The temperature dependence of porphyrin production in Propionibacterium acnes after incubation with 5-aminolevulinic acid (ALA) and its methyl ester (m-ALA)
Received
9th September 2005
, Accepted 19th October 2005
First published on 10th November 2005
Abstract
Topical PDT treatment of the common skin disease acne vulgaris is now in clinical use. Propionibacterium acnes (P. acnes) is known to play an important role in acne. 5-Aminolevulinic acid (ALA) supplementation leads to an enhanced porphyrin production in the bacteria. Subsequent illumination with light of the proper wavelengths can reduce the number of bacteria and this might at least partly explain the PDT effect on acne. We have assessed the effects of temperature on P. acnes washed cell suspensions incubated for 4 h with ALA or ALA methyl ester (m-ALA). The effect on porphyrin production of both the cell suspension incubation temperature as well as the initial growth temperature of the cultivated cells prior to harvesting and use in suspension experiments was investigated. The bacterial porphyrin content was estimated from fluorescence emission spectra. It was found that incubation with ALA or m-ALA at a temperature 42 °C resulted in an approx. 100% and 33% increase in the total amount of PDT-relevant porphyrins produced as compared to incubation at 37 °C. These results support increasing the skin temperature during incubation with ALA or m-ALA in the clinic. The initial growth temperature, prior to the incubation, had no apparent effect on the ALA or m-ALA induced porphyrins. Activation energy studies indicate slightly higher temperature dependence in the case of ALA produced porphyrins as compared to m-ALA produced porphyrins (77 and 65 kJ mol−1, respectively).
1 Introduction
Propionibacterium acnes (P. acnes) is the predominant bacterial resident within the pilosebaceous follicles of sebum-rich areas of the human skin.1–3P. acnes has also been reported to be closely connected with the skin disease acne vulgaris.4
This Gram-positive bacterium produces small amounts of porphyrins, mostly coproporphyrin III (CpIII) and uroporphyrin III (UpIII).5–8 Due to the porphyrin production clusters of P. acnes bacteria may be seen as fluorescent spots on the skin when irradiated with broad-band near UV-light (cf. Woods light). The endogenous porphyrins make the bacteria light sensitive and several investigations point to light destruction mechanisms that are mediated via excited porphyrins and subsequent singlet oxygen reactions.5,9,10
A limiting factor in the porphyrin biosynthesis in several eukaryotic as well as prokaryotic cells is the formation of 5-aminolevulinic acid (ALA). This fact is taken advantage of in the so called photodynamic therapy, PDT, used against some types of cancer. By presenting the cancer cells to ALA or ALA-derivatives, the cells increase their porphyrin content, in most cases protoporphyrin IX (PpIX), and are subsequently illuminated and killed via photosensitizing mechanisms (ALA-PDT).
ALA is also taken up by P. acnes and the result is an increased concentration of endogenous CpIII and UpIII. This enhances the light sensitivity of the bacteria.5 There is a current interest in using ALA-PDT in the treatment of acne disease with ALA administered to the skin.4,11–13
It is known that the rate of PpIX production in cancer cells after ALA incubation depends on the incubation temperature.14,15 A clinical use of ALA-PDT against acne makes it relevant to study the effect of temperature during ALA incubation of the bacteria. The natural environment of the P. acnes bacteria in the skin will vary according to a number of parameters. The temperature of the surroundings and the person's level of activity seem to be important factors and it will in most cases be substantially lower than 37 °C used in most studies. Furthermore, the initial growth temperature (i.e. prior to ALA/ALA ester incubation) of the bacteria could play a role in the porphyrin pattern of the bacteria.
In ALA-PDT treatment of various forms of skin cancer it has been found that the methylated form of ALA (m-ALA) shows better results compared to ALA due to a more efficient transport through the stratum corneum of the skin.16,17 The use of m-ALA might also be more efficient in the use of ALA-PDT against acne and we, therefore, wanted to compare the use of m-ALA compared to that of ALA on P. acnes.
2 Materials and methods
All chemicals were purchased from Sigma-Aldrich (St. Louis, MO) unless specified otherwise.
2.1 Bacteria and growth conditions
P. acnes were purchased from American Type Culture Collection (ATCC number 6919), grown anaerobically in the dark on blood–agar plates at 37 °C and replated once a week.18 Prior to the experiments the bacteria were grown at different temperatures (for different duration): At 37 °C (2–3 days of growth), at 30 °C (proper growth requiring 5 days), and at 25 °C (7 days). No growth was found for bacteria grown, under these conditions, at 21 °C or below. The temperature variation during growth and incubation was less than 0.1 °C.
Bacterial suspensions were prepared as follows: An experimental buffer solution (PIPES 20 mM, KCl 100 mM, pH 7.2, approx. 1 ml) was added to the bacterial growth on each blood–agar Petri-plate and with the help of a sterile glass-rod and a Pasteur pipette, the cells were harvested into suspension and transferred to a 20 ml test-tube. The test-tube was filled with buffer solution and the bacteria were then washed twice by centrifugation (2,500 g, 5 min) and resuspended in the buffer. The bacterial suspension was then diluted with experimental buffer until the optical density (OD) was between 0.98 and 1.02 at 550 nm as measured by a spectrophotometer (Shimadzu, UV-1601PC). At this OD the bacterial density was approximately 5 × 108 cells ml−1.18
2.2 Incubation with ALA and m-ALA
5-Aminolevulinic acid (ALA) and ALA methyl ester (m-ALA) were dissolved in dimethyl sulfoxide to a concentration of 500 mM (stock solution). Dissolution and dilution was performed immediately before addition to the bacteria. The bacteria were incubated with ALA or m-ALA to a final concentration of 1 mM in experimental buffer for 4 h under dark conditions.
2.3 Fluorescence spectroscopy
The ALA and m-ALA induced porphyrins were monitored using the fluorescence emission spectra. Aliquots of 2 ml bacterial suspensions were transferred to standard disposable plastic cuvettes and placed in a Perkin Elmer LS50B fluorescence spectrometer. The excitation was set to 405 nm and the fluorescence emission was monitored at 550–700 nm. The fairly broad range of wavelengths measured was motivated from the need to have a control of the autofluorescence from the bacteria—most easily discernible at the lower or higher wavelengths. The excitation and emission slits were both 5 nm and the emission monochromator speed was 300 nm min−1.
2.4 Arrhenius plots and activation energies
The activation energy, Ea, for ALA and m-ALA was determined by the method of linear least squares regression analysis of a plot of log k against 1/T according to the Arrhenius equation:
Here A is a constant, R is the general gas constant (8.314 kJ mol−1), and T is the absolute temperature in Kelvin. The rate constant k of the ALA/m-ALA induced porphyrins was approximated using the assumption that k = Δc/Δt
∝
I/τ, where Δc is the increase in porphyrin concentration, Δt is the corresponding increase in incubation time, I is the fluorescence intensity at the characteristic wavelength, and τ is the total incubation time.
3 Results
3.1 Fluorescence measurements and description of spectra
The induction of PDT-relevant porphyrins in P. acnes was determined using fluorescence emission spectroscopy. The fluorescence emission intensity increased with increasing incubation time in an approx. linear fashion up to 4 h for both ALA and m-ALA (data not shown). Figs. 1 and 2 show the fluorescence emission spectra of the total induced porphyrins in P. acnes suspensions after 4 h ALA and m-ALA incubation, respectively. The incubation temperatures were between 10 and 42 °C as indicated in the insert. Prior to the incubation the bacteria were grown at 37 °C for 2–3 days.
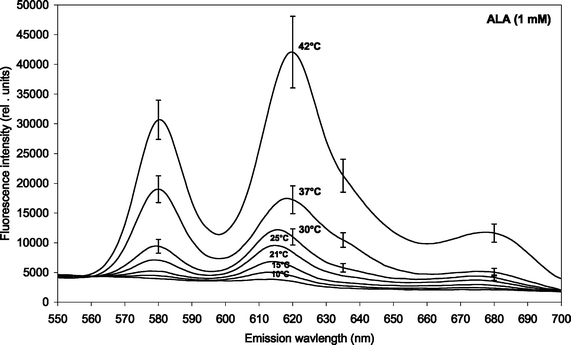 |
| Fig. 1 Fluorescence emission spectra of ALA-induced porphyrins in P. acnes (both endogenous and exogenous porphyrins). The bacteria were incubated with 1 mM ALA for 4 h at different temperatures (10, 15, 21, 25, 30, 37 and 42 °C). Prior to the incubation the bacteria were cultivated at 37 °C for 2–3 days. Mean spectra based on four independent experiments. The standard errors of the mean (SE) have been included for the three highest temperatures only (for clarity). | |
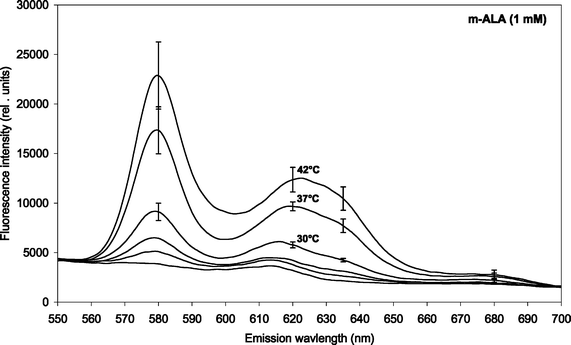 |
| Fig. 2 Fluorescence emission spectra of m-ALA-induced porphyrins in P. acnes (both endogenous and exogenous porphyrins). The bacteria were incubated with 1 mM m-ALA for 4 h at different temperatures (15, 21, 25, 30, 37 and 42 °C). Prior to the incubation the bacteria were cultivated at 37 °C for 2–3 days. Mean spectra based on four independent experiments including SE. Note: Different scaling of y-axis as compared to Fig. 1 above. | |
Spectral peaks were found at about 580 nm and 620 nm, and there is probably a shoulder at about 635 nm. The 620 nm peak was ascribed to coproporphyrin III and uroporphyrin III5,19,20 whereas the shoulder at 635 nm corresponds to protoporphyrin IX.5 The presence of PpIX was thus marginal under the conditions of the present study. The peak at 580 nm has been ascribed earlier to metalloporphyrins being formed.7 It is likely that the metalloporphyrins are formed from either free-base porphyrins by Mg2+-ions present in the culture medium, or by incorporation of zinc ions originating from the glassware.
Fig. 3 and 4 show the temperature dependence of fluorescence emission at 620 nm (due to endogenous CpIII and UpIII) from P. acnes suspensions after ALA and m-ALA incubation, respectively. It can be seen that the amount of PDT-relevant porphyrins formed in P. acnes in vitro depends on the incubation temperature. The values at 10–15 °C represent the background autofluorescence from the cell suspensions (i.e. no porphyrins formed). As the incubation temperature is increased the PDT-relevant porphyrin concentration is increased correspondingly. As an example, the fluorescence (adjusted for autofluorescence) was increased by approx. 100% (ALA) and approx. 33% (m-ALA) when the incubation temperature was raised from 37 to 42 °C. The figures also show that the growth temperature (i.e. the temperature during the cell cultivation stage and prior to the ALA/m-ALA incubation) did not influence the amount of PDT-relevant porphyrins formed—with the exception of ALA incubation at 42 °C.
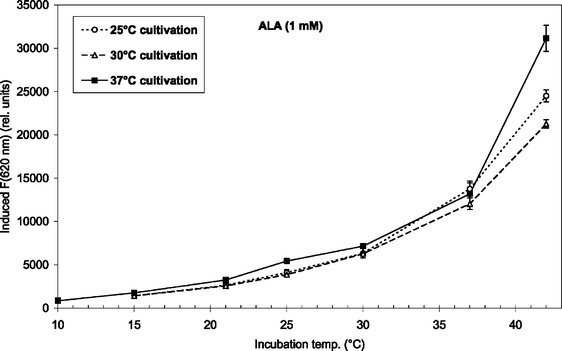 |
| Fig. 3 ALA-induced fluorescence at 620 nm from endogenous porphyrins after 4 h incubation (1 mM ALA) at temperatures between 10 and 42 °C. Prior to the incubation the bacteria were grown at 25 °C (7 days), 30 °C (5 days), or 37 °C (2–3 days) as indicated in the figure insert. | |
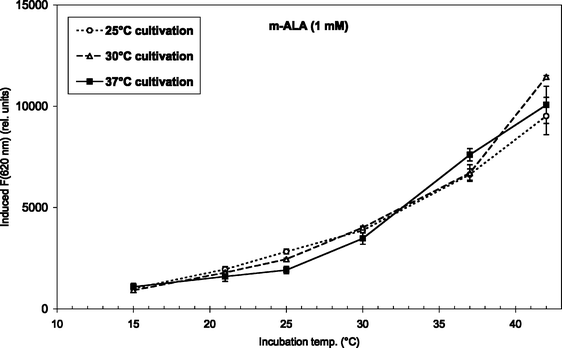 |
| Fig. 4 m-ALA-induced fluorescence at 620 nm from endogenous porphyrins after 4 h incubation (1 mM m-ALA) at temperatures between 15 and 42 °C. Prior to the incubation the bacteria were grown at 25 °C (7 days), 30 °C (5 days), or 37 °C (2–3 days) as indicated in the figure insert. | |
3.2 Arrhenius plots and activation energies
To find the activation energies, the logarithm of the mean fluorescence intensities (as given in Fig. 3 and 4) were plotted as a function of inverse absolute temperature. The results with regression lines are shown in Fig. 5 and 6, for ALA and m-ALA, respectively. Based on the calculated regression lines from the Arrhenius plots the activation energies could be determined as presented in Table 1.
Table 1 Activation energies (Ea) for CpIII/UpIII induction (monitored as fluorescence at 620 nm). Bacteria were incubated as washed cell suspensions with ALA or m-ALA for 4 h at temperatures between 10 and 42 °C. Prior to this the bacteria were cultivated at 25 °C (7 days), 30 °C (5 days) or 37 °C (2–3 days)
Substance |
Growth temp./°C |
E
a/kJ mol−1 |
R
2
|
ALA |
25 |
78.87 |
0.997 |
ALA |
30 |
73.87 |
0.992 |
ALA |
37 |
80.30 |
0.983 |
m-ALA |
25 |
61.17 |
0.994 |
m-ALA |
30 |
68.54 |
0.997 |
m-ALA |
37 |
66.04 |
0.977 |
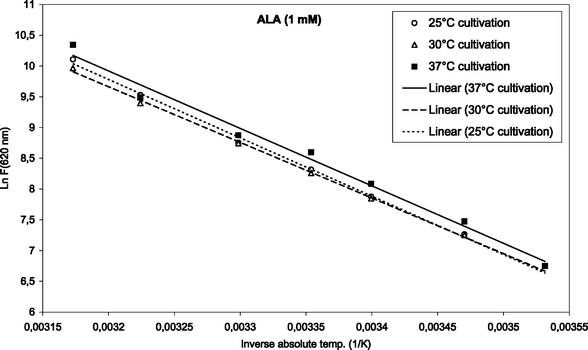 |
| Fig. 5 Arrhenius plot of temperature-dependent ALA-induced porphyrins (1 mM, up to 42 °C). The initial cultivation temperature was either 25, 30 or 37 °C as indicated in the figure. | |
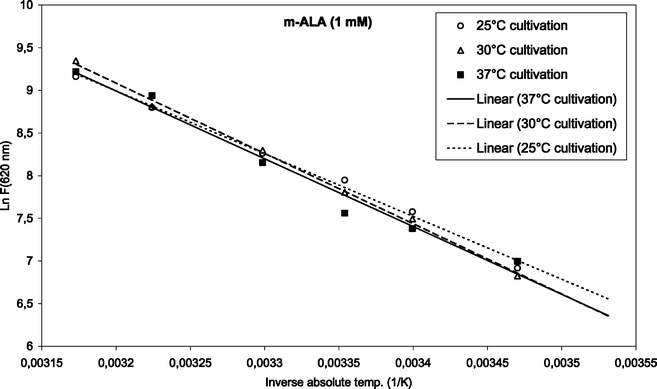 |
| Fig. 6 Arrhenius plot of temperature-dependent m-ALA-induced porphyrins (1 mM, up to 42 °C). The initial cultivation temperature was either 25, 30 or 37 °C as indicated in the figure. | |
4 Discussion
Clinical use of PDT for acne
The results in the present study show that elevating the skin temperature during ALA application could be a factor in improving ALA-based PDT against acne. The amount of PDT-relevant porphyrins formed in P. acnes in vitro was increased by approx. 100% (ALA) and 33% (m-ALA), respectively, when the incubation temperature was raised from 37 to 42 °C. Thus, it would be favourable to heat the skin (e.g. up to 42 °C) during the topical ALA or ALA ester application. An increased temperature could also be used for decreasing the incubation time which would be useful from a clinical point of view.
It was also found that the initial cultivation temperature of the bacteria (25, 30 or 37 °C) did not influence the amount of PDT-relevant porphyrins induced after incubation with ALA or m-ALA at incubation temperatures between 10 and 37 °C. This is an advantage from a clinical point of view as it means that topical application of ALA or m-ALA will induce porphyrins independent of the skin temperature that the patient has had prior to the clinic visit. This temperature will normally be lower than 37 °C. At 42 °C ALA-incubation there was a maximum porphyrin production in those bacteria that had been cultivated at 37 °C. However, initial cultivation at 30 °C resulted in less porphyrins than initial cultivation at 25 °C (when ALA-incubated at 42 °C). This deserves further study.
The optimum temperature during irradiation of the skin has not been highlighted in the present report. It may be that the temperature during the illumination should preferably be reduced. It has been reported that cells illuminated at low temperatures are more efficiently inactivated than cells illuminated at normal temperatures.21 Whether this is also true for bacteria remains to be found.
Production of porphyrins in P. acnes.
The rationale for using ALA-PDT against acne is based on ALA/ALA esters penetrating the sebaceous glands of the skin and reaching P. acnes in the deeper recess of this gland. This leads to a corresponding porphyrin production in the P. acnes bacteria and a corresponding enhanced sensitivity towards blue and red light. The bacteria can thus be killed via photosensitizing reactions. The ALA-PDT method of treating acne may thus resemble the traditional methods, i.e. application of antimicrobial drugs such as benzoyl peroxide, as it aims to reduce the number of P. acnes present. Although, P. acnes is not pathogenic by normal standards22 the bacterium appears to be the main target for (both oral and topical) traditional antimicrobial treatment. It should be emphasised that the pathogenesis of acne vulgaris is by no means clear and PDT could be beneficial by several mechanisms, not only directly against P. acnes.23
P. acnes bacteria in the skin are known to produce endogenous porphyrins, particularly coproporphyrin III and uroporphyrin III.5,8,19,24 Although the quantity of endogenous porphyrins produced in P. acnes is small, ALA or m-ALA supplementation to the growth medium or to a cell suspension incubation medium greatly enhances the rate of porphyrins produced in vitro, as shown in the present study. Both CpIII and UpIII have been reported to be efficient singlet oxygen (1O2) producers19 and ALA or m-ALA incubation thus increases the photosensitivity.5 In addition to the PDT-relevant porphyrins CpIII and UpIII we also find metalloporphyrins (at 580 nm) in the P. acnes strain used. Metalloporphyrins are also found after ALA incubation of Escherichia coli.25 In contrast to ALA-incubated mammalian cells, the concentration of protoporphyrin IX in the P. acnes bacteria was only marginal under the conditions used.
The present study does not address the bacterial survival rate in different concentrations of the ALA or m-ALA. However, this relevant point will hopefully be answered in a study now under way.
Activation energy analysis.
The activation energy (Ea) for PDT-relevant porphyrin production was derived from Arrhenius plots based on the fluorescence data. Although the method applied is crude some relevant results were achieved for the 4 h incubation intervals used in the experiments. The overall Ea for the production of CpIII and UpIII in the bacteria does of course not specify the different reaction steps involved in the porphyrin production. For P. acnes the Ea of the ALA-induced CpIII and UpIII fluorescence was 77.7 kJ mol−1 on average (Table 1). This value is similar to the value reported by Cardalda et al. for the activity of the mammalian enzyme porphobilinogen deaminase (PBG-D), 76.1 kJ mol−1.26 If we assume that eukaryotic and prokaryotic PBG-D are somewhat similar, then this suggests that PBG-D represents the rate-limiting step for CpIII and UpIII production in P. acnes bacteria when incubated with ALA. In the case of methyl ALA-induced CpIII and UpIII fluorescence in P. acnes, the mean Ea was found to be 65.3 kJ mol−1. This is slightly lower than found in the case of ALA and may indicate a different transport mechanism for m-ALA. We have not performed any studies of uptake mechanisms for ALA and m-ALA. However, in the case of eukaryotic cells, there is some evidence that ALA and m-ALA are transported into cells via different routes.27,28
In the case of ALA incubation, the Ea varied between 73.9 and 80.3 kJ mol−1 when using different cultivation temperatures. Similarly for m-ALA the Ea varied between 61.2 and 68.5 kJ mol−1. However, there was no apparent correlation between the growth temperatures used and the activation energy of the ALA or m-ALA-induced CpIII/UpIII. The growth temperature, therefore, did not play a decisive role for the production of CpIII/UpIII after ALA/m-ALA incubation.
The formation of protoporphyrin IX (PpIX) in human skin during topical application of ALA is also temperature dependent, with an activation energy of about 71.2 kJ mol−1.14 It is interesting to observe that this temperature dependence was found to be mainly related to porphyrin production and not to ALA penetration into the skin, which was almost temperature independent.14
ALA versus m-ALA.
In ALA-PDT treatment of various forms of skin cancer it has been found that m-ALA produces better results than ALA due to a better transport through the stratum corneum of the skin.16,17 The results of the present study indicate that m-ALA may be an interesting alternative to ALA in a PDT treatment of acne due to potentially better penetration properties into the deeper recess of the sebaceous glands. This deserves further clarification.
Abbreviations
ALA, 5-aminolevulinic acid; CpIII, coproporphyrin III; Ea, activation energy; m-ALA, ALA methyl ester; P. acnes, Propionibacterium acnes; PDT, photodynamic therapy; PpIX, protoporphyrin IX; UpIII, uroporphyrin III.
Acknowledgements
Post doctoral funding from the Faculty of Science at NTNU is gratefully acknowledged by S. Ramstad.
References
- K. J. McGinley, G. F. Webster and J. J. Leyden, Regional variations of cutaneous Propionibacteria, Appl. Environ. Microbiol., 1978, 35, 62–66 CAS.
- J. P. Leeming, K. T. Holland and W. J. Cunliffe, The microbial ecology of pilosebaceous units isolated from human skin, J. Gen. Microbiol., 1984, 130, 803–807 CAS.
- J. P. Leeming, K. T. Holland and W. J. Cunliffe, The pathological and ecological significance of microorganisms colonizing acne vulgaris comedones, J. Med. Microbiol., 1985, 20, 11–16 CrossRef CAS.
- W. J. Cunliffe and V. Goulden, Phototherapy and acne vulgaris, Br. J. Dermatol., 2000, 142, 853–856 CrossRef.
- S. Ramstad, C. M. Futsaether and A. Johnsson, Porphyrin sensitization and intracellular calcium changes in the prokaryote Propionibacterium acnes, J. Photochem. Photobiol., B, 1997, 40, 141–148 CrossRef CAS.
- R. Romiti, M. Schaller and K. J. G. Plewig, High-performance liquid chromatography analysis of porphyrins in Propionibacterium acnes, Arch. Dermatol. Res., 2000, 292, 320–322 CrossRef CAS.
- A. Johnsson, B. Kjeldstad and T. B. Melø, Fluorescence from pilosebaceous follicles, Arch. Dermatol. Res., 1987, 279, 190–193 CAS.
- B. Kjeldstad, A. Johnsson and S. Sandberg, Influence of pH on porphyrin production in Propionibacterium acnes, Arch. Dermatol. Res., 1984, 276, 396–400 CrossRef CAS.
- B. Kjeldstad, Different photoinactivation mechanisms in Propionibacterium acnes for near-ultraviolet and visible light, Photochem. Photobiol., 1987, 46, 363–366 CrossRef CAS.
- T. B. Melø and G. Reisæther, Photodestruction of endogenous porphyrins in relation to cellular inactivation of Propionibacterium acnes, Z. Naturforsch., 1986, 41c, 867–872 Search PubMed.
- W. Hongcharu, C. R. Taylor, Y. Chang, D. Aghassi, K. Suthamjariya and R. R. Anderson, Topical ALA-Photodynamic therapy for the treatment of acne vulgaris, J. Invest. Dermatol., 2000, 115, 183–192 CrossRef CAS.
- Y. Itoh, Y. Nimomiya, S. Tajima and A. Ishibashi, Photodynamic therapy for acne vulgaris with topical 5-aminolevulinic acid, Arch. Dermatol., 2000, 136, 1093–1095 CAS.
- B. Pollock, D. Turner, M. R. Stringer, R. A. Bojar, V. Goulden, G. I. Stables and W. J. Cunliffe, Topical aminolaevulinic acid-photodynamic therapy for the treatment of acne vulgaris: a study of clinical efficacy and mechanism of action, Br. J. Dermatol., 2004, 151, 616–22 CrossRef CAS.
- J. Moan, K. Berg, O. B. Gadmar, V. Iani, L. W. Ma and P. Juzenas, The temperature dependence of protoporphyrin IX production in cells and tissues, Photochem. Photobiol., 1999, 70, 669–673 CrossRef CAS.
- P. Juzenas, R. Sørensen, V. Iani and J. Moan, Uptake of topically applied 5-aminolevulinic acid and production of protoporphyrin IX in normal mouse skin: dependence on skin temperature, Photochem. Photobiol., 1999, 69, 478–481 CrossRef CAS.
- A. Juzeniene, P. Juzenas, V. Iani and J. Moan, Topical application of 5-aminolevulinic acid and its methylester, hexylester and octylester derivatives: considerations for dosimetry in mouse skin model, Photochem. Photobiol., 2002, 76, 329–334 CrossRef CAS.
- Q. Peng, K. Berg, J. Moan, M. Kongshaug and J. M. Nesland, 5-Aminolevulinic acid based photodynamic therapy: principles and experimental research, Photochem. Photobiol., 1997, 65, 235–251 CrossRef CAS.
- C. M. Futsaether, C. M. Kjeldstad and A. Johnsson, Intracellular pH changes induced in Propionibacterium acnes by UV-A radiation and blue light, J. Photochem. Photobiol., B, 1995, 31, 125–131 CrossRef CAS.
- K. Arakane, A. Ryu, C. Hayashi, T. Msunaga, K. Shinmoto, S. Mashiko, T. Nagano and M. Hirobe, Singlet oxygen (1Δg)
generation from coproporphyrin in Propionibacterium acnes on irradiation, Biochem. Biophys. Res. Commun., 1996, 223, 578–582 CrossRef CAS.
- H. Ashkenazi, Z. Malik, Y. Harth and Y. Nitzan, Eradication of Propionibacterium acnes by its endogenic porphyrins after illumination with high intensity blue light, FEMS Immunol. Med. Microbiol., 2003, 21, 17–24 CrossRef.
- J. Moan and T. Christensen, Photodynamic inactivation of cancer cells in vitro. Effect of irradiation temperature and dose fractionation, Cancer Lett., 1979, 6(6), 331–5 CrossRef CAS.
- C. G. Burkhart, C. N. Burkhart and P. F. Lehmann, Acne: a review of immunologic and microbiologic factors, Postgrad. Med. J., 1999, 75, 328–331 CAS.
- T. Maisch, R. Szeimies, G. Jori and C. Abels, Antibacterial photodynamic therapy in dermatology, Photochem. Photobiol. Sci., 2004, 3, 907–917 RSC.
- S. L. Wei-Li, A. R. Shalita and M. B. Poh-Fitzpatrick, Comparative studies of porphyrin production in Propionibacterium acnes and Propionibacterium granulosum, J. Bacteriol., 1978, 133, 811–815.
- K. Szocs, F. Gabor, G. Csik and J. Fidy, δ-Aminolaevulinic acid-induced porphyrin synthesis and photodynamic inactivation of E. coli B, J. Photochem. Photobiol., B, 1999, 50, 8–17 CrossRef CAS.
- C. A. Cardalda, A. A. Juknat, F. G. Princ and A. Batlle, Rat harderian gland porphobilinogen deaminase: characterization studies and regulatory action of protoporphyrin IX, Arch. Biochem. Biophys., 1997, 347, 69–77 CrossRef CAS.
- E. Rud, O. A. Gederaas, A. Hogset and K. Berg, 5-Aminolevulinic acid, but not 5-aminolevulinic acid esters, is transported into adenocarcinoma cells by system BETA transporters, Photochem. Photobiol., 2000, 71, 640–647 CAS.
- O. A. Gederaas, A. Holroyd, S. B. Brown, D. Vernon, J. Moana and K. Berg, 5-Aminolaevulinic acid methyl ester transport on amino acid carriers in a human colon adenocarcinoma cell line, Photochem. Photobiol., 2001, 73, 164–169 CrossRef CAS.
|
This journal is © The Royal Society of Chemistry and Owner Societies 2006 |
Click here to see how this site uses Cookies. View our privacy policy here.