DOI:
10.1039/B510677J
(Paper)
Green Chem., 2006,
8, 91-95
Cerium (IV) ammonium nitrate (CAN) as a catalyst in tap water: A simple, proficient and green approach for the synthesis of quinoxalines
Received
27th July 2005
, Accepted 18th October 2005
First published on 9th November 2005
Abstract
Various biologically important quinoxaline derivatives have been efficiently synthesized in excellent yields using catalytic amounts of cerium (IV) ammonium nitrate (CAN) in water. This inexpensive, nontoxic, and readily available catalytic system (5 mol%) in water efficiently catalyzes the condensation reaction of various 1,2-diketones and 1,2-diamines. Several aromatic as well as aliphatic 1,2-diketones and aromatic 1,2-diamines such as substituted phenylene diamines, and tetra amines were further subjected to condensation using catalytic amounts of CAN to yield the products in excellent yield. Besides this, ambient conditions, excellent product yields and water as a universal solvent display both economic and environmental advantages.
Introduction
The chemical industry is a major contributor to environmental pollution due to the ubiquitous use of several hazardous organic solvents, flammables, explosives, carcinogens, etc. The development of nonhazardous alternatives is not only due to the increased regulatory pressure focusing on organic solvents, but also of great importance with respect to green chemistry. However, performing organic reactions in water is one of the fundamental challenges to organic chemists. Water emerged as a useful alternative solvent for several organic reactions1 owing to many of its potential advantages such as safety, economy and environmental concern. Reactions in water have been very useful to the synthetic chemist for many years and their utility is reflected by the many studies to discover new processes with which they can be performed catalytically and stereoselectively.
Among lanthanide reagents, cerium (IV) ammonium nitrate (CAN) is the most notable one electron oxidant and has been utilized extensively for a broad variety of oxidative transformations in organic chemistry.2 Additionally, many advantages such as excellent solubility in water, inexpensiveness, eco-friendly nature, uncomplicated handling and high reactivity makes CAN a potent catalyst in organic syntheses. Recently, studies have also revealed protocols for mild conditions, fast conversions and convenient work-up procedures using CAN, to make it a powerful tool in chemistry under green and safer approaches. Besides this, CAN is able to catalyze various organic transformations not only based on the Lewis acidic property, but also with electron transfer capability. Quinoxaline derivatives are important components of several pharmacologically active compounds3 and a number of antibiotics such as Echinomycin, Leromycin and Actinomycin, which are known to inhibit the growth of Gram-positive bacteria and are also active against various transplantable tumors.4 Besides this, they are well known for their application in dyes,5 efficient electroluminescent materials,6 organic semiconductors,7 building blocks for the synthesis of anion receptors,8 cavitands,9 dehydroannulenes10 and DNA cleaving agents.11 A number of methods have been developed for the synthesis of substituted quinoxalines and the most common method is the condensation of an aryl 1,2-diamine with 1,2-dicarbonyl compounds in refluxing ethanol or acetic acid.12 In addition to the above catalytic methods, reports are also known using zeolites,13 microwave14 and solid phase synthesis.15 Nevertheless, most of these methods suffer from unsatisfactory product yields, critical product isolation procedures, expensive and detrimental metal precursors and harsh reaction conditions, which limit their use. As part of our ongoing program to develop more efficient and environmentally benign methods for organic syntheses using economic and ecofriendly materials as catalysts, we have looked into the synthesis of quinoxalines using catalytic amounts of CAN in tap water.
Results and discussion
In the beginning, efforts were directed towards the catalytic evaluation of CAN towards the synthesis of quinoxalines. Initially, a blank reaction was carried out using benzil and o-phenylenediamine in boiling ethanol, resulting in the formation of a condensation product after 45 min (50% Y). With similar substrates, using catalytic amounts of CAN either in CH3CN or in any protic solvent, afforded the products in quantitative yield during 20 min. Thus, in the absence of CAN, the reaction was slow and also requires harsh refluxing conditions with unsatisfactory yields. While screening different solvents, following the report by Xi et al.,2b we attempted the condensation of benzil and o-phenylenediamine using CAN in water at room temperature for the synthesis of quinoxalines. To our surprise, the reaction proceeded smoothly during 10 min and resulted in the formation of products in quantitative yield (Scheme 1).
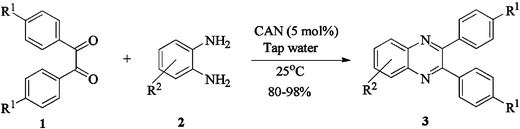 |
| Scheme 1 | |
The method of CAN catalyzed synthesis of quinoxalines is very simple, efficient, clean, and without any other side products. It was also observed during the reaction that the substrates were insoluble and do not react in the absence of CAN. By adding catalytic amounts of CAN, the reaction mixture turns into a clear reddish brown colored solution and the product precipitates out as a solid in most cases. In order to evaluate the amount of CAN required for this transformation, a couple of experiments were carried out using varying amounts of CAN. As less as 3 mol% of CAN is sufficient to catalyze the reaction to the same extent, but needs prolonged reaction times (>50 min). Conducting the reaction either by using 5 mol% or 10 mol% of CAN results in almost the same yield, without even affecting the reaction rate. Thus, under an optimized reaction condition, 1,2-diketone (1 mmol) and 1,2-diamine (1.2 mmol) in water (1.0 mL) were mixed with CAN (0.05 mmol) and stirred at room temperature for 10–50 min. After completion of the reaction, a simple filtration affords the products in good to excellent yield. In order to demonstrate the versatility of the CAN promoted synthesis of quinoxalines, a series of 1,2-diketones and 1,2-diamines were subjected to condensation using catalytic amounts of CAN (Table 1).With symmetrical aromatic diamines the reaction showed good product yields. In the case of diamines, such as naphthalene-2,3-diamine, steric factors played a key role in affecting the rate of reaction and the reaction requires a longer time. With electron donating substituents in the amine part, increased yields of products were obtained and the effect is the reverse with electron withdrawing substituents. On the other hand, electron donating substituents associated with aromatic 1,2-diketone decreased the product yields and with electron withdrawing groups the effect is opposite. However, the variations in the yields were very small and both substituted aromatic diamines such as 4-chloro and 3-methyl gave the condensed products in excellent yields with different substituted 1,2-diketones. To check the versatility of this method, we have also studied compounds other than symmetrical 1,2-diketones, such as furil and 1-phenyl-1,2-propanedione, and obtained the products in excellent yields. In the case of unsymmetrical 1,2-diketones such as 1-phenyl-1,2-propanedione, different aromatic diamines delivered a 1 ∶ 1 ratio of regioisomers in almost quantitative yield (3r and 3s). In another variation, tetra amines such as 3,3′,4,4′-tetraamino-1,1′-biphenyl 4 underwent condensation with diketone (benzil) in the presence of catalytic amounts of CAN (5 mol%) affording the product 5 in 80% yield (Scheme 2). This methodology is very useful for the synthesis of a sterically hindered amines such as 2,3,2′,3′-tetra phenyl [6,6′] biquinoxalinyl 5. Though the intimate mechanistic details of this condensation reaction are not yet fully understood, a feasible pathway might involve the complexation of cerium with the diketone by acting as a Lewis acid and also playing a complex role in promoting the dehydration steps.
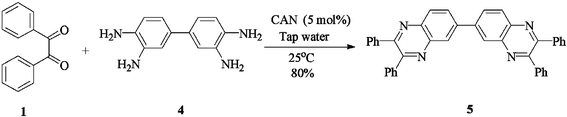 |
| Scheme 2 | |
Table 1 Synthesis of quinoxalines using different aromatic and heterocyclic 1,2-diketones
Entrya |
Productb |
|
Time/min |
Yield (%)c |
All reactions were performed on a 1 mmol scale using 5 mol% of CAN in 1 mL of water.
All products were well characterized using 1H-NMR, 13C-NMR and elemental analysis.
Isolated yields of pure products after passing through a small bed of silica.
The yield belongs to the overall yield of two regio isomers which are formed in 1 ∶ 1 ratio.
|
1 |
|
3a
|
10 |
98 |
2 |
|
3b
|
30 |
92 |
3 |
|
3c
|
10 |
90 |
4 |
|
3d
|
25 |
90 |
5 |
|
3e
|
10 |
94 |
6 |
|
3f
|
20 |
92 |
7 |
|
3g
|
30 |
90 |
8 |
|
3h
|
40 |
90 |
9 |
|
3i
|
10 |
88 |
10 |
|
3j
|
20 |
85 |
11 |
|
3k
|
30 |
82 |
12 |
|
3l
|
10 |
95 |
13 |
|
3m
|
15 |
93 |
14 |
|
3n
|
25 |
89 |
15 |
|
3o
|
50 |
87 |
16 |
|
3p
|
10 |
91 |
17 |
|
3q
|
50 |
80 |
18 |
|
3r
|
10 |
86d |
19 |
|
3s
|
30 |
83d |
Conclusion
In summary, we describe a simple, efficient, and ecofriendly method for the synthesis of quinoxalines from various 1,2-diketones and 1,2-diamines using cheap and readily available CAN as a catalyst. The ambient conditions, high reaction rates, excellent product yields and simple filtration, not only make this methodology an alternative platform to the conventional acid/base catalyzed thermal processes, but it also becomes significant under the umbrella of environmentally greener and safer processes. Moreover, water as a universal solvent used with CAN has both economic and environmental advantages, and the ability to further explore this efficient catalytic system to various organic syntheses.
Experimental
General
All chemicals were of research grade and were used as obtained from Aldrich or Acros. The reactions were carried out in a round-bottomed flask of 10 ml capacity at room temperature. Analytical thin layer chromatography was performed with E. Merck silica gel 60F glass plates and flash chromatography by use of E. Merck silica gel 60 (230–400 mesh). All melting points were determined on a MEL-TEMP II melting point apparatus and were uncorrected. 1H and 13C NMR spectra were recorded with a Bruker EX 400 FT NMR. All NMR data were obtained in CDCl3 solution and chemical shifts (δ) were given in ppm relative to TMS and are compared with the reported literature values. Elemental analyses were analyzed by HERAEUS VarioEL-III (for CHN).
General procedure for the synthesis of quinoxalines
A mixture containing 1,2-diketone 1 (1 mmol), 1,2-diamine 2 (1.2 mmol) and cerium (IV) ammonium nitrate (5 mol%) in water (1 mL) were stirred at room temperature for 10 min (monitored by TLC). After completion of the reaction, the crude product was filtered, washed with water (3 × 10 mL) and dried to obtain quinoxaline 3a in almost pure form (98% Y). In the case of liquid compounds, the crude reaction mixture was diluted with EtOAc (15 mL) and washed with water (3 × 10 mL). The organic layer was dried over anhydrous MgSO4, followed by the evaporation of the solvent to obtain the crude product, which was passed through a small plug of silica to obtain pure product.
White solid, (0.276 g, 98%); mp 126–127 °C; 1H NMR (CDCl3, 400 MHz): δ 8.20 (m, 2H), 7.76 (m, 2H), 7.56 (m, 4H), 7.35 (m, 6H); 13C NMR (CDCl3, 100 MHz): δ 153.50, 141.29, 139.15, 130.01, 129.93, 129.27, 128.87, 128.33; Anal. Calcd. for C20H14N2: C, 85.08; H, 5.00; N, 9.92. Found: C, 84.97; H, 4.94; N, 10.00.
2,3-Diphenyl-[g]quinoxaline (3b)
Yellow solid, mp 187–188 °C, 1H NMR (CDCl3, 400 MHz): δ 8.68 (s, 2H), 8.07 (m, 2H), 7.51 (m, 6H), 7.32 (m, 6H); 13C NMR (CDCl3, 100 MHz): δ 154.10, 138.98, 137.78, 133.92, 129.73, 128.90, 128.41, 128.14, 127.40, 126.61. Anal. Calcd. for C24H16N2: C, 86.72; H, 4.85; N, 8.43. Found: C, 86.60; H, 4.74; N, 8.30.
Pale yellow solid, mp 137 °C, 1H NMR (CDCl3, 400 MHz): δ 8.06 (d, J = 8.5 Hz, 1H), 7.94 (s, 1H), 7.55 (dd, J = 8.5, 1.4 Hz, 1H), 7.46 (dd, J = 8.1, 1.9 Hz, 4H), 7.14 (d, J = 7.9 Hz, 4H), 2.58 (s, 3H), 2.36 (s, 6H); 13C NMR (CDCl3, 100 MHz): δ 153.34, 152.61, 141.27, 140.11, 139.69, 138.60, 138.53, 136.64, 132.01, 129.85, 129.82, 128.98, 128.70, 128.03, 21.93, 21.40. Anal. Calcd. for C23H20N2: C, 85.15; H, 6.21; N, 8.63. Found: C, 84.97; H, 5.94; N, 8.52.
Yellow solid, mp 198 °C, 1H NMR (CDCl3, 400 MHz): δ 8.71 (s, 2H), 8.09 (m, 2H), 7.53 (m, 6H), 7.18 (d, J = 8 Hz, 4H), 2.39 (s, 6H); 13C NMR (CDCl3, 100 MHz): δ 154.25, 139.04, 138.03, 136.55, 133.97, 129.84, 129.01, 128.54, 127.42, 126.57, 21.45. Anal. Calcd. for C26H20N2: C, 86.64; H, 5.59; N, 7.77. Found: C, 85.95; H, 5.44; N, 8.01.
Pale yellow solid, mp 151 °C, 1H NMR (CDCl3, 400 MHz): δ 8.10 (d, J = 2.3 Hz, 1H), 8.04 (d, J = 8.9 Hz 1H), 7.64 (dd, J = 8.9, 2.2 Hz, 1H), 7.5 (m, 4H), 6.88 (d, J = 8.7 Hz, 4H), 3.82 (s, 6H); 13C NMR (CDCl3, 100 MHz): δ 160.45, 160.37, 153.82, 153.17, 141.33, 139.55, 135.14, 131.38, 131.33, 131.26, 130.47, 130.23, 127.89, 113.85, 55.34. Anal. Calcd. for C22H17ClN2O2: C, 70.12; H, 4.55; N, 7.43. Found: C, 69.62; H, 4.14; N, 7.10.
2,3-difuran-2-yl-quinoxaline(3l)
Pale brown solid, mp 131 °C, 1H NMR (CDCl3, 400 MHz): δ 8.07 (m, 2H), 7.66 (m, 2H), 7.56 (d, J = 1.6 Hz, 2H), 6.6 (d, J = 3.4 Hz, 2H), 6.48 (m, 2H); 13C NMR (CDCl3, 100 MHz): δ 150.81, 144.19, 142.57, 140.57, 130.35, 129.07, 113.01, 111.92. Anal. Calcd. for C16H10N2O2: C, 73.27; H, 3.84; N, 10.68. Found: C, 72.97; H, 3.24; N, 10.23.
Dark brown solid, mp 157 °C, 1H NMR (CDCl3, 400 MHz): δ 8.59 (s, 2H), 7.98 (m, 2H), 7.60 (s, 2H), 7.45 (m, 2H), 6.67 (d, J = 3.3 Hz, 2H), 6.52 (m, 2H); 13C NMR (CDCl3, 100 MHz): δ 151.00, 144.43, 143.07, 137.05, 134.20, 128.50, 127.46, 126.90, 113.54, 112.00. Anal. Calcd. for C20H12N2O2: C, 76.91; H, 3.87; N, 8.97. Found: C, 76.47; H, 3.34; N, 8.25.
Orange solid, mp 56 °C, 1H NMR (CDCl3, 400 MHz): δ 8.13–8.02 (m, 2H), 7.75–7.60 (m, 4H), 7.57–7.47 (m, 3H), 2.77 (s, 3H); 13C NMR (CDCl3, 100 MHz): δ 154.40, 152.35, 140.75, 141.07, 138.85, 129.59, 129.08, 128.85, 128.79, 128.42, 128.14, 24.31. Anal. Calcd. for C15H12N2: C, 81.79; H, 5.49; N, 12.72. Found: C, 81.37; H, 5.24; N, 12.31.
2-Methyl-3-phenyl-benzo[g]quinoxaline (3q)
Yellow solid, mp 134 °C, 1H NMR (CDCl3, 400 MHz): δ 8.66 (d, 2H), 8.10 (m, 2H), 7.71 (m, 2H), 7.56 (m, 5H) 2.80 (s, 3H); 13C NMR (CDCl3, 100 MHz): δ 155.73, 153.47, 139.07, 137.95, 137.92, 133.88, 133.58, 129.21, 128.94, 128.62, 128.53, 128.46, 127.68, 126.70, 126.47, 126.38, 25.01. Anal. Calcd. for C19H14N2: C, 84.42; H, 5.22; N, 10.36. Found: C, 84.07; H, 5.02; N, 10.13.
2,6-Dimethyl-3-phenyl-quinoxaline (3r)
Yellow viscous oil, 1H NMR (CDCl3, 400 MHz): δ 7.96–7.79 (m, 4H), 7.67–7.55 (m, 4H), 7.54–7.43 (m, 8H), 2.70 (s, 6H), 2.52 (d, 6H); 13C NMR (CDCl3, 100 MHz): δ 154.52, 153.84, 152.00, 151.16, 141.12, 140.91, 139.93, 139.42, 139.33, 139.25, 138.86, 131.70, 131.20, 128.68, 128.59, 128.55, 128.42, 128.21, 127.77, 127.51, 126.89, 24.11, 24.03, 21.67, 21.57. Anal. Calcd. for C16H14N2: C, 82.02; H, 6.02; N, 11.96. Found: C, 81.95; H, 5.94; N, 11.85.
6-Chloro-3-methly-2-phenyl-quinoxaline (3s)
Orange solid, mp 80 °C, 1H NMR (CDCl3, 400 MHz): δ 8.10–7.95 (m, 4H), 7.75–7.58 (m, 6H), 7.57–7.47 (m, 6H), 2.75 (s, 6H); 13C NMR (CDCl3, 100 MHz): δ 155.52, 154.84, 153.47, 152.66, 141.32, 141.11, 139.53, 139.32, 138.48, 138.43, 135.22, 134.69, 130.52, 130.30, 130.07, 129.45, 129.12, 129.05, 128.79, 128.47, 127.96, 127.19, 24.39, 24.35. Anal. Calcd. for C15H11ClN2: C, 70.73; H, 4.35; N, 11.00. Found: C, 69.97; H, 4.04; N, 10.83.
2,3,2′,3′-Tetraphenyl-[6,6′] biquinoxalinyl (5)
Pale yellow solid, mp > 295 °C, 1H NMR (CDCl3, 400 MHz): δ 8.6 (s, 2H), 8.33 (d, J = 8.6 Hz, 2H), 8.20 (dd, J = 8.7, 1.7 Hz, 2H), 7.58 (m, 8H), 7.41 (m, 12H); 13C NMR (CDCl3, 100 MHz): δ 154.19, 153.75, 141.48, 141.28, 140.98, 139.00, 129.98, 129.92, 129.55, 129.01, 128.35, 127.50. Anal. Calcd. for C40H26N4: C, 85.38; H, 4.66; N, 9.96. Found: C, 84.91; H, 4.54; N, 9.50.
Acknowledgements
Financial support of this work by the National Science Council of the Republic of China and National Taiwan Normal University (ORD93-C) is gratefully acknowledged.
References
-
(a)
C. J. Li and T. H. Chan, Organic Reactions in Aqueous Mediam, John Wiley & Sons, New York, 1997 Search PubMed;
(b)
P. T. Anastas and J. C. Warner, Green Chemistry: Theory and Practice, Oxford University Press, Oxford, 1998 Search PubMed;
(c) U. M. Lindstrom, Chem. Rev., 2002, 102, 2751 CrossRef;
(d) C. J. Li, Chem. Rev., 2005, 105, 3095 CrossRef CAS;
(e)
A. Lubineau and J. Auge, in Topics in Current Chemistry, Springer, Berlin, Heidelberg, 1999, vol. 206, p. 1 Search PubMed.
-
(a) V. Nair, T. D. Suja and M. Kishor, Tetrahedron Lett., 2005, 46, 3217 CrossRef CAS;
(b) C. Xi, Y. Jing and X. Yang, Tetrahedron Lett., 2005, 46, 3909 CrossRef CAS;
(c) V. Nair, L. balagopal, R. Rajan and J. Mathew, Acc. Chem. Res., 2004, 37, 21 CrossRef CAS;
(d) V. Nair, J. Mathew and Prabhakaran, J. Chem. Soc. Rev., 1997, 127 Search PubMed;
(e) J. R. Hwu and K. Y. King, Curr. Sci., 2001, 8, 1043;
(f) T. L. Ho, Synthesis, 1973, 354;
(g)
T. L. Ho, Organic Synthesis by Oxidation with Metal Compounds, Plenum, New York, 1986 Search PubMed;
(h)
T. Imamoto, Lanthanide Reagents in Organic synthesis, Academic, Londan, 1994, p. 119 Search PubMed.
-
(a) M. M. Ali, M. M. F. Ismail, M. S. A. EI-Gabby, M. A. Zahran and T. A. Ammar, Molecules, 2000, 5, 864 Search PubMed;
(b) R. Sarges, H. R. Howard, R. C. Browne, L. A. Label and P. A. Seymour, J. Med. Chem., 1990, 33, 2240 CrossRef CAS;
(c) G. Sakata, K. Makino and Y. Kurasawa, Heterocycles, 1998, 27, 2481;
(d) G. Arthur, K. B. Elor, G. S. Robert, Z. Z. Guo, J. P. Richard, D. Stanley, R. K. John and T. Sean, J. Med. Chem., 2005, 48, 744 CrossRef;
(e) E. S. Lainne, J. S. William and C. R. Robert, J. Med. Chem., 2002, 45, 5604 CrossRef CAS;
(f) J. Andres, Z. Belen, A. Ibnacio and M. Antonio, J. Med. Chem., 2005, 48, 2019 CrossRef.
-
(a) A. Dell, D. H. William, H. R. Morris, G. A. Smith, J. Feeney and G. C. K. Roberts, J. Am. Chem. Soc., 1975, 97, 2497 CrossRef CAS;
(b) C. Bailly, S. Echepare, F. Gago and M. Waring, Anti-Cancer Drug Des., 1999, 15, 291;
(c) S. Sato, O. Shiratori and K. Katagiri, J. Antibiot., 1967, 20, 270.
- E. D. Brock, D. M. Lewis, T. I. Yousaf and H. H. Harper, (The Procter and Gamble Company, USA) WO 9951688, 1999 Search PubMed.
- K. R. Justin Thomas, V. Marappan, T. L. Jiann, C. Chang-Hao and T. Yu-ai, Chem. Mater., 2005, 17, 1860 CrossRef CAS.
-
(a) S. Dailey, J. W. Feast, R. J. Peace, R. C. Saga, S. Till and E. L. Wood, J. Mater. Chem., 2001, 11, 2238 RSC;
(b) D. O'Brien, M. S. Weaver, D. G. Lidzey and D. D. C. Bradley, Appl. Phys. Lett., 1996, 69, 881 CrossRef.
- L. S. Jonathan, M. Hiromitsu, M. Toshihisa, M. L. Vincent and F. Hiroyuki, Chem. Commun., 2002, 862 RSC.
-
(a) L. S. Jonathan, M. Hiromitsu, M. Toshihisa, M. L. Vincent and F. Hiroyuki, J. Am. Chem. Soc., 2002, 124, 13474 CrossRef CAS;
(b) P. C. Peter, Z. Gang, A. M. Grace, H. Carlos and M. G. T. Linda, Org. Lett., 2004, 6, 333 CrossRef CAS.
- O. Sascha and F. Rudiger, Synlett, 2004, 1509.
-
(a) T. Kazunobu, T. Ryusuke, O. Tomohiro and M. Shuichi, Chem. Commun., 2002, 212 RSC;
(b) L. S. Hegedus, M. G. Marc, J. W. Jory and P. B. Joseph, J. Org. Chem., 2003, 68, 4179 CrossRef CAS.
-
(a)
VOGEL's Textbook of Practical Organic Chemistry, 5th edn, 1989, p. 1190 Search PubMed;
(b)
D. J. Brown, Quinoxalines: supplements II, in The Chemistry of Heterocyclic Compounds, ed. E. C. Taylor and P. Wipf, John Wiley and Sons, New Jersey, 2004 Search PubMed.
-
(a) A. Sylvain and D. Elisabet, Tetrahedron Lett., 2002, 43, 3971 CrossRef;
(b) B. Jose, A. Fernando, L. Ramon and C. Maria-Paz, Synthesis, 1985, 313;
(c) A. R. Steven, D. W. Cecilia and J. K. T. Richard, Chem. Commun., 2003, 2286 RSC;
(d) G. Shyamaprosad and K. A. Avijit, Tetrahedron Lett., 2005, 46, 221 CrossRef;
(e) C. Yoram, Y. M. Amatzya and R. Mordecai, J. Am. Chem. Soc., 1986, 108, 7044;
(f) N. P. Xekoukoulotakis, M. Hadjiantoniou and A.J. Maroulis, Tetrahedron Lett., 2000, 41, 10299 CrossRef CAS;
(g) D. Venugopal and M. Subrahmanmyam, Catal. Commun., 2001, 2, 219 CrossRef.
-
(a) G. Shyamaprosad and K. A Avijit, Tetrahedron Lett., 2002, 43, 8371 CrossRef;
(b) Z. Zhijian, D. W. David, E. W. Scoot, H. L. William and W. L. Craig, Tetrahedron Lett., 2004, 45, 4873 CrossRef.
-
(a) W. Zemin and J. E. Nicholas, Tetrahedron Lett., 2001, 42, 8115 CrossRef;
(b) A. A. Orazio, D. C. Lucia, F. Paolino, M. Fabio and S. Stefania, Synlett, 2003, 8, 1183;
(c) K. S. Sanjay, G. Priya, D. Srinavas and K. Bijoy, Synlett, 2003, 14, 2147.
|
This journal is © The Royal Society of Chemistry 2006 |