Monitoring urban heavy metal pollution using the House Sparrow (Passer domesticus)
Received
26th July 2005
, Accepted 14th October 2005
First published on 9th November 2005
Abstract
The House Sparrow (Passer domesticus) is one of the most successful birds in the urban environment, and has a global distribution. The present study aims to provide baseline data about metals in sparrows from urban environments in the West Bank and to investigate the possibility of using the House Sparrow to monitor metal pollution in urban environments. Concentrations of Cu, Cd, Pb and Zn were measured in different tissues and organs of male and female juvenile (1–4 weeks old) and adult House Sparrows from the West Bank. Tissues and organs had the following order of metal richness: liver > stomach > bone > lung, feathers > muscles > egg contents, brain > heart > egg shell. Significant correlation coefficients were observed for the concentrations of Cu, Pb, and Zn in the egg shell and for the egg contents. Male and female Sparrows showed no significant differences in their metal concentrations. Adult Sparrows collected from rural areas were found to have significantly less Cu, Pb, and Zn (but not Cd) concentrations than those from urban environments. In order to investigate metal accumulation with age, metal concentrations were plotted against age stage (egg, 1-, 2-, 3-, and 4-week-old juveniles and adults). Significant relationships were observed between age stage and Cu, Pb, and Zn concentrations. The results provide some evidence for the potential of the House Sparrow as a biomonitor for urban heavy metal pollution. However, further issues regarding metal physiological regulation and the correlations between metals in the environment and those in tissues of the House Sparrows have to be addressed before recommending this bird as a biomonitor for urban metal pollution.
1. Introduction
Large quantities of pollutants have continuously been introduced into ecosystems as a consequence of urbanization and industrial processes. Metals are persistent pollutants that can be biomagnified in the food chains, becoming increasingly dangerous to human and wildlife.1 This has led to the development of monitoring schemes aimed at directly measuring levels of contaminants in various organisms, and biomonitoring schemes that use indicator species to estimate the levels in other parts of the ecosystem.2.
Therefore, assessing pollutants in different components of the ecosystem has become an important task in preventing risk to natural life and public health.3 Analyzing pollutants in living organisms is more attractive and promising than analyzing pollutants of the abiotic environment, as living organisms provide precise information about the bioavailability of pollutants and the magnification and bio-transference of pollutants.4
Earlier studies have led to an increased interest in the use of birds as monitors of geographical, historical and global patterns of heavy metal pollution in the environment, as they occupy a wide range of trophic levels in different food chains.5–9 Birds are easier to study and monitor than other bioindicators, making them ideal for monitoring purposes.10–12 In addition, metals in bird nestlings, like Sparrows, reflect local pollution levels, as nestlings are fed entirely on food collected by parents near their breeding colony.5
Birds, like other organisms, are harmed by heavy metals. For example, metals were found to suppress the immune system of birds,13 increase aggressive behavior in territorial song birds,14 cause reproductive dysfunction, increased susceptibility to diseases and stress and changes in behavioral patterns.15,16 High lead levels in the urban-dwelling Pigeon (Columba livia) were observed in specimens from London.17 Several symptoms of lead intoxication were detected, mainly in specimens with the largest lead burden. Symptoms included increased kidney weight, altered mitochondrial structure and function, and the presence of renal intranuclear inclusion bodies.
In urban environments, feral Pigeons (Columba livia) were suggested as a biomonitor for urban Pb contamination and as a model for chronic lead toxicity.18–21 Another common urban-dwelling bird, which has not received as much attention as the feral Pigeon, is the House Sparrow. This is reflected by the limited number of studies investigating this bird.3,22,23
The House Sparrow is a non-migratory sedentary bird that is primarily associated with urban environments. It inhabits houses, farms, villages, industrial facilities, etc. Its ecological niche is characterized by the interaction with anthropogenic structures and the incorporation of different rubbish substances.3 Moreover, the bird has a global distribution, is not endangered, seems to be tolerant of urban environmental stress, and has a high reproductive rate. These characteristics make the House Sparrow one of the most suitable candidates for urban biomonitoring of heavy metals.
In the West Bank, studies investigating heavy metals in urban birds are completely lacking. Consequently, the present study aims at establishing baseline data about levels of metals in an urban-dwelling bird (the House Sparrow) from the West Bank, and to draw attention to the possibility of using it as a possible biomonitor for urban heavy metal pollution.
2. Materials and methods
2.1. Sample collection and preparation
In 2002–2003, a total of 40 House Sparrows of different ages were collected (under license from Palestinian Ministry for Environmental Affairs, MEnA) from urban (two cities: Qalqilia and Ramallah) and rural (two villages: Birzeit and Immatin) regions in the West Bank. Specimens had the following distribution: 20 adults (10 urban and 10 rural, each consisting of 5 males and 5 females), and 20 juveniles (5 specimens from each of the four juvenile age stages). Adult birds were caught by cage traps. Nests with eggs were visited regularly to determine the hatching date. Juveniles from each desired age group were then collected by hand from these nests. In addition, 10 fresh-laid eggs were collected from nests in the same regions. Birds were sacrificed, put in plastic bags, and deep-frozen for later analysis. Eggs were kept in the refrigerator (4 °C) until analysis. Thereafter, birds were dissected and specimens of following tissues were obtained: breast feathers and muscles, liver, lungs, heart, brain, stomach (with contents) and bones (femur and tibia fibula).
To remove loosely adhering external contaminants, feathers were washed thoroughly with acetone and then with doubly distilled water.24 All specimens were transferred into clean, acid-washed glass vials, and oven-dried at 60 °C until constant weights were obtained.
2.2. Analytical procedure
Specimens were digested in a mixture of super-pure nitric and perchloric acids (Merck) (2 ∶ 1, v/v). The volume of the digestion mixture was 10 times the sample’s mass in g. After soaking the specimens in the acid mixture overnight at room temperature, the mixture was gradually heated to 200 °C in a sand bath over a period of 3 h. Digestion was then continued until no fumes were observed and the mixture became pale yellow.23,25 Mixtures were then diluted to 25 mL with doubly distilled water. Blanks and reference material (Copepod homogenate, MA-A-1 TM, IAEA) were run with the samples. Finally, concentrations of Cu, Cd, Pb, and Zn were measured by inductively coupled plasma – atomic emission spectrophotometry (ICP Optima 3000, Perkin–Elmer, USA). Certified values of the reference material and the percent recoveries obtained are shown in Table 1.
Table 1 Mean certified values (± standard error), and the range of percent recoveries obtained for metals in the reference material copepod homogenate, MA-A-1 TM, IAEA
Metal |
Mean certified value/μg g−1 |
Recovery (%) |
Cu |
7.6 ± 0.2 |
95–103 |
Cd |
0.75 ± 0.03 |
91–98 |
Pb |
2.1 ± 0.3 |
94–101 |
Zn |
158 ± 2 |
93–105 |
2.2. Statistical analysis
Statistical analysis of the data was carried out using SYSTAT for Windows software (SYSTAT for Windows Inc.26). Differences were considered significant where P was ≤0.05. Data were tested for normality using the Shapiro–Wilk Normality Test before ANOVA was applied. The Tukey Test was used to determine which pairs of means differed significantly. The T-test was applied to check for differences between specimens from rural and urban environments and between males and females. Pearson’s correlation was applied to inspect correlations between metals in egg shell and egg contents.
3. Results and discussion
3.1. Metals in tissues and eggs
Some descriptive statistics of the concentrations of metals in tissues and eggs of the House Sparrows collected from an urban environment in the West Bank are shown in Table 2. Different tissues and eggs showed a great variation in mean metal concentration. Concentrations of Zn were the highest in all tissues analyzed, and those of Cd were clearly the lowest. Mean Cu concentration in tissues ranged between 0.81 μg g−1 (bones) to 39.2 μg g−1 (liver). Mean Cd concentrations ranged between 0.01 μg g−1 (egg shell) and 0.07 μg g−1 (liver). Concentrations of Pb were between 1.41 μg g−1 (muscles) to 34.2 μg g−1 (liver) while concentrations of Zn ranged between 19.6 μg g−1 (heart) to 150 μg g−1 (bones). In general, the concentrations of Zn and Cu were always higher than those of Cd and Pb. This might be because the former two metals are essential, having a biological role, while the latter are non-essential metals that have no biological function.3 The levels of Pb were much higher than Cd in all organs, reflecting its abundance as an urban pollutant (leaded fuel was still used in the Palestinian Territories at the time of the study). The low concentrations of Cd in different tissues could be due to the lack of heavy industry in the Palestinian regions. Similarly low concentrations of Cd in roadside plants (0.050–0.017 μg g−1) and soil (0.09–0.59 μg g−1) were observed in previous studies from the same region.25 In addition, Jaradat and Momani27 found that levels of Cd in roadside plants and dust from neighboring Jordan were below detection limits of the flame atomic absorption spectrophotometer.
Table 2 Descriptive statistics of trace metal concentrations in tissues and eggs of adult House Sparrows (Passer domesticus) from urban regions in the West Bank. Values represent means of 10 samples ± standard error. Minimum and maximum values are shown in parentheses
Organ |
Metal concentration/μg g−1 dry weight |
Cu |
Cd |
Pb |
Zn |
Bone |
0.8 ± 0.1 |
0.03 ± 0.00 |
14.3 ± 1.3 |
150.4 ± 8.6 |
(0.48–1.2) |
(0.02–0.04) |
(10.0–17.2) |
(128.0–175.0) |
Brain |
9.8 ± 1.0 |
0.02 ± 0.00 |
2.4 ± 0.3 |
48.2 ± 2.1 |
(7.0–12.0) |
(0.01–0.03) |
(1.7–3.8) |
(40.0–52.0) |
Egg contents |
4.3 ± 0.5 |
0.02 ± 0.00 |
1.6 ± 0.2 |
62.0 ± 2.6 |
(2.5–5.6) |
(0.01–0.03) |
(1.1–2.0) |
(55.0–70.0) |
Egg shell |
1.0 ± 0.1 |
0.01 ± 0.00 |
3.3 ± 0.6 |
20.0 ± 1.9 |
(0.9–1.2) |
(0.01–0.02) |
(1.5–4.8) |
(14.3–25.5) |
Feathers |
19.5 ± 1.6 |
0.02 ± 0.00 |
8.1 ± 1.3 |
54.9 ± 5.3 |
(15.4–24.7) |
(0.01–0.03) |
(4.6–11.1) |
(38.0–67.0) |
Heart |
3.1 ± 0.6 |
0.02 ± 0.00 |
1.9 ± 0.2 |
19.6 ± 1.5 |
(1.3–4.8) |
(0.01–0.03) |
(1.4–2.5) |
(16.0–25.0) |
Liver |
39.2 ± 6.9 |
0. 07 ± 0.01 |
34.2 ± 6.2 |
131.4 ± 14.3 |
(22.0–55.0) |
(0.06–0.08) |
(11.8–46.0) |
(93.0–165.0) |
Lung |
1.9 ± 0.3 |
0.03 ± 0.01 |
4.9 ± 0.9 |
70.4 ± 1.6 |
(1.1–2.8) |
(0.02–0.05) |
(2.5–7.8) |
(66.0–75.0) |
Muscle |
15.8 ± 1.1 |
0.03 ± 0.01 |
1.4 ± 0.2 |
61.9 ± 6.5 |
(13.8–19.0) |
(0.01–0.05) |
(1.1–1.8) |
(38.9–77.5) |
Stomach |
22.8 ± 3.8 |
0.04 ± 0.00 |
4.7 ± 0.7 |
109.1 ± 14.7 |
(12.5–35.5) |
(0.03–0.06) |
(3.1–6.8) |
(68.0–155.0) |
Results of the present study were compared to other studies found in the literature for sparrows (Table 3). Cd concentrations in the present study were lower than those reported for Sparrows from other regions. On the other hand, concentrations of Pb were clearly higher than those reported in the literature, possibly due to the banning of leaded fuel in industrialized countries some time ago (up the end of the study, leaded fuel was still used in the Palestinian Territories). The concentrations of the essential metals, Cu and Zn, were comparable to those found in the literature.
Table 3 Mean concentrations of metals in livers of sparrows according to different studies found in literature, compared to the present study
Region |
Metal concentration/μg g−1 dry weight |
Cu |
Cd |
Pb |
Zn |
Ref. |
Rural |
61.8 |
0.48 |
2.7 |
154 |
3
|
Urban |
42.0 |
1.31 |
3.7 |
205 |
3
|
Industrial |
17.2 |
0.24 |
— |
107 |
23
|
Industrial |
25.7 |
0.12 |
— |
137 |
23
|
Non-industrial |
15.5 |
0.24 |
— |
104 |
23
|
Urban |
27.0 |
1.01 |
— |
172 |
22
|
Rural |
27.0 |
0.07 |
18.0 |
97 |
Present study |
Urban |
38.2 |
0.07 |
37.6 |
121 |
Present study |
When ranked according to metal richness (Table 4), tissues and eggs showed a variable capacity for metal accumulation. This is clearly expressed by the high total rank score (TRS) of the liver (39) and the low one of the egg shell (10). Tissues and organs had the following order of metal richness: liver > stomach > bone > lung, feathers > muscles > egg contents, brain > heart > egg shell. The liver is the site of detoxification in the body; this explains its high metal content.
Table 4 Tissues and eggs of adult House Sparrows (Passer domesticus) from urban regions in the West Bank ranked according to their richness in metals. TRS: total rank score
Organ |
Cu |
Cd |
Pb |
Zn |
TRS |
Bone |
1 |
8 |
9 |
10 |
28 |
Brain |
6 |
5 |
4 |
3 |
18 |
Egg contents |
5 |
5 |
2 |
6 |
18 |
Egg shell |
2 |
1 |
5 |
2 |
10 |
Feathers |
8 |
5 |
8 |
4 |
25 |
Heart |
4 |
5 |
3 |
1 |
13 |
Liver |
10 |
10 |
10 |
9 |
39 |
Lung |
3 |
8 |
7 |
7 |
25 |
Muscle |
7 |
8 |
1 |
5 |
21 |
Stomach |
9 |
9 |
6 |
8 |
32 |
Gragnaniello et al.3 observed high concentrations of metals in House Sparrows from urban environments. Chao et al.23 found that concentrations of metals in Tree Sparrows (Passer montanus) were high in liver and bones, and low in muscles. These results are in agreement with this study. The high metal concentration in the stomach may be because it was analyzed with its contents. This indicates the importance of food as a source of metals. According to Burger and Gochfeld,28 avian exposure occurs from three sources: feeding (the major source), inhalation, and maternal metals deposited in eggs. Egg contents did not exhibit abnormal metal levels, indicating that House Sparrows do not sequester significant amounts of heavy metals in their eggs. Egg shell, on the other hand, contained twice as much Pb as egg contents, but less Cd, Cu and Zn. Walsh29 found that Pb transfer to eggs is low. Dauwe et al.30 found that egg contents from a polluted site had higher concentrations of the essential metals, Cu and Zn, than egg shell, but lower concentrations of Pb and Cd. These findings are in agreement with the results of the present study. According to Morera et al.,31 Cu and Zn are carried by vitellogenin from the liver to maturing oocytes during egg formation. In egg white, ovalbumin and conalbumin bind both Cu and Zn (Richards and Steel32).
Lungs and feathers occupied the fourth and fifth ranks in metal richness, respectively. The high rank occupied by the lungs could reflect atmospheric pollution in the urban environment. Use of feathers as biomonitors of heavy metal contamination has been recommended by many researchers.9,33–36 Metals in feathers reflect environmental contamination during the short period of feather growth but not necessarily body burden.37 However, feathers have been promoted extensively as a non-lethal and non-invasive indicator.34 In the present study, feathers were found to occupy the fifth rank in metal richness, which makes them good indicators for metals.
3.2. Metals in egg content and egg shell
Significant correlations (p < 0.05) between the concentrations of Cu, Pb and Zn in egg shell and egg contents were observed. Correlation coefficients were 0.95, 0.63, and 0.92 for Cu, Pb, and Zn, respectively. Cd did not exhibit a significant correlation. This indicates that levels of Cu, Pb, and Zn in the shell reflect their levels in the egg contents. In other words, shell can be used as an indicator for these metals in the egg content. From the correlation coefficients, it is clear that the shell reflects the concentration of the Cu and Zn better than Pb. According to Dauwe et al.,30 egg shell can be used as an indicator for metal pollution, especially in contaminated sites.
3.3. Metals in sparrows from urban and rural regions
In order to check the ability of the House Sparrow to reflect environmental differences in metal contamination, adult sparrows were collected from rural areas in addition to those from urban areas. Livers of Sparrows from urban areas were found to have significantly higher Cu, Pb, and Zn concentrations than those from rural regions (Table 5), indicating bioaccumulation of these metals in Sparrows from urban environments. Cd concentrations in specimens from urban and rural habitats did not show any significant difference. In a previous study, Swaileh et al.25 did not observe any elevated levels of Cd in roadside soil and vegetation from the West Bank. This means that, so far, Cd might not be an important pollutant in the Palestinian Territories. In addition, Jaradat and Momani27 found that levels of Cd in roadside plants and dust from neighboring Jordan were below detection limits of the flame atomic absorption spectrophotometer, which indicates that Cd is not a significant pollutant in the region. This is due to a lack of significant heavy industry. Generally, these results provide some early indications about the possibility of using the House Sparrow as a bioindicator for urban metal pollution. However, the diverse feeding habit of this bird must be taken into consideration when drawing conclusions concerning metal pollution relevant to human health.
Table 5 Concentrations of metals in the liver of adult House Sparrows (Passer domesticus) collected from two regions in the West Bank. Values represent means of 10 samples ± standard error
Region |
Metal concentration/μg g−1 dry weight |
Cu |
Cd |
Pb |
Zn |
Urban |
39.2 ± 6.9 |
0.07 ± 0.00 |
34.2 ± 6.2 |
131.4 ± 14.3 |
Rural |
27.0 ± 2.7 |
0.07 ± 0.00 |
18.0 ± 1.6 |
97.0 ± 3.7 |
P (t-test) |
0.039 |
0.957 |
0.005 |
0.029 |
3.4. Metals in males and females
No statistical differences between metals in the livers of male and female specimens were observed (Table 6). According to Donaldson and Braune,38 the principal reason for differences in metals between male and female birds is the ability of the female to deposit metals into eggs and the physiological differences with regard to some metals between males and females. He concluded that Cd and Pb are not readily transferred to eggs; therefore, they are not expected to differ between males and females. Hutton and Goodman20 found that female Pigeons (Columba livia) from London had higher bone lead levels than males. Similar results were observed by Janiga and Žemberyová.19
Table 6 Concentrations of metals in the livers of adult male and female House Sparrows (Passer domesticus) collected from urban environments in the West Bank. Values represent means ± standard error. Minimum and maximum values are shown in parentheses
Sex |
Metal concentration/μg g−1 dry weight |
Cu |
Cd |
Pb |
Zn |
Males (n = 5) |
39.2 ± 6.8 |
0.07 ± 0.00 |
36.1 ± 4.7 |
122.4 ± 11.5 |
(22.0–55.0) |
(0.06–0.08) |
(21.0–46.0) |
(93.0–152.0) |
Females (n = 5) |
33.7 ± 3.9 |
0.07 ± 0.00 |
29.0 ± 3.1 |
124.4 ± 18.9 |
(24.5–48.0) |
(0.06–0.08) |
(23.1–40.0) |
(77.6–180.0) |
P (t-test) |
0.51 |
0.42 |
0.24 |
0.93 |
3.5. Metal accumulation with age
To determine metal accumulation with age of House Sparrows, eggs and specimens of different ages were collected and analyzed for metal content. Levels of Cu, Pb, and Zn were found to accumulate significantly (ANOVA, P < 0.001) with increasing age stage (Fig. 1). Cd levels, on the other hand, did not exhibit a significant accumulation pattern. The Tukey test showed that adults contained significantly higher levels of Cu, Pb and Zn than all other age stages, whereas all other pairwise comparisons between age stages resulted in no significant difference. The strongest multiple correlation (R) values were obtained for Pb (0.92), followed by Cu (0.88), and Zn (0.77). Fig. 1 shows that accumulation of these three metals starts early after hatching, and reaches a maximum value in adults. Adults contained about 4 times Pb as much as one-month-old juveniles and about 25 times as much as the eggs. They contained twice as much Cu as much as one-month-old juveniles and 9 times as much as the eggs. However, Zn levels in adults were about 1.5 times as high as in one-month-old juveniles and 2 times as high as in eggs. The concentration of Pb in adults compared to one-month-old juveniles or eggs reflects urban Pb pollution, which originates mainly from leaded gasoline. In addition, Pb is a non-essential metal that can be bioaccumulated in living tissues. Zn and Cu, on the other hand, are essential metals which play a biological role in living organisms such as in egg development and feather formation.9,31 Nam et al.39 investigated the effect of age on the accumulation of Pb and Cd in feral Pigeons (Columba livia), and found that concentrations of both metals increased in the order: eggs < chicks < adults. Burger40 reviewed literature data on age differences in metal levels in feathers and found that age differences occurred for metals in 17 species. Burger and Gochfeld41 found that young Brown Noddy and Wood Storks had significantly lower levels of Cd and Pb than adults. Maedgen et al.42 found that concentrations of metals in adult Royal Terns were markedly higher than those in prefledgling terns, suggesting that accumulation of metals continues as birds age.
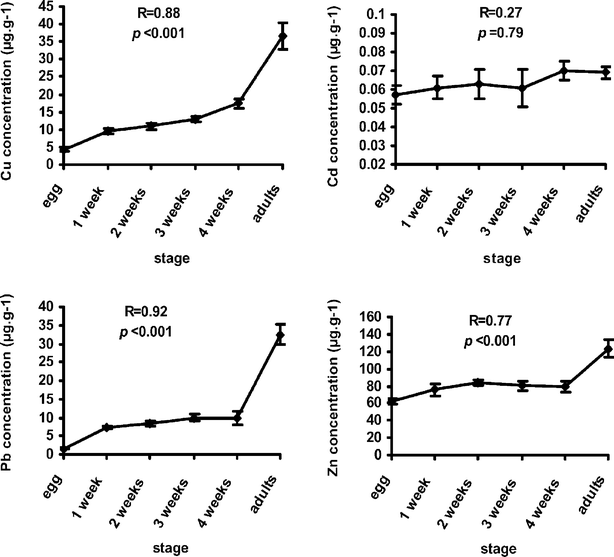 |
| Fig. 1 Metal levels in the livers of different age stages of House Sparrows (Passer domesticus) collected from urban environments in the West Bank. 5–10 samples were analysed for each age stage. | |
Acknowledgements
The authors would like to thank Mr N. Halaweh for his assistance in collecting and dissecting the samples. The efforts of Mr Azmi Tucktuck in reading metal concentrations using the ICP are gratefully acknowledged.
References
- E. Martínez-López, P. María-Mojica, J. E. Martínez, J. F. Calvo, D. Romero and A. J. García-Fernández, Bull. Environ. Contam. Toxicol., 2005, 74, 477 CrossRef CAS.
- J. Burger, Auk, 113, 399 Search PubMed.
- S. Gragnaniello, D. Fulgione, M. Milone, O. Soppelsa, P. Cacace and L. Ferrara, Bull. Environ. Contam. Toxicol., 2001, 66, 719 CrossRef CAS.
- D. J. H. Phillips, Environ. Pollut. (Amsterdam, Neth.), 1977, 13, 281 Search PubMed.
-
R. W. Furness, in Birds as Monitors of Environmental Change, ed. R. W. Furness and J. J. D. Greenwood, Chapman Hall, London, 1993, pp. 86–143 Search PubMed.
- M. Gochfeld, Arch. Environ. Contam. Toxicol., 1997, 33, 66.
- J. Burger, G. E. Woolfenden and M. Gochfeld, Arch. Environ. Contam. Toxicol., 1999, 37, 385 CrossRef CAS.
- M. Eens, R. Pinxten, R. F. Verheyen, R. Blust and L. Bervoets, Ecotoxicol. Environ. Saf., 1999, 44, 81 CrossRef CAS.
- S. Muralidharan, R. Jayakumar and G. Vishnu, Bull. Environ. Contam. Toxicol., 2004, 73, 285 CAS.
- S. D. Sundlof, M. G. Spalding, J. D. Wentworth and C. K. Steible, Arch. Environ. Contam. Toxicol., 1994, 27, 299 CAS.
- J. B. Cohen, J. S. Barclay, A. R. Major and J. P. Fisher, Arch. Environ. Contam. Toxicol., 2000, 38, 83 CrossRef CAS.
- P. A. Movalli, Environ. Pollut. (Amsterdam, Neth.), 2000, 109, 267 Search PubMed.
- T. Snoeijs, T. Dauwe, R. Pinxten, F. Vandesande and M. Eens, Arch. Environ. Contam. Toxicol., 2004, 46, 399 CAS.
- E. Janssens, T. Dauwe, E. Van Duyse, J. Beernaert, R. Pinxten and M. Eens, Arch. Environ. Contam. Toxicol., 2003, 45, 121 CrossRef CAS.
- D. H. White, K. A. King, C. A. Mitchell and B. M. Mulhern, Bull. Environ. Contam. Toxicol., 1986, 36, 376 CrossRef CAS.
-
R. W. Furness, in Environmental Contamination in Wildlife: Interpreting Tissue Concentrations, ed. W. N. Beyer, G. H. Heinz and A. W. Redmon-Norwood, CRC Press Inc., Boca Raton, FL, 1996, pp. 389–404 Search PubMed.
- M. Hutton, Environ. Pollut., Ser. A, 1980, 22(4), 281 Search PubMed.
- P. A. E. L. Schilderman, J. A. Hoogwerff, F. J. van Schooten, L. M. Maas, E. J. C. Moonen, B. J. H. van Os, J. H. van Wijnen and J. C. S. Kleinjans, Environ. Health Perspect., 1997, 105(3), 322 CrossRef CAS.
- M. Janiga and M. Žemberyová, Arch. Environ. Contam. Toxicol., 1998, 35, 70 CrossRef CAS.
- M. Hutton and G. T. Goodman, Environ. Pollut., Ser. A, 1980, 22(3), 207 Search PubMed.
- D. H. Nam, D. P. Lee and T. H. Koo, Environ. Monit. Assess., 2004, 95(1–3), 13 CrossRef CAS.
-
P. Kaminski, in Nestling Mortality of Granivorous Birds due to Microorganisms and Toxic Substances, ed. J. Pinwski, B. P. Kavanagh and B. Pinowska, PWN-Polish Sci. Publ., Warszawa, 1995, pp. 31–55 Search PubMed.
- P. Chao, Z. Guangmei, Z. Zhengwang and Chengyyi, Bull. Environ. Contam. Toxicol., 2003, 71, 142 CrossRef CAS.
- J. Burger, J. Toxicol. Environ. Health, 1995, 45, 369 CrossRef CAS.
- K. M. Swaileh, R. M. Hussein and Abu-Elhaj, Arch. Environ. Contam. Toxicol., 2004, 47(1), 23 CAS.
-
SYSTAT
for Windows Software, SYSTAT for Windows Inc., Evanston, IL, USA, ver. 11, 2004 Search PubMed.
- Q. M. Jaradat and K. A. Momani, Turk J. Chem., 1999, 23, 209 Search PubMed.
- J. Burger and M. Gochfeld, Environ. Monit. Assess., 1999, 58, 105 CrossRef CAS.
-
P. M. Walsh, in Heavy Metals in the Marine Environment, ed. R. W. Furness and P. S. Rainbow, CRC Press Inc., Boca Raton, FL, 1990, pp. 183–204 Search PubMed.
- T. Dauwe, L. Bervoets, R. Blust, R. Pinxten and M. Eens, Belg. J. Zool., 1999, 129(2), 439 Search PubMed.
- M. Morera, C. Sanpera, S. Crespo, L. Jover and X. Ruiz, Arch. Environ. Contam. Toxicol., 1997, 33, 71 CrossRef CAS.
- M. P. Richards and N. C. Steel, J. Exp. Zool. Supp., 1987, 1, 39 Search PubMed.
- A. A. Goede and M. De Bruin, Environ. Monit. Assess., 1986, 7, 249 CrossRef CAS.
- J. Burger, Rev. Environ. Toxicol., 1993, 5, 203 Search PubMed.
- K. Dmowski, Acta Ornithol., 1999, 34(1), 1 Search PubMed.
- A. S. Spahn and T. W. Sherry, Arch. Environ. Contam. Toxicol., 1999, 37, 377 CrossRef.
- M. F. Wolfe, S. Schwarzbach and R. A. Sulaiman, Environ. Toxicol. Chem., 1998, 17, 146 CrossRef CAS.
- G. M. Donaldson and B. M. Braune, Arch. Environ. Contam. Toxicol., 1999, 3, 110 CrossRef.
- D. H. Nam, D. P. Lee and T. H. Koo, Environ. Monit. Assess., 2004, 95(1–3), 23 CrossRef CAS.
- J. Burger, J. Toxicol. Environ. Health, 1994, 41, 207 CrossRef CAS.
- J. Burger and M. Gochfeld, Environ. Toxicol. Chem., 1995, 14(7), 1233 CrossRef CAS.
- J. L. Maedgen, C. S. Hacker, G. D. Schroder and F. W. Weir, Arch. Environ. Contam. Toxicol., 1982, 11(1), 99 CAS.
|
This journal is © The Royal Society of Chemistry 2006 |