DOI:
10.1039/B306699A
(Paper)
New J. Chem., 2004,
28, 26-33
Supramolecular assembly of ferrocenes via hydrogen bonds: dimensional variation in ferrocenylpyrimidine complexes with carboxylic acids and aromatic alcohols
Received
(in Montpellier, France)
12th June 2003
, Accepted 29th August 2003
First published on 22nd October 2003
Abstract
Co-crystallization of 5-ferrocenylpyrimidine (FcPM) with various carboxylic acids and aromatic alcohols produces hydrogen-bonded supramolecular architectures. Thus, reaction of FcPM with compounds containing two hydrogen-bonding sites gives 2:1 co-crystals with discrete structures of the type [(FcPM)2·D] [where D
=
succinic acid (1), hydroquinone (2), resorcinol (3) and 2,2′-thiodiglycolic acid (4)]. A complex with a chiral chain structure, [FcPM·{(R)-(+)-1,1′-bi-2-naphthol}]n (5), is obtained by the combination of FcPM with (R)-(+)-1,1′-bi-2-naphthol. The binaphthol molecules form hydrogen-bonded helical chains, which carry ferrocene units as pendants. The combination of FcPM with trimesic acid and pyromellitic acid produces supramolecular complexes with tape structures, [FcPM·(trimesic acid)]n (6) and [FcPM2·(pyromellitic acid)]n (7), respectively. The tape structure of 6 consists of repeating units of large hexagonal rings while that of 7 consists of rhomboidal rings. Combination of FcPM with phloroglucinol produces a layered structure complex, [FcPM·(phloroglucinol)·2H2O]n (8), exhibiting three-dimensional hydrogen bonding. In this complex, FcPM molecules link hydrogen-bonded sheets composed of phloroglucinol and water molecules. The dimensionality of the assembled structures is influenced by the number of hydrogen-bonding substituents on the donor molecules.
Introduction
Currently, there is much interest in the synthesis of materials possessing desired crystallographic architectures as well as specific physical and chemical properties.1 Coordination bonds and hydrogen bonds can be used to control the organization of molecules or ions in the solid state and both have been widely used in crystal engineering and supramolecular design.2,3 To synthesize redox-active supramolecules with organometallic motifs, we have designed several ferrocene-based supramolecular building blocks and assembled them via coordination bonds; side-chain coordination polymers, main-chain polymers as well as ferrocene clusters have been synthesized so far.4 As the next target, we focused on the use of hydrogen bond for assembling these organometallic components. Among the molecules we designed, 5-ferrocenylpyrimidine (FcPM)4a,b is interesting for supramolecule construction because the pyrimidine moiety can act not only as a metal coordination site, but also as a hydrogen-bond accepting site. For example, several functional molecular assemblies, which show guest inclusion properties, catalytic activities, etc., have been synthesized using the hydrogen-bonding abilities of pyrimidine derivatives.5
Thus, to design hydrogen-bonded molecular assemblies containing ferrocenes, we combined FcPM and various organic hydrogen-bond donors, shown in Fig. 1, which are frequently employed as building blocks of hydrogen-bonded crystals.3,6 Hydrogen-bond donors, carrying two, three or four hydrogen-bonding substituents, have been selected to investigate hydrogen-bond topology and dimensionality. We used phenol derivatives such as hydroquinone, resorcinol, (R)-(+)-1,1′-bi-2-naphthol and phloroglucinol, and carboxylic acids: succinic acid, 2,2′-thiodiglycolic acid, trimesic acid and pyromellitic acid.
Metallocenes7 play important roles in the field of materials science, as components of catalysts,8 molecular magnets9 and non-linear optical materials.10 In particular, the redox activity of metallocenes leads to a variety of interesting electrical and photophysical phenomena.11 So far, several hydrogen-bonded supramolecules derived from metallocenes have been reported, in which the organometallic component works as a hydrogen-bond donor or as a hydrogen-bond acceptor.12 However, hydrogen-bonded complexes of mono-substituted ferrocenes have received less attention.13 In the present paper, we describe the structural variation in hydrogen-bonded co-crystals of 5-ferrocenylpyrimidine (FcPM), with the hydrogen-bond topology ranging from discrete to three-dimensional. Together with our previous study on the assembly of FcPM via coordination bonds, our studies demonstrate the crystal engineering possibilities of ferrocene-containing materials.
Results and discussion
A. Synthesis
FcPM and various hydrogen-bond donors were mixed in a ratio of 1∶1 and dissolved in methanol or acetonitrile. The solutions were left to evaporate slowly at room temperature in air. Single crystals of carboxylic acid complexes were obtained from methanol solutions, while those of phenol complexes (except for complex 5) were from acetonitrile solutions. Compounds 1–8 were all isolated in good yields as the sole products. The composition of the products was either 1∶1 or 2∶1 FcPM∶donor stoichiometry and were not affected by changes in the stoichiometry of FcPM and donors used in the reaction. Crystal data, data collection parameters and analysis statistics for complexes 1–8 are listed in Table 1. In all compounds, O–H⋯N hydrogen bonds are formed, as summarized in Table 2. The hydrogen-bond distances in carboxylic acid complexes (1, 4, 6, and 7) are 2.67–2.78 Å and those in phenol complexes (2, 3, 5, and 8) are 2.69–2.81 Å, which are typical values.14 Other hydrogen-bond donors, such as 1,2,3- and 1,2,4- benzenetricarboxylic acid, oxalic acid, malonic acid, squaric acid and catechol, were also tested but they failed to co-crystallize with FcPM.
|
1
|
2
|
3
|
4
|
5
|
6
|
7
|
8
|
I > 0.0σ(I)
R
1 = Σ||Fo| − |Fc||/Σ|Fo|; Rw = [Σw(Fo2 − Fc2)2/Σw(Fo2)2]1/2.
|
Formula |
C32H30N4O4Fe2 |
C34H30N4O2Fe2 |
C34H30N4O2Fe2 |
C32H30N4O4SFe2 |
C34H26N2O4Fe |
C23H18N2O6Fe |
C34H30N4O8Fe2 |
C20H22N2O5Fe |
Formula weight |
646.31 |
638.33 |
638.33 |
678.37 |
550.44 |
474.25 |
782.37 |
426.25 |
Crystal system |
Monoclinic |
Monoclinic |
Monoclinic |
Monoclinic |
Monoclinic |
Triclinic |
Monoclinic |
Orthorhombic |
Space group |
P21/n
(No.14) |
P21/n
(No.14) |
P21/n
(No.14) |
P21/c
(No.14) |
P21
(No.4) |
Pī
(No.2) |
C2/c
(No. 15) |
P
bcm
(No.57) |
a/Å |
9.755(4) |
10.801(5) |
10.766(3) |
7.406(3) |
9.320(4) |
11.037(2) |
12.474(4) |
7.997(3) |
b/Å |
8.478(3) |
7.696(5) |
7.576(3) |
19.131(7) |
10.951(5) |
12.652(4) |
13.687(3) |
14.278(2) |
c/Å |
17.791(3) |
17.052(4) |
17.452(4) |
20.727(3) |
13.149(4) |
7.518(5) |
19.390(3) |
17.219(2) |
α/deg |
90 |
90 |
90 |
90 |
90 |
104.42(3) |
90 |
90 |
β/deg |
95.72(2) |
95.10(3) |
94.59(2) |
92.42(3) |
99.32(3) |
96.41(3) |
94.10(2) |
90 |
γ/deg |
90 |
90 |
90 |
90 |
90 |
88.21(2) |
90 |
90 |
U/Å3 |
1464.1(8) |
1411(1) |
1418.9(6) |
2933(1) |
1324.3(9) |
1010.4(8) |
3302(1) |
1966.3(10) |
Z
|
2 |
2 |
2 |
4 |
2 |
2 |
4 |
4 |
μ/cm−1 |
10.34 |
10.66 |
10.61 |
11.04 |
6.04 |
7.91 |
4.93 |
8.00 |
T/K |
296 |
296 |
296 |
296 |
296 |
296 |
296 |
296 |
Measured reflections |
3797 |
3653 |
3679 |
7461 |
3391 |
4892 |
3777 |
2703 |
Observed reflections (I > 2.0σ(I)] |
2305 |
2729 |
2071 |
4327 |
3385a |
2935 |
3926 |
1437 |
b
R
1
|
0.060 |
0.050 |
0.049 |
0.041 |
0.042 |
0.042 |
0.052 |
0.057 |
b
R
w
|
0.180 |
0.148 |
0.143 |
0.129 |
0.137 |
0.112 |
0.164 |
0.171 |
Table 2 Hydrogen bond distances in 1–8
Complex |
D–H⋯A |
D⋯A/Å |
Symmetry code |
Carboxylic acid oxygen.
Phenolic oxygen.
Disordered hydrogen.
|
1
|
O1a–H15⋯N1 |
2.681(5) |
x, −1 + y, z |
2
|
O1b–H14⋯N1 |
2.805(4) |
x, −1 + y, z |
3
|
O1b–H14⋯N1 |
2.793(8) |
1/2 − x, 1/2 + y, 1/2 − z |
|
O2b–H26⋯N1 |
2.882(8) |
1/2 − x, 1/2 + y, 1/2 − z |
4
|
O1a–H25⋯N1 |
2.697(5) |
x, 1/2 − y, 1/2 + z |
|
O3a–H30⋯N3 |
2.695(5) |
−1 + x, −1 + y, z |
5
|
O1b–H13⋯O2 |
2.812(6) |
−x, 1/2 + y, 1 − z |
|
O2b–H20⋯N2 |
2.686(7) |
1 − x, −1/2 + y, 1 − z |
6
|
O1a–H16⋯N1 |
2.673(3) |
−1 − x, −1 − y, −1 − z |
|
O3a–H17⋯N2 |
2.695(3) |
−1 − x, −y, −z |
|
O5a–H18⋯O6 |
2.678(3) |
−2 − x, −1 − y, −z |
7
|
O1a–H13⋯N1 |
2.778(3) |
|
|
O3a–H14⋯N2 |
2.737(4) |
3/2 − x, −1/2 − y, 2 − z |
8
|
O1b–H10⋯N |
2.742(5) |
−x, −1/2 + y, z |
|
O2b–Hc⋯O3w |
2.715(4) |
|
|
O3w–Hc⋯O1 |
2.787(4) |
−1 + x, y, z |
|
O3w–Hc⋯O3w |
2.667(8) |
−x, −1 − y, −1 − z |
B. Discrete complexes with 2∶1 stoichiometry: [(FcPM)2·D] [D
=
succinic acid (1), hydroquinone (2), resorcinol (3) and 2,2′-thiodiglycolic acid (4)]
The combination of FcPM with organic molecules that have two hydrogen-donor substituents, such as succinic acid, hydroquinone, resorcinol and 2,2′-thiodiglycolic acid, produced discrete complexes with a 2∶1 stoichiometry: [(FcPM)2·D] [with D
=
succinic acid (1), hydroquinone (2), resorcinol (3) and 2,2′-thiodiglycolic acid (4)]. The structures of 1–4 have been determined crystallographically. In these complexes, two FcPM molecules are bridged by the donor molecule via the O–H⋯N hydrogen bonds, as shown in Fig. 2. Thus, one of the two nitrogen atoms of FcPM is involved in hydrogen bonding, while the other is free. The discrete units of 1 and 2 lie on inversion centres, while that of 4 has no inversion symmetry. The resorcinol molecule in 3 shows two-fold disorder around the centre of the benzene ring with a site occupancy of 1∶1; the discrete unit was observed to have an apparent inversion centre. In Fig. 2(c), only one disordered site of the resorcinol molecule is shown for simplicity. The crystal structures, packing modes and cell parameters of 1–3 are very similar and they are almost isomorphous with each other. It is highly interesting that such a similarity is observed, independent of the nature of the hydrogen-bonding species (i.e., carboxylic acids or phenols) or substituent position (i.e., meta or para isomers). The common feature in these complexes is that the intermolecular N(1)⋯N(1*) separations through the hydrogen bonds are about 10 Å, which may presumably result in similar packing structures. In the crystals of 1–4, the N⋯N distances are 10.62, 9.77, 9.76 and 9.86 Å, respectively, and the intramolecular O⋯O distances in the hydrogen-bond donors are 5.92, 5.52, 4.66 and 6.34 Å, respectively.
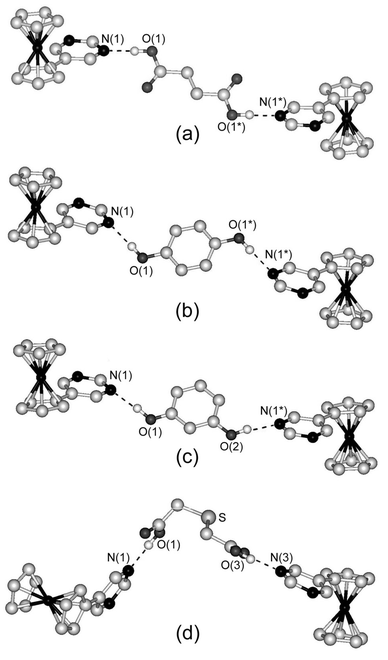 |
| Fig. 2 Structures of the discrete units in (a) 1, (b)
2, (c)
3 and (d)
4. Dashed lines indicate hydrogen bonds. Hydrogen atoms bonded to carbon atoms are omitted for clarity. For complex 3, only one disordered site of the resorcinol molecule is shown for clarity. | |
C. Chiral chain complex with ferrocenyl pendants: [FcPM·{(R)-(+)-1,1′-bi-2-naphthol}]n (5)
To construct a chiral supramolecular assembly of FcPM, we used commercially available (R)-(+)-1,1′-bi-2-naphthol, which is a representative chiral molecule with two hydrogen-bonding sites. The combination of this molecule with FcPM produced a 1∶1 complex, [FcPM·{(R)-(+)-1,1′-bi-2-naphthol}]n (5). The absolute structure of 5 was confirmed by referring to the Flack parameter. Fig. 3 shows the hydrogen-bonded chain structure of 5, in which binaphthols are linked via O1–H⋯O2* intermolecular hydrogen bonds [2.812(6)
Å] to form a chiral chain. The O2 atoms are further bonded to FcPM molecules via the O2–H⋯N2 hydrogen bond [2.686(7)
Å]. Thus, FcPM molecules are attached to the helical chain of binaphthol molecules as pendants. Only one nitrogen atom of FcPM is involved in hydrogen bonding. The ferrocene moieties along the chain are arranged in the same direction, which leads to the polar structure of the crystal.
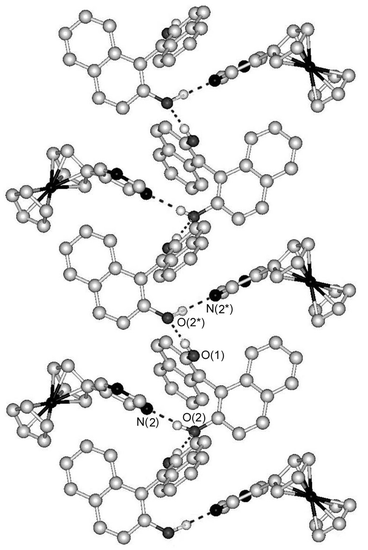 |
| Fig. 3 Part of the chiral chain structure of 5. Dashed lines indicate hydrogen bonds. Hydrogen atoms bonded to carbon atoms are omitted for clarity. | |
Among the hydrogen-bond donors we used, this was the only molecule that has chirality. The control of chirality in ferrocene-based materials is interesting from the viewpoint of nonlinear optical properties. As a related example, Lee and Chung13b have reported the synthesis and structure of hydrogen-bonded supramolecules composed of 1,1′-bis(ethenyl-4-pyridiyl)ferrocene and 1,1′-bi-2-naphthol. In their system, control of the assembled structures was achieved by changing the recrystallization solvent and the relationship between the hydrogen-bonded ferrocene assembled structures and SHG efficiencies has been demonstrated. In the present study, we tried to obtain different co-crystals by changing solvents and also tried to co-crystallize FcPM with racemic 1,1′-bi-2-naphthol, but both strategies were unsuccessful.
D. Tape-structure complexes with hydrogen-bonded cyclic motifs: [FcPM·(trimesic acid)]n (6) and [(FcPM)2·(pyromellitic acid)]n (7)
To expand the dimensionality of FcPM complexes, we combined FcPM with trimesic acid and pyromellitic acid, which have three and four carboxyl groups, respectively. Combination of FcPM with trimesic acid produced a 1∶1 complex, [FcPM·(trimesic acid)]n (6). Fig. 4 shows the tape structure of 6, which runs along the [0,1,1] direction. The tape is formed by a large hexagonal ring repeating unit consisting of four molecules of trimesic acid and two molecules of FcPM. Two of the carboxyl groups of trimesic acid link FcPM via O–H⋯N hydrogen bonds, while the other carboxyl group forms a homomeric self-complementary hydrogen bond. Two ferrocene moieties of adjacent tapes are located inside the hexagonal ring. The inversion centre lies at the centre of the self-complementary hydrogen bond. Judging from the normal bond lengths of 1.299(4)
Å
(C–OH) and 1.234(4)
Å
(C
O), there seems to be no proton transfer occurring along the hydrogen bonds1f,15 within the carboxylic acid dimer.
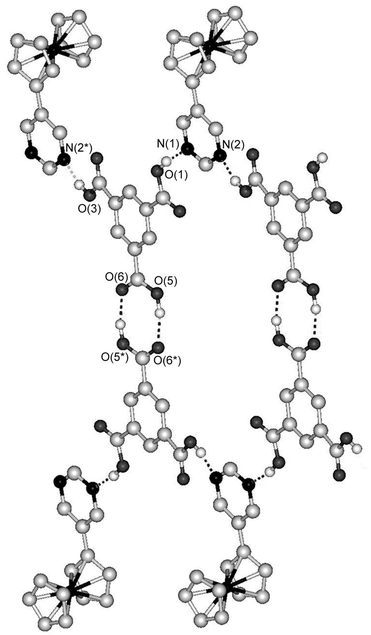 |
| Fig. 4 Part of the tape structure of 6. Dashed lines indicate hydrogen bonds. Hydrogen atoms bonded to carbon atoms are omitted for clarity. | |
On the other hand, the combination of FcPM with pyromellitic acid produced a 2∶1 complex, [(FcPM)2·(pyromellitic acid)]n (7). This complex also exhibits a tape structure, which runs along the a axis. Fig. 5 shows the tape structure, which consists of a square ring repeating unit formed from two molecules of pyromellitic acid and two molecules of FcPM. In contrast to the planar hexagonal repeating unit in 6, the square unit in 7 is tilted, because neighbouring carboxyl groups of pyromellitic acid are perpendicular to each other. The inversion centre of 7 lies at the centre of pyromellitic acid.
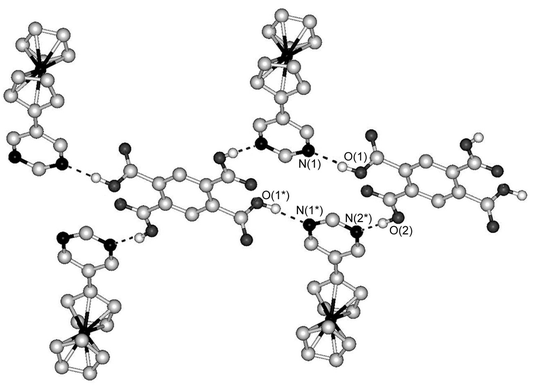 |
| Fig. 5 Part of the tape structure of 7. Dashed lines indicate hydrogen bonds. Hydrogen atoms bonded to carbon atoms are omitted for clarity. | |
Fig. 6 shows a schematic illustration of the expanded structures of 6 and 7. The tape structures in both complexes are double-chain structures, which are composed of alternate linking of FcPM and hydrogen-bond donors. The trimesic acid in 6 is dimerized through self-complementary hydrogen bonding and works as a unit that contains four hydrogen bonding sites. Thus, from the viewpoint of hydrogen-bonding topology, trimersic acid in 6 plays the same role as pyromellitic acid in 7.
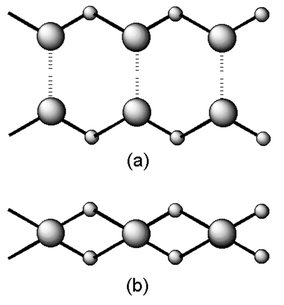 |
| Fig. 6 Schematic illustrations of the tape structures in (a) 6 and (b) 7. | |
E. Three-dimensional hydrogen-bonded complex with a layered structure: [FcPM·(phloroglucinol)·2H2O]n (8)
The combination of FcPM with phloroglucinol, which contains three hydroxyl groups, produced a three-dimensionally hydrogen-bonded complex, [FcPM·(phloroglucinol)·2H2O]n (8). The complex crystallizes in the space group Pbcm and the asymmetric unit is comprised of a half molecule of FcPM, a half molecule of phloroglucinol and one H2O molecule. Due to the crystallographically imposed mirror symmetry on FcPM, the Cp ring and pyrimidine ring are co-planar. This is somewhat exceptional because the rings in FcPM are generally twisted due to the steric hindrance of the ring hydrogens, as observed in the crystals of FcPM, complexes 1–7 and in some metal complexes.4a,b
Complex 8 has a layered structure, composed of two-dimensional phloroglucinol–water sheets that are linked by FcPM molecules via hydrogen bonds. Fig. 7(a) shows the structure of the hydrogen-bonded sheet, which extends within the ab plane. The hydroxyl groups of phloroglucinol form hydrogen bonds with water molecules. Two of the phenolic hydrogens are further hydrogen-bonded to FcPM molecules that are located outside the sheet. The hydrogen bond distances of O1…N and O1…O3w are 2.747(4) and 2.787(4)
Å, respectively. Thus, FcPM molecules are located between the phloroglucinol–water sheets, as shown in Fig. 7 (c). Fig. 7 (d) shows the connection between FcPM and phloroglucinol molecules along the c axis. Several examples of hydrogen-bonded co-crystals of phloroglucinol have been reported so far16 and in particular, the structure of phloroglucinol·2H2O is interesting with reference to the present structure: it consists of two-dimensional sheets formed by phloroglucinol and water molecules,17 in which each phenol group is hydrogen-bonded to two water molecules. Therefore, complex 8 may be regarded as an insertion product of FcPM into phloroglucinol·2H2O, although the hydrogen-bonding topology of the sheets is not exactly the same.
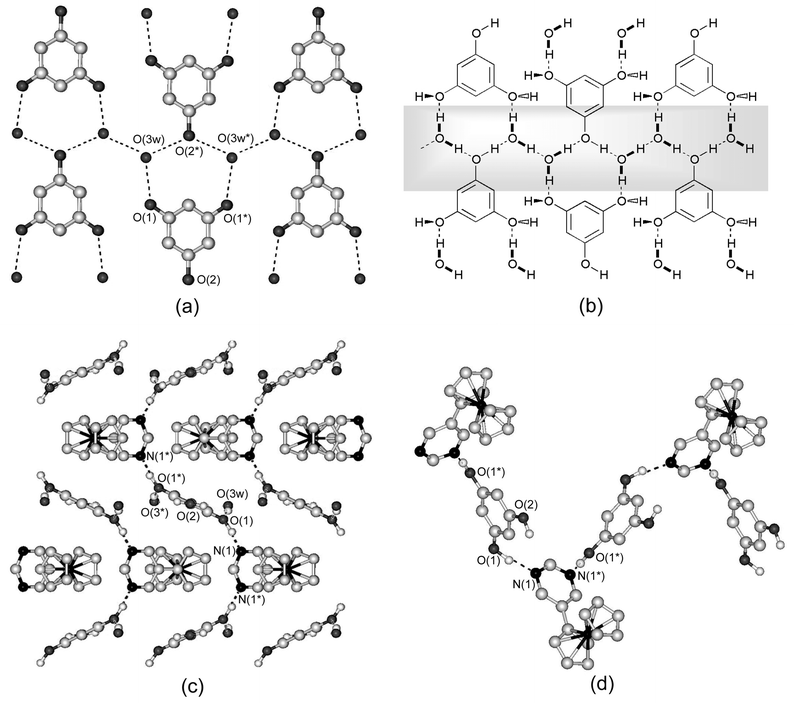 |
| Fig. 7 Crystal structure of 8. (a) Two-dimensional sheet composed of phloroglucinol and two H2O molecules and (b) a schematic illustration in which the shaded part indicates hydrogen-bonded chains (see text). (c) Packing diagram of 8 viewed along the a axis. (d) One-dimensional hydrogen-bonded chain of FcPM and phloroglucinol. Dashed lines indicate hydrogen bonds. Hydrogen atoms bonded to carbon atoms are omitted for clarity. | |
Fig. 7(b) shows a schematic representation of the phloroglucinol–water sheet. It is interesting to note that the sheet involves one-dimensional hydrogen-bonded chains, which are formed by O2 atoms of phloroglucinol and water molecules, as shown in the shaded part of Fig. 7(b). X-Ray structure analysis revealed that phloroglucinol lies on a two-fold screw axis and the hydrogen positions in this one-dimensional chain are disordered. Thus, the chain is observed as the averaged structure of ⋯O2–H⋯O3w–H⋯O2–H⋯ and ⋯H–O2⋯H–O3w⋯H–O2⋯, which indicates that the hydrogen atoms are either statistically or dynamically disordered. Indeed, one-dimensional hydrogen-bonded systems are of interest from the viewpoint of proton dynamics involving the mechanism of solitonic excitation.18 Study of a possible dynamical behaviour in this solid is in progress in our laboratories by means of solid-state NMR and dielectric spectroscopy.
We also investigated the release of the water molecules from the crystals of 8. Thermogravimetric analysis revealed a gradual weight loss of ca. 8–9% of the original weight between 70–130
°C, which corresponds to the loss of the two H2O molecules (calcd 8.4%).
F. Dimensionality in hydrogen-bonded supramolecules of FcPM
As shown above, the combination of FcPM with various hydrogen-bond donors produces supramolecular architectures formed by O–H⋯N hydrogen bonds. The hydrogen-bonding ability of FcPM may be better than that of pyrimidine, due to the strong electron-releasing effect of the ferrocenyl group. We can recognize some correlations between the number of hydrogen-bonding substituents and the dimensionality of the supramolecular architectures; upon increasing the number of hydrogen-bonding substituents on the donor molecule the dimensionality of the assembled structure increases. The dimensionality of the hydrogen bonding in the present complexes can be summarized as follows: hydrogen-bond donors with two hydrogen-bonding sites give low-dimensional structures, that is , discrete complexes (1–4) and a one-dimensional complex (5), in which only one of the nitrogen atoms of FcPM forms a hydrogen bond. However, for donors with more than three hydrogen-bonding substituents, the resulting complexes show high-dimensional structures, in which both nitrogen atoms of FcPM are involved in hydrogen bonds: trimesic acid (1,3,5-tricarboxybenzene) and pyromellitic acid (1,2,4,5-tetracarboxybenzene) produce the tape complexes 6 and 7, while phloroglucinol (1,3,5-trihydroxybenzene) forms a layered complex with three-dimensional hydrogen bonds. FcPM can therefore be considered a flexible hydrogen-bond acceptor, which will lead to a diversity of architectures. It has been demonstrated that the hydrogen-bonding supramolecules involving trimesic acid show honeycomb 2-D structures or laminates and accommodate guest molecules in the cavities.19 However, complex 6 does not have such an extended structure, presumably because of the bulkiness of the ferrocenyl moiety, which occupies the honeycomb cavities. On the other hand, the tape structure of pyromellitic acid complexes as in 7 is more common, as can be found in the crystal structure of pyromellitic acid·2DMSO.20
In our previous study on the assembly of ferrocene-based materials via coordination bonds, topologically interesting architectures, such as redox-active molecular chains, 2-D structures and metallocene clusters, were obtained.4 In the present work, we have demonstrated the hydrogen-bond directed organization of organometallic components, which leads to a variation of the dimensionality, ranging from discrete to three-dimensional. In particular, the correlation between the dimensionality and the kind of hydrogen-bond donors has been shown. One of the advantages of the bulk assembled materials is the ease of structural characterization by crystallography. Studies on the assembly of redox-active components will further build the foundations for the construction and analysis of functional 2-D surfaces organized by hydrogen bonds or coordination bonds.
Experimental
General methods
All reagents and solvents were commercially available except for 5-ferrocenylpyrimidine (FcPM),4b which was synthesized by following the literature procedure. Infrared spectra were recorded on a JASCO FT-IR 230 spectrometer as KBr pellets in the 4000–400 cm−1 range. Thermogravimetric analysis was performed on a Seiko TG/DTA 6200. Elemental analyses were performed on a Perkin–Elmer 2400CHN Elemental Analyzer.
Preparations
[(FcPM)2·(succinic acid)] (1).
To a solution of FcPM (26 mg, 1.0
×
10−4 mol) in 2 mL of methanol, a solution of succinic acid (12 mg, 1.0
×
10−4 mol) in 2 mL of methanol was added. After standing for a few days, orange crystals were formed in good yield (40–75%) as the sole product, which was characterized by X-ray diffraction, infrared spectra and elemental analysis. FT-IR v/cm−1: 3100–2200 br (OH) and 1697 s (CO). Anal. calcd for C32H30N4O4Fe2: C, 59.47; H, 4.68; N, 8.67; found C, 59.67; H, 4.73; N, 8.64%.
[(FcPM)2·(hydroquinone)] (2).
Prepared as for 1 using FcPM (26 mg, 1.0
×
10−4 mol) in 2 mL of acetonitrile and hydroquinone (11 mg, 1.0
×
10−4 mol) in 2 mL of acetonitrile. Orange prismatic crystals. FT-IR v/cm−1: 3300–2400 br (OH). Anal. calcd for C34H30N4O2Fe2: C, 63.98; H, 4.74; N, 8.78; found: C, 63.87; H, 4.73; N, 8.77%.
[(FcPM)2·(resorcinol)] (3).
Prepared as for 1 using FcPM (26 mg, 1.0
×
10−4 mol) in 2 mL of acetonitrile and resorcinol (11 mg, 1.0
×
10−4 mol) in 2 mL of acetonitrile. Orange prismatic crystals. FT-IR v/cm−1: 3300–2400 br (OH). Anal. calcd for C34H30N4O2Fe2: C, 63.98; H, 4.74; N, 8.78; found: C, 63.00; H, 5.12; N, 7.19%.
[(FcPM)2·(2,2′–thiodiglycolic acid)] (4).
Prepared as for 1 using FcPM (26 mg, 1.0
×
10−4 mol) and 2,2′-thiodiglycolic acid (15 mg, 1.0
×
10−4 mol). Orange plate-like crystals. FT-IR v/cm−1: 3300–2400 br (OH) and 1706 s (CO). Anal. calcd for C32H30N4O4Fe2S: C, 56.66; H, 4.46; N, 8.26; found: C, 56.46; H, 4.53; N, 7.99%.
[FcPM·{(R)-(+)-1,1′-bi-2-naphthol}]n (5).
Prepared as for 1 using FcPM (26 mg, 1.0
×
10−4 mol) and (R)-(+)-1,1’-bi-2-naphthol (29 mg, 1.0
×
10−4 mol). Orange prismatic crystals. FT-IR v/cm−1: 3600–2800 br (OH). Anal. calcd for C34H26N2O2Fe: C, 74.19; H, 4.76; N, 5.09; found: C, 73.94; H, 4.85; N, 4.93%.
[FcPM·(trimesic acid)]n (6).
Prepared as for 1 using FcPM (26 mg, 1.0
×
10−4 mol) and trimesic acid (21 mg, 1.0
×
10−4 mol). Orange prismatic crystals. FT-IR v/cm−1: 3300–2500 br (OH) and 1698 s (CO). Anal. calcd for C23H18N2O6Fe: C, 58.25; H, 3.83; N, 5.91; found: C, 58.45; H, 3.87; N, 5.89%.
[(FcPM)2·(pyromellitic acid)]n (7).
Prepared as for 1 using FcPM (26 mg, 1.0
×
10−4 mol) and pyromellitic acid (25 mg, 1.0
×
10−4 mol). Orange prismatic crystals. FT-IR v/cm−1: 3100–2500 br (OH) and 1722 s (CO). Anal, calcd for C34H30N4O8Fe2: C, 58.34; H, 3.87; N, 7.16; found: C, 58.11; H, 3.94; N, 7.03%.
[FcPM·(phloroglucinol)·2H2O]n (8).
Prepared as for 1 using FcPM (26 mg, 1.0
×
10−4 mol) in 2 mL of acetonitrile and phloroglucinol·2H2O (16 mg, 1.0
×
10−4 mol) in 2 mL of acetonitrile. Orange plate-like crystals. FT-IR v/cm−1: 3100–2500 br (OH). Anal. calcd for C20H22N2O5Fe: C, 56.36; H, 5.20; N, 6.57; found: C, 56.24; H, 5.17; N, 6.64%.
Crystal structure analysis
X-Ray diffraction data for single crystals were collected on a Rigaku AFC-5S four-circle diffractometer equipped with a graphite crystal and incident beam monochromator using Mo-Kα radiation (λ
=
0.71073 Å) at 296 K. All calculations were performed using the teXsan crystallographic software package.21 These structures were solved by direct methods (SIR 9222) and expanded using Fourier techniques. Refinements were carried out on F2 and an absorption correction was applied (ϕ scan). The hydrogen atoms attached to carbon atoms were inserted at the calculated positions and allowed to ride on their respective parent atoms. The hydrogen atoms attached to oxygen atoms were located on the electron density maps and refined at fixed distances from the respective parent atoms, except for the water hydrogen atoms in compound 8, which could not be located. The absolute structure of 5 was determined based on the Flack parameter.†
Acknowledgements
This work was supported by the Morino Foundation for Molecular Science. We acknowledge JST (Japan Science and Technology Corporation) for the loan of experimental equipment. We are grateful to Mr. K. Takazawa for his assistance with the synthesis. We thank Mr. H. Iwata and Prof. T. Kitazawa (Toho University) for their help with the thermogravimetric (TG) analysis. We also thank Mr. M. Nakama (WarpStream Ltd., Tokyo) for constructing the Web-DB system.
References
-
(a) S. R. Seidel and P. J. Stang, Acc. Chem. Res., 2002, 35, 972 CrossRef CAS;
(b) O. R. Evans and W. Lin, Acc. Chem. Res., 2002, 35, 511 CrossRef CAS;
(c) F. A. Cotton, C. Lin and C. A. Murillo, Acc. Chem. Res., 2001, 34, 759 CrossRef CAS;
(d) S.-S. Sun and A. J. Lee, Coord. Chem. Rev., 2002, 230, 171 CrossRef CAS;
(e) R. J. Puddephatt, Chem. Commun., 1998, 1055 RSC;
(f)
Structure Correlation, eds. H.-B. Bürgi and J. D. Dunitz, VCH, Weinheim, 1994 Search PubMed.
-
(a)
Comprehensive Supramolecular Chemistry, eds. J.-M. Lehn, J. L. Atwood, J. E. D. Davis, D. D. MacNicol and F. Vögtle, Pergamon Press, Oxford, 1990–1996, vols. 1–11 Search PubMed;
(b)
J.-M. Lehn, Supramolecular Chemistry, Concepts and Perspectives, VCH, Weinheim, 1995 Search PubMed;
(c) T. J. Prior, D. Bradshaw, S. J. Teat and M. J. Rosseinsky, Chem. Commun., 2003, 500 RSC;
(d) G. F. Swiegers and T. J. Malefetse, Coord. Chem. Rev., 2002, 225, 91 CrossRef CAS;
(e) G. F. Swiegers and T. J. Malefetse, Chem.-Eur. J., 2001, 7, 3637 CrossRef CAS;
(f) T. J. Prior and M. J. Rosseinsky, Chem. Commun., 2001, 1222 RSC;
(g) S. R. Batten, CrystEngComm, 2001, 3, 67 RSC;
(h) B. Moulton and M. J. Zaworotko, Chem. Rev., 2001, 101, 1629 CrossRef CAS;
(i) M. J. Zaworotko, Chem. Commun., 2001, 1 RSC;
(j) P. Day, J. Chem. Soc., Dalton Trans., 2000, 3483 RSC;
(k) G. F. Swiegers and T. J. Malefetse, Chem. Rev., 2000, 100, 3483 CrossRef CAS;
(l) S. Leininger, B. Olenyuk and P. J. Stang, Chem. Rev., 2000, 100, 853 CrossRef CAS;
(m) P. J. Hagrman, D. Hagrman and J. Zubieta, Angew. Chem., Int. Ed., 1999, 38, 2638 CrossRef;
(n) C. J. Kepert, D. Hesek, P. D. Beer and M. J. Rosseinsky, Angew. Chem., Int. Ed., 1998, 37, 3158 CrossRef CAS;
(o) M. Fujita, Chem. Soc. Rev., 1998, 27, 418 RSC;
(p) B. Olwnyuk, A. Fechtenkötter and P. J. Stang, J. Chem. Soc., Dalton Trans., 1998, 1707 RSC;
(q) M. C. T. Fyfe and J. F. Stoddart, Acc. Chem. Res., 1997, 30, 393 CrossRef CAS.
-
(a) N. Shan, A. D. Bond and W. Jones, New J. Chem., 2003, 27, 365 RSC;
(b) R. D. B. Walsh, M. W. Bradner, S. Fleischman, L. A. Morales, B. Moulton, N. Rodriguez-Hornedo and M. J. Zaworotko, Chem. Commun., 2003, 186 RSC;
(c) N. Shan, A. D. Bond and W. Jones, Cryst. Eng., 2002, 5, 9 CrossRef CAS;
(d) C. B. Aakeröy, A. M. Beatty and B. A. Helfrich, J. Am. Chem. Soc., 2002, 124, 14
425;
(e) T. D. Hamilton, G. S. Papaefstathiou and L. R. MacGillivray, J. Am. Chem. Soc., 2002, 124, 11
606 CAS;
(f) G. S. Papaefstathiou and L. R. MacGillivray, Angew. Chem., Int. Ed., 2002, 41, 2070 CrossRef CAS;
(g) G. S. Papaefstathiou, A. J. Kipp and L. R. MacGillivray, Chem. Commun., 2001, 2462 RSC;
(h) F. Grepioni, S. Gladiali, L. Scaccianoce, P. Ribeiro and D. Braga, New J. Chem., 2001, 25, 690 RSC;
(i) L. R. MacGillivray, J. L. Reid and J. A. Ripmeester, J. Am. Chem. Soc., 2000, 122, 7817 CrossRef CAS;
(j) M. Tadokoro, H. Konno, T. Kitajima, H. Shimada-Umemoto, N. Nakanishi, K. Isobe and K. Nakasuji, Proc. Natl. Acad. Sci. USA, 2002, 99, 4950 CrossRef CAS;
(k) M. Tadokoro and K. Nakasuji, Cood. Chem. Rev., 2000, 198, 205 Search PubMed;
(l) A. D. Burrows, R. W. Harrington, M. F. Mahon and S. J. Teat, Eur. J. Inorg. Chem., 2003, 766 CrossRef CAS.
-
(a) R. Horikoshi, M. Ueda and T. Mochida, New J. Chem., 2003, 27, 933 RSC;
(b)
R. Horikoshi, C. Nambu and T. Mochida, Inorg. Chem., in press Search PubMed;
(c) R. Horikoshi, T. Mochida and H. Moriyama, Inorg. Chem., 2002, 41, 3017 CrossRef CAS;
(d) R. Horikoshi, T. Mochida, R. Torigoe and Y. Yamamoto, Eur. J. Inorg. Chem., 2002, 3197 CrossRef CAS;
(e)
R. Horikoshi, K. Hagiwara and T. Mochida, submitted.
-
(a) T. Tanaka, T. Tasaki and Y. Aoyama, J. Am. Chem. Soc., 2002, 124, 12
453 CAS;
(b) M. Naito, Y. Sasaki, T. Dewa, Y. Aoyama and Y. Okahara, J. Am. Chem. Soc., 2001, 123, 11
037 CrossRef CAS.
-
(a) L. R. Nassimbeni and H. Su, Acta Crystallogr., Sect. B., 2001, 57, 394 CrossRef CAS;
(b) C. J. Burchell, G. Ferguson, A. J. Lough, R. M. Gregson and C. Glidewell, Acta Crystallogr., Sect. B., 2001, 57, 329 CrossRef CAS;
(c) S. Fukushima, H. Hosomi, S. Ohba and F. Kawashima, Acta Crystallogr., Sect. C., 1999, 55, 120 CrossRef;
(d) Y. Dobashi, K. Kobayashi, N. Sato and A. Dobashi, Tetrahedron Lett., 1998, 39, 2985 CrossRef CAS;
(e) V. R. Pedireddi, S. Chatterjee, A. Ranganathan and C. N. R. Rao, Tetrahedron, 1998, 54, 9457 CrossRef CAS.
-
(a)
Ferrocenes: Homogenous Catalysis, Organic Synthesis, Materials Science, eds. A. Togni and T. Hayashi, Wiley-VCH, Weinheim, 1995 Search PubMed;
(b)
N. J. Long, Metallocenes: An Introduction to Sandwich Complexes, Blackwell Science Inc., Cambridge, MA, 1998 Search PubMed;
(c) P. Nguyen, P. Gómez-Elipe and I. Manners, Chem. Rev., 1999, 99, 1515 CrossRef CAS;
(d) R. D. A. Hudson, J. Organomet. Chem., 2001, 637–639, 47 CrossRef CAS.
-
(a) Z. Hou and Y. Wakatsuki, Coord. Chem. Rev., 2002, 211, 1 CrossRef CAS;
(b) H. Zhu, G.-X. Jin and N. Hu, J. Organomet. Chem., 2002, 655, 186 CrossRef.
-
(a) J. S. Millar, A. J. Epstein and W. M. Reiff, Acc. Chem. Res., 1988, 21, 114 CrossRef CAS;
(b) D. Ruiz-Molina, C. Sporer, K. Wurst, P. Jaitner and J. Veciana, Angew. Chem., Int. Ed., 2000, 39, 3688 CrossRef CAS.
-
(a) H. S. Nalwa, Appl. Organomet. Chem., 1991, 5, 349 CrossRef CAS;
(b) T. Farrell, T. Meyer-Friedrichsen, M. Malessa, D. Hasse, W. Saak, I. Asselberghs, K. Wostyn, K. Clays, A. Persoons, J. Heck and A. R. Manning, J.
Chem. Soc., Dalton Trans., 2001, 29 RSC.
-
(a) H. Plenio and C. Aberle, Chem.-Eur. J., 2001, 7, 4438 CrossRef CAS;
(b) M. C. B. Colbert, J, Lewis, N. J. Long, P. R. Raithby, A. J. P. White and D. J. Williams, J. Chem. Soc., Dalton Trans., 1997, 99 RSC;
(c) H. Plenio, C. Aberle, Y. A. Shihadeh, J. M. Lloris, R. Martínez-Máñez, T. Pardo and J. Soto, Chem.-Eur. J., 2001, 7, 2848 CrossRef CAS;
(d) T. Mochida, Mol. Cryst. Liq. Cryst., 2000, 343, 205 Search PubMed;
(e) J. Jiao, G. J. Long, F. Grandjean, A. M. Beatty and T. P. Fehlner, J. Am. Chem. Soc., 2003, 125, 7522 CrossRef CAS.
-
(a) D. Braga, L. Maini, M. Polito, L. Scaccianoce, G. Cojazzi and F. Grepioni, Coord. Chem. Rev., 2001, 216–217, 225 CrossRef;
(b) D. Braga and F. Grepioni, Acc. Chem. Res., 2000, 33, 601 CrossRef CAS;
(c) D. Braga and F. Grepioni, J. Chem. Soc., Dalton Trans., 1999, 1 RSC;
(d) D. Braga, O. Benedi, L. Maini and F. Greponi, J. Chem. Soc., Dalton Trans., 1999, 2611 RSC.
-
(a) D. M. Shin, Y. K. Chung and I. S. Lee, Cryst. Growth Des., 2002, 2, 493 Search PubMed;
(b) I. S. Lee and Y. K. Chung, Organometallics, 1999, 18, 5080 CrossRef CAS.
-
P. Schuster, G. Zundel and C. Sandorfy, The Hydrogen Bond, Recent Developments in Theory and Experiments, II. Structure and Spectroscopy, North-Holland Publishing Company, Amsterdam, 1976 Search PubMed.
- S. J. Grabowski and T. M. Krygowski, Chem. Phys. Let., 1999, 305, 247 Search PubMed.
-
(a) R. Liu, K.-F. Mok and S. Valiyaveettil, New J. Chem., 2001, 25, 890 RSC;
(b) K. Biradha and M. J. Zaworotko, J. Am. Chem. Soc., 1998, 120, 6431 CrossRef CAS.
- S. C. Wallwork and H. M. Powell, Acta Crystallogr., 1957, 10, 48 CrossRef CAS.
-
(a)
A. D. Davydov, Solitons in Molecular Systems, Reidel, Dordecht, Holland, 1985 Search PubMed;
(b)
Proton Transfer in Hydrogen-Bonded Systems, ed. T. Bountis, Plenum Press, New York, 1992 Search PubMed;
(c) M. Szafrański, A. Katrusiak and G. J. McIntyre, Phys. Rev. Lett., 2002, 89, 21
5507 CAS;
(d) M. Szafrański and A. Katrusiak, Chem. Phys. Lett., 2000, 318, 427 CrossRef CAS;
(e) A. Katrusiak and M. Szafrański, Phys. Rev. Lett., 1999, 82, 576 CrossRef CAS.
-
(a) O. Ermer and J. Neudörfl, Helv. Chim. Acta, 2001, 84, 1268 CrossRef CAS;
(b) O. Ermer and J. Neudörfl, Chem.-Eur. J., 2001, 7, 4961 CrossRef CAS;
(c) F. H. Herbstein, M. Kapon and V. Shteiman, Acta. Crystallogr., Sect. B, 2001, 57, 692 CrossRef CAS;
(d) R. E. Melendez, Angew. Chem., Int. Ed. Engl., 1995, 34, 2654 CrossRef CAS;
(e) K. Biradha, D. Dennis, V. A. MacKinnon, C. V. K. Sharma and M. J. Zawortoko, J. Am. Chem. Soc., 1998, 120, 11
894 CAS;
(f) S. Kolotuchin, C. V. K. Sharma, M. J. Zawortoko, C. Bauer and R. D. Rogers, Angew. Chem., Int. Ed. Engl., 1996, 35, 2213 CrossRef CAS;
(g) B. R, Bhogala and A. Nangia, Cryst. Growth Des., 2003, 3, 547 Search PubMed;
(h) B. R, Bhogala, P. Vishweshwar and A. Nangia, Cryst. Growth Des., 2002, 2, 325 Search PubMed.
- Z. M. Jin, Y. J. Pan, L. Shen, M. C. Li and M. L. Hu, Acta. Crystallogr. Sect. C, 2003, 59, o205 CrossRef.
-
teXsan: Crystal Structure Analysis Package, Molecular Structure Corporation, The Woodlands, TX, USA, 1985 and 1999 Search PubMed.
- A. Altomare, M. C. Burla, M. Camalli, M. Cascarano, C. Giacovazzo, A. Guagliardi and G. Polidori, J. Appl. Crystallogr., 1994, 27, 435 CrossRef.
|
This journal is © The Royal Society of Chemistry and the Centre National de la Recherche Scientifique 2004 |
Click here to see how this site uses Cookies. View our privacy policy here.