Molecular iodine: a highly efficient catalyst in the synthesis of quinolines via Friedländer annulation
Received
17th October 2005
, Accepted 3rd November 2005
First published on 28th November 2005
Abstract
A mild and efficient route for the synthesis of quinolines and polycyclic quinolines via Friedländer annulation, utilizing molecular iodine (1 mol%) as a new catalyst, is described.
Introduction
Recently, molecular iodine has received considerable attention as an inexpensive, nontoxic, readily available catalyst for various organic transformations, affording the corresponding products in excellent yields with high selectivity. The mild Lewis acidity associated with iodine enhanced its usage in organic synthesis to realize several organic transformations using stoichiometric levels to catalytic amounts. Owing to numerous advantages associated with this eco-friendly element, iodine has been explored as a powerful catalyst for various organic transformations.1,2 For instance, it shows high efficiency in the Hantzsch reaction1a as well as the cyanation of imines.1b,1o Recently, we found that it was also efficient as a catalyst in the synthesis of quinolines and polycyclic quinolines via Friedländer annulation, which is disclosed herein.
As a privileged fragment, quinoline is a ubiquitous subunit in many quinoline-containing natural products with remarkable biological activities.3 Members of this family have wide applications in medicinal chemistry, being used as antimalarial, antiinflammatory, antiasthmatic, antibacterial, antihypertensive, and tyrosine kinase inhibiting agents.3 In addition, quinolines are valuable synthons, used for the preparation of nano- and mesostructures with enhanced electronic and photonic properties.4 Because of their importance as substructures in a broad range of natural and designed products, significant effort continues to be directed into the development of new quinoline-based structures5 and new methods for their construction.6
As part of a continuing effort in our laboratory toward the development of new methods for the expeditious synthesis of biologically relevant heterocyclic compounds,7 we became interested in the possibility of developing a novel and efficient method to construct the quinoline scaffold. Though some methods such as the Skraup, Doebner–von Miller, and Combes reactions8 are available, the protocol reported by Friedländer is one of the most simple and straightforward methods for the synthesis of polysubstituted quinolines. The Friedländer annulation, that is, a condensation followed by a cyclodehydration between 2-aminoaryl ketones and α-methylene ketones, is catalyzed by both acids and bases. Brønsted acids like sulfamic acid, hydrochloric acid, sulfuric acid, p-toluene sulfonic acid and phosphoric acid were widely used as catalysts.9 However, many of these methods require harsh reaction conditions and lead to several side reactions. Under base catalysis conditions, o-aminobenzophenone fails to react with simple ketones such as cyclohexanone and β-keto esters.9 Recently, Lewis acids such as FeCl3, Mg(ClO4)2, ZnCl2, SnCl2, Bi(OTf)3, Sc(OTf)3, silver phosphotungstate, sodium fluoride, and NaAuCl4·2H2O have been reported to be effective for the synthesis of quinolines.10 However, many of these procedures also suffered from harsh reaction conditions, low yields, difficulties in work-up, and the use of stoichiometric and/or relatively expensive reagents. And in some cases, high catalyst loading had to be employed in order to obtain respectable yields. Since quinoline derivatives are increasingly useful and important in pharmaceuticals and industry, the development of a simple, eco-benign, low cost protocol is still desirable.
Inspired by reports of continuation of interest in catalytic applications of elemental iodine for organic transformation,1,2 we considered employing molecular iodine as a catalyst for Friedländer quinoline synthesis since most of the iodine-catalyzed transformations are acid-induced processes. As Friedländer annulations are among the most important acid-mediated reactions, development of a reaction that uses catalytic amounts of low toxic, economic, readily available iodine should greatly contribute to the creation of environmentally benign processes.
Results and discussion
An initial study was performed by the treatment of 2-aminobenzophenone 1a with ethyl acetoacetate 2a in EtOH in the presence of a catalytic amount of I2 (10 mol%) at room temperature. To our delight, we observed the formation of ethyl-2-methyl-4-phenylquinoline-3-carboxylate 3a. Complete conversion and 96% isolated yield were obtained after 16 hours. Further studies established that 1 mol% of catalyst was also efficient in this reaction (1 mol%: 16 hours, 96% yield). Moreover, it is noteworthy that this reaction could be run under air without loss of efficiency (Scheme 1).
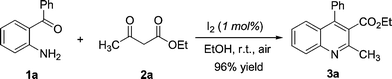 |
| Scheme 1 Reaction of 1a and 2a catalyzed by I2 (1 mol%) in EtOH at room temperature. | |
Among the solvents (EtOH, THF, H2O, CH3CN, toluene) screened, EtOH was demonstrated as the best solvent. Under solvent-free conditions, the reaction also proceeded smoothly to afford the corresponding product although the yield was slightly lower (89%). To demonstrate the generality of this method, we next investigated the scope of this reaction under the optimized conditions (EtOH, 1 mol% of iodine, r.t.) and the results are summarized in Table 1. As shown in Table 1, this method is equally effective for both cyclic and acyclic ketones. Various substituted 2-aminoaryl ketones 1 such as 2-aminobenzophenone, 2-aminoacetophenone, and 2-amino-5-chlorobenzophenone reacted smoothly with α-methylene ketones 2 to produce a range of quinoline derivatives. Complete conversion and good to excellent isolated yields were observed for all substrates employed. This reaction is very clean and free from side reactions, such as self-condensation of ketones, which are normally observed under basic conditions. Unlike reported methods, the present protocol does not require high temperature or drastic conditions to produce quinoline derivatives. In the absence of a catalyst, the reaction did not yield any product even after long reaction times. Interestingly, cyclic ketones such as cyclopentanone and cyclohexadione also underwent smooth condensation with 2-aminoaryl ketones to afford the respective tricyclic quinolines (for example: Table 1, entries 3 and 4).
Table 1 Molecular iodine-catalyzed Friedländer synthesis of quinolinesa
Conclusions
In conclusion, we describe a mild and efficient route for the synthesis of quinolines and polycyclic quinolines utilizing molecular iodine as a novel catalyst via Friedländer annulation. This method not only provides an excellent complement to quinoline synthesis via Friedländer annulation, but also avoids the use of hazardous acids or bases and harsh reaction conditions. The advantages of this method include good substrate generality, the use of inexpensive reagents and catalyst under mild conditions, and experimental operational ease. Reactions employing iodine as a catalyst for other organic transformations are currently under investigation in our research group, and will be reported in due course.
Experimental
General procedure
A mixture of the 2-aminoaryl ketone 1 (0.5 mmol), the α-methylene ketone 2 (0.6 mmol, 1.2 equiv.) and I2 (1 mol%) in EtOH (1.0 mL) was stirred at r.t. After completion of the reaction as indicated by TLC, the reaction mixture was quenched with water (15 mL) and extracted with EtOAc (2 × 10 mL). Evaporation of the solvent followed by purification on silica gel afforded pure quinoline. (All the products are known compounds. The characterizations of these compounds are identical with the literature reports.10)
3a: ethyl-2-methyl-4-phenylquinoline-3-carboxylate.
1H NMR (500 MHz, CDCl3): δ (ppm) 0.94 (t, J = 7.1 Hz, 3H), 2.79 (s, 3H), 4.04–4.09 (m, 2H), 7.36–7.72 (m, 8H), 8.07 (d, J = 8.4 Hz, 1H).
3c: 9-phenyl-2,3-dihydro-1H-cyclopenta[b]quinoline.
1H NMR (400 MHz, CDCl3): δ (ppm) 2.16–2.19 (m, 2H), 2.91 (t, J = 7.3 Hz, 2H), 3.25 (t, J = 7.6 Hz, 2H), 7.36–8.10 (m, 9H).
3d: 9-phenyl-3,4-dihydroacridin-1(2H)-one.
1H NMR (400 MHz, CDCl3): δ (ppm) 2.24–2.27 (m, 2H), 2.69 (t, J = 6.6 Hz, 2H), 3.36 (t, J = 6.4 Hz, 2H), 7.17–7.19 (m, 2H), 7.40–7.46 (m, 6H), 8.05 (d, J = 8.7 Hz, 1H).
3e: ethyl-2,4-dimethylquinoline-3-carboxylate.
1H NMR (500 MHz, CDCl3): δ (ppm) 1.41 (t, J = 7.1 Hz, 3H), 2.61 (s, 3H), 2.71 (s, 3H), 4.45–4.49 (m, 2H), 7.46 (t, J = 7.6 Hz, 1H), 7.66 (t, J = 7.6 Hz, 1H), 7.91 (d, J = 8.4 Hz, 1H), 7.99 (d, J = 8.4 Hz, 1H).
3f: 1-(2,4-dimethylquinolin-3-yl)ethanone.
1H NMR (500 MHz, CDCl3): δ (ppm) 2.54 (d, J = 12.3 Hz, 6H), 2.62 (s, 3H), 7.49 (t, J = 7.6 Hz, 1H), 7.66 (t, J = 7.6 Hz, 1H), 7.91 (d, J = 8.3 Hz, 1H), 7.99 (d, J = 8.4 Hz, 1H).
3g: ethyl-6-chloro-2-methyl-4-phenylquinoline-3-carboxylate.
1H NMR (500 MHz, CDCl3): δ (ppm) 0.94 (t, J = 7.1 Hz, 3H), 2.77 (s, 3H), 4.04–4.09 (m, 2H), 7.34–7.66 (m, 7H), 8.00 (d, J = 9.0 Hz, 1H).
3h: 1-(6-chloro-2-methyl-4-phenylquinolin-3-yl)ethanone.
1H NMR (500 MHz, CDCl3): δ (ppm) 2.00 (s, 3H), 2.68 (s, 3H), 7.33–7.35 (m, 2H), 7.53–7.66 (m, 5H), 8.00 (d, J = 8.9 Hz, 1H).
3i: 7-chloro-9-phenyl-2,3-dihydro-1H-cyclopenta[b]quinoline.
1H NMR (400 MHz, CDCl3): δ (ppm) 2.14–2.18 (m, 2H), 2.89 (t, J = 7.3 Hz, 2H), 3.21 (t, J = 7.8 Hz, 2H), 7.32–7.34 (m, 2H), 7.52–7.53 (m, 5H), 7.98 (d, J = 9.2 Hz, 1H).
3k: ethyl 6-chloro-4-(2-chlorophenyl)-2-methylquinoline-3-carboxylate.
1H NMR (400 MHz, CDCl3): δ (ppm) 0.95–0.98 (m, 3H), 2.81 (s, 3H), 4.06–4.08 (m, 2H), 7.27–7.55 (m, 6H), 8.02 (d, J = 9.3 Hz, 1H).
3l: 1-(6-chloro-4-(2-chlorophenyl)-2-methylquinolin-3-yl)ethanone.
1H NMR (400 MHz, CDCl3): δ (ppm) 2.15 (s, 3H), 2.71 (s, 3H), 7.23–7.28 (m, 2H), 7.40–7.67 (m, 4H), 8.01 (d, J = 9.2 Hz, 1H).
References
-
(a) S. Ko, M. N. V. Sastry, C. Lin and C.-F. Yao, Tetrahedron Lett., 2005, 46, 5771 CrossRef CAS;
(b) J. S. Yadav, B. V. S. Reddy, M. Srinivas and K. Sathaiah, Tetrahedron Lett., 2005, 46, 3489 CrossRef CAS;
(c) C.-M. Chu, S. Gao, M. N. V. Sastry and C.-F. Yao, Tetrahedron Lett., 2005, 46, 4971 CrossRef CAS;
(d) W.-Y. Chen and J. Lu, Synlett, 2005, 1337 CrossRef CAS;
(e) B. S. Lee, S. Mahajan and K. D. Janda, Synlett, 2005, 1325 CAS;
(f) M. C. Bagley, C. Glover and D. Chevis, Synlett, 2005, 649 CrossRef CAS;
(g) B. K. Banik, M. Fernandez and C. Alvarez, Tetrahedron Lett., 2005, 46, 2479 CrossRef CAS;
(h) B. K. Banik, M. Chapa, J. Marquez and M. Cardona, Tetrahedron Lett., 2005, 46, 2341 CrossRef CAS;
(i) P. D. Lokhande, S. S. Sakate, K. N. Taksande and B. Navghare, Tetrahedron Lett., 2005, 46, 1573 CrossRef CAS;
(j) P. Gogoi, P. Hazarika and D. Konwar, J. Org. Chem., 2005, 70, 1934 CrossRef CAS;
(k) J. Sun, Y. Dong, L. Cao, X. Wang, S. Wang and Y. Hu, J. Org. Chem., 2004, 69, 8932 CrossRef CAS;
(l) K. V. N. S. Srinivas and B. Das, Synthesis, 2004, 2091;
(m) R. S. Bhosale, S. V. Bhosale, T. Wang and P. K. Zubaidha, Tetrahedron Lett., 2004, 45, 7187 CrossRef CAS;
(n) B. P. Bandgar and S. V. Bettigeri, J. Chem. Res., 2004, 389 Search PubMed;
(o) L. Royer, S. K. De and R. A. Gibbs, Tetrahedron Lett., 2005, 46, 4595 CrossRef CAS.
-
(a) K. K. Rana, C. Guin, B. Banerjee and S. C. Roy, J. Indian Chem. Soc., 2003, 80, 1005 CAS;
(b) J. S. Yadav, B. V. S. Reddy, K. V. Rao, K. S. Raj, P. P. Rao, A. R. Prasad and D. Gunasekar, Tetrahedron Lett., 2004, 45, 6505 CrossRef CAS;
(c) P. Phukan, J. Org. Chem., 2004, 69, 4005 CrossRef CAS;
(d) B. P. Bandgar, S. V. Bettigeri and N. S. Joshi, Synth. Commun., 2004, 34, 1447 CrossRef CAS;
(e) X. Gang, Y. Zhu, H. Birch, H. A. Hjuler and N. J. Bjerrum, Appl. Catal., A, 2004, 261, 91 CrossRef CAS;
(f) P. Phukan, Synth. Commun., 2004, 34, 1065 CrossRef CAS;
(g) J. S. Yadav, B. V. S. Reddy and M. S. Reddy, Synlett, 2003, 1722 Search PubMed;
(h) B. Karimi and D. Zareyee, Synthesis, 2003, 1875 CrossRef CAS;
(i) C.-S. Liu, C.-F. Zhao and G.-X. Luo, Hecheng Huaxue, 2003, 11, 254 CAS;
(j) R. Saeeng, U. Sirion, P. Sahakitpichan and M. Isobe, Tetrahedron Lett., 2003, 44, 6211 CrossRef CAS;
(k) J. S. Yadav, B. V. S. Reddy, C. V. Rao and M. S. Reddy, Synthesis, 2003, 247 CrossRef CAS;
(l) B. P. Bandgar and K. A. Shaikh, Tetrahedron Lett., 2003, 44, 1959 CrossRef CAS;
(m) J. S. Yadav, B. V. S. Reddy, M. S. Reddy and A. R. Prasad, Tetrahedron Lett., 2002, 43, 9703 CrossRef CAS;
(n) J. S. Yadav, B. V. S. Reddy, K. Sadasiv and G. Satheesh, Tetrahedron Lett., 2002, 43, 9695 CrossRef CAS;
(o) J. S. Yadav, B. V. S. Reddy, C. V. Rao and K. V. Rao, J. Chem. Soc., Perkin Trans. 1, 2002, 1401 RSC;
(p) B. Karimi and B. Golshani, Synthesis, 2002, 784 CrossRef CAS;
(q) J. S. Yadav, P. K. Chand and S. Anjaneyulu, Tetrahedron Lett., 2002, 43, 3783 CrossRef CAS;
(r) M. K. Basu, S. Samajdar, F. F. Becker and B. K. Banik, Synlett, 2002, 319 CrossRef CAS;
(s) J. S. Yadav, B. V. S. Reddy, C. V. Rao, P. K. Chand and A. R. Prasad, Synlett, 2001, 1638 CrossRef CAS;
(t) S. Samajdar, M. K. Basu, F. F. Becker and B. K. Banik, Tetrahedron Lett., 2001, 42, 4425 CrossRef CAS;
(u) C. Mukhopadhyay, F. F. Becker and B. K. Banik, J. Chem. Res., Synop., 2001, 28 Search PubMed;
(v) N. Deka and J. C. Sarma, J. Org. Chem., 2001, 66, 1947 CrossRef CAS;
(w) H. M. S. Kumar, B. V. S. Reddy, E. J. Reddy and J. S. Yadav, Chem. Lett., 1999, 857 CrossRef CAS;
(x) K. M. Kim, D. J. Jeon and E. K. Ryu, Synthesis, 1998, 835 CrossRef CAS;
(y) N. Deka, D. J. Kalita, R. Borah and J. C. Sarma, J. Org. Chem., 1997, 62, 1563 CrossRef CAS.
-
(a) R. D. Larsen, E. G. Corley, A. O. King, J. D. Carrol, P. Davis, T. R. Verhoeven, P. J. Reider, M. Labelle, J. Y. Gauthier, Y. B. Xiang and R. Zamboni, J. Org. Chem., 1996, 61, 3398 CrossRef CAS;
(b) Y. L. Chen, K. C. Fang, J. Y. Sheu, S. L. Hsu and C. C. Tzeng, J. Med. Chem., 2001, 44, 2374 CrossRef CAS;
(c) G. Roma, M. D. Braccio, G. Grossi and M. Chia, Eur. J. Med. Chem., 2000, 35, 1021 CrossRef CAS;
(d) D. Doube, M. Bloun, C. Brideau, C. Chan, S. Desmarais, D. Eithier, J. P. Falgueyeret, R. W. Friesen, M. Girad, Y. Girad, J. Guay, P. Tagari and R. N. Yong, Bioorg. Med. Chem. Lett., 1998, 8, 1255 CrossRef CAS;
(e) M. P. Maguire, K. R. Sheets, K. Mcvety, A. P. Spada and A. Zilberstein, J. Med. Chem., 1994, 37, 2129 CrossRef CAS;
(f) O. Bilker, V. Lindo, M. Panico, A. E. Etiene, T. Paxton, A. Dell, M. Rogers, R. E. Sinden and H. R. Morris, Nature, 1998, 289.
-
(a) A. K. Aggarwal and S. A. Jenekhe, Macromolecules, 1991, 24, 6806 CrossRef CAS;
(b) X. Zhang, A. S. Shetty and S. A. Jenekhe, Macromolecules, 1999, 32, 7422 CrossRef CAS;
(c) S. A. Jenekhe, L. Lu and M. M. Alam, Macromolecules, 2001, 34, 7315 CrossRef CAS.
- M. Z. Hoemann, G. Kumaravel, R. L. Xie, R. F. Rossi, S. Meyer, A. Sidhu, G. D. Cuny and J. R. Hauske, Bioorg. Med. Chem. Lett., 2000, 10, 2675 CrossRef CAS.
- A subset of recent work in this area includes:
(a) W. Du and D. P. Curran, Org. Lett., 2003, 5, 1765 CrossRef CAS;
(b) D. M. Lindsay, W. Dohle, A. E. Jensen, F. Kopp and P. Knochel, Org. Lett., 2002, 4, 1819 CrossRef CAS;
(c) M. Matsugi, F. Tabusa and J. Minamikawa, Tetrahedron Lett., 2000, 41, 8523 CrossRef CAS;
(d) P. G. Dormer, K. K. Eng, R. N. Farr, G. R. Humphrey, J. C. McWilliams, P. J. Reider, J. W. Sager and R. P. Volante, J. Org. Chem., 2003, 68, 467 CrossRef CAS.
-
(a) J. Wu, L. Zhang and X. Sun, Chem. Lett., 2005, 34, 796 CrossRef CAS;
(b) J. Wu, X. Sun and L. Zhang, Chem. Lett., 2005, 34, 550 CrossRef CAS.
-
(a) C. S. Cho, B. H. Oh, J. S. Kim, T.-J. Kim and S. C. Shim, Chem. Commun., 2000, 1885 RSC;
(b) B. Jiang and Y. C. Si, J. Org. Chem., 2002, 67, 9449 CrossRef CAS;
(c) H. Skraup, Chem. Ber., 1880, 13, 2086 Search PubMed;
(d) P. Friedländer, Chem. Ber., 1882, 15, 2572 Search PubMed;
(e) R. H. Mansake and M. Kulka, Org. React., 1953, 7, 59;
(f) R. J. Linderman and S. K. Kirollos, Tetrahedron Lett., 1990, 31, 2689 CrossRef CAS;
(g) M. E. Theclitou and L. A. Robinson, Tetrahedron Lett., 2002, 43, 3907 CrossRef CAS.
- E. A. Fehnel, J. Heterocycl. Chem., 1966, 31, 2899 Search PubMed.
-
(a) L. Strekowski and A. Czamy, J. Fluorine Chem., 2000, 104, 281 CrossRef CAS;
(b) Y. Z. Hu, G. Zang and R. P. Thummel, Org. Lett., 2003, 5, 2251 CrossRef CAS;
(c) A. Arcadi, M. Chiarini, S. Di Giuseppe and F. Marinelli, Synlett, 2003, 203 CrossRef CAS;
(d) B. R. McNaughton and B. L. Miller, Org. Lett., 2003, 5, 4257 CrossRef CAS;
(e) J. S. Yadav, B. V. S. Reddy and K. Premlatha, Synlett, 2004, 963 CrossRef CAS;
(f) J. S. Yadav, B. V. S. Reddy, P. Sreedhar, R. S. Rao and K. Nagaiah, Synthesis, 2004, 2381 CrossRef CAS;
(g) K. Mogilaih and C. S. Reddy, Synth. Commun., 2003, 3131 CrossRef;
(h) A. Walser, T. Flyll and R. I. Fryer, J. Heterocycl. Chem., 1975, 12, 737 Search PubMed;
(i) S. K. De and R. A. Gibbs, Tetrahedron Lett., 2005, 46, 1647 CrossRef CAS;
(j) P. Arumugam, G. Karthikeyan, R. Atchudan, D. Muralidharan and P. T. Perumal, Chem. Lett., 2005, 34, 314 CrossRef CAS;
(k) J. Wu, L. Zhang and T.-N. Diao, Synlett, 2005, 2653 CrossRef CAS.
|
This journal is © The Royal Society of Chemistry 2006 |