Photodynamic inactivation of Escherichia coli by novel meso-substituted porphyrins by 4-(3-N,N,N-trimethylammoniumpropoxy)phenyl and 4-(trifluoromethyl)phenyl groups
Received
23rd September 2005
, Accepted 19th October 2005
First published on 22nd November 2005
Abstract
The photodynamic effect of novel cationic porphyrins, with different pattern of meso-substitution by 4-(3-N,N,N-trimethylammoniumpropoxy)phenyl (A) and 4-(trifluoromethyl)phenyl (B) groups, have been studied in both solution bearing photooxidizable substrates and in vitro on a typical Gram-negative bacterium Escherichia coli. In these sensitizers, the cationic groups are separated from the macrocycle ring by a propoxy spacer. Thus, the charges have a high mobility and a minimal influence on photophysical properties of the porphyrin. These compounds produce singlet molecular oxygen, O2(1Δg), with quantum yields of ∼0.41–0.53 in N,N-dimethylformamide. In methanol, the L-tryptophan photodecomposition increases with the number of cationic charges in the sensitizer. In vitro investigations show that cationic porphyrins are rapidly bound to E. coli cells in ∼5 min. A higher binding was found for A3B3+ porphyrin, which is tightly bound to cells still after three washing steps. Photosensitized inactivation of E. coli cellular suspensions follows the order: A3B3+ > A44+
≫ ABAB2+ > AB3+. Under these conditions, a negligible effect was found for 5,10,15,20-tetra(4-sulfonatophenyl)porphyrin (TPPS44−) that characterizes an anionic sensitizer. Also, the results obtained for these new cationic porphyrins were compared with those of 5,10,15,20-tetra(4-N,N,N-trimethylammonium phenyl)porphyrin (TTAP4+), which is a standard active sensitizer established to eradicate E. coli. The photodynamic activity of TTAP4+ is quite similar to that produced by A44+. Studies in an anoxic condition indicate that oxygen is necessary for the mechanism of action of photodynamic inactivation of bacteria. The higher photodynamic activity of A3B3+ was confirmed by growth delay experiments. Photodynamic inactivation capacities of these sensitizers were also evaluated in E. coli cells immobilized on agar surfaces. Under these conditions, A3B3+ porphyrin retains a high activity to inactivate localized bacterial cells. Therefore, tricationic porphyrin A3B3+ is an interesting sensitizer with potential applications in photodynamic inactivation of bacteria in liquid suspensions or on surfaces.
Introduction
The emergence of antibiotic resistance amongst pathogenic bacteria has led to a major research effort to find alternative antibacterial therapies.1 Bacterial photodynamic inactivation (PDI) is based on the administration of a photosensitizer, which is preferentially accumulated in the microbial cells. The subsequent irradiation with visible light, in the presence of oxygen, specifically produces cell damages that inactivate the microorganisms.2,3 Two oxidative mechanisms can occur after photoactivation of the photosensitizer. In the type I photochemical reaction, the photosensitizer interacts with a biomolecule to produce free radicals, while in the type II mechanism, singlet molecular oxygen, O2(1Δg), is produced as the main species responsible for cell inactivation.4,5 Both mechanisms can occur simultaneously and the ratio between the two processes depends mainly of the sensitizer, substrate and the nature of the medium.
Previous investigation showed that porphyrin derivatives can photosensitize the inactivation of various microbial pathogens.6–11 In general, these studies show that Gram-positive bacteria are efficiently photoinactivated by a variety of sensitizers, whereas Gram-negative bacteria are usually resistant to the action of negatively charged or neutral agents.3 The resistance of Gram-negative bacteria to the action of photoactivated sensitizers has been ascribed to the presence of a highly organized outer membrane, which hinders the interaction of the photosensitizer with the cytoplasmic membrane and intercepts the photogenerated reactive species.7,9,12 Cationic porphyrins have shown to photoinduce direct inactivation of Gram-negative bacteria without the presence of an additional permeabilization agent.9 The positive charges on the photosensitizer molecule appear to promote a tight electrostatic interaction with negatively charged sites at the outer surface of the Gram-negative bacteria, increasing the efficiency of the photoinactivation processes.3
In previous studies, we have evaluated cationic porphyrins as agents to eradicate Gram-negative bacteria.11 Thus, 5-(4-trifluorophenyl)-10,15,20-tris(4-trimethylammoniumphenyl) porphyrin iodide and its metal complex with Pd(II) showed to be active photosensitizers to inactivate Escherichia coli cells.11 Also, a cationic Zn(II) tetramethyltetrapyridino porphyrazinium salt was used as sensitizer to produce photohemolysis of human red blood cells and inactivation of Gram-negative bacteria.13
In this paper, the photodynamic activity of a novel series of cationic porphyrin derivatives (Scheme 1) was compared in solution bearing biologically related substrates and in a typical Gram-negative bacterium E. coli. In these porphyrins, the cationic centers are isolated from the porphyrin ring by a propoxy bridge.14 Thus, the charges have minimal influence on the electronic density of the tetrapyrrolic macrocycle. This addresses the consistency of the photophysical properties of the sensitizer. Also, this chain provides a higher mobility of the charge, which could facilitate the interaction with the outer membrane of the Gram-negative bacteria. Also, the cationic charges in these porphyrins are combined in different patterns with trifluoromethyl groups. These highly lipophilic groups increase the amphiphilic character of the structure.14–16 The behavior of these new cationic porphyrins on E. coli were compared with those obtained for (5,10,15,20-tetra(4-N,N,N-trimethylammoniumphenyl)porphyrin (TTAP4+), which is a standard active sensitizer established to eradicate E. coli6,7 and (5,10,15,20-tetra(4-sulfonatophenyl) porphyrin (TPPS44−) (Scheme 1), which was used as an anionic model. Also in this study, the photoinactivation efficiencies of these sensitizers were evaluated in E. coli cells immobilized on a surface, which allows establishing conditions for the treatment of pathogenic microorganisms growing as localized foci of infection.
Materials and methods
General
Absorption and fluorescence spectra were recorded on a Shimadzu UV-2401PC spectrometer and on a Spex FluoroMax fluorometer, respectively. All the chemicals from Aldrich (Milwaukee, WI, USA) were used without further purification. Sodium dodecyl sulfate (SDS) from Merck (Darmstadt, Germany) was used as received. Solvents (GR grade) from Merck were distilled. Ultrapure water was obtained from Labconco (Kansas, MO, USA) equipment model 90901-01.
Porphyrins
5-[4-(3-N,N,N-Trimethylammoniumpropoxy)phenyl]-10,15,20-tris(4-trifluoromethyl phenyl) porphyrin (AB3+), 5,15-di[4-(3-N,N,N-trimethylammoniumpropoxy)phenyl]-10,20-di(4-trifluoromethylphenyl) porphyrin iodide (ABAB2+), 5,10,15-tris[4-(3-N,N,N-trimethylammoniumpropoxy)phenyl]-20-(4-trifluoromethylphenyl) porphyrin iodide (A3B3+) and 5,10,15,20-tetrakis[4-(3-N,N,N-trimethylammoniumpropoxy)phenyl] porphyrin iodide (A44+) were synthesized as previously described.14 Tetraphenylporphyrin (TPP), (5,10,15,20-tetra(4-N,N,N-trimethylammonium phenyl)porphyrin p-tosylate (TTAP4+) and (5,10,15,20-tetra(4-sulfonatophenyl)porphyrin (TPPS44−) sodium salt were purchased from Aldrich.
Steady state photolysis
Spectra were recorded using 1 cm path length quartz cuvettes. Solutions of 9,10-dimethylanthracene (DMA, 35 µM) in 2 mL of N,N-dimethylformamide (DMF) and photosensitizer (λ = 420 nm, absorbance 0.3) were irradiated with monochromatic light at λ = 420 nm from a 75 W high-pressure Xe lamp through a high intensity grating monochromator, Photon Technology Instrument (P & P). The light intensity was determined as 0.45 mW cm−2 (Radiometer Laser Mate-Q, Coherent). Photooxidation of DMA was used to determine singlet molecular oxygen, O2(1Δg), production by the photosensitizers,17,18 using TPP as the standard (ΦΔ = 0.62).19 Measurements of the sample and reference under the same conditions afforded ΦΔ for the porphyrins by direct comparison of the slopes in the linear region of the plots. On the other hand, solutions of L-tryptophan (Trp, 20 µM) and photosensitizer (λ = 420 nm, absorbance 1.5) in 2 mL of methanol were irradiated with visible light in the same conditions described below for the treatment of E. coli cultures. The kinetics of photooxidation was studied by following the decrease of the absorbance (A) at λmax = 378 nm for DMA and the fluorescence intensity (F) at λ = 340 nm for Trp. The Trp fluorescence was excited by 290 nm light. A control experiment showed that under these experimental conditions the fluorescence intensity is linearly correlated with Trp concentration. The observed rate constants (kobs) were obtained by a linear least-squares fit of the semilogarithmic plot of lnA0/A or lnF0/Fvs. irradiation time. All the experiment were performed at 25.0 ± 0.5 °C. The pooled standard deviation of the kinetic data, using different prepared samples, was less than 5%.
Bacterial strain and preparation of cultures
E. coli strain (EC7) recovered from clinical urogenital material was used. The bacterial strain was identified according to conventional procedures.20 The antibiotic resistance profiles are: ceftazidime (sensitive), levofloxacin (sensitive), ampicillin (resistant), aminosulbactam (resistant), cephalotin (limit) gentamycin (sensitive) and sulfamethoxazol-trimethoprim (resistant). E. coli strain was grown aerobically at 37 °C in 30% w/v tryptic soy (TS) broth overnight. Aliquots (∼40 µL) of this culture were aseptically transferred to 4 mL of fresh medium (30% w/v TS broth) and incubated at 37 °C to mid-logarithmic phase (absorbance ∼0.6 at 660 nm). Cells in the logarithmic phase of growth were harvested by centrifugation of broth cultures (3000 g for 15 min) and re-suspended in 4 mL of 10 mM phosphate-buffered saline (PBS, pH = 7.0). Then the cells were diluted 1/1000 in PBS, corresponding to ∼106 colony forming units (CFU) mL−1. In all the experiments, 2 mL of the cell suspensions in Pirex brand culture tubes (13 × 100 mm) were used and the sensitizer was added from a stock solution of porphyrin (4.5 × 10−4 M) in DMF. Viable bacteria were monitored and their number calculated by counting the number of colony forming units after appropriated dilution on TS agar plates.11,13 Bacterial cultures grown under the same conditions with and without photosensitizers kept in the dark as well as illuminated cultures without porphyrin served as controls.
Porphyrin binding to bacterial cells
Suspensions of E. coli (2 mL, ∼106 CFU mL−1) in PBS were incubated in dark at 37 °C with 1 µM of sensitizer for different times. The cultures were centrifuged (3000 g for 15 min) and then re-suspended with PBS. The cell pellets, after different washing steps (0 and 3), obtained by centrifugation (3000 g for 15 min) were re-suspended in 2% aqueous SDS (2 mL), incubated overnight at 4 °C and sonicated for 30 min.6 The concentration of sensitizer in the supernatant was measured by spectrofluorimetry (λexc = 420 nm, λem = 655 nm) in solution of 2% SDS in PBS. The fluorescence values obtained from each sample were referred to the total number of bacteria contained in the suspension. The concentration of the porphyrin in this sample was estimated by comparison with a calibration curve obtained with standard solutions of the sensitizer in 2% SDS ([sensitizer] ∼ 0.05–1.0 µM). The A3B3+ porphyrin binding to cells was evaluated as described above, incubating the cultures for 5 min with different sensitizer concentrations (1–10 µM).
Photosensitized inactivation of bacteria cells in PBS suspension
Cell suspensions of E. coli (2 mL, ∼106 CFU mL−1) in PBS were incubated with the appropriate concentration of porphyrin for 30 min in the dark at 37 °C. After that, two protocols were followed: (a) irradiation of cultures without cell washing step, which means bearing the supernatant with the porphyrin and (b) washing the cells three times with PBS prior to irradiation. In both cases, the cultures were exposed for different time intervals to visible light. The light source used was a Novamat 130 AF slide projector equipped with a 150 W lamp. The light was filtered through a 2.5 cm glass cuvette filled with water to absorb heat. A wavelength range between 350–800 nm was selected by optical filters. The light intensity at the treatment site was 90 mW cm−2 (Radiometer Laser Mate-Q, Coherent). Control experiments were carried out without illumination in the absence and in the presence of sensitizer, and with addition of DMF excluding the porphyrins. The solvent DMF of the photosensitizer was not toxic for bacteria. Control and irradiated cell suspensions were serially diluted with PBS, each solution was plated in triplicate on TS agar and the number of colonies formed after 18–24 h incubation at 37 °C was counted. Each experiment was repeated separately three times.
Studies in the absence of oxygen were performed as described above but displacing the oxygen with argon in the cultures flasks for 10 min before irradiation and maintaining an argon atmosphere during the illumination.
Photosensitized growth delay experiment
E. coli cells were grown overnight as described above. A portion (60 µL) of this culture was transfer to 20 mL of fresh 9% w/v TS broth medium. The suspension was homogenized and aliquots of 2 mL were incubated at 37 °C. The culture grown was measured by turbidity at 660 nm using a Tuner SP-830 spectrophotometer.11 When the cultures reached the log phase (absorbance ∼0.09), an appropriated amount of sensitizer was added. Then the flasks were irradiated with visible light, as described above.
Porphyrin uptake in solution and photosensitized inactivation of bacteria cells on agar
Suspensions of E. coli (2 mL, ∼106 CFU mL−1) in PBS were incubated for 30 min in dark at 37 °C with 1 µM of sensitizer. The cells were diluted 1/100 in PBS and 50 µL of the cultures were spread on TS agar plates of 5 cm diameter.21 The cultures were incubated for 15 min and then the plates were irradiated for 10 min with visible light as described above. The number of colonies formed after 18–24 h incubation at 37 °C was counted. Also, E. coli cellular viability in this medium was determined for cultures treated with 1 µM of porphyrin A3B3+ at different irradiation times. Controls with and without photosensitizers kept in the dark as well as illuminated controls without porphyrin were curried out. Each experiment was repeated separately three times.
Porphyrin uptake and photosensitized inactivation of bacteria cells on agar
TS agar plates (5 cm diameter) were spread with 10 nmol of porphyrin on an area of 0.6 cm2 from a 4.5 × 10−4 M solution in DMF. The solvent was evaporated and the plates were spread with the suspension of E. coli (∼106 CFU mL−1) in PBS. Then the plates were incubated at 37 °C for 20 minutes in dark. After that, the plates were irradiated as described above for 10 min and incubated for 18–24 h at 37 °C in dark. Plates with and without photosensitizers kept in the dark and plates without porphyrin and irradiated were used as controls. Each experiment was repeated separately three times.
Results and discussion
Spectroscopic studies
The absorption spectra of cationic porphyrins in DMF show the typical Soret (∼420 nm) and four Q-bands (∼515, 550, 592, 650 nm), characteristic of free-base porphyrins.14,22 The spectra of these porphyrins were also analyzed in different media. Representative results for A3B3+ porphyrin are shown in Fig. 1A. Similar behaviors were observed for the other cationic synthesized porphyrins. In all cases, a small hypsochromic shift on the location of the Soret absorption bands was observed upon solubilization of porphyrins in methanol respect to DMF. In PBS, the spectra of these porphyrins show a broadening of Soret band, indicating that aggregation occurs, as is typical for many porphyrin derivatives.23–25 In particular, for porphyrins AB3+ and ABAB2+ this broadening is accompanied by the formation of a new band at about 442 nm.26
The steady-state fluorescence emission spectra of these cationic porphyrins in DMF show two bands (∼655 and 720 nm) in the red spectral region, which are characteristic for similar free-base porphyrins. These bands have been assigned to Q(0–0) and Q(0–1) transitions.14,27 The spectra were compared in different solvents. Fig. 1B shows characteristic results for A3B3+ porphyrin. As can be observed, the fluorescence intensity considerable diminished in water and PBS with respect to organic solvents. The fluorescence quantum yields (ϕF) were calculated in DMF, giving the values 0.10, 0.12, 0.13 and 0.12 for AB3+, ABAB2+, A3B3+ and A44+, respectively.14 The fluorescence spectra of cationic porphyrins were also analyzed in biological medium. The E. coli cells were treated with 1 µM of sensitizer for 30 min at 37 °C in dark. After one washing step, a cellular suspension in PBS showed a shape close to that of the fluorescence emission in homogeneous media with maxima at ∼657 and 723 nm (Fig. 1B). This result indicates that the binding of porphyrins to E. coli cells leads to photosensitizer disaggregation with respect to PBS, possibly favored by the cellular microenvironment where the sensitizer is localized.28
Photosensitized decomposition of substrates
Photooxidation of 9,10-dimethylanthracene.
The photodecomposition of DMA sensitized by porphyrins was used to evaluated the O2(1Δg) production.29,18Fig. 2A shows the semilogarithmic plots describing the progress of the reaction for DMA. From these plots the values of the observed rate constant (kobsDMA) were obtained, irradiating the system at Soret band (Table 1). The quantum yields of O2(1Δg) production (ΦΔ) were calculated comparing the slope for the porphyrin compounds with the corresponding slope obtained for the reference, TPP, in DMF.28 As can be observed in Table 1, a similar efficiency in the O2(1Δg) production was found for these porphyrins in DMF. However, the values of ΦΔ can significantly change in a different medium, diminishing when the sensitizer is partially aggregated.4,5 Also, the biological microenvironment of the sensitizer can induce important modifications in the photophysics of the porphyrin established in solution.30 In consequence, there are limitations to predict photodynamic efficiencies of sensitizers in biological systems on the basic of photophysical investigations in homogeneous solution.
Table 1 Kinetic parameters for the photooxidation of DMA and Trp sensitized by porphyrins and singlet oxygen quantum yield (ΦΔ)
Porphyrin |
k
obs
DMA/s−1a |
Φ
Δ
a
,
b
|
k
obs
Trp/s−1c |
DMF.
Using TPP as reference.28
Methanol.
|
AB3+ |
(4.3 ± 0.1) × 10−4 |
0.41 ± 0.05 |
(0.8 ± 0.1) × 10−4 |
ABAB2+ |
(5.5 ± 0.1) × 10−4 |
0.53 ± 0.05 |
(1.1 ± 0.1) × 10−4 |
A3B3+ |
(5.5 ± 0.1) × 10−4 |
0.53 ± 0.05 |
(1.4 ± 0.1) × 10−4 |
A44+ |
(5.3 ± 0.1) × 10−4 |
0.51 ± 0.05 |
(1.5 ± 0.1) × 10−4 |
TTAP4+ |
(6.8 ± 0.1) × 10−4 |
0.65 ± 0.05 |
(1.2 ± 0.1) × 10−4 |
TPP |
(6.4 ± 0.1) × 10−4 |
0.62 ± 0.05 |
(0.7 ± 0.1) × 10−4 |
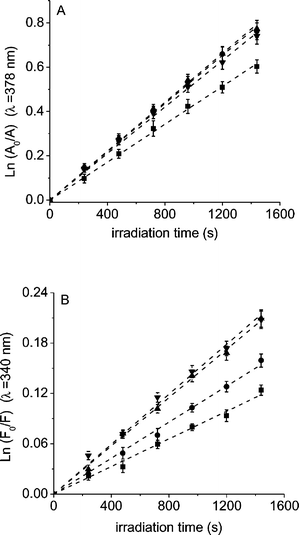 |
| Fig. 2 First-order plots for the photooxidation of: (A) DMA (35 µM) in DMF and (B) Trp (25 µM) in methanol, photosensitized by porphyrin AB3+ (■), ABAB2+ (●), A3B3+ (▲) and A4+ (▼) irradiated with light at λ = 420 nm. Values represent mean ± standard deviation of three separate experiments. | |
Decomposition of L-tryptophan.
The amino acid Trp was used as substrate models for the compounds of biological interest that would be potential targets of porphyrin photosensitization. This substrate can be efficiently photooxidized by both type I and type II reaction mechanism.29,31,32 The photo-process follows first-order kinetics with respect to Trp concentration, as showed in Fig. 2B for [Trp] = 25 µM. From the plots in Fig. 2B, the values of the kobsTrp were calculated for porphyrins. As can be observed in Table 1, a lower kobsTrp value was obtained for non-charged porphyrin, TPP, and the photooxidation rates slowly increase with the number of positive charges in the porphyrin macrocycle. In previous studies, electron transfer quenching of porphycene or phthalocyanine-excited states by Trp has been postulated.33,34 Also, the photosensitized oxidation of Trp was found to be more than two orders of magnitude more efficient via electron transfer than via O2(1Δg).35 Since cationic porphyrin can interact with Trp, probably by electrostatic attraction, an electron transfer pathway may also be contributing together with type II photoprocess to their decomposition.4,28
Binding of porphyrin to E. coli cells
The capacity of these porphyrins (see Scheme 1) to bind to bacterial cells was compared after unwashing and three washing steps. The E. coli cultures were incubated with 1 µM of porphyrin for different times at 37 °C in the dark. In each case, the sensitizer associated with cells was determined by fluorescence analysis (see Materials and methods). The amounts of porphyrin recovered after unwashing step are summarized in Table 2. Under these conditions, the cationic porphyrins reach the highest value of cell-bound sensitizer at short time (∼5 min). Prolonging the incubation time to 30 min does not cause an appreciable increase in the amount of photosensitizer bound to E. coli cells. The results show that the binding of tricationic A3B3+ porphyrin (∼0.8 nmol/106 cells) is over two times higher than those for the other cationic porphyrins. This behavior suggests that compound A3B3+ has particularly high binding affinity for bacterial cells. In these conditions, the binding of tetracationic porphyrins (A4+ and TTAP4+) to E. coli cells reaches a value of ∼0.4 nmol/106 cells. On the other hand, all cationic porphyrins are higher bound to cells than the anionic porphyrin TPPS44−.
Table 2 Recovery of porphyrins (nmol/106 cells) from E. coli cells treated with 1 µM of sensitizer at 37 °C in dark for different incubation times and after different washing steps
Incubation time/min |
Washing steps |
AB3+ |
ABAB2+ |
A3B3+ |
A4+ |
TTAP4+ |
TPPS44− |
5 |
0 |
0.28 ± 0.04 |
0.21 ± 0.05 |
0.73 ± 0.07 |
0.37 ± 0.06 |
0.37 ± 0.06 |
0.03 ± 0.01 |
5 |
3 |
0.14 ± 0.03 |
0.12 ± 0.02 |
0.51 ± 0.08 |
0.15 ± 0.03 |
0.18 ± 0.04 |
(3.2 ± 0.5) × 10−4 |
10 |
0 |
0.26 ± 0.05 |
0.30 ± 0.04 |
0.86 ± 0.06 |
0.31 ± 0.06 |
0.43 ± 0.08 |
0.03 ± 0.01 |
10 |
3 |
0.18 ± 0.04 |
0.10 ± 0.02 |
0.70 ± 0.05 |
0.17 ± 0.03 |
0.25 ± 0.05 |
(4.0 ± 0.7) × 10−4 |
30 |
0 |
0.30 ± 0.05 |
0.36 ± 0.06 |
0.84 ± 0.07 |
0.31 ± 0.06 |
0.38 ± 0.06 |
0.04 ± 0.01 |
30 |
3 |
0.16 ± 0.03 |
0.10 ± 0.02 |
0.66 ± 0.08 |
0.20 ± 0.04 |
0.22 ± 0.05 |
(3.5 ± 0.6) × 10−4 |
In addition, the binding of tricationic porphyrin A3B3+ was analyzed incubating the E. coli cells with different concentration of sensitizer for 5 min at 37 °C in the dark. As shown in Fig. 3, the cell-bound photosensitizer increases with the porphyrin concentration until ∼5 µM. At higher concentrations (5–10 µM), the binding of A3B3+ tends to a saturation value of ∼2.5 nmol/106 cells.
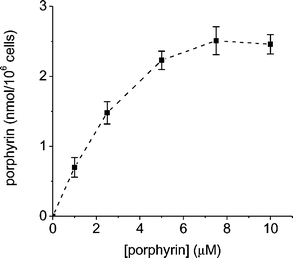 |
| Fig. 3 Amount of A3B3+ porphyrin recovered after unwashing step from E. coli cells (∼106 CFU mL−1) incubated for 5 min with different porphyrin concentrations at 37 °C in dark. Values represent mean ± standard deviation of three separate experiments. | |
Moreover, the amount of porphyrin binds to cells was determined after three washing steps under the same experimental conditions. As can be observed in Table 2, a fraction of the cell-bound porphyrin was removed by repeated washing with PBS, suggesting that some porphyrin molecules were weakly bound to the cells. Thus, both tetracationic sensitizers, A4+ and TTAP4+, retain ∼60% of the initial bound porphyrin, while this percentage decreases to about ∼50% and ∼30% for monocationic AB3+ and dicationic ABAB2+ porphyrins, respectively. However, about ∼70% of tricationic sensitizer A3B3+ is kept even after three washing steps with respect to unwashing step.
In contrast, this is not the tendency found for the anionic TPPS44− porphyrin, which considerably diminishes (∼99%) with three washing steps. This result is in concordance with that previously found for TPPS44− in E. coli cells.6 In that case, ∼7% of TPPS44− remains bound to cell after three washing steps. A similar behavior was also observed for a negatively charged tetra-sulfonated phthalocyanine after successive washing with PBS of E. coli cells.36 Only 0.22% of phthalocyanine is kept bound to cells after three washing steps. Consequently the amount of cationic porphyrins remaining at this stage can be considered to represent the fraction of tightly bound porphyrin.6,7,10,11
Photosensitized inactivation of E. coli cells in PBS suspension
Suspensions of E. coli cells in PBS were treated with 1 µM of sensitizer for 30 min at 37 °C in dark, the cultures were irradiated with visible light without washing step (Fig. 4). Control experiments showed that the viability of E. coli was unaffected by illumination alone or by dark incubation with 1 µM of the photosensitizer for 30 min, indicating that the cell mortality obtained after irradiation of the cultures treated with the porphyrin is due to the photosensitization effect of the agent produced by visible light. Thus, the viability of E. coli cells after irradiation was dependent upon both light exposure level and the porphyrin used in the treatment (Fig. 4).
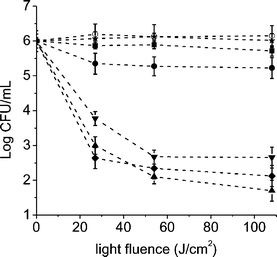 |
| Fig. 4 Survival curves of E. coli cells (∼106 CFU mL−1) incubated with 1 µM of AB3+ (■), ABAB2+ (●), A3B3+ (▲), A4+ (▼), TTAP4+ (✦) and TPPS44− (★) porphyrins for 30 min at 37 °C in dark and exposed to visible light for different irradiation times. Control culture untreated (○). Values represent mean ± standard deviation of three separate experiments. | |
As seen in Fig. 4, the E. coli cells are rapidly photoinactivated when the unwashed cultures treated with tricationic A3B3+ and tetracationic, A44+ and TTAP4+, porphyrins are exposed to visible light. The cell inactivation produced by TTAP4+ and A44+ porphyrins represents about 99.98% and 99.96%, respectively. Comparable behaviors were previously reported for E. coli cells treated with 10 µg mL−1 of tetracationic porphyrins, 5,10,15,20-tetra(4-N,N,N-trimethylammoniumphenyl)porphyrin iodide (T4MAP) and 5,10,15,20-tetra(4-N-methylpyridyl) porphyrin iodide (T4MPyP) after 30 min of irradiation with visible light (6 mW cm−2).6 Also, a high photoinactivation E. coli cells was found for cultures incubated with T4MPyP and irradiated with different doses of light.9,12 As a result, a ∼1.8 log decrease of E. coli survival was reported for cells treated with 8.3 µM of sensitizer and irradiated for 15 min with white light (150 mW cm−2).10 However, the photoinactivation efficiency considerably diminishes for cells treated with a lower concentration of T4MPyP (0.8 µM).
In particular, the tricationic A3B3+ porphyrin exhibits a photosensitizing activity causing a ∼4.5 log decrease of cell survival, when the cultures are treated with 1 µM of sensitizer and irradiated with a light fluence of 108 J cm−2 (20 min of irradiation). These results represent a value greater than 99.997% of cellular inactivation. The photodynamic activity of porphyrin A3B+3 is a little higher than those of tetracationic porphyrins a longer irradiation time used (20 min, Fig. 4). As can be observed in Fig. 4, a light fluence of 54 J cm−2 (10 min of irradiation) can also be used to produce a high photoinactivation (∼99.994%) of E. coli cells treated with A3B3+. This is a more appropriated light dose for non-oncologic applications of PDI in a clinical study.
On the other hand, photosensitization of cells by dicationic ABAB2+ porphyrin produces a low diminishing in the cellular viability (∼1 log). Also, a low cellular photoinactivation was observed for cells treated with monocationic porphyrin AB3+ (<1 log), consistent with previous results for monocationic sensitizers in Gram-negative bacteria.7 As expected due to its low binding to E. coli cells, no inactivation effect was found for cultures treated with 1 µM of anionic TPPS44− porphyrin still after 20 min of irradiation.6 This result is in agreement with that reported before for TPPS44−, indicating that these non-cationic porphyrins are unsuccessful sensitizers for Gram-negative bacteria under these conditions.6
In previous studies we have analyzed the photodynamic effect of a tricationic 5-(4-trifluorophenyl)-10,15,20-tris(4-trimethylammoniumphenyl)porphyrin iodide in the same E. coli strain.11 In this sensitizer, the cationic groups are directly attached to the phenyl groups of the porphyrin ring. Treatment of the bacterial cells with 1 µM of this porphyrin produced a ∼3.6 log decrease of cell survival, after 30 min of irradiation. Therefore, the new tricationic A3B3+ porphyrin evaluated in the present study is a more effective photosensitizer for ∼1 log under the same experimental conditions. Also, tetracationic 5,10,15,20-tetrakis-(4-N-methylpyridil)porphyrin derivatives, where one N-methyl group was replaced by a hydrocarbon chain ranging from C6 to C22, were evaluated to eradication bacteria.10 Thus, T4MPyP and its C6 analog, produced a significant antibacterial activity only at concentration of 8.3 µM, while the C10, C14 and C18 analogs caused similar effect at a 10-fold lower concentration. The C10 and C14 derivatives produced a 4 log drop in survival of E. coli cells upon 15 min irradiation (150 mW cm−2) in the presence of 0.8 µM porphyrin.10 The elevated photoefficiency of C10 and C14 sensitizers was related to an increase in the hydrophobicity of the photosensitizer molecule which enhances its affinity for bacterial cells, hence promoting a more efficient photoinactivation. Similar conclusions were also interpreted for amphiphilic cationic porphyrins as photoinactivating agents for bacteria.7 In our case, the cationic charges of the A3B3+ porphyrin are combined with a highly lipophilic trifluoromethyl group, which increases the amphiphilic character of the structure.14 Also, the cationic centers are spaced from the porphyrin ring by an aliphatic chain, which provides a higher mobility of the charges facilitating the binding to E. coli cells. This affinity is consequently accompanied by an increase in the photocytotoxic activity.
Moreover, photoinactivation of E. coli cells was evaluated after three washing steps. Under this condition, the photodynamic effect is mainly associated with porphyrins that are tighter bound to cells. The photoinactivation activity of tricationic A3B3+ porphyrin remains similar than that found for unwashed cells. This can be associated with their tight binding to E. coli cells.11 The photocytotoxic effect for cultures treated with tetracationic A44+ and TTAP4+ porphyrins diminishes ∼1 log with respect to unwashed cells, which is in agreement with a loss of the amount of cell-bound sensitizer after three washing steps.
To evaluate the influence of oxygen atmosphere, the photoinactivation of E. coli cells was analyzed under an anoxic condition. Thus, the irradiation of the cellular suspension treated with 1 µM of sensitizer was performed under argon atmosphere. In Table 3, the cellular survivals are compared under both conditions, air and argon atmosphere. As can be observed, in all cases the loss of viability was highly oxygen dependent. Practically, no cell inactivation occurs using these porphyrins as sensitizer under a low oxygen concentration. A similar behavior was previously found for E. coli cell inactivation sensitized by N-alkylpyridylporphyrins and their Zn(II) complexes.37 The anoxic condition indicates that oxygen is necessary for the mechanism of action of photodynamic inactivation of bacteria. However, the results showed in Table 3 can not be used to establish the predominant mechanism of photoreaction produced by the photosensitizers used in this study.38
Table 3 Photoinactivation of E. coli cells incubated with 1 µM of sensitizer for 30 min at 37 °C in dark and irradiated for 10 min with visible light
Porphyrin |
Air/Log CFU mL−1a |
Argon/Log CFU mL−1b |
Cell survival (%)c |
Cellular suspension in PBS in atmosphere of air.
Cellular suspension in PBS in atmosphere of argon.
Cells immobilized on TS agar.
No colonies formation was detected.
|
Control |
6.1 ± 0.5 |
6.1 ± 0.4 |
100 ± 5 |
AB3+ |
5.9 ± 0.4 |
5.9 ± 0.3 |
82 ± 5 |
ABAB2+ |
5.3 ± 0.5 |
5.9 ± 0.4 |
78 ± 4 |
A3B3+ |
2.1 ± 0.4 |
5.5 ± 0.5 |
—d |
A44+ |
2.7 ± 0.3 |
5.3 ± 0.4 |
60 ± 4 |
TTAP4+ |
2.3 ± 0.3 |
5.6 ± 0.2 |
50 ± 4 |
TPPS44− |
6.1 ± 0.4 |
6.0 ± 0.4 |
93 ± 5 |
Photosensitized growth delay of E. coli cultures
Growth delay of E. coli cultures sensitized by porphyrins was carried out in medium (9% w/v TS broth). These experiments were performed to ensure that PDI of cells is still possible when the cultures were not under starvation conditions or the potential damaging effects of phosphate buffer washing.11,36,37 Thus, when fresh cultures of E. coli reached the log phase (Fig. 5, absorbance ∼0.09), an appropriated amount of sensitizer was added and the flasks were irradiated with visible light at 37 °C. As can be observed in Fig. 5, growth was arrested when E. coli cultures were treated with cationic porphyrins and illuminated. After 30 min of irradiation in the presence of 5 µM of sensitizer A3B3+, the cells no longer appeared to be growing as measured by turbidity at 660 nm. As observed above, the effect of A3B3+ porphyrin is faster than those of tetracationic porphyrins, A44+ and TTAP4+. Also these experiments indicate that a higher activity is shown for A3B3+ porphyrin with respect to that previously found for cultures treated with 10 µM of 5-(4-trifluorophenyl)-10,15,20-tris(4-trimethylammoniumphenyl) porphyrin iodide.11
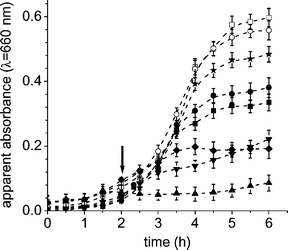 |
| Fig. 5 Photosensitized growth delay curves of E. coli cells incubated with 10 µM of AB3+ (■), 10 µM of ABAB2+ (●), 5 µM of A3B3+ (▲), 5 µM of A44+ (▼), 5 µM of TTAP4+ (✦) and 5 µM of TPPS44− (★) porphyrins and exposed to different irradiation times with visible light in 9% w/v TS broth at 37 °C. The arrow marks the time point where the appropriate concentration of porphyrin was added. Control culture untreated irradiated (○) and in dark (□). Values represent mean ± standard deviation of three separate experiments. | |
Furthermore, a minor effect in the growth delay was found for cells treated with 10 µM of AB3+ and ABAB2+ porphyrins. On the other hand, E. coli cells exposed to porphyrins in the dark or not treated with sensitizer and illuminated showed no growth delay compared with controls. Therefore, the data illustrate that the observed growth delay is due to the photoinactivation effect of the sensitizers on the cells.36 These results agree with those observed above, and also under these conditions 10 µM of TPPS44− do not produce significant bacterial inactivation.
Inactivation of E. coli cells on surfaces
The photodynamic activity of these photosensitizers was evaluated in E. coli cells immobilized on TS agar. This approach can be used to inactivate bacteria growing in vivo as localized foci of infection, on skin or on accessible mucous membrane.39,40 Also, photodynamic treatment has been proposed as a new possibility protecting foods from microbial spoilage.21
In a first approach, the cellular suspensions of E. coli in PBS were incubated with 1 µM of sensitizer for 30 min at 37 °C in dark. After that, the cells were plated and the plates incubated for 15 min at 37 °C in the dark. Thus, in this experiment the sensitizer was uptake in solution and then the cells were fixed on agar surface. The plates were then illuminated as described above (Materials and methods) for 10 min (54 J cm−2). The cell survivals after 18–24 h of incubation in dark are summarized in Table 3. As can be seen, no colony formation was observed for cells treated with porphyrin A3B3+. Also, considerable photodynamic inactivation was found for tetracationic TTAP4+ and A44+ porphyrins. As previously observed in PBS suspension, ABAB2+ and AB3+ porphyrins produce a low cellular photoinactivation of ∼10%, whereas in this condition a very little effect is produced for TPPS44−. Moreover, the photodynamic activity of A3B3+ porphyrin was evaluated under these conditions at different irradiation times. The results are shown in Fig. 6. As can be observed, the cell viability rapidly diminishes and the colony formation was not detected after 10 min of visible light irradiation. Thus, under this condition, a light dose of 54 J cm−2 allows a complete E. coli cells inactivation on agar surface.
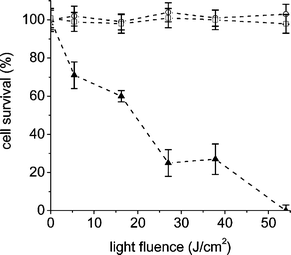 |
| Fig. 6 Inactivation of E. coli cells on nutrient agar. The cell suspension (∼106 CFU mL−1) in PBS was incubated with 1 µM of porphyrin A3B3+ (▲) for 30 min at 37 °C. Then, cells were plated on agar and irradiated with visible light for different times. Control culture untreated irradiated (□) and in dark (○). Values represent mean ± standard deviation of three separate experiments. | |
On the other hand, new experiments were performed to evaluate the photodynamic inactivation of E. coli cells that were not initially treated with the sensitizer in solution. In these experiments, the cells were grown as lawn on TS agar surface containing the sensitizer in a small area. Firstly, 10 nmol of different porphyrins were homogenously distributed in ∼0.6 cm2 on TS agar. The solvent was evaporated and the plates were spread with a suspension of E. coli, which allows obtaining a lawn of bacteria. The cultures were kept in dark for 20 min at 37 °C. During this period, the binding of sensitizer to E. coli cells can take place. Afterward, the plates were irradiated with visible light for 10 min and incubated for 18–24 h at 37 °C in dark. Characteristic results are shown in Fig. 7. As can be observed, growth of E. coli cells was not detected in the area treated with A3B3+ porphyrin. Similar behavior was also observed for tetracationic TPPA4+ porphyrin; however, in this case some small colonies were identified inside of the area. Samples of these colonies were aseptically transferred to fresh TS broth medium and plated on TS agar. After incubation at 37 °C, the viability of the E. coli cells was clearly evidenced by the formation of colonies. In contrast, modification of the lawn was not observed for controls treated with porphyrins and keeping in dark. Thus, the photodynamic inactivation was mainly observed for plates treated with A3B3+ and TTAP4+ porphyrins, although the photodamage increases using A3B3+ porphyrin probably due to a higher binding to cells as observed above in bacterial suspension.
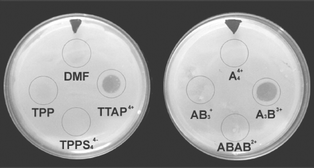 |
| Fig. 7 Inactivation of E. coli cells on TS agar irradiated with visible light for 10 min. The circles indicate the area where 10 nmol of sensitizer was spread from a solution 4.5 × 10−4 M in DMF. | |
Conclusions
New cationic porphyrins were evaluated as photosensitizers to inactivate E. coli cells in suspension of PBS and on agar surface. In these porphyrins, the cationic centers are isolated from the tetrapyrrolic macrocycle by a propoxy bridge. Thus, the cationic charges have minimal influence on the photophysical properties of the sensitizers. In addition, this chain provides a higher mobility of the charge, which could facilitate the interaction with the outer membrane of the Gram-negative bacteria. In solution, these sensitizers photodecompose DMA with a similar efficiency, producing O2(1Δg) with quantum yields of ∼0.41–0.53 in DMF (Table 1). Also, all the porphyrins studied give comparable photooxidation rates of Trp decomposition. However, in biological media the photophysical properties obtained in solution can significantly change, mainly depending on the cellular targets and the microenvironment where the sensitizer is localized.30 An active photocytotoxic effect on Gram-negative bacteria requires, not only of a high photodynamic activity of the sensitizer, if not also of the binding of the sensitizer to bacterial cells. These studies indicate that the tricationic A3B3+ porphyrin is highly bound to E. coli cells in comparison with other cationic porphyrins. Moreover, cationic porphyrins present a tight binding to E. coli still after three washing steps, in contrast with the anionic TPPS44− porphyrin. In vitro studies show that the photosensitized inactivation of E. coli cellular suspensions in PBS treated with 1 µM follows the order: A3B3+ > A44+
≫ ABAB2+ > AB3+. Also, a high photoinactivation activity of E. coli was found for TTAP4+, whereas a negligible effect was found for an anionic sensitizer, TPPS44−. The photodamage induced by these cationic porphyrins was also confirmed for the growth delay studies, indicating a faster inactivation of the E. coli cultures by tricationic A3B3+ porphyrin. In all cases, PDI of E. coli cells suspensions considerably diminishes in anoxic condition, indicating that an oxygen atmosphere is required for the mechanism of bacterial photodynamic inactivation. When the cells were treated with sensitizer in solution and immobilized on TS agar, it can be evidenced that A3B3+ porphyrin exerts a higher photodynamic activity than tetracationic TTAP4+ and A+4 porphyrins. Even so, the photodynamic inactivation capacity of A3B3+ porphyrin remains high for immobilized cells on agar surfaces containing an area spread with the sensitizer.
On the other hand, the mechanism of bacteria photoinactivation is not yet fully understood. Previous studies using T4MPyP conclude that the structural damage to the membrane of Gram-negative bacteria is induced by the singlet oxygen produced upon photosensitization when the photosensitizers reach the inner membrane and this damage plays a major role in bacterial photoinactivation.9 However, little is know about the affected sub-cellular sites and the nature of macromolecular damage during PDI killing of Gram-negative bacteria. It is known that tri- and tetra-cationic porphyrins take part in complex formation with nucleic acids, inducing lesions upon photoactivation.41,42 Studies of mechanistic aspects conclude that T4MPyP-dependent PDI of E. coli is primarily dependent on genomic DNA photodamage rather than on protein or membrane malfunctions.12 Thus, in the present investigation the efficiency of A3B3+ porphyrin could be influenced by the DNA binding. Further in vitro studies concerning the mechanism of cell inactivation produced by these cationic porphyrins are presently in progress in our laboratory.
Therefore, the results indicate that the tricationic A3B3+ porphyrin, bearing charges separated from the macrocycle ring by a spacer, is an interesting photosensitizer agent with applications in the treatment of bacteria in suspensions and on surfaces by PDI.
Acknowledgements
Authors are grateful to Consejo Nacional de Investigaciones Científicas y Técnicas (CONICET) of Argentina, Fundación Antorchas and SECYT Universidad Nacional de Río Cuarto for financial support. E.N.D. is a Scientific Members of CONICET. D.A.C. thanks Fundación Antorchas for a research fellowship.
References
- P. W. Taylor, P. D. Stapleton and J. P. Luzio, New ways to treat bacterial infections, Drug Discovery Today, 2002, 7, 1086–1091 CrossRef.
- M. Wainwright, Photodynamic antimicrobial chemotherapy (PACT), J. Antimicrob. Chemother., 1998, 42, 13–28 CrossRef CAS.
- M. R. Hamblin and T. Hasan, Photodynamic therapy: a new antimicrobial approach to infectious disease?, Photochem. Photobiol. Sci., 2004, 3, 436–450 RSC.
- M. Ochsner, Photophysical and photobiological processes in photodynamic therapy of tumours, J. Photochem. Photobiol., B, 1997, 39, 1–18 CrossRef CAS.
- M. C. DeRosa and R. J. Crutchley, Photosensitized singlet oxygen and its applications, Coord. Chem. Rev., 2002, 233–234, 351–371 CrossRef CAS.
- M. Merchat, G. Bertoloni, P. Giacomini, A. Villanueva and G. Jori, Meso-substituted cationic porphyrins as efficient photosensitizers of Gram-positive and Gram-negative bacteria, J. Photochem. Photobiol., B, 1996, 32, 153–157 CrossRef CAS.
- M. Merchat, G. Spikes, G. Bertoloni and J. Jori, Studies on the mechanism of bacteria photosensitization by meso-substituted cationic porphyrins, J. Photochem. Photobiol., B, 1996, 35, 149–157 CrossRef CAS.
- Y. Nitzan, A. Balzam-Sudakevitz and H. Ashkenazi, Eradication of Acinetobacter baumanni by photosensitized agents in vitro, J. Photochem. Photobiol., B, 1998, 42, 211–218 CrossRef CAS.
- Y. Nitzan and H. Ashkenazi, Photoinactivation of Acinetobacter baumannii and Escherichia coli B by cationic hydrophilic porphyrin at various light wavelengths, Curr. Microbiol., 2001, 42, 408–414 CrossRef CAS.
- E. Reddi, M. Ceccon, G. Valduga, G. Jori, J. C. Bommer, F. Elisei, L. Latterini and U. Mazzucato, Photophysical properties and antibacterial activity of meso-substituted cationic porphyrin, Photochem. Photobiol., 2002, 75, 462–470 CrossRef CAS.
- D. Lazzeri, M. Rovera, L. Pascual and E. N. Durantini, Photodynamic studies and photoinactivation of Escherichia coli using meso-substituted cationic derivatives with asymmetric charge distribution, Photochem. Photobiol., 2004, 80, 286–293 CrossRef CAS.
- M. Salmon-Divon, Y. Nitzan and Z. Malik, Mechanistic aspect of Escherichia coli photodynamic inactivation by cationic tetra-meso(N-methylpyridyl)porphine, Photochem. Photobiol. Sci., 2004, 3, 423–429 RSC.
- E. A. Dupouy, D. Lazzeri and E. N. Durantini, Photodynamic activity of cationic and non-charged Zn(II) tetrapyridinoporphyrazine derivatives: biological consequences in human erythrocytes and Escherichia coli, Photochem. Photobiol. Sci., 2004, 3, 992–998 RSC.
- D. A. Caminos and E. N. Durantini, Synthesis of asymmetrically meso-substituted porphyrins bearing amino groups as potential cationic photodynamic agents, J. Porphyrin Phthalocyanine, 2005, 9, 334–342 Search PubMed.
- A. Ando and I. Kumadaki, Progress on the syntheses of fluorine analogs of natural porphyrins potentially useful for the diagnosis and therapy of certain cancers, J. Fluor. Chem., 1999, 100, 135–146 Search PubMed.
- J. C. P. Grancho, M. M. Pereira, M. da G. Miguel, A. M. Rocha Gosnsalves and H. D. Burrows, Synthesis, spectra and photophysics of some free base tetrafluoroalkyl and tetrafluoroaryl porphyrins with potential applications in imaging, Photochem. Photobiol., 2002, 75, 249–256 CAS.
- M. E. Milanesio, M. S. Moran, E. I. Yslas, M. G. Alvarez, V. Rivarola and E. N. Durantini, Synthesis and biological evaluation of methoxyphenyl porphyrin derivatives as potential photodynamic agents, Bioorg. Med. Chem., 2001, 9, 1943–1949 CrossRef CAS.
- R. W. Redmond and J. N. Gamlin, A compilation of singlet yields from biologically relevant molecules, Photochem. Photobiol., 1999, 70, 391–475 CAS.
- R. Schmidt and E. Afshari, Effect of solvents on the phosphorescence rate constant of singlet molecular oxygen (1Δg, J. Phys. Chem., 1990, 94, 4377–4378 CrossRef.
-
J. G. Holt, N. R. Krieg, P. H. A. Sneath, J. T. Staley, and S. T. Williams, in Bergey's manual of determinative bacteriology, ed. W. R. Hensyl, Williams and Wilkins, Baltimore, MD, ninth edition, 1994 Search PubMed.
- M. Kreitner, K.-H. Wagner, G. Alth, R. Ebermann, H. Foiβy and I. Elmadfa, Haematoporphyrin- and sodium chlorophyllin-induced phototoxicity towards bacteria and yeasts-a new approach for safe foods, Food Control, 2001, 12, 529–533 CrossRef CAS.
-
K. M. Smith, in Porphyrins and Metalloporphyrins, General features of the structure and chemistry of porphyrin compounds, Elsevier, Amsterdam, 1975, ch. 1, pp. 20–27 Search PubMed.
- K. Kano, K. Fukuda, H. Wakami, R. Nishiyabu and R. F. Pasternack, Factor influencing self-aggregation tendencies of cationic porphyrins in aqueous solutions, J. Am. Chem. Soc., 2000, 122, 7494–7502 CrossRef CAS.
- D. L. Akins, H.-R. Zhu and C. Guo, Aggregation of tetraaryl-substituted porphyrins in homogeneous solution, J. Phys. Chem., 1996, 100, 5420–5425 CrossRef CAS.
- G. Csík, E. Balog, I. Voszka, F. Tölgyesi, D. Oulmi, Ph. Maillard and M. Momenteau, Glycosylated derivatives of tetraphenyl porphyrin: photophysical characterization, self-aggregation and membrane binding, J. Photochem. Photobiol., B, 1998, 44, 216–224 CrossRef CAS.
- K. Kano, K. Fukuda, H. Wakame, R. Nishiyabu and R. F. Pasternack, Factor influencing self-aggregation tendencies of cationic porphyrins in aqueous solutions, J. Am. Chem. Soc., 2000, 122, 7494–7502 CrossRef CAS.
- M. Pineiro, A. L. Carvalho, M. M. Pereira, A. M. d'A. Rocha Gonsalves, L. G. Arnaut and S. J. Formosinho, Photoacoustic measurements of porphyrin triplet-state quantum yields and singlet-oxygen efficiencies, Chem.–Eur. J., 1998, 4, 2299–2307 CrossRef CAS.
- M. E. Milanesio, M. G. Alvarez, J. J. Silber, V. Rivarola and E. N. Durantini, Photodynamic activity of monocationic and non-charged methoxyphenylporphyrin derivatives in homogeneous and biological media, Photochem. Photobiol. Sci., 2003, 2, 926–933 RSC.
- M. E. Milanesio, M. G. Alvarez, E. I. Yslas, C. D. Borsarelli, J. J. Silber, V. Rivarola and E. N. Durantini, Photodynamic studies of metallo 5,10,15,20-tetrakis(4-methoxyphenyl) porphyrin: photochemical characterization and biological consequences in a human carcinoma cell line, Photochem. Photobiol., 2001, 74, 14–21 CAS.
- B. M. Aveline and R. W. Redmond, Can cellular phototoxicity be accurately predicted on the basic of sensitizer photophysics?, Photochem. Photobiol., 1999, 69, 306–316 CAS.
- A. Segalla, C. Milanesi, G. Jori, H.-G. Capraro, U. Isele and K. Schieweck, CGP 55398, a liposomal Ge(IV) phthalocyanine bearing two axially ligated cholesterol moieties: a new potential agent for photodynamic therapy of tumours, Br. J. Cancer, 1994, 69, 817–825 CAS.
- H. Ali and J. E. van Lier, Metal complexes as photo- and radiosensitizers, Chem. Rev., 1999, 99, 2379–2450 CrossRef.
- G. Ferraudi, G. A. Argüello, H. Ali and J. E. van Lier, Types I and II sensitized photooxidation of aminoacid by phthalocyanines: a flash photochemical study, Photochem. Photobiol., 1988, 47, 657–660 CrossRef CAS.
- M. E. Daraio, A. Völker, P. A. Aramendía and E. San Román, Tryptophan quenching of zinc-phthalocyanine and porphycene fluorescence in micellar CTAC, Photochem. Photobiol., 1998, 67, 371–377 CAS.
- J. M. Wessels, C. S. Foote, W. E. Ford and M. A. J. Rodgers, Photooxidation of tryptophan: O21Δg) versus electron-transfer pathway, Photochem. Photobiol., 1997, 65, 96–102 CrossRef CAS.
- A. Minnock, D. I. Vernon, J. Schofield, J. Griffiths, J. H. Parish and S. B. Brown, Photoinactivation of bacteria. Use of a cationic water-soluble zinc phthalocyanines to photoinactivate both Gram-negative and Gram-positive bacteria, J. Photochem. Photobiol., B, 1996, 32, 159–164 CrossRef CAS.
- L. Benov, I. Batinić-Haberle, I. Spasojević and I. Fridovich, Isomeric N-alkylpyridylporphyrins and their Zn(II) complexes: inactive as SOD mimics but powerful photosensitizers, Arch. Biochem. Biophys., 2002, 402, 159–165 CrossRef CAS.
- B. M. Aveline, R. M. Sattler and R. W. Redmond, Environmental effects on cellular photosensitization: correlation of phototoxicity mechanism with transient absorption spectroscopy measurements, Photochem. Photobiol., 1998, 68, 51–62 CrossRef CAS.
- A. Orenstein, D. Klein, J. Kopolovic, E. Winkler, Z. Malik, N. Keller and Y. Nitzan, The use of porphyrins for eradication of Staphylococcus aureus in burn wound infections, FEMS Immunol. Med. Microbiol., 1998, 19, 307–314 CrossRef CAS.
- S. Wood, B. Nattress, J. Kirkham, R. Shore, S. Brookes, J. Griffiths and C. Robinson, An in vitro study of the use of photodynamic therapy for the treatment of natural oral plaque biofilms formed in vivo, J. Photochem. Photobiol., B, 1999, 50, 1–7 CrossRef CAS.
- P. Kubát, K. Lang, P. Anzenbacher, Jr., K. Jursíková, V. Král and B. Ehrenberg, Interaction of novel cationic meso-tetraphenylporphyrins in the ground and excited states with DNA and nucleotides, J. Chem. Soc., Perkin Trans. 1, 2000, 933–941 RSC.
- H. Li, O. S. Fedorova, A. N. Grachev, W. R. Trumble, G. A. Bohach and L. Czuchajowski, A series of meso-tris(N-methyl-pyridiniumyl)-(4-alkylamidophenyl) porphyrins: synthesis, interaction with DNA and antibacterial activity, Biochim. Biophys. Acta, 1997, 1354, 252–260 CrossRef CAS.
|
This journal is © The Royal Society of Chemistry and Owner Societies 2006 |
Click here to see how this site uses Cookies. View our privacy policy here.