °C) starting material remained intact and at higher temperatures (110–140 °C) only debromination was observed under a variety of conditions, including those previously employed by Cossy to α-arylate piperidinones.14a,b We also briefly investigated the possibility of cyclisation via a benzyne intermediate by treating 4 with 2 equivalents of LiTMP but this led to decomposition.15
 |
| Scheme 8
Reagents and conditions: i, methyl diethylmalonate, NaH, DMF, 40 °C then 5 M HCl; ii KOH, dioxane, reflux, then p-xylene, reflux (65% from 5); iii, diethylmalonate, NaH, DMF, r.t. then 5 M HCl (80%); iv, Pd(dba)2 (12 mol%), dppp (18 mol%), t-BuOK, PhMe, 130 °C (17%). | |
More stabilised enolates are generally better coupling partners for Pd-catalysed α-arylation. To this end we treated cyclic sulfamidate 5 with diethyl malonate to afford α-ester lactam 22 in 80% yield and as a 2 : 1 mixture of diastereomers. This species did indeed undergo successful Pd-catalysed cyclisation at higher temperatures to afford tricycle 23 but the reaction was disappointingly inefficient.†† A screen of Pd source (Pd(OAc)2, Pd(dba)2), solvent (DMF, dioxane, p-xylene, toluene), base (Cs2CO3, t-BuONa, t-BuOK), temperature (100–150 °C) and ligand (dppf, dppp, dppe, Xantphos, BINAP) failed to rectify this problem and at best we were able to obtain the tricyclic adduct 23 in only 17% yield (Scheme 8). In all cases, the main byproducts were debrominated and decarboxylated adducts. Decarboxylation could potentially occur either via hydrolysis of ester of 22 to the corresponding acid (due to adventitious water) or via a Krapcho-type process promoted by released bromide.16‡‡
A potentially more robust approach to 1 involves Pd catalysed cyclisation via an alkene (i.e. an intramolecular reductive Heck reaction). Cyclic sulfamidate 5 thus reacted with the potassium enolate of triethylphosphonoacetate to afford, after hydrolysis and lactamisation, α-phosphono lactam 24 in 84% yield (2 : 1 d.r. at C(3)) (Scheme 9). This compound then underwent efficient Horner–Wadsworth–Emmons reaction with paraformaldehyde to afford exo-alkene 25 in 74% yield; in our hands 25 proved to be sensitive to chromatography and was used in subsequent stages without purification. Exposure of 25 to reductive Heck conditions did not lead to cyclisation but routinely resulted in debromination and/or double bond isomerisation within the starting material.17 However, the desired cyclisation could be achieved under radical conditions. Generation of the requisite aryl radical by slow addition (over 1.5 h) of a solution of Bu3SnH and AIBN in PhH to 25 under high dilution conditions resulted in cyclisation to deliver the target tricycle 26 in 62% yield. Reduction of the lactam carbonyl using conditions previously reported by Funk on a synthesis of racemic 1 yielded amine 27 ([α]20D +8.3 (c 0.5, CHCl3); lit. [α]28D +8.1 (c 1.2, CHCl3),3h [α]20D +9.4 (c 0.3, CHCl3),3j [α]20D +8.7 (c 1.06, CHCl3)3n). This constitutes a formal asymmetric synthesis of (−)-1; O-demethylation of amine 27 has been reported by a number of groups in yields ranging from 57–86%.
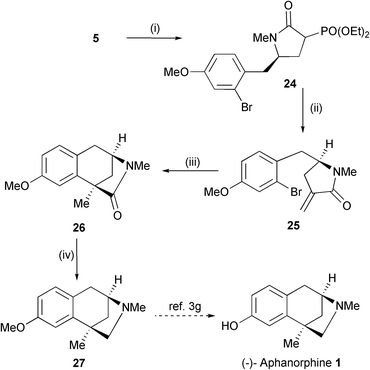 |
| Scheme 9
Reagents and conditions: i, (EtO)2OPCH2CO2Et, t-BuOK, THF, 40 °C then 5 M HCl (84%); ii, NaH, paraformaldehyde, THF (74%); iii, Bu3SnH–AIBN (added over 1.5 h), PhH, reflux (62% + 18% of 30); iv, LiAlH4, THF (93%). | |
The aryl radical cyclisation (25
→
26) is noteworthy, particularly in light of previous related work by Ishibashi3j,m who used a similar strategy to access the tricyclic core of 1. In their system a thio-substituted radical acceptor, as in 28, was required to achieve an efficient cyclisation (Scheme 10). Model studies on the corresponding des-methoxy series had shown that in the absence of such an activating group, 1,5-hydrogen atom abstraction competed leading to the isolation of endo-alkene 29. We also observe the formation of a similar byproduct 30 but in our system there is no requirement for additional activation of the alkene to achieve an efficient cyclisation to form 26. Interestingly the amount of 30 does increase if the Bu3SnH is added over a shorter time period (e.g. addition over 45 minutes affords 26 in 50% yield along with 25% of 30). This observation strongly suggests that 1,5-hydrogen atom abstraction may not be the only pathway corresponding to the formation of 30 and that direct isomerisation may also contribute. We have also briefly investigated using TMS3SiH to carry out this cyclisation but this has proved to be less efficient.18
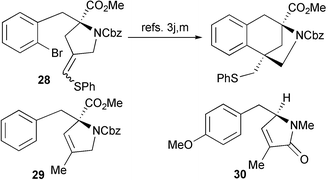 |
| Scheme 10 | |
Conclusions
In summary, we have demonstrated the applicability of cyclic sulfamidate 5 as a key intermediate for the facile synthesis and evaluation of a range of cyclisation precursors leading to the tricyclic core of 1. The availability of enantioenriched alkylating agents, such as 5, was also demonstrated by applying two efficient and general literature methods for the asymmetric synthesis of amino acids. Pd-catalysed cyclisation of the three different precursors investigated was largely unsuccessful and is perhaps a reflection of the steric demands of forming the C(1)–C(9a) bond associated with 1. Aryl radical cyclisation was, however, effective and allowed the completion of an efficient asymmetric synthesis of 1 in 15% overall yield in 12 steps from a commercial starting material. This demonstration of the versatile and modular nature of this cyclic sulfamidate mediated lactam methodology should open the way to its application in other target directed projects.
Experimental
General
General experimental details have been reported recently.4c,5 Experimental procedures associated with the synthesis of (−)-1 which were included as Electronic Supporting Information within ref. 5 have been omitted from here.
(2-Bromo-4-methoxyphenyl)methanol.
19 To a solution of 7a (10.0 g, 46.5 mmol) in THF (10 mL) and MeOH (10 mL) at r.t. was added NaBH4 (879 mg, 23.25 mmol) portionwise over 5 minutes, causing vigorous gas evolution. The reaction mixture was stirred at r.t. for 1.5 h and then concentrated in vacuo. The residue was dissolved in Et2O (100 mL), water (100 mL) and aq. 2 M HCl (10 mL) and the organic portion was isolated, washed with water (2 × 70 mL), dried (Na2SO4) and concentrated in vacuo to afford the alcohol (10.09 g, 100%) as a colourless oil; νmax/cm−1 (film) 3329 (br), 1603 (m), 1490 (m), 1234 (s), 1030 (s); δH (400 MHz, CDCl3) 3.80 (3H, s, ArOCH3), 4.69 (2H, d, J = 6.5, Ar CH2OH), 6.87 (1H, dd, J = 9.0 and 3.0, C5-H), 7.12 (1H, d, J = 3.0, C3–H), 7.35 (1H, d, J = 9.0, C6–H), a signal attributable to –OH was not observed. The spectroscopic properties of this compound were consistent with the data available in the literature.
2-Bromo-1-bromomethyl-4-methoxybenzene 8.
20 To an ice-cooled (0 °C) solution of alcohol (10.09 g, 46.5 mmol) in Et2O (150 mL) was added PBr3 (8.61 mL, 90.6 mmol) (N.B. anhydrous conditions impair the reaction efficiency). The mixture was placed under a N2 atmosphere and allowed to warm slowly to r.t. overnight. The resulting orange solution was then cautiously poured into vigorously stirred ice-cooled (0 °C) water (200 mL) and the organic portion was isolated, washed with saturated aq. NaHCO3 solution (100 mL) and then brine (100 mL), dried (Na2SO4) and concentrated in vacuo to afford bromide 8 (12.37 g, 95%) as a colourless crystalline solid; m.p. 58–59 °C (Et2O) [Lit.20 59–60 °C (CCl4–hexanes)]; νmax/cm−1 (film) 1602 (s), 1494 (m), 1245 (s), 1029 (m); δH (400 MHz, CDCl3) 3.80 (3H, s, ArOCH3), 4.61 (2H, s, ArCH2Br), 6.84 (1H, dd, J = 9.0 and 2.5, C5–H), 7.12 (1H, d, J = 2.5, C3–H), 7.36 (1H, d, J = 9.0, C6–H. The spectroscopic properties of this compound were consistent with the data available in the literature.
(R)-3-(2-Bromo-4-methoxyphenyl)-N-((1S,2S)-2-hydroxy-1-methyl-2-phenylethyl)-N-methyl-2-methylaminopropionamide 9.
Anhydrous LiCl (8.90 g, 210 mmol, obtained by heating commercially available LiCl at 150 °C and 0.01 mmHg for 12 h) was added to a 3 necked, 500 mL round bottomed flask fitted with a thermometer. The reaction vessel was then evacuated (0.01 mmHg) and, with vigorous stirring, the LiCl was further dried using a heatgun. The vessel was allowed to cool to r.t. and then purged with N2. (S,S)-(+)-Pseudoephedrine sarcosinamide7 (8.11 g, 34.4 mmol) was added and the contents of the flask were suspended in anhydrous THF (75 mL) and cooled to 0 °C. The mixture was then deoxygenated by careful evacuation followed by N2 purge (3 cycles). In a separate flask LDA solution was prepared as follows. Anhydrous diisopropylamine (9.64 mL, 68.76 mmol) was dissolved in anhydrous THF (30 mL) and the resulting solution was cooled to 0 °C and then deoxygenated (see earlier). n-BuLi in hexanes (2.49 M, 67.08 mmol) (N.B. the molarity of the n-BuLi solution was accurately determined using the procedure of Watson and Eastham,21 as recommended by Myers) was then added dropwise, via syringe, over 8 minutes and the mixture was stirred at 0 °C for 20 minutes. The LDA solution was then added dropwise via cannula (5 mL THF line wash) to the flask containing the sarcosinamide–LiCl slurry over 28 minutes to form a bright yellow suspension (N.B. the addition rate was maintained such that the internal temperature of the receiving flask did not exceed 5 °C). The mixture was stirred at 0 °C for 35 minutes and then a solution of bromide 8 (10.1 g, 36.1 mmol) in anhydrous THF (20 mL) was added dropwise via syringe over 10 minutes (N.B. the addition rate was maintained such that the internal temperature of the receiving flask did not exceed 5 °C). The mixture was stirred at 0 °C for a further 3 h, during which time it became almost colourless. The mixture was then poured into aq. 1 M HCl (200 mL) and diluted with EtOAc (200 mL). The aqueous portion was isolated and the organic portion was washed with aq. 1 M HCl (200 mL). The combined aqueous portions were then basified to pH 14 by addition of aq. 12.5 M NaOH (during this addition the solution temperature was maintained below 25 °C by use of an ice bath). The mixture was then extracted with CH2Cl2 (5 × 200 mL) and the combined organic extracts were dried (K2CO3) and concentrated in vacuo to afford a pale yellow oil. This crude residue was then purified by FCC (EtOAc–MeOH–Et3N 74 : 3 : 3) to yield the product 9 (11.55 g, 77%, 92% d.e.) as a colourless foam. Crystallisation of this material was unsuccessful under a variety of conditions and diastereomeric enrichment could also not be achieved conveniently by chromatography. NMR analysis of this material was complex due to the presence of two diastereomers, each existing as a mixture of rotamers (one major and one minor) and so full characterisation is presented for the major rotamer of the major diastereomer only; νmax/cm−1 (film) 3318 (br), 1604 (s), 1492 (m), 1241 (m), 1027 (m), 907 (m); δH (400 MHz, CDCl3) 0.77 (3H, d, J = 7.0, CH–CH3), 2.14 (3H, s, NCH3), 2.52 (3H, s, NCH3), 2.67 (1H, dd, J = 13.0 and 9.0, C3–H), 3.04 (1H, dd, J = 13.0 and 6.0, C3–H), 3.71 (3H, s, ArOCH3), 3.76 (1H, dd, J = 9.0 and 6.0, C2–H), 4.47 (1H, d, J = 8.0, CHOH), 4.70 (1H, m, CH–CH3), 6.69 (1H, dd, J = 8.5 and 2.5, ArCH), 7.03 (1H, d, J = 8.5, ArCH), 7.04 (1H, d, J = 2.5, ArCH), 7.10–7.36 (5H, m, ArCH), signals attributable to –OH and –NHCH3 were not observed; δC (100 MHz, CDCl3) 14.2 (CH–CH3), 30.5 and 34.4 (NCH3
× 2), 39.5 (C-3), 55.5 (ArOCH3), 56.2 (CH–CH3), 59.2 (C-2), 75.7 (COH), 113.4, 117.8 and 124.9 (ArC
× 3), 126.7, 127.8 and 128.4 (ArCH × 5), 129.0 (ArC), 132.4 (ArCH), 142.3 and 159.0 (ArC
× 2), 176.1 (C-1); m/z (CI+) 435 and 437 ([M + H]+, 100 and 73%); HRMS: (CI+) Found: [M + H]+ 435.1284, C21H28N2O379Br requires 435.1283; Anal. Calcd for C21H28N2O3Br: C, 57.94; H, 6.25; N, 6.43. Found: C, 58.31; H, 6.17; N, 6.25%. Characteristic 1H NMR signals for minor rotamers/diastereomers: 2.22, 2.34, 2.36, 2.58, 2.82 and 2.88 (6 × NCH3 signals). The diastereomeric purity of this compound was estimated by HPLC of a peracetylated derivative (Ac2O, pyridine) of 9 (Chiralpak AD-H, isocratic hexanes-i-PrOH 80 : 20, 1.0 mL min−1, 25 °C); tR (major) = 10.2 min and tR (minor) = 8.2 min.
(R)-3-(2-Bromo-4-methoxyphenyl)-2-(tert-butoxycarbonyl-methylamino)propionic acid 10.
NaOH pellets (2.88 g, 71.9 mmol) were added to a solution of alkylation adduct 9 (7.136 g, 16.37 mmol) in MeOH (46 mL) and water (33 mL) and the resulting mixture was heated at reflux (ca. 90 °C oil bath temperature) for 22.5 h. The mixture was then cooled to r.t. and MeOH was removed in vacuo causing the formation of a pseudoephedrine precipitate. The mixture was diluted with water (150 mL) and extracted with CH2Cl2 (2 × 150 mL). The combined organic extracts were then washed with water (150 mL). The aqueous portions were combined and concentrated to ca. 150 mL. Dioxane (200 mL) and NaHCO3 (6.04 g, 71.9 mmol) were added and the resulting fine suspension was cooled to 0 °C prior to the addition of Boc2O (4.29 g, 19.7 mmol). The mixture was stirred at 0 °C for 50 minutes and then subsequently stirred at r.t. for 2 h. The mixture was then diluted with water (200 mL) and extracted with EtOAc (200 mL). The organic portion was extracted with aq. 0.2 M NaHCO3 (150 mL) and the aqueous portions were combined before being acidified to pH 3 by careful addition of aq. 1 M HCl. The solution was then extracted with EtOAc (3 × 250 mL) and the organic extracts were combined, washed with water (200 mL) and then brine (100 mL), dried (Na2SO4) and then concentrated in vacuo to afford acid 10 (6.08 g, 99%, 3 : 2 rotamer ratio A–B) as a pale yellow oil; [α]20D +73.3 (c = 1.8, CHCl3); νmax/cm−1 (film) 2976 (br), 1697 (s), 1494 (s), 1243 (m), 1150 (s); δH (400 MHz, CDCl3) 1.34 (9H, s, NCH3CO2C(CH3)3of A), 1.42 (9H, s, NCH3CO2C(CH3)3of B), 2.69 (3H, s, NCH3Boc of B), 2.77 (3H, s, NCH3Boc of A), 3.05 (1H, dd, J = 14.0 and 11.0, C3–Hof A), 3.26 (1H, s, J = 13.5 and 10.5, C3–Hof B), 3.37–3.47 (2H, m, C3–Hof A and B), 3.78 (6H, s, ArOCH3of A and B), 4.64–4.79 (2H, m, C2–Hof A and B), 6.76–6.82 (2H, m, ArCH), 7.03–7.16 (4H, m, ArCH), signals attributable to CO2H were not observed; δC (100 MHz, CDCl3) 28.2 (NCH3CO2C(CH3)3of A), 28.3 (NCH3CO2C(CH3)3of B), 33.1 (NCH3Boc of A), 34.2 (C-3 of B), 34.6 (NCH3Boc of B), 34.9 (C-3 of A), 55.5 (ArOCH3of B), 55.6 (ArOCH3of A), 59.6 (C-2 of A), 60.2 (C-2 of B), 80.7 (NCH3CO2C(CH3)3of B), 80.8 (NCH3CO2C(CH3)3of A), 113.5 (ArCH of A), 113.6 (ArCH of B), 117.9 (ArCH of B), 118.3 (ArCH of A), 124.7 (2 signals) and 128.6 (2 signals) (ArC
× 4), 131.7 (ArCH of A), 131.9 (ArCH of B), 154.9 (ArCof A), 156.1 (ArCof B), 159.0 (NCH3CO2C(CH3)3of B), 159.1 (NCH3CO2C(CH3)3of A), 175.6 (CO2H of B), 176.1 (CO2H of A); m/z (CI+) 390 and 388 ([M + H]+, 18 and 20%), 290 and 288 ([M + H-Boc]+, 93 and 100); HRMS: (CI+) Found: [M + H]+ 388.0753, C16H23NO579Br requires 388.0760.
(E) and (Z) 3-(2-Bromo-4-methoxyphenyl)-2-formylamino-acrylic acid ethyl ester 14.
To a suspension of NaH (60% dispersion in mineral oil, 223 mg, 5.58 mmol) in anhydrous THF (5 mL) at 35 °C was added, via syringe, a solution of ethyl isocyanoacetate (526 mg, 4.65 mmol) and bromide 7a (1.00 g, 4.65 mmol) in anhydrous THF (5 mL) dropwise over 5 minutes to form an orange suspension which was stirred at r.t. for 3 h. After cooling to 0 °C, aq. 1.6 M AcOH (5 mL) was added and the mixture was concentrated in vacuo. The residue was dissolved in CH2Cl2 (50 mL), washed with water (2 × 25 mL), dried (Na2SO4) and concentrated in vacuo to afford an orange solid. Purification by FCC (EtOAc—hexanes 1 : 1–1 : 0) afforded 14 (625 mg, 41%, 1 : 1 mixture of geometric isomers A–B) as a colourless solid; νmax/cm−1 (solid) 3274 (br m), 2940 (br), 1694 (s), 1597 (s), 1488 (s), 1291 (m), 1235 (s), 1027 (s); δH (400 MHz, CDCl3) 1.31–1.42 (6H, m, CO2CH2CH3of A and B), 3.77–3.82 (6H, m, ArOCH3of A and B), 4.25–4.38 (4H, m, CO2CH2CH3of A and B), 6.79 (1H, d, J = 8.0, ArCHof B), 6.86 (1H, d, J = 8.0, ArCHof A), 7.11–7.19 (2H, m, ArCHof A and B), 7.36–7.52 (4H, m, ArCH and C3–H), 7.55 (1H, br s, NHof B), 7.68 (1H, br s, NHof A), 8.10–8.19 (2H, m, NCOHof A and B); δC (100 MHz, CDCl3) 14.2 and 14.3 (CO2CH2CH3), 55.6 and 55.7 (ArOCH3), 62.1 and 62.4 (CO2CH2CH3), 113.6, 114.3, 118.0 and 118.6 (ArCH × 4), 122.9, 124.8, 125.6, 125.8 (2 signals) and 126.5 (C-2 × 2 and ArC
× 4), 126.3 (2 signals), 130.2 and 130.6 (ArCH × 2 and C-3 × 2), 159.1 (NCOH), 160.5 (2 signals) (ArC × 2), 163.8 (NCOH), 164.2 and 164.9 (CO2CH2CH3
× 2); m/z (CI+) 323 and 328 ([M + H]+, 78% and 80%), 284 (100), 282 (98); HRMS: (CI+) Found: [M + H]+ 328.0179, C13H15NO479Br requires 328.0184.
(R)-3-(2-Bromo-4-methoxyphenyl)-2-methylaminopropionic acid methyl ester.
To an ice-cooled (0 °C) solution of ester 17 (284 mg, 0.73 mmol) in anhydrous DMF (2.6 mL) was added MeI (92 µL, 1.47 mmol) and then NaH (60% dispersion in mineral oil, 38 mg, 0.95 mmol) to form a fine suspension which was stirred at r.t. for 14 h. TFA (0.75 mL) was then added and the mixture was stirred for a further 22 h. The mixture was diluted with aq. 1 M HCl (15 mL) and washed with Et2O (2 × 10 mL). The aqueous portion was then neutralised with saturated aq. NaHCO3 and extracted with CH2Cl2 (3 × 10 mL). The combined organic extracts were dried (Na2SO4) and concentrated in vacuo to afford the amine (180 mg, 82%) as a pale yellow oil; [α]20D
−13.6 (c = 0.9, CHCl3); νmax/cm−1 (film) 2949 (m), 1734 (s), 1606 (s), 1495 (s), 1439 (m), 1242 (s), 1030 (s); δH (400 MHz, CDCl3) 2.37 (3H, s, NHCH3), 2.97 (1H, dd, J = 13.5 and 7.5, C3–H), 3.03 (1H, dd, J = 13.5 and 7.0, C3–H), 3.53 (1H, dd, J = 7.5 and 7.0, C2–H), 3.66 (3H, s, CO2CH3), 3.77 (3H, s, ArOCH3), 6.78 (1H, dd, J = 8.5 and 2.5, ArCH), 7.07–7.13 (2H, m, ArCH), no signal attributable to NH was observed; δC (100 MHz, CDCl3) 34.7 (NHCH3), 38.7 (C-3), 51.6 (CO2CH3), 55.4 (ArOCH3), 63.2 (C-2), 113.4 and 118.0 (ArCH × 2), 127.8 and 128.7 (ArC
× 2), 131.6 (ArCH), 158.8 (ArC), 174.8 (C-1); m/z (CI+) 303 ([M + H]+, 100%); HRMS: (CI+) Found: [M + H]+ 302.0383, C12H17NO379Br requires 302.0392. This material was converted to amino alcohol 12 as follows: to an ice-cooled (0 °C) solution of amine (113 mg, 0.37 mmol) in anhydrous THF (2.1 mL) was added, via syringe, a solution of LiAlH4 in THF (1 M, 0.56 mmol) dropwise over 1 minute. The mixture was stirred at 0 °C for 15 minutes and then quenched by sequential addition of water (21 µL), aq. 4 M NaOH (21 µL) and then water (63 µL) to form a colourless precipitate. The mixture was filtered through Celite®, washing with CH2Cl2 (20 mL), and concentrated in vacuo to afford amino alcohol 12 (95 mg, 93%, 62% e.e.) as a pale yellow wax. The enantiomeric purity of this material was determined by conversion to cyclic sulfamidate 5 and subsequent analysis by chiral HPLC.
(R)-3-(2-Bromo-4-methoxyphenyl)-2-methylaminopropan-1-ol 12.
Via Compound 10.
To a solution of acid 10 (6.06 g, 16.1 mmol) in THF (37 mL) at 0 °C was added Et3N (2.47 mL, 17.7 mmol) and then ethyl chloroformate (1.70 mL, 17.7 mmol) causing the immediate formation of a colourless precipitate. The mixture was stirred at 0 °C for 50 minutes and was then filtered (washing with THF (37 mL)). The filtrate was then added dropwise to an ice-cooled (0 °C) suspension of NaBH4 (1.53 g, 40.3 mmol) in water (37 mL) causing vigorous gas evolution. The mixture was stirred at 0 °C for 2.5 h and was then poured into aq. 0.5 M HCl (120 mL) and extracted with CH2Cl2 (3 × 100 mL). The combined organic extracts were washed with brine (100 mL), dried (Na2SO4) and then concentrated in vacuo to afford 11 as a colourless oil. This residue was dissolved in CH2Cl2 (30 mL) and then TFA (30 mL) was added resulting in immediate bubbling. The mixture was stirred at r.t. for 0.5 h and then concentrated in vacuo. The residue was partitioned between CH2Cl2 (100 mL), saturated aq. NaHCO3 (100 mL) and water (50 mL). The organic portion was isolated and the aqueous portion was further extracted with CH2Cl2 (2 × 80 mL). The combined organic extracts were then dried (Na2SO4) and concentrated in vacuo to afford amino alcohol 12 (3.77 g, 85%) as a colourless wax. This material was used for the synthesis of cyclic sulfamidate 5 (90% e.e.) without further purification.5
Characterisation of 12 (in >98% e.e.) has been reported previously.5
(S)-5-(2-Bromo-4-methoxybenzyl)-1,3-dimethylpyrrolidin-2-one 4.
To a solution of methylated diethylmalonate (154 µL, 0.89 mmol) in anhydrous DMF (5 mL) was added, at r.t., NaH (60% dispersion in mineral oil, 35 mg, 0.89 mmol) to form a colourless solution. After 20 minutes sulfamidate 5 (142 mg, 0.42 mmol) was added and the mixture was heated at 40 °C for 17 h. The mixture was then cooled to r.t. and aq. 5 M HCl (0.45 mL) was added. After stirring for 3 h, the mixture was neutralised with saturated aq. NaHCO3 and stirred for a further 0.5 h prior to extraction with Et2O (3 × 20 mL). The combined organic extracts were washed with water (3 × 20 mL), dried (Na2SO4) and concentrated in vacuo to afford a pale yellow oil. This residue was dissolved in dioxane (1 mL) and water (1 mL) and KOH (33 mg, 0.58 mmol) was added. The mixture was then heated at reflux for 1.5 h, cooled to r.t., diluted with aq. 1 M HCl (20 mL) and extracted with CH2Cl2 (3 × 20 mL). The combined organic extracts were then dried (Na2SO4) and concentrated in vacuo. The residue was dissolved in p-xylene (2 mL) and heated at reflux for 14 h. The mixture was then cooled to r.t. and concentrated in vacuo. The residue was then purified by FCC (EtOAc–petrol 1 : 1) to afford the lactam 4 (87 mg, 65%, 2 : 1 d.r. A–B) as a colourless oil; νmax/cm−1 (film) 1686 (s), 1605 (m), 1493 (m), 1242 (m), 1028 (m); δH (400 MHz, CDCl3) 1.15 (3H, d, J = 7.0, C3–CH3of B), 1.21 (3H, d, J = 7.0, C3–CH3of A), 1.33 (1H, ddd, J = 13.0, 9.0 and 8.0, C4–Hof A), 1.59 (1H, ddd, J = 13.0, 9.0 and 8.0, C4–Hof B), 2.06 (1H, ddd, J = 13.0, 8.5 and 2.5, C4–Hof B), 2.15 (1H, ddd, J = 13.0, 9.0 and 7.0, C4–Hof A), 2.31–2.42 (1H, m, C3–Hof A), 2.45–2.54 (2H, m, C3–Hof B and C5–CH2of A), 2.57 (1H, dd, J = 13.5 and 9.0, C5–CH2of B), 2.88 (3H, s, NCH3of B), 2.91 (3H, s, NCH3of A), 3.18 (1H, dd, J = 13.5 and 4.5, C5–CH2of B), 3.41 (1H, dd, J = 13.0 and 4.5, C5–CH2of A), 3.67–3.75 (2H, m, C5–Hof A and B), 6.81–6.84 (2H, m, ArCH), 7.70–7.13 (4H, m, ArCH); δC (100 MHz, CDCl3) 16.3 and 16.9 (C2–CH3of A and B), 28.2 (NCH3of A), 28.5 (NCH3of B), 32.3 (C-4 of A), 33.6 (C-4 of B), 34.7 (C5–CH2of B), 36.3 (C5–CH2of A), 37.8 (C-3 of A), 40.0 (C-3 of B), 55.5 (2 signals) (ArOCH3of A and B), 57.7 (C-5 of B), 58.2 (C-5 of A), 113.7 and 113.8 and 118.3 (2 signals) (ArCH × 4), 124.8, 124.9, 128.4 and 128.8 (ArC
× 4), 131.7 (2 signals) (ArCH × 2), 159.0 (2 signals) (ArC
× 2), 177.2 (C-2 of A), 177.8 (C-2 of B); m/z (CI+) 314 and 312 ([M + H]+, 90 and 100%); HRMS: (CI+) Found: [M + H]+ 312.0584, C14H19NO279Br requires 312.0599.
(S)-5-(2-Bromo-4-methoxybenzyl)-1-methyl-2-oxopyrrolidine-3-carboxylic acid ethyl ester 22.
To a solution of diethylmalonate (452 µL, 2.98 mmol) in anhydrous DMF (17 mL) was added NaH (60% dispersion in mineral oil, 119 mg, 2.98 mmol) to form a colourless solution which was stirred at r.t. for 30 minutes. Sulfamidate 5 (500 mg, 1.49 mmol) was then added and the mixture was stirred at r.t. for 15 h. Aq. 5 M HCl (1.5 mL) was then added and, after stirring at r.t. for 3 h, the mixture was neutralised with saturated aq. NaHCO3 and extracted with Et2O (3 × 25 mL). The combined organic portions were washed with water (3 × 25 mL), dried (Na2SO4) and concentrated in vacuo to afford a pale yellow oil which was purified by FCC (EtOAc–petrol 3 : 2) to yield lactam 22 (441 mg, 80%, 2 : 1 d.r. A–B) as a colourless, amorphous solid; m.p. 58–60.5 °C (Et2O–CH2Cl2); νmax/cm−1 (film) 1735 (m), 1690 (s), 1493 (m), 1241 (m), 1031 (m); δH (400 MHz, CDCl3) 1.28 (3H, t, J = 7.0, OCH2CH3of A), 1.33 (3H, t, J = 7.0, CO2CH2CH3of B), 2.00–2.24 (3H, m, C4–Hof A and B), 2.35 (1H, dt, J = 13.0 and 8.0, C4–Hof A), 2.57 (1H, dd, J = 13.5 and 9.0, C5–CH2of A), 2.68 (1H, dd, J = 13.5 and 10.5, C5–CH2of B), 2.92 (3H, s, NCH3of A), 2.94 (3H, s, NCH3of B), 3.24 (1H, dd, J = 13.5 and 4.5, C5–CH2of A), 3.35–3.43 (3H, m, C5–CH2of B and C3–Hof A and B), 3.79 (6H, s, ArOCH3of A and B), 3.74–3.86 (1H, m, C5–Hof B), 3.86–3.94 (1H, m, C5–Hof A), 4.24–4.29 (4H, m, CO2CH2CH3of A and B), 6.80–6.86 (2H, m, ArCHof A and B), 7.07 (1H, d, J = 8.5, ArCHof A), 7.11–7.15 (2H, m, ArCHof A and B), 7.25 (1H, d, J = 8.5, ArCHof B); δC (100 MHz, CDCl3) 14.1 and 14.2 (CO2CH2CH3of A and B), 27.0 (C-4 of B), 27.8 (C-4 of A), 28.7 (2 signals) (NCH3of A and B), 38.3 (C5–CH2of B), 39.2 (C5–CH2of A), 47.3 (C-3 of A), 47.9 (C-3 of B), 55.6 (2 signals) (ArOCH3of A and B), 58.0 (C-5 of B), 58.5 (C-5 of A), 61.6 (CO2CH2CH3of A), 61.8 (CO2CH2CH3of B), 113.7, 113.9, 118.3 and 118.4 (ArCH × 4), 124.7, 125.0, 128.0 and 128.3 (ArC
× 4), 131.6 and 132.3 (ArCH × 2), 159.2 (2 signals) (ArC
× 2), 169.7, 169.9, 170.3 and 170.6 (C-2 and CO2CH2CH3of A and B); m/z (CI+) 372 and 370 ([M + H]+, 87 and 100%); HRMS: (CI+) Found: [M + H]+ 370.0654, C16H20NO479Br requires 370.0654; Anal. Calcd for C16H20NO4Br: C, 51.91; H, 5.44; N, 4.12. Found: C, 52.10; H, 5.70; N, 3.88%.
(1S,9R)-4-Methoxy-10-methyl-11-oxo-10-azatricyclo-[7.2.1.02,7]dodeca-2,4,6-triene-1-carboxylic acid ethyl ester 23.
Bromide 22 (40 mg, 0.10 mmol), t-BuOK (20 mg, 0.18 mmol, freshly sublimed at 180 °C and ca. 0.01 mmHg), Pd(dba)2 (6.9 mg, 0.012 mmol) and dppp (7.4 mg, 0.018 mmol) were placed in a re-sealable tube, dissolved in anhydrous toluene (0.5 mL, degassed by three freeze–pump–thaw cycles) and heated at 130 °C for 15 h. The reaction mixture was then cooled to r.t., diluted with saturated aq. NH4Cl solution (2 mL) and extracted with EtOAc (3 × 2 mL). The organic extracts were dried (Na2SO4) and concentrated in vacuo to afford a pale brown oil which was then purified by preparative TLC (EtOAc–hexanes 1 : 1) to afford tricycle 23 (5.8 mg, 17%) as a colourless oil; [α]20D +40.0 (c = 0.4, CHCl3); νmax/cm−1 (film) 1739 (s), 1695 (m), 1232 (m); δH (400 MHz, CDCl3) 1.36 (3H, t, J = 7.2, CO2CH2CH3), 2.27 (1H, d, J = 11.0, C1–CH2), 2.72 (1H, dd, J = 11.0 and 5.5, C1–CH2), 2.83 (3H, s, NCH3), 2.94 (2H, d, J = 2.5, C8–H), 3.78 (3H, s, ArOCH3), 3.92 (1H, dt, J = 5.5 and 2.5, C9–H), 4.32–4.42 (2H, m, CO2CH2CH3), 6.79 (1H, dd, J = 8.5 and 2.5, C5–H), 7.01 (1H, d, J = 8.5, C6–H), 7.22 (1H, d, J = 2.5, C3–H); δC (125 MHz, CDCl3) 14.4 (CO2CH2CH3), 27.9 (NCH3), 29.8 (C-8), 37.9 (C1–CH2), 54.6 (ArOCH3), 55.4 (C-9), 56.2 (C-1), 61.5 (CO2CH2CH3), 111.4 (C-3), 114.8 (C-5), 123.8 (C-7), 131.2 (C-6), 135.7 (C-2), 158.2 (C-4), 169.6 and 171.5 (C-11 and CO2CH2CH3); m/z (CI+) 290 ([M + H]+, 100%); HRMS: (CI+) Found: [M + H]+ 290.1382, C16H20NO4 requires 290.1392.
Acknowledgements
We acknowledge EPSRC and GSK for financial support of this research programme, and Dow Pharma for providing the Rh hydrogenation catalysts used in this study
References
- N. Gulavita, A. Hori, Y. Shimizu, P. Laszlo and J. Clardy, Tetrahedron Lett., 1988, 29, 4381 CrossRef CAS.
- For a review of the synthesis of pentazocine 2 and related benzomorphans, see: D. C. Palmer and M. J. Strauss, Chem. Rev., 1977, 77, 1 Search PubMed.
- For total and formal syntheses of (−)-1, see:
(a) S. Takano, K. Inomata, T. Sato, M. Takahashi and K. Ogasawara, J. Chem. Soc., Chem. Commun., 1990, 290 RSC;
(b) A. N. Hulme, S. S. Henry and A. I. Meyers, J. Org. Chem., 1995, 60, 1265 CrossRef;
(c) K. O. Hallinan and T. Honda, Tetrahedron, 1995, 51, 12211 CrossRef CAS;
(d) A. Fadel and P. Arzel, Tetrahedron: Asymmetry, 1995, 6, 893 CrossRef CAS;
(e) A. I. Meyers, W. Schmidt and B. Santiago, Heterocycles, 1995, 40, 525 CrossRef CAS;
(f) M. Node, H. Imazato, R. Kurosaki, Y. Kawano, T. Inoue, K. Nishide and K. Fuji, Heterocycles, 1996, 42, 811 CrossRef CAS;
(g) S. Shiotani, H. Okada, K. Nakamata, T. Yamamoto and F. Sekino, Heterocycles, 1996, 43, 1031 CrossRef CAS;
(h) M. Shimizu, T. Kamikubo and K. Ogasawara, Heterocycles, 1997, 46, 21 CrossRef CAS;
(i) A. Fadel and P. Arzel, Tetrahedron: Asymmetry, 1997, 8, 371 CrossRef CAS;
(j) O. Tamura, T. Yanagimachi, T. Kobayashi and H. Ishibashi, Org. Lett., 2001, 3, 2427 CrossRef CAS;
(k) K. Tanaka, T. Taniguchi and K. Ogasawara, Tetrahedron Lett., 2001, 42, 1049 CrossRef CAS;
(l) A. S. ElAzab, T. Taniguchi and K. Ogasawara, Heterocycles, 2002, 56, 39 CrossRef CAS;
(m) O. Tamura, T. Yanagimachi and H. Ishibashi, Tetrahedron: Asymmetry, 2003, 14, 3033 CrossRef CAS;
(n) H. Zhai, S. Luo, C. Ye and Y. Ma, J. Org. Chem., 2003, 68, 8268 CrossRef CAS;
(o) S. K. Taylor, M. Ivanovic, L. J. Simons and M. M. Davis, Tetrahedron: Asymmetry, 2003, 14, 743 CrossRef CAS;
(p) Y. Kita, J. Futamura, Y. Ohba, Y. Sawama, J. K. Ganesh and H. Fujioka, J. Org. Chem., 2003, 68, 5917 CrossRef CAS;
(q) H. Hu and H. Zhai, Synlett, 2003, 2129 CAS;
(r) For syntheses of (+)-1, see: S. Takano, K. Inomata, T. Sato and K. Ogasawara, J. Chem. Soc., Chem. Commun., 1989, 1591 Search PubMed;
(s) M. Katoh, H. Inoue, A. Suzuki and T. Honda, Synlett, 2005, 2820 CAS;
(t) For syntheses of (±)-1, see: T. Honda, A. Yamamoto, Y. Cui and M. Tsubuki, J. Chem. Soc., Perkin Trans. 1, 1992, 531 Search PubMed;
(u) J. R. Fuchs and R. L. Funk, Org. Lett., 2001, 3, 3923 CrossRef CAS.
-
(a) A. J. Williams, S. Chakthong, D. Gray, R. M. Lawrence and T. Gallagher, Org. Lett., 2003, 5, 811 CrossRef CAS;
(b) J. F. Bower, J. Švenda, A. J. Williams, J. P. H. Charmant, R. M. Lawrence, P. Szeto and T. Gallagher, Org. Lett., 2004, 6, 4727 CrossRef CAS;
(c) J. F. Bower, S. Chakthong, J. Švenda, A. J. Williams, R. M. Lawrence, P. Szeto and T. Gallagher, Org. Biomol. Chem., 2006, 4, 1868 RSC.
- J. F. Bower, P. Szeto and T. Gallagher, Chem. Commun., 2005, 5793 RSC.
-
(a) Y. Lear and T. Durst, Can. J. Chem., 1997, 75, 817 CAS;
(b) D. L. Comins and J. D. Brown, J. Org. Chem., 1984, 49, 1078 CrossRef CAS.
-
(a) A. G. Myers, J. L. Gleason and T. Y. Yoon, J. Am. Chem. Soc., 1995, 117, 8488 CrossRef CAS;
(b) A. G. Myers, J. L. Gleason, T. Y. Yoon and D. W. Kung, J. Am. Chem. Soc., 1997, 119, 656 CrossRef CAS;
(c) A. G. Myers and J. L. Gleason, Org. Synth., 1999, 76, 57 CAS.
- D. Alker, K. J. Doyle, L. M. Harwood and A. McGregor, Tetrahedron: Asymmetry, 1990, 1, 877 CrossRef CAS.
- After completion of this work, a report on the successful Rh-catalysed asymmetric hydrogenation of N-formyl dehydroamino esters, related to 14, was published, see: L. Panella, A. M. Aleixandre, G. J. Kruidhof, J. Robertus, B. L. Feringa, J. G. de Vries and A. J. Minnaard, J. Org. Chem., 2006, 71, 2026 Search PubMed.
- U. Schmidt, A. Lieberknecht and J. Wild, Synthesis, 1984, 53 CrossRef CAS.
- M. J. Burk, J. E. Feaster, W. A. Nugent and R. L. Harlow, J. Am. Chem. Soc., 1993, 115, 10125 CrossRef CAS.
- J. R. Coggins and N. L. Benoiton, Can. J. Chem., 1971, 49, 1968 CAS.
- For a review on Pd catalysed α-arylation reactions, see: D. A. Culkin and J. F. Hartwig, Acc. Chem. Res., 2003, 36, 234 Search PubMed.
-
(a) J. Cossy, A. de Filippis and D. G. Pardo, Org. Lett., 2003, 5, 3037 CrossRef CAS;
(b) J. Cossy, A. de Filippis and D. G. Pardo, Synlett, 2003, 2171 CrossRef CAS;
(c) For a recent example of an intramolecular Pd-catalysed α-arylation reaction employing a piperidinone, see: C. Botuha, C. M. S. Galley and T. Gallagher, Org. Biomol. Chem., 2004, 2, 1825 Search PubMed.
- J. D. Stewart, S. C. Fields, K. S. Kochhar and H. W. Pinnick, J. Org. Chem., 1987, 52, 2110 CrossRef CAS.
- A. P. Krapcho, G. A. Glynn and B. J. Grenon, Tetrahedron Lett., 1967, 8, 215 CrossRef.
- For two recent applications of intramolecular reductive Heck couplings in natural product synthesis, see:
(a) M. Ichikawa, M. Takahashi, S. Aoyagi and C. Kibayashi, J. Am. Chem. Soc., 2004, 126, 16553 CrossRef CAS;
(b) B. M. Trost, O. R. Thiel and H. C. Tsui, J. Am. Chem. Soc., 2003, 125, 13155 CrossRef CAS . These processes both employ electron deficient alkenes as key components.
- B. Giese, B. Kopping and C. Chatgilialoglu, Tetrahedron Lett., 1989, 30, 681 CrossRef CAS.
- W. G. Earley, J. A. Dority, V. Kumar and J. P. Mallamo, Heterocycles, 1995, 41, 309 CrossRef CAS.
- A. K. Ghosh, J. K. Mukhopadhyaya and U. R. Ghatak, J. Chem. Soc., Perkin Trans. 1, 1997, 2747 RSC.
- S. C. Watson and J. F. Eastham, J. Organomet. Chem., 1967, 9, 165 CrossRef CAS.
Footnotes |
† 1,2-Dibromotetrachloroethane was effective on a smaller scale (0.3 mmol) and only traces of chlorinated adduct 7b were observed (<10%). On a larger scale, chlorination is most likely promoted by inferior reaction exotherm control during addition of the brominating agent. |
‡ Bromination is best conducted using reagent grade Et2O; anhydrous Et2O slows the reaction and results in slightly diminished yields of 8 (85% vs. 95%). |
§ We have been unable to enrich this d.e. by chromatography or crystallisation. |
¶ The oxidation step is most efficient on a small scale (100 mg; 82%) but can be conducted on larger scales (2 g) although yields were more variable (57–74%). |
|| The reason for the failure of this reaction is at present unclear.9 |
** The intermediacy of 19 and 20 is inferred although analogous intermediates have been isolated on a model des-bromo series. |
†† At lower temperature (<100 °C) either oxidative addition of Pd(0) into the Ar–Br bond is slow or cyclisation does not occur. |
‡‡ An attempt was made to sequester released bromide by addition of AgOTf but this led to decomposition. |
|
This journal is © The Royal Society of Chemistry 2007 |