DOI:
10.1039/B511140D
(Paper)
Soft Matter, 2006,
2, 60-65
Mesogenic dimers composed of a calamitic and a bent-core mesogenic unit†
Received
4th August 2005
, Accepted 23rd September 2005
First published on 20th October 2005
Abstract
Three mesogenic dimers have been synthesized in which a five-ring bent-core moiety is connected with different calamitic units via flexible spacers. The mesophase behavior of the dimers have been investigated by polarizing microscopy, differential scanning calorimetry, X-ray diffraction on oriented samples and by dielectric and electro-optical measurements. We found that two dimers exhibit a dimorphism columnar–nematic whereas the third one forms a columnar phase only. On the basis of the X-ray data a possible structure model of one of the columnar phases is proposed. The nematic phase exhibits unusual properties. A smectic-like texture can be induced by applying an electric field, which is unknown for nematic phases formed by rod-like mesogens.
Introduction
Liquid crystals represent a unique state of matter which is immediate in symmetry and structure between the solid crystalline state and the isotropic liquid state. To a great extent the type of thermotropic liquid crystalline phases is determined by the shape of the molecules. Rod-like molecules (“calamitic” compounds) preferably form either nematic or smectic phases which possess a one-dimensional density modulation. Disc-like molecules (“discotic” compounds) can also exhibit nematic phases, but they preferably form columnar phases with a two-dimensional modulated structure. If the shape of the molecules significantly deviates from the classic rod- or disc-like shape new phases as well as subphases of well-known phases occur. An interesting example is bent-core mesogens which are the subject of extensive investigations during the last few years in particular because they show new mesophases with unusual properties compared to smectic phases formed by calamitic compounds. Due to their shape the molecules can be packed in a polar fashion giving rise to new smectic mesophases with a long range correlation of the lateral dipoles, i.e., to smectic phases with ferroelectric or antiferroelectric properties.1–3
The paper under study is concerned with a new type of mesogenic dimers. Mesogenic dimers consist, in principle, of two mesogenic units which are connected by a flexible spacer4. In most cases the mesogenic dimers described in the literature are symmetric, i.e., the connected mesogenic units are identical.4,5 Nevertheless, the mesophase behavior of the dimers can strongly deviate from that of the “monomers”. Of special interest are dimers where structurally quite different mesogenic units are linked, for example polar and non-polar monomers6 or chiral and achiral monomers.7
In this paper we present new mesogenic dimers in which one mesogenic unit possesses a bent shape whereas the other one is a weakly polar calamitic unit of different size. The combination of bent and calamitic mesogenic units in the dimers under discussion should clarify whether and to what extent the mesophase behavior of the monomers can be modified. For example, the question arises if this combination can lead to polymorphism variants with “banana phases” as well as mesophases typical for calamitic compounds (N, SmA, SmC) which could be realized in binary mixtures of bent-core and calamitic compounds.8,9 Note that recently a twin molecule was reported where a bent-core unit is linked with a terminal-polar calamitic mesogen.10 This compound forms a biaxial nematic (Nb) and a biaxial SmA phase (SmAb).
Results and discussion
Synthesis of the compounds 1a–1c and their phase transition temperatures
The transition temperatures of the final compounds 1a–c, of the corresponding intermediates 2a–c and 3a–c as well as calamitic mesogens 4a–c are listed in Table 1, the twin mesogens were prepared as sketched in Fig. 1.
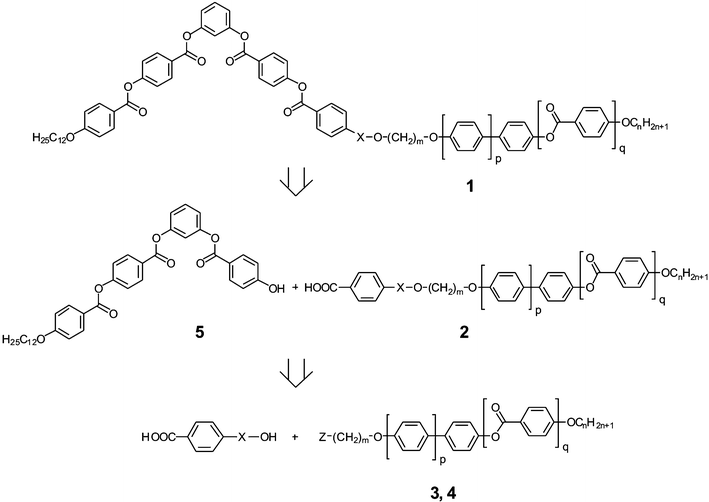 |
| Fig. 1 Retrosynthetic path to prepare compounds 1a–c. | |
Table 1 Mesophase behavior of the dimers 1a–c, intermediates 2a–c and 3a–c as well as of corresponding calamitic compounds 4a–c
No. |
X |
Z |
m
|
p
|
q
|
n
|
Mesophase behaviour |
1a
|
— |
— |
11 |
1 |
1 |
8 |
Cr 142.5 Colob 154.5 N 158.0 I |
1b
|
— |
— |
6 |
0 |
1 |
6 |
Cr 148.5 (Colr 125.5 N 145.5) I |
1c
|
C O |
— |
6 |
1 |
0 |
6 |
Cr 149.0 (Col 141.5) I |
2a
|
— |
— |
11 |
1 |
1 |
8 |
Cr 176 N 224 I |
2b
|
— |
— |
6 |
0 |
1 |
6 |
Cr 188 (SmC 171) N 206 I |
2c
|
C O |
— |
6 |
1 |
0 |
6 |
Cr 193 I |
3a
|
— |
Br |
11 |
1 |
1 |
8 |
Cr 113 SmX 119 SmC 144 SmA 156 N 174 I |
3b
|
— |
Br |
6 |
0 |
1 |
6 |
Cr 57 N 79 I |
3c
|
— |
OH |
6 |
1 |
0 |
6 |
Cr 146 I11 |
4a
|
— |
H |
10 |
1 |
1 |
8 |
Cr 125 SmX1 165 SmX2 185.9 N 186 I12 |
4b
|
— |
H |
6 |
0 |
1 |
6 |
Cr 64.5 N 90 I13 |
4c
|
— |
H |
6 |
1 |
0 |
6 |
Cr 124 I14 |
The 4-substituted benzoic acids 2a and 2b were prepared by etherification reaction of the ω-bromoalkyl substituted calamitic molecules 3a and 3b with benzyl 4-hydroxybenzoic acid and following deprotection by means of ammonium formate and palladium on carbon. Compound 2c was synthesized by esterification of 4-(6-hydroxyhexyloxy)-4′-(hexyloxy)biphenyl 3c11 with mono benzyl terephthalate (via the acid chloride) and following deprotection. The phenolic four-ring compound 5 prepared from 3-benzyloxyphenol as described by Murthy et al.15 was reacted with the corresponding benzoic acids 2a–c, which have been converted before in the acid chlorides by means of oxalyl chloride. The experimental procedure for the preparation of compound 1b starting with 3bvia2b, see ESI†.
Experimental details for the physical measurements
The transition temperatures were determined by differential scanning calorimetry (DSC Pyris 1, Perkin Elmer) and polarizing microscopy (Leitz Orthoplan). X-Ray diffraction measurements on non-oriented samples were done with a Guinier film camera. X-Ray investigations on oriented samples were performed with a 2D detector (HI-Star, Siemens AG). Oriented samples were obtained by annealing of a drop of the liquid crystal on a glass plate after slow cooling of the isotropic liquid. The sample was irradiated parallel to the glass plate. Dielectric measurements were carried out using the HP 4192A and the Solartron Schlumberger S/1260 impedance analyzer. The sample was put in a gold-coated brass capacitor with a plate distance of 0.1 mm which was calibrated with cyclohexane. Electro-optical investigations were done in commercially available cells (E. H. C. Corp.) which were heated on the hot stage of the polarizing microscope. The voltage signals were generated by a power supply (Keithley 3910).
Compound 1a: mesophase behavior, X-ray diffraction measurements, electro-optical and dielectric studies
Compound 1a forms two mesophases. The high-temperature phase could be easily identified as a nematic phase by its characteristic texture. Depending on the boundary conditions the nematic phase displays an uniform planar texture, a schlieren or a marbled texture. The nematic phase of compound 1a exists in a narrow temperature range, only. By the application of a relatively low ac field (1.1 V µm−1; 25 Hz) fluctuating domains appear which are obviously of Williams–Kapustin type, since the period of the domains corresponds to the cell thickness (6 µm), see Fig. 2.
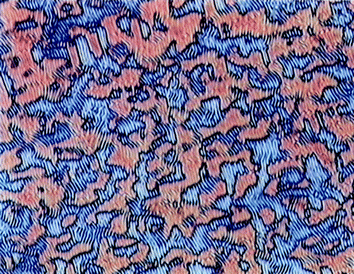 |
| Fig. 2 Domain pattern in the nematic phase of compound 1a by application of an ac field of 1.1 V µm−1
(25 Hz); Sample thickness: 6 µm; period of the domains: 6 µm; temperature: 155 °C. | |
At higher fields and higher frequencies (10 V µm−1; 400 Hz) a domain pattern arises where the domains are parallel to the director of the planar oriented nematic phase (Fig. 3). The period of the domains (20 µm) was found to be clearly higher than the cell thickness. The low-temperature phase exhibits a spherulitic texture which points to a columnar mesophase (Fig. 4). This mesophase also does not show any electro-optical response at relatively high electric fields (40 V µm−1).
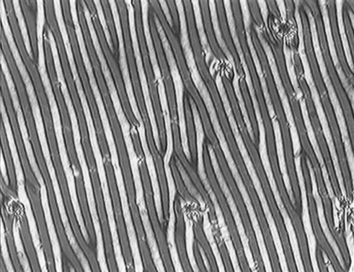 |
| Fig. 3 Field-induced domains parallel to the director in the nematic phase of compound 1a
(applied voltage: 70 V (400 Hz); sample thickness: 6 µm; period of the domains: 20 µm; temperature: 155 °C). | |
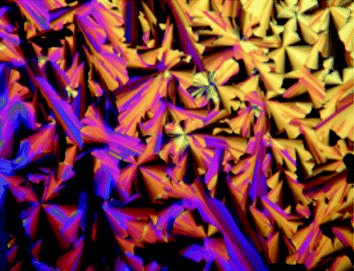 |
| Fig. 4 Texture of the columnar phase of compound 1a. | |
The assignment of the low-temperature phase was succeeded by X-ray diffraction measurements on an oriented sample. The X-ray diffraction pattern of the surface-aligned sample (Table 2) points to a columnar mesophase with a primitive oblique cell (a
= 56.7 Å, b
= 42.3 Å, γ
= 91°). In the wide angle region a diffuse scattering could be observed, which gives evidence that the lateral distances between the molecules are at random. The position of the maximum of the scattering indicates a tilt of the mesogenic units of about 40° with respect to the a-axis of the cell.
Table 2 Small angle part of the X-ray scattering, miller indexes, Bragg angles and layer spacings (compound 1a at 153 °c); the lines indicate the assumed reciprocal lattice. The additional observable reflections belong to different monodomains
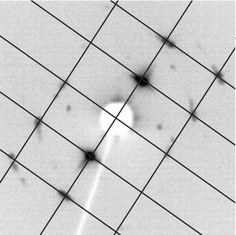
|
Index [hk] |
2θ/° |
d/Å |
10 |
1.56 |
56.7 |
01 |
2.08 |
42.3 |
11 |
2.63 |
33.6 |
20 |
3.13 |
28.2 |
21 |
3.77 |
23.4 |
Dielectric measurements were performed in the planar oriented nematic phase of compound 1a. Experimental details of the measurements and the fit procedure are given elsewhere.16Fig. 5 shows the dielectric absorption measured parallel (p) and perpendicular (s) to the nematic director. A small dielectric relaxation range at 300 kHz is only seen if the nematic director is parallel to the electrical measuring field. Therefore this process must be related to the reorientation of a longitudinal dipole moment. In the “banana” part of sample 1a the longitudinal dipoles compensate each other; therefore the molecular reason of this effect must be the partial reorientation of the small dipole moment in the calamitic unit. Like in “classical” calamitic molecules the relaxation frequency for the reorientation about the short molecular axes decreases at the I–N transition by a factor of 4. Such independent dynamics of de-coupled units has also been observed and interpreted in nematic liquid crystals consisting of two mesogenic units connected via a flexible spacer.17
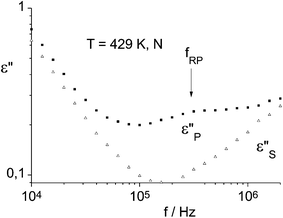 |
| Fig. 5 Dielectric loss in the nematic phase of compound 1a measured parallel (p) and perpendicular (s) to the nematic director. The reorientation about the long molecular axes seen in the increase of ε//S at f > 200 kHz is superimposed by a standing wave. | |
The limits of the dielectric constants are presented in Fig. 6. Thereby the designation ε0 was used only for the low frequency limit in the columnar phase because here a more intense relaxation range could be separated from the conductivity. The related relaxation frequency is 4 kHz. It depends only slightly on temperature. It can not be decided whether this relaxation is caused only by a collective motion or by superposition of the collective process with the reorientation about the long molecular axis. The large step in the relaxation frequency for the reorientation about the long axes from more than 5 MHz in the nematic phase to at least 50 kHz in the columnar phase (estimated from separation of both processes) indicates a closer packing of the “banana” part compared to “normal” columnar phases.18 In the nematic phase a dielectric anisotropy of Δε
=
−0.22 was found. The negative value of the dielectric anisotropy just after the phase transition and no strong decrease of ε1S at the I–N transition proves that at least the reorientation about the long axes of the “banana” part takes place in the nematic phase.
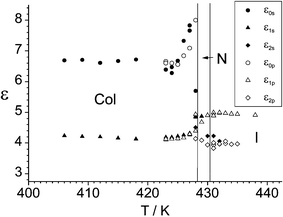 |
| Fig. 6 Limits of the dielectric constants of sample 1a measured at different temperatures. | |
Compound 1b: field-induced formation of a smectic-like texture in the nematic phase
Compound 1b, which possesses a shorter spacer, exhibits the same phases as compound 1a. However, in contrast to compound 1a the mesophases are monotropic and the clearing temperature is lower by 12.5 K. Unfortunately, the low-temperature phase crystallizes very quickly so that structure investigations were impossible. But the characteristic mosaic texture is a clear indication for a columnar phase like in compound 1a. For compound 1b the nematic phase could be investigated in a wider temperature range. We found also in this case an unusual electro-optical response. The application of a relatively high dc field (≈ 25 V µm−1) leads to the formation of domains parallel to the director. With increasing field a texture is obtained which is reminiscent of a focal-conic texture of smectic or cholesteric phases (see Fig. 7) which disappears if the field is removed. Similar behavior was only reported for the nematic phase of a few bent-core compounds,19,20 but the origin for this behavior is not yet known.
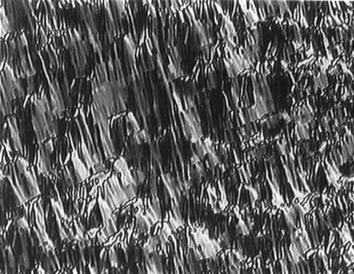 |
| Fig. 7 Field-induced texture of the nematic phase of compound 1b
(Applied voltage: 150 V; sample thickness: 6 µm; temperature: 130 °C). | |
Compound 1c which contains an additional carbonyl group in the spacer (in comparison to compound 1b) forms a columnar mesophase, only. The columnar structure is indicated by the mosaic texture and proved by X-ray diffraction measurements. Sufficiently oriented samples of compound 1c show the typical X-ray scattering pattern of a centered, rectangular cell (a
= 58.4 Å, b
= 176.0 Å), for X-ray data see Table 3. The diffuse scattering in the wide angle region points to a tilted arrangement of mesogenic units in the cell. These are inclined with respect to the a-axis taking a tilt angle of about 28°.
Table 3 Small angle part of the X-ray pattern, Miller indexes, Bragg angles and layer spacings of compound 1c at 140 °C; as a guide for the eye the reciprocal lattice is sketched by the lines
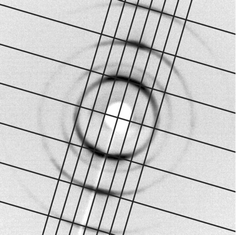
|
Index [hk] |
2θ/° |
d/Å |
02 |
1.00 |
88.0 |
11 |
1.59 |
55.4 |
13 |
2.14 |
41.3 |
20 |
3.02 |
29.2 |
31 |
4.52 |
19.4 |
Competition of calamitic and bent-core moieties in the liquid-crystalline dimers 1a–c
The connection of a bent-core mesogen with a calamitic moiety by means of a flexible spacer results in twin molecules with unusual mesophase behavior, which is clearly different from the liquid crystalline properties of the “monomeric” mesogens. The symmetric five-ring bent-core compound, 1,3-phenylene bis[4-(4-n-dodecyloxybenzoyloxy)benzoate], substituted at both terminal positions by dodecyloxy groups, exhibits a SmCP phase up to 117 °C.21 Compared with this bent monomeric unit the mesophase stability of all dimers 1a–1c is clearly increased, caused by the linking to a calamitic unit.
It is interesting to compare the N–I transition temperatures of corresponding calamitic monomers 4a–b
(see Table 1) and those of the dimers 1a–b. It is seen that the clearing temperature of the dimer 1b is 55.5 K higher than that of the calamitic monomer 4b. For the calamitic three-ring mesogen 4a the situation is reversed; the clearing temperature of the dimer 1a is 28 K lower than that of the corresponding calamitic monomer 4a. The reason for this behavior is not so clear. The connection of the calamitic three-ring unit 4a with the bent-core unit may lead to an overall structure which is more disordered than that of the monomeric calamitic mesogen because of the relatively long and flexible spacer. In the case of compound 4b the clearing temperature is relatively low in comparison to compound 4a. This is plausible since the anisotropic repulsion forces which are mainly responsible for the long-range orientational order are considerably smaller for the shorter compound 4b. In the case of compound 1b the bent-core part obviously stabilizes the orientational order of the nematic phase, particularly, since the spacer of the dimer 1b is clearly shorter than for the dimer 1a.
A basic question is whether by the combination of a bent-core unit and a calamitic unit also typical phases of these structure classes occur. First of all, all dimers under discussion do not form a polar SmCPA phase as found for the “monomeric” banana-shaped compound.21 Instead columnar phases were proved, which are often observed for bent-core mesogens (designated as B1 phase), but never for twin molecules consisting of two calamitic moieties. Comparable dimers in which two bent-core mesogenic units are connected by a hydrocarbon spacer were not reported up to now.22 Since the molecular conformation of the new dimers 1a–c is unknown and a lot of different shapes can be assumed, the construction of a structural model is very difficult and has to be a speculative one. Based on the special features of the molecular structure we made the following assumptions: (a) the bent-shaped and rod-like moieties of the molecule are sufficiently de-coupled by the spacer, (b) the moieties of different shape and flexibility (bent-shaped core, rod-like core and aliphatic core) tend to separate each other, (c) the molecules accept an irregular up and down orientation and (d) the aliphatic parts are able to interdigitate. Using these assumptions a structural model has been derived (Fig. 8). The lattice parameter a corresponds to the length of the dimer obtained from CPK models considering the observed tilt angle of 40° of the mesogenic units. This model is in agreement with the results of dielectric measurements which show that the dynamic of the bent and the calamitic units are nearly independent from each other.
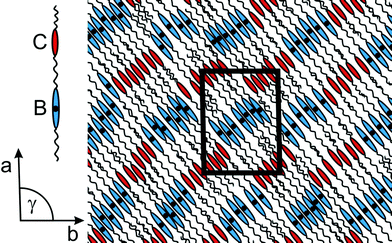 |
| Fig. 8 Model of the columnar phase of compound 1a
(C: calamitic core; B: bent-shaped core; the dots inside the bent-shaped cores represent the polar axes of the bent parts being perpendicular to the paper plane. It is assumed that both directions—up and down—exist.). | |
Conclusions
The linking of a bent-core mesogen with a calamitic moiety by means of a flexible spacer results in dimers which have unusual liquid crystalline properties. The mesophase behavior strongly depends on the different molecular fragments, e.g., size and structure of the calamitic part as well as length and polarity of the spacer. For all three compounds a columnar phase was proved, a model of this phase could be proposed. There are hints for a microsegregation of the calamitic from the bent-core units within the columnar phase. It is remarkable that two of the compounds additionally exhibit a nematic phase, which is preferably found for rod-like molecules. However, this nematic phase possesses unusual electro-optical effects, which are clearly different from those known for calamitic compounds, e.g., the field induced formation of focal-conic textures which was only reported for the nematic phase of some bent-core mesogens.
Acknowledgements
This work has been supported by the Deutsche Forschungsgemeinschaft, especially the Graduiertenkolleg “Self-organisation by coordinative and non-covalent interactions”.
References
- T. Niori, F. Sekine, J. Watanabe, T. Furukawa and H. Takezoe, J. Mater. Chem., 1996, 6, 1231 RSC.
- D. R. Link, G. Natale, R. Shao, J. E. Maclennan, N. A. Clark, E. Körblova and D. M. Walba, Science, 1997, 278, 1924 CrossRef CAS.
- G. Pelzl, S. Diele and W. Weissflog, Adv. Mater., 1999, 11, 707 CrossRef CAS.
-
C. T. Imrie and G. R. Luckhurst, Liquid Crystal Dimers in Handbook of Liquid Crystals, Wiley-VCH, Weinheim, 1998, vol. 2B, ch. X Search PubMed and references therein.
- C. T. Imrie and P. A. Henderson, Curr. Opin. Colloid Interface Sci., 2002, 7, 298 Search PubMed.
- G. S. Attard, R. W. Date, C. T. Imrie, G. R. Luckhurst, S. J. Roskilly, J. M. Seddon and L. Taylor, Liq. Cryst., 1994, 16, 529 CrossRef CAS.
- F. Hardouin, M. Achard, M. Laguerre and D.-H. Ko, Liq. Cryst., 1999, 26, 589 CrossRef CAS.
- M. W. Schröder, S. Diele, N. Pancenko, W. Weissflog and G. Pelzl, J. Mater. Chem., 2002, 12, 1331 RSC.
- M. W. Schröder, S. Diele, G. Pelzl, N. Pancenko and W. Weissflog, Liq. Cryst., 2002, 29, 1039 CrossRef CAS.
- C. V. Yelamaggad, S. Krishna Prasad, G. G. Nair, I. S. Shashikala, S. Shankar Rao, C. V. Lobo and S. Chandrasekhar, Angew. Chem., Int. Ed., 2004, 43, 3419.
- M. Itoh and R. W. Lenz, J. Polym. Sci., Part A: Polym. Chem., 1991, 29, 1407 CrossRef CAS.
-
T. Shoji, S. Takehara, H. Ogawa, M. Osawa and T. Fujisawa, 13th Japanese Symposium on Liquid Crystals, 1987 Search PubMed, Fukuoka 1Z13.
- G. Pelzl, B. Oertel and D. Demus, Z. Chem., 1986, 26, 67 CAS.
- A. Laschewsky, W. Paulus, H. Ringsdorf, A. Schuster, G. Frick and A. Mathy, Thin Solid Films, 1992, 191, 210.
- H. N. S. Murthy and B. K. Sadashiva, J. Mater. Chem., 2004, 14, 2813 RSC.
- H. Kresse, H. Schlacken, U. Dunemann, M. W. Schröder and G. Pelzl, Liq. Cryst., 2002, 29, 1509 CrossRef CAS.
- D. A. Dunmur, G. R. Luckhurst, M. R. De La Fuente, S. Diez and M. A. Perez Jubindo, J. Chem. Phys., 2001, 115, 8681 CrossRef CAS.
- H. Schmalfuss, D. Shen, C. Tschierske and H. Kresse, Liq. Cryst., 2000, 27, 1235 CrossRef.
- G. Pelzl, A. Eremin, S. Diele, H. Kresse and W. Weissflog, J. Mater. Chem., 2002, 12, 2591 RSC.
- W. Weissflog, S. Sokolowski, H. Dehne, B. Das, S. Grande, M. W. Schröder, A. Eremin, S. Diele, G. Pelzl and H. Kresse, Liq. Cryst., 2004, 31, 923 CrossRef CAS.
- M. W. Schröder, S. Diele, G. Pelzl and W. Weissflog, ChemPhysChem, 2004, 4, 99 CrossRef.
- G. Dantlgraber, S. Diele and C. Tschierske, Chem. Commun., 2002, 2768 RSC.
Footnote |
† Electronic supplementary information (ESI) available: Synthetic procedures. See DOI: 10.1039/b511140d |
|
This journal is © The Royal Society of Chemistry 2006 |