Terrestrial ecosystems, increased solar ultraviolet radiation and interactions with other climatic change factors†
First published on 10th January 2003
Abstract
Based on research to date, we can state some expectations about terrestrial ecosystem response as several elements of global climate change develop in coming decades. Higher plant species will vary considerably in their response to elevated UV-B radiation, but the most common general effects are reductions in height of plants, decreased shoot mass if ozone reduction is severe, increased quantities of some phenolics in plant tissues and, perhaps, reductions in foliage area. In some cases, the common growth responses may be lessened by increasing CO2 concentrations. However, changes in chemistry of plant tissues will generally not be reversed by elevated CO2. Among other things, changes in plant tissue chemistry induced by enhanced UV-B may reduce consumption of plant tissues by insects and other herbivores, although occasionally consumption may be increased. Pathogen attack on plants may be increased or decreased as a consequence of elevated UV-B, in combination with other climatic changes. This may be affected both by alterations in plant chemistry and direct damage to some pathogens. Water limitation may decrease the sensitivity of some agricultural plants to UV-B, but for vegetation in other habitats, this may not apply. With global warming, the repair of some types of UV damage may be improved, but several other interactions between warming and enhanced UV-B may occur. For example, even though warming may lead to fewer killing frosts, with enhanced UV-B and elevated CO2 levels, some plant species may have increased sensitivity to frost damage.
Introduction
Several environmental changes are being imposed on terrestrial ecosystems, including increased solar ultraviolet-B radiation and warming at higher latitudes, increasing carbon dioxide levels globally, and regional tropospheric air pollution and atmospheric nitrogen deposition. Potentially significant changes in the frequency and nature of precipitation and storms are also predicted as the Earth warms. Depending on location, many of these factors will exert their influence on ecosystems more or less concurrently. Terrestrial ecosystems include agricultural lands (agroecosystems), less intensively managed lands such as forests, grasslands, and savannahs, and unmanaged lands such as deserts, tundra, etc. This overview addresses how increased UV-B radiation, interacting with other global change factors, may affect many of the important ecosystem processes and attributes, such as plant biomass production, plant consumption by herbivores including insects, disease incidence of plants and animals, changes in species abundance and composition, and mineral nutrient cycling. Some aspects of ecosystem function, e.g., nutrient cycling, are treated in more detail in this volume.1
The present report consists of a brief update of our understanding of UV radiation effects, followed by coverage of factor interactions as much as they have been researched to date (Fig. 1). Ideally, these interactions should be considered across, as well as within, trophic levels. Trophic level refers to groups of organisms constituting different stages of the food chain in an ecosystem, e.g., primary producers (plants), various levels of consumers (herbivores, carnivores, etc.), and decomposer organisms.
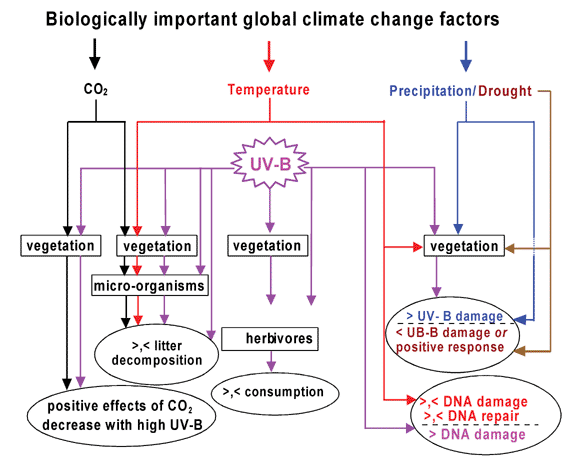 |
| Fig. 1 Major interactions of elevated UV-B with other climate change factors in terrestrial ecosystems. Lines indicate influence of climate change factors on different trophic levels (in rectangles) that affect processes (in ovals). Colors of lines: black, elevated CO2; red, elevated temperature; violet, enhanced UV-B; blue and brown, abundance and deficit of moisture, respectively. The symbols > and < refer to more and less, respectively. | |
It is now some 30 years since the first suggestions of stratospheric ozone reduction appeared (e.g., refs. 2 and 3). Within a few years of these early concerns about the atmosphere, several studies of UV-B effects on higher plants appeared and these continue to represent the emphasis of research in this area. Direct effects of UV-B on insects and other terrestrial animal life have traditionally received comparatively little attention as they are often assumed to be protected from damaging effects of solar UV either by their behavioral patterns or by largely UV-opaque body coverings (e.g., fur, feathers, exoskeletons of insects, etc.). Terrestrial microbes have also received little attention though they are usually poorly shielded from penetrating solar UV. In recent years, more attention has been paid to UV-B influence on species interactions in an ecosystem context.
Biologically effective UV radiation for plants
The concept of biologically effective UV radiation has been discussed.4 This involves weighting the radiation at different wavelengths with a factor to indicate its relative biological effectiveness. An example of erythemal (human sunburn) effectiveness is given in this volume.4 For other biological effects, different weighting functions appear to be more appropriate. Earlier reports of this panel (e.g., refs. 5 and 6) have portrayed the importance of different assumptions of the wavelength dependency of UV effects on plants and other organisms. These dependencies involve different degrees to which longer wavelength UV (UV-A) participates in various UV effects on plants. There are also important implications of this in calculating biologically effective UV based on different degrees of ozone reduction (see radiation amplification factors, this volume4) and in evaluating experiments with lamps to supplement the UV. A radiation amplification factor (RAF) refers to the relative increase of biologically effective UV radiation for each increment of ozone column change.4 The greater the degree to which longer wavelengths in the UV-B and UV-A are effective in biological reactions, the smaller is the RAF, i.e., less of an increase in biologically effective radiation for a given level of ozone reduction.7,8 Biological weighting functions are also used to compare the biologically effective UV radiation from lamp systems with that from the Sun due to ozone reduction, since these UV lamps do not accurately simulate solar radiation.7
A new action spectrum for characteristics of higher plant growth and morphology indicates that UV-A participates in these effects more than originally assumed in earlier action spectra.9 The new spectrum resembles the commonly used generalized plant response function10 except that it indicates appreciable sensitivity into the UV-A. This spectrum was also tested by exposing plants in the field to different combinations of solar and artificial radiation and this indicated that the new spectrum is relevant under field conditions.11 When used for the ozone reduction problem, this new spectrum predicts less of an increase of biologically effective radiation for a given decrease in ozone thickness (lower RAF) than the generalized plant spectrum. However, it also suggests that the levels of ozone reduction simulated in lamp experiments, often adjusted according to the generalized plant spectrum, results in less simulated ozone reduction than if computed using the new spectrum. For example, if one used the generalized plant spectrum to adjust lamps to result in a 30% simulated ozone reduction, this would only be an 8% ozone reduction if the resulting supplemental radiation is calculated according to the new plant growth spectrum.11
Plant growth is a complex response that integrates the influences of many environmental factors, including UV-B, on several physiological processes. It is worth pointing out that the new spectrum that describes the wavelength dependency of growth inhibition may not be appropriate to describe the spectral response of individual physiological processes. The shape of the spectra for individual processes will depend on the particular chromophores involved and, among other factors, the optical shielding imposed on these chromophores by surrounding molecules and cellular structures. Little work has been carried out to define appropriate weighting functions for individual responses under physiologically meaningful conditions. For DNA damage, various action spectra were tested in the field in southern Argentina as the Antarctic “ozone hole” passed over the experimental site and altered the wavelength composition of the solar radiation. The comparisons of plant DNA damage responses with different action spectra indicated an action spectrum with little participation of UV-A as the most appropriate12 Similarly, Mazza et al.13 found that the accumulation of phenolic sunscreens in field-grown soybeans was significantly enhanced by solar UV-B, whereas the UV-A component of sunlight had little effect. Therefore, for this particular response, a steep action spectrum also appeared to provide a good description of wavelength dependency.
A recent view of enhanced UV-B effects on plants: A synthesis using meta-analysis
Most UV-B research on terrestrial ecosystems continues to be focused on plants and emphasizes experiments addressing the sequence of events upon exposure to levels of UV-B radiation corresponding to stratospheric ozone reduction under outdoor conditions. It was recognized long ago, that if such experiments were conducted in glasshouse or growth-cabinet conditions, the effects of the added UV-B radiation were greatly exaggerated. Thus, over 20 years ago investigators began to conduct such experiments outdoors using special UV lamp systems. There are now well over 100 such studies on different species of plants. Meta-analysis is a technique to use quantitative and statistical information provided in a collection of individual studies in a combined analysis to assess how well the overall research predicts common trends and results. Of the ca. 100 studies reviewed, 62 provided enough quantitative information suitable for a meta-analysis14 for several types of managed and unmanaged ecosystems (Fig. 2).
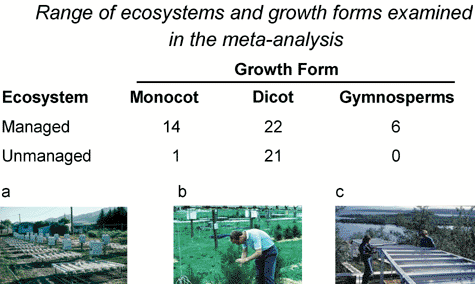 |
| Fig. 2 Types of vegetation examined in the meta-analysis. The numbers refer to studies for individual species. Photographs of three different systems are also shown (a. wheat and wild oat experiments in Logan, Utah, USA, W. Beyschlag; b. Loblolly pine in Maryland, USA, A. Teramura; c. Subarctic heath in Sweden, from ref. 5). | |
Of the 10 physiological and morphological traits examined, overall significance of elevated UV-B in the meta-analysis could only be concluded for shoot mass, plant height and leaf area and increased UV-B absorbing pigments (including flavonoids and other phenolic compounds). The other traits, including changes in chlorophyll and carotenoid pigments, reproductive yield, leaf mass per unit leaf area, net photosynthesis, and photosystem II activity of the photosynthetic system, might have been affected in some individual studies, but the overall effect was not sufficiently robust to be significant in the meta-analysis. For the four characteristics that were found to be significant, Fig. 3 shows the array of responses in individual studies to elevated UV-B relative to controls. For leaf area, the significant reduction was caused by the manner in which the experimental replicate was selected. When authors selected the individual plant as the replicate, the average response was significant. (These data are included in Fig. 3.) When they selected the plot as the replicate, the response was not significant.14 For shoot biomass, there are two arrays of studies shown in Fig. 3 corresponding to the level of ozone reduction being simulated, 10–20% and >20%. Only the group of studies simulating >20% ozone reduction yielded a significant average response in the meta-analysis for shoot mass. (In these studies, the level of simulated ozone reduction was usually effected by adjusting the output of the UV lamps with the old generalized plant spectrum.10 Had the new plant growth spectrum9 been used, the levels of simulated ozone reduction would have been much smaller, as explained in the foregoing section.)
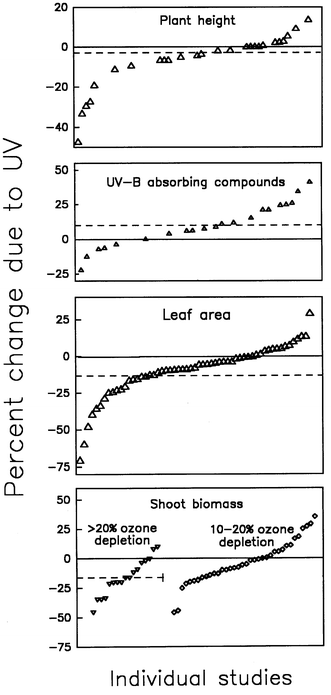 |
| Fig. 3 The response of four plant characteristics in field studies in the order of increasing positive effects. These experiments all employed supplemental UV-B from lamp systems. Each symbol represents a different study. The dashed line in each represents the average response calculated by the meta-analysis over all studies shown. The average responses shown were significant at P < 0.05. For shoot mass, the studies are grouped into two arrays corresponding to studies in which the level of simulated stratospheric ozone reduction is between 10 and 20%, and those in which the simulated ozone reduction was greater than 20%. | |
Although all these studies in the meta-analysis involved lamp systems under outdoor conditions in natural sunlight, the methods included different degrees of replication and control of the lamp intensities. Most of these studies employed an “on–off” system of lamp control, sometimes called “square-wave”, while others used more elaborate control that gradually changed lamp output according to ambient solar UV-B, sometimes called “modulated” control. Thus, all studies are not of equivalent quality; they also do not have equal capability to discriminate plant responses. While this should be borne in mind, there is increased value in comparing a large number of such experiments through meta-analysis, since it affords a quantitative comparison.
Apart from experiments with UV-emitting lamps, there is a lesser number of experiments using special filters that remove, or attenuate the UV-B in normal sunlight (along with appropriate control filters that are largely transparent to UV-B). In the majority of cases, when sunlight UV-B was attenuated, plants exhibited better growth which indicates that normal sunlight UV-B reduces growth to some extent.15–17 Attenuation experiments have been carried out in areas that are currently exposed to enhanced solar UV-B levels, such as the southern tip of South America (Tierra del Fuego, Argentina) and on the Antarctic Peninsula.18,19 Herbaceous plants native to both regions were negatively affected by the ambient solar UV-B levels. A comparison between the growth inhibition data collected in these UV-B attenuation experiments carried out in Tierra del Fuego and the Antarctic Peninsula showed that a similar fractional level of UV-B attenuation by filters (approximately 80%) had effects on plant growth that increased with the level of ozone depletion (i.e., from Tierra del Fuego to Antarctica).18
As can be seen in Fig. 3, some UV-B lamp studies reported enhancement in some plant characteristics (plant height, leaf area, and shoot mass), although most studies reported decreases in these characteristics. There are also a few recent reports of filter studies where solar UV-B promoted plant growth.20,21 Mechanisms mediating this apparent enhancement of growth are not known and it is not clear that this would occur over the long term in a natural setting.
Apart from higher plant responses, other effects of enhanced UV-B radiation on terrestrial ecosystems are represented by too few studies to allow for meta-analysis.
Do small effects of UV-B accumulate through the years?
An intriguing, and potentially important, phenomenon suggested by some earlier research is that even small effects of UV-B radiation might accumulate to produce larger effects in subsequent years in perennial plants. The first suggestions of this were in seedlings of one of four seed sources of a conifer. In these plants, the effect of exposing the plants to elevated UV-B from lamps became progressively more expressed in subsequent years.22 However, this did not appear to be significant in young trees tested from the other three seed sources. Indications of cumulative effects of elevated UV-B in Subarctic heath perennials were apparent for some traits of some species, but not for others (e.g., ref. 23). Also, early indications of cumulative effects disappeared over a longer period of time.24 Thus, while there may be some indications of this accumulation phenomenon in certain specific instances, there is no convincing evidence for it as a general trend. However, its potential significance should not be dismissed, since it might affect competitive persistence of some species through time.
Analogous to these putative cumulative effects, an apparent carry-over and accumulation of elevated UV-B effects on plant growth form, from generation to generation, have been reported for a desert annual plant species.25,26 If this is a widespread phenomenon among species, an amplification of sorts might be effected. The mechanism for this is not understood.
Although suspected for some time, there are now a few new reports indicating that enhanced UV-B may affect the genetic stability of plants causing long-term heritable effects, with a high frequency of mutations which are generally considered to be deleterious to organisms. High UV-B exposure can activate what is known as “mutator transposons” in maize that amplify the mutation effect of the UV-B beyond the immediate DNA damage.27 In Arabidopsis, increased UV-B was found not only to cause direct DNA damage; but also, errors in DNA repair leading to an increased tendency for mutations in subsequent generations.28,29 In cyanobacteria, considerable genetic polymorphism was found in highly stressful environments, and was presumed to be caused by UV-B-induced genome instability and replication errors.30 These instabilities could affect future generations, and result in an increased mutation rate even after the ozone layer has recovered.
Recent reports of UV-B effects on soil and soil surface processes such as nitrogen fixation and litter decomposition suggest that these changes are not transient phenomena. Elevated UV-B treatments applied over several years may become apparent years after the treatments had been initiated. This was the case for depression of nitrogen fixation in some species of cyanobacteria31 that had been exposed to elevated UV-B for several years in both high Arctic and Subarctic locations. A one year exposure to elevated UV-B became apparent four years later as accelerated decomposition of oak leaf litter.32 Both nitrogen fixation and litter decomposition are nutrient cycling processes that are important in ecosystem function.
Insect herbivory of plants
Insects have enormous potential to consume vegetation, but the degree to which they feed on different species is dependent not only on the species of plants, but also on a suite of other environmental factors, including UV-B radiation. There are now over 20 reports of various insect–plant species combinations that have been studied with respect to the influence of UV-B radiation (Table 1).
Table 1 Effects of UV-B radiation on insect herbivory
Insect |
Type of expt.a |
Plant species |
UV-B effect on herbivory/insects |
Possible mechanisme |
Study |
C = controlled environment chamber, G = greenhouse, F = field UV-B supplement from lamps, E = field UV-B exclusion.
Field-treated material used in laboratory feeding trials.
More herbivory under UV-A and UV-B lamps compared to controls, but no specific UV-B effect.
UV-A and UV-B responses cannot be separated.
“Indirect effect” implies that an UV-B effect mediated by changes in the plant was demonstrated in a bioassay, even if the nature of the changes was not identified. (C), “choice” bioassay, (NC)
“no choice” bioassay.
|
Caliothrips phaseoli
(thrips) |
E |
Glycine max
(soybean) |
Less herbivory33 |
Direct response of insects to solar UV-B |
34
|
Diabrotica speciosa
(leaf beetle), lepidopteran larvae, grasshoppers |
E |
Glycine max
(soybean) |
Less herbivory |
|
35
|
Anticarsia gemmatilis
(moth larva) |
Eb |
Glycine max
(soybean) |
Slower growth, higher mortality |
Indirect effect. (NC)e
Increased phenolics but decreased lignin
|
|
Schistocera gregaria
(desert locust) |
Fb |
Lolium perenne, Festuca rubra, F. arundinaceae, F. pratensis
|
No response in 3 species; in Festuca pratensis, preference for endophyte-infected plants changed |
Indirect effect. (C)e
Loline content changed, but this did not influence herbivory
|
36
|
Various chewing insects (not identified) |
E |
Gunnera magellanica
(devil's strawberry) |
Less herbivory |
Not known |
37
|
Spodoptera litura, Graphania mutans
(moth larva) |
C |
Trifolium repens
(white clover) |
Tendency toward slight reduction in herbivory |
Indirect effect. (NC)
Slight N increase, larger carbohydrate decrease, population-specific changes in cyanogenesis
|
38
|
Epirrita antumnata
(moth larva) |
F |
Betula pubescens
(mountain birch) |
More herbivory |
Mechanism not known. (NC)
Laboratory study indicated direct UV-B preference
|
39
|
Precis coenia, Trichoplusia ni
(both lepidopteran larvae) |
G |
Plantago lanceolata
(English plantain) |
Precis—no effect
Trichoplusia—more growth from eating treated material but direct UV-B growth inhibition
|
Direct inhibitory effect of UV-B on insect growth; indirect effects. (NC)
Reduced crown and reproductive growth; some increase in leaf N and verbascosides
|
40
|
Various chewing insects (not identified) |
F |
Quercus robur
(pedunculate oak) |
No UV-B effectc |
|
41
|
Caliothrips phaseoli
(thrips) |
E |
Glycine max
(soybean) |
Less herbivory |
Indirect effect (C) and direct UV-B avoidance |
33,34
|
Lepidoptera: Noctuidae
(moth larva) |
E |
Gunnera magellanica
(devil's strawberry) |
Less herbivory |
Indirect effect. (C)
Increase in leaf N
|
42
|
Strophingia ericae
(psyllid) |
F |
Calluna vulgaris
(heather) |
Reduced insect populations |
Not known. Reduced amino acid isoleucine |
43
|
Operophtera brumata
(moth larva) |
G |
Betula pendula
(silver birch) |
More herbivory |
Indirect effect. (C)
Leaf flavonoids increased, but flavonoids added to an artificial diet did not increase feeding.
|
44
|
Insects not identified |
F |
Vaccinium myrtillus, V. uliginosum, V. vitis-idaea
(heathland shrubs) |
More herbivory in V. myrtillus, less in V. uliginosum, no effect in V. vitis-idaea |
Mechanism not known |
45,46
|
Pieris rapae, (butterfly larva)
Trichoplusia ni
(moth larva) |
C |
Arabidopsis thaliana
|
Pieris: less herbivory and less insect weight gain |
Indirect effect. (NC)
Leaf flavonoids increased
|
47
|
Coleoptera
(leaf beetles) |
E |
Datura ferox
(summer annual) |
Less herbivory |
Indirect effect. (C)
Mechanism not known
|
48
|
Acronicta, Nycteola, Orthosia, Ptiloden
(moth larva) |
F |
Quercus robur
(pedunculate oak) |
No specific UV-B effectc |
|
49
|
Autographa gamma
(moth larva) |
C |
Pisum sativum
(pea) |
Less herbivory, but greater insect growth |
Indirect effect. (NC)
Higher phenolic and N contents
|
50
|
Ostrinia nubilalis
(European corn borer) |
Ed |
Zea mays
(corn) |
Less herbivory |
Indirect effect. (NC)
More cell-wall-bound truxillic and truxinic acids
|
51
b
|
Trichoplusia ni
(moth larva) |
G |
Citrus jambhiri
(rough lemon) |
Decrease in survivorship and growth |
UV-B increased furanocoumarin levels |
52
|
Generally, when there was an effect, a higher level of UV-B led to less insect herbivory and/or reduced insect growth compared to lower levels of UV-B. (Most of these experiments were conducted by filtering ambient solar radiation.) The magnitude of the effects can be sizeable, with potential ecosystem-level consequences for species composition, organic matter decomposition and nutrient cycling. In several cases, it was possible to show, using feeding bioassays, that the effects of the UV-B radiation were mediated through the host plant, i.e., they were the result of UV-induced changes in the characteristics of the plant tissues. Two types of bioassays have been used: In the “no choice” bioassays (NC, in Table 1) insects were given either UV-B-exposed or control plant material (exposed to less, or no UV-B), whereas in the “choice”
(C) bioassays the insects received both types of pretreated plant materials in the same feeding area. Of course, the interpretation of the response (altered herbivory or insect performance) in the context of projecting ecosystem-level consequences requires consideration of the type of experiment that was used to detect UV-B effects on plant–insect interactions. It is also important to point out that the nature of the UV-B-induced changes in plant characteristics that cause herbivory responses is not known for any system; the possible mechanisms listed in Table 1 are based on correlative, circumstantial evidence. Insects are generally thought to be blind to variations in the UV-B component of sunlight, since their visual systems are primarily sensitive to UV-A radiation. However, one field study showed that a species of thrips can perceive and avoid solar UV-B under natural daylight conditions34, and laboratory study showed behavioral responses to artificial UV-B in a moth caterpillar.39
Bacteria and fungi
Fungi and bacteria play crucial roles in ecosystem function including decomposition of dead biological material, mineral nutrient cycling and as pathogens of plants and animals. In the last few years, more attention has been paid to direct UV-B effects on these microbes if they are exposed to sunlight (such as on foliage surfaces or litter). Changes in species composition and biodiversity of these microbes in response to UV-B have been documented and many of these changes appear to be related to how well species and strains of these fungi and bacteria tolerate UV.53–56 Beneficial fungi that infect plant roots and assist in absorption of nutrients (termed mycorrhizae), although not exposed to solar radiation, might be indirectly affected by UV-B exposure of the host plant shoots.57,58 This would need to be mediated by systemic tissue changes in the roots caused by UV-B exposure of the shoots.
Bacteria and fungi can also be pathogenic for both plants and animals, although plant pathogens have received more attention than animal pathogens with respect to UV-B radiation. As compiled by Paul,59 plant disease incidence can be increased or reduced by UV-B radiation. Increasing disease severity is thought to primarily involve modifications in the host plant tissues, while decreased severity appears due either to host plant changes or direct UV-B damage to the pathogen (Table 2).
Table 2 Effects of UV-B radiation on plant-microbe interactions for living plants and plant litter
A. Experiments on live plants |
Microbe genus |
Type of expt.a |
Plant species |
Fungal response to UV-B |
Plant response to UV-B |
Study |
C = controlled environment chamber, G = greenhouse, F = field UV-B supplement from lamps, E = field UV-B exclusion.
All or some decomposition conducted in laboratory.
|
Aureobasidium
(phylloplane yeast) |
E |
Nothofagus antarctica (southern beech)
|
Proportionately less on upper leaf surface, several other plant-microbe systems not affected |
None |
53
|
Bacteria (e.g.Clavibacter) |
E |
Arachis hypogene
(peanut) |
Altered species composition |
None |
55
|
Microsphaera
(powdery mildew pathogen) |
F |
Quercus robur
(pedunculate oak) |
Increased infection |
Photosynthesis decreased due to increased mildew |
60
|
Neotyphodium
(leaf endophyte) |
F |
Lolium perenne
(rye grass) |
No effect |
Reduced yield in the presence of the leaf endophyte |
61
|
Septoria
(leaf blotch pathogen) |
C |
Triticum aestivum
(wheat) |
Fewer lesions on plant (a direct response to UV-B) or no effect depending on time of year |
Not assessed. If changes occurred, they did not affect the pathogen |
62
|
Exobasidium
(blister blight pathogen) |
E |
Camellia sinensis
(tea) |
Reduced infection sites; no effect on sporulation |
Not assessed |
63
|
Aureobasidium, Sporobolomyces
(phylloplane yeasts) |
F |
Quercus robur
(pedunculate oak) |
Abundance on the upper; but not lower leaf surface affected for some sampling dates. Several other fungi not affected |
Not assessed |
64
|
Fusarium
(damping-off pathogen) |
C |
Spinacia oleracea
(spinach) |
More damping off evident |
Decreased shoot growth |
65
|
Bullera
(phylloplane yeasts) |
E |
Vicia faba
(faba bean), Malus domestica
(apple), Quercus robur
(pedunculate oak), Pisum sativum
(pea) |
Less colony forming units isolated from leaves |
Not assessed |
66
|
Pyricularia
(also known as Magnaporthe)
(rice blast pathogen) |
G |
Oryza sativa
(rice) |
Greater lesions on plant in a few cases (an indirect UV-B effect mediated through the plant) |
Reduced plant height, leaf area, dry weight in a few cases |
67
|
Cercospora
(leaf spot pathogen) |
C |
Beta vulgaris
(sugar beet) |
Not assessed |
Reduced dry weight of leaf laminae and other plant parts in the presence of the pathogen |
68
|
Colletotrichum, Cladosporium
(anthracnose and scab pathogens) |
G |
Cucumis sativus
(cucumber) |
Increased infection in some cases (an indirect UV-B effect mediated through the plant) |
Reduced plant height, leaf area, dry weight; increased leaf mass per area |
69
|
Puccinia
(leaf rust pathogen) |
F |
Triticum aestivum
(wheat) |
Increased infection with results varying some by wheat cultivar |
Little; if any, reduction in dry weight and seed yield |
70
|
Diplocarpon
(blackspot pathogen) |
G |
Rosa
(rose) |
Inhibition only when conidia were germinating |
No response |
71
|
Potato virus S |
G |
Chenopodium quinoa
|
Fewer lesions |
No response |
72
|
|
B. Experiments on plant litter |
Fungal genus |
Type of expt.a |
Plant species |
Fungal response to UV-B |
Plant response to UV-B |
Study |
Cladosporium, Cystodendron, Phoma
|
F |
Betula pubescens
(mountain birch) |
Changes in fungal community structure |
Live plants received no treatments |
73
|
Aspergillus, Cladosporium, Epicoccum
|
Fb |
Brassica napus
(oil seed rape) |
Direct and indirect effects on fungal competitive ability |
Increased flavonoids |
74
|
Cladosporium, Acremonium, and others (saprotrophs) |
F |
Quercus robur
(pedunculate oak) |
Reduced fungal colonization of decomposing leaves; change in fungal species composition |
Some transitory UV-B effect on mass loss of decomposing litter |
75
|
Mucor, Truncatella, Penicillium
(saprotrophs) |
Fb |
Vaccinium uliginosum
(a heathland shrub) |
Reduced fungal colonization of decomposing leaves; change in fungal species composition |
Altered leaf litter quality |
76
|
Pathogens of insects and other animals may also be influenced by solar UV radiation. Studies involving biological control of insect pests using pathogens provide some indication of how solar UV may affect insect pathogens. For example, Braga et al.77,78 showed that fungal strains of an insect pathogen were sensitive to solar UV-B radiation and to lamp UV-B in a range corresponding to that in sunlight. These particular fungi commonly used in biological control of insects such as grasshoppers were isolated from soil fungi that would not normally be exposed to sunlight. However, if strains isolated from locations at different latitudes were exposed to UV-B, their relative UV-B sensitivity corresponded to the respective latitudinal differences in solar UV-B at their sites of origin. Viral pathogens of insects are also inhibited by UV-B radiation79 Thus, as used for biological control, attention to UV-B sensitivity is necessary and enhanced UV-B stemming from ozone reduction would further limit their usefulness.
Global environmental changes
The changing environment we are now witnessing and will likely experience in the coming decades involves both global climate change and broad regional changes. Both the predictability and the rate of these environmental changes vary widely. Solar UV-B has increased at higher latitudes, and CO2 and temperature are continuing to increase globally. Regionally, nitrogen deposition and tropospheric ozone have increased. Changes in regional precipitation frequency and weather systems are driven by global climate change and are highly important, but less well understood and predicted.
Thus, all the foregoing changes need to be considered as acting in concert with stratospheric ozone reduction. Experimental work is progressing, usually with two-factor interactions, e.g., elevated CO2 and UV-B. However, the complications and costs of two- or multi-factor experiments clearly limit how representative and comprehensive such experiments can be.
Drawing on the existing experimental data base, we attempt to generalize how elevated UV-B might interact with each of the other factors with respect to vegetation and ecosystem responses. For example, some combinations of factors appear to have largely additive effects, although these can operate in opposite directions. There can also be significant interactions, i.e., at different levels of one factor there is a non-additive response to a second factor (synergistic). The following sections contain such generalizations.
Elevated CO2 and UV-B
Several studies are now available in which plants, and sometimes combinations of plants and insect herbivores, were subjected to combinations of two or more levels of CO2 and UV-B. Typically, elevated CO2 treatments involved a doubling of CO2 and elevated UV-B treatments corresponded to a simulated 15 to 30% ozone depletion (assuming the generalized plant spectrum10 as explained earlier). Generally, if either elevated CO2 or UV-B exerted effects, the CO2 influence was more pronounced than that of the enhanced UV-B. Also, the effects of elevated CO2 and enhanced UV-B caused responses in opposite directions, e.g., additional CO2 stimulated plant growth and enhanced UV-B tended to depress growth.46,69,80–89 In all these studies, the effects of the two factors were usually counteractive, as just described. Synergistic effects were rare.
Combined application of UV-B and elevated CO2 followed by short exposures to ozone, as would be experienced from regional air pollution, resulted in interesting interactions. The stimulating effect of elevated CO2 on plant growth was apparent when plants were exposed to ambient (normal) UV-B. If given both elevated UV-B and high CO2, the stimulating effect of the high CO2 was reduced. These patterns are typical of the combined effects of elevated UV-B and high CO2. Application of a high ozone exposure following growth in the combinations of UV-B and CO2 eliminated the stimulating effect of high CO2 if the plants had been exposed to low UV-B, but did not if the plants had been given elevated UV-B along with the elevated CO2.86
Apart from plant growth and related processes like photosynthesis, the attractiveness of plant foliage to insect herbivores may vary under a combination of elevated CO2 and enhanced UV-B. Lavola et al.90 found that insects preferred plants grown with enhanced UV-B, contrary to the general trend described above, and the combination of high CO2 and enhanced UV-B led to even further tendency of the insects to consume foliage. In another study of this nature, enhanced UV-B either increased or reduced herbivory, depending on the plant species, but if the enhanced UV-B were combined with high CO2, or if the plants were given just high CO2 by itself, there was no effect on herbivory relative to controls (low UV-B and normal CO2).46
Climate change will likely result in modifications in the timing and amount of precipitation on a regional scale, although predicting these changes is difficult. Experiments to date largely suggest that if enhanced UV-B is applied to plants undergoing drought stress, the UV-B response seen with adequately watered plants is usually dampened (e.g., refs. 91–93), at least where cultivated plants are concerned. However, some species native to the Mediterranean seemed to thrive during periods of water limitation if exposed to elevated UV-B.94
Atmospheric nitrogen (N) deposition in many regions leads to N fertilization of vegetation. Nitrogen supply to vegetation has received relatively little attention in relation to UV-B radiation. One study involving a combination of different levels of N supply and two levels of UV-B showed that as cucumber plants received more N, their growth was depressed to a greater degree by elevated UV-B.95 If N deficient, these plants were not responsive to UV-B in that study.
Warming and UV-B
The Earth is warming and this is especially apparent in many regions at higher latitudes. These are also the latitudes where ozone depletion is more pronounced. Some responses to the combination of these factors might be rather predictable, such as increased repair (an enzymatic process) of DNA damage at higher temperatures. This has been experimentally demonstrated in terrestrial plants for DNA damage (manifest as DNA dimers). There was little repair of DNA damage at low temperatures and very adequate repair in a temperature range of 24–30 °C.96,97 The effectiveness of DNA repair was low at relatively high temperature.97,98
Of course, temperatures that are above or below the optimum for a particular organism can limit performance and, in the extreme, cause direct damage. Interactions among factors can occur such that the limits of temperature tolerance are altered. A study on Subarctic heath species showed that enhanced UV-B considerably increased the frost sensitivity in three of four species tested.99 In one species, this meant that the lower temperature limit of frost tolerance for some plants was 5 °C higher than for plants not given elevated UV-B. Furthermore, elevated CO2 led to an increase in frost sensitivity of these species and if both elevated CO2 and enhanced UV-B were applied, there was a further increase in frost sensitivity. Thus, even with warming at high latitudes, the frost damage to some of the plant species may be increased at higher CO2 and UV-B.99
When low temperatures are generally limiting growth or other processes, but not causing damage, warming should render a benefit to growth, but the degree to which this interacts with different levels of UV-B is not well understood. As appears to be the case with combinations of elevated CO2 and enhanced UV-B, the effects of warming and UV-B usually exhibit few synergistic effects, based on the available experiments. This is the case for the response of Antarctic plants to combinations of warming and UV-B manipulations.100,101 However, at high temperatures, some synergistic effects of enhanced UV-B and the elevated temperatures have been reported. In some tropical legumes, enhanced UV-B reduced growth of the plants at moderate temperatures (20 to 30 °C), but at 40 °C, chloroplasts in the leaves were modified and this masked UV-B depressions of growth (e.g., refs. 102 and 103).
Interaction of global climate change factors across trophic levels
The interactions addressed above largely involve the effects of factor combinations on a single trophic level, usually higher plants. However, environmental factors may also interact by affecting different components of the same trophic chain (Fig. 1). For example, plant–insect interactions may be affected by the responses of both plants and insects to changes in UV-B, temperature, and precipitation patterns. Enhanced UV-B can affect the quality of plant foliage as a food source for insect herbivores and this has been shown in several studies (see earlier section). Of the climatic change factors, insects themselves are primarily affected by temperature104 and changes in the frequency and patterns of precipitation.105 These factors may exert a direct influence on the insect, and also indirect effects mediated through changes and seasonal timing of the vegetation. Thus, factor interactions across trophic levels might involve warming, altering plant and insect seasonal timing104, and enhanced UV-B affecting the quality of plant tissue for insect consumption. The micro-organisms that are responsible for decomposing dead plant and animal materials constitute another trophic level. Warming may accelerate decomposition, given sufficient moisture, although UV-B exposure of vegetation when alive can change the decomposability of the plant material after senescence and death.76 Furthermore, UV-B can also directly affect the microbes decomposing the plant litter if they are exposed to sunlight.76,106 Thus, climatic change factors may affect or operate at different trophic levels, thereby complicating the analysis resulting from single trophic level studies.
Climate change over a longer span of time will also affect the geographic distribution of vegetation and animal populations.107 Migration of different species in response to warming can involve shifts to higher latitudes and altitudes108–110, which in turn would change their exposure to prevailing solar UV-B in these new locations.
Concluding remarks
This assessment emphasizes generalizations that can be made about the effects of enhanced UV-B on terrestrial ecosystems and those of UV-B when interacting with other climatic factors. Clearly, as with any generalization, exceptions are to be found and the generalizations necessarily involve simplifications. Also, when moving from effects of UV radiation and other factors at single trophic levels (such as higher plants) to whole-system function, numerous complications arise and experimentation becomes more difficult and costly. Nevertheless, progress is being made and we feel the conclusions drawn here are realistic.
References
-
R. G. Zepp, T. V. Callaghan and D. J. Erickson, Interactive effects of ozone depletion and climate change on biogeochemical cyclesPhotochem. Photobiol. Sci., 2003, this issue (DOI: 10.1039/b211154n) Search PubMed.
- H. Johnston, Reduction of stratospheric ozone by nitrogen oxide catalysts from supersonic transport exhaust, Science, 1971, 173, 517–522 CAS.
- P. J. Crutzen, SSTs—a threat to the Earth's ozone shield, Ambio, 1972, 1, 41–51 Search PubMed.
-
R. L. McKenzie, L. O. Björn, A. Bais and M. Ilyas, Changes in biologically active ultraviolet radiation reaching the Earth's surfacePhotochem. Photobiol. Sci., 2003, in press Search PubMed.
- M. M. Caldwell, A. H. Teramura, M. Tevini, J. F. Bornman, L. O. Björn and G. Kulandaivelu, Effects of increased solar ultraviolet radiation on terrestrial plants, Ambio, 1995, 24, 166–173 Search PubMed.
- M. M. Caldwell, L. O. Björn, J. F. Bornman, S. D. Flint, G. Kulandaivelu, A. H. Teramura and M. Tevini, Effects of increased solar ultraviolet radiation on terrestrial ecosystems, J. Photochem. Photobiol. B, 1998, 46, 40–52 Search PubMed.
-
M. M. Caldwell, L. B. Camp, C. W. Warner and S. D. Flint, in Stratospheric ozone reduction, solar ultraviolet radiation and plant life, ed.M. M. Caldwell, Springer, Berlin, 1986, pp. 87–111 Search PubMed.
- S. Madronich, R. L. McKenzie, M. M. Caldwell and L. O. Björn, Changes in ultraviolet radiation reaching the Earth's surface, Ambio, 1995, 24, 143–152 Search PubMed.
-
S. D. Flint and M. M. Caldwell, A biological spectral weighting function for ozone depletion research with higher plantsPhysiol. Plant., 2003, 117, 137–144 Search PubMed.
-
M. M. Caldwell, in Photophysiology, ed. A. C. Giese, Academic Press, New York, 1971, vol. 6, pp. 131–177 Search PubMed.
-
S. D. Flint and M. M. Caldwell, Field testing of UV biological spectral weighting functions for higher plantsPhysiol. Plant., 2003, 117, 145–153 Search PubMed.
- M. C. Rousseaux, C. L. Ballaré, C. V. Giordano, A. L. Scopel, A. M. Zima, M. Szwarcberg-Bracchitta, P. S. Searles, M. M. Caldwell and S. B. Diaz, Ozone depletion and UVB radiation: Impact on plant DNA damage in southern South America, Proc. Natl. Acad. Sci., 1999, 96, 15310–15315 Search PubMed.
- C. A. Mazza, H. E. Boccalandro, C. V. Giordano, D. Battista, A. L. Scopel and C. L. Ballaré, Functional significance and induction by solar radiation of ultraviolet-absorbing sunscreens in field-grown soybean crops, Plant Physiol., 2000, 122, 117–126 CrossRef CAS.
- P. S. Searles, S. D. Flint and M. M. Caldwell, A meta-analysis of plant field studies simulating stratospheric ozone depletion, Oecologia, 2001, 127, 1–10 Search PubMed.
- J. E. Hunt and D. L. McNeil, The influence of present-day levels of ultraviolet-B radiation on seedlings of two southern hemisphere temperate tree species, Plant Ecol., 1999, 143, 39–50 Search PubMed.
- K. Lingakumar, P. Amudha and G. Kulandaivelu, Exclusion of solar UV-B (280–315 nm) radiation on vegetative growth and photosynthetic activities in Vigna unguiculata L, Plant Sci., 1999, 148, 97–103 CrossRef CAS.
- C. A. Mazza, D. Battista, A. M. Zima, M. Szwarcberg-Bracchitta, C. V. Giordano, A. Acevedo, A. L. Scopel and C. L. Ballaré, The effects of solar ultraviolet-B radiation on the growth and yield of barley are accompanied by increased DNA damage and antioxidant responses, Plant Cell Environ., 1999, 22, 61–70 CrossRef CAS.
- C. L. Ballaré, M. C. Rousseaux, P. S. Searles, J. G. Zaller, C. V. Giordano, T. M. Robson, M. M. Caldwell, O. E. Sala and A. L. Scopel, Impacts of solar ultraviolet-B radiation on terrestrial ecosystems of Tierra del Fuego (Southern Argentina). An overview of recent progress, J. Photochem. Photobiol. B, 2001, 62, 67–77 Search PubMed.
- T. A. Day, C. T. Ruhland and F. S. Xiong, Influence of solar ultraviolet-B radiation on Antarctic terrestrial plants: results from a four year field study, J. Photochem. Photobiol. B: Biol., 2001, 62, 78–87 Search PubMed.
- W. J. I. Cybulski and W. T. Peterjohn, Effects of ambient UV-B radiation on the above-ground biomass of seven temperate-zone plant species, Plant Ecol., 1999, 145, 175–181 Search PubMed.
- J. A. Zavala and J. F. Botto, Impact of solar UV-B radiation on seedling emergence, chlorophyll fluorescence, and growth and yield of radish (Raphanus sativus), Funct. Plant Biol., 2002, 29, 797–804 Search PubMed.
- J. H. Sullivan and A. H. Teramura, The effects of ultraviolet-B radiation on loblolly pine. 2. Growth of field-grown seedlings, Trees, 1992, 6, 115–120 CrossRef.
- L. O. Björn, T. V. Callaghan, I. Johnsen, J. A. Lee, Y. Manetas, N. D. Paul, M. Sonesson, A. R. Wellburn, D. J. S. Coop, H. S. Heide-Jorgensen, C. Gehrke, D. Gwynn-Jones, U. Johanson, A. Kyparissis, E. Levizou, D. Nikolopoulos, Y. Petropoulou and M. Stephanou, The effects of UV-B radiation on European heathland species, Plant Ecol., 1997, 128, 252–264 Search PubMed.
- G. K. Phoenix, D. Gwynn-Jones, T. V. Callaghan, D. Sleep and J. A. Lee, Effects of global change on a sub-Arctic heath: effects of enhanced UV-B radiation and increased summer precipitation, J. Ecol., 2001, 89, 256–267 CrossRef.
- C. F. Musil, Accumulated effect of elevated ultraviolet-B radiation over multiple generations of the arid-environment annual Dimorphotheca sinuata DC. (Asteraceae), Plant Cell Environ., 1996, 19, 1017–1027 CAS.
- C. F. Musil, G. F. Midgley and S. J. E. Wand, Carry-over of enhanced ultraviolet-B exposure effects to successive generations of a desert annual: interaction with atmospheric CO2 and nutrient supply, Global Change Biol., 1999, 5, 311–329 Search PubMed.
- V. Walbot, UV-B damage amplified by transposons in maize, Nature, 1999, 397, 398–399 CrossRef CAS.
- G. Ries, G. Buchholz, H. Frohnmeyer and B. Hohn, UV-damage-mediated induction of homologous recombination in Arabidopsis is dependent on photosynthetically active radiation, Proc. Natl. Acad. Sci., 2000, 97, 13425–13429 Search PubMed.
- G. Ries, W. Heller, H. Puchta, H. Sandermann, H. K. Seidlitz and B. Hohn, Elevated UV-B radiation reduces genome stability in plants, Nature, 2000, 406, 98–101 CrossRef CAS.
- V. Dvornyk, O. Vinogradova and E. Nevo, Long-term microclimate stress causes rapid adaptive radiation of kaiABC clock gene family in a cyanobacterium Nostoc linckia from “Evolution Canyons” I and II, Israel, Proc. Natl. Acad. Sci., 2002, 99, 2082–2087 Search PubMed.
- B. Solheim, U. Johanson, T. V. Callaghan, J. A. Lee, D. Gwynn-Jones and L. O. Björn, The nitrogen fixation potential of arctic cryptogram species is influenced by enhanced UV-B radiation, Oecologia, 2002, 133, 90–93 Search PubMed.
- K. K. Newsham, J. M. Anderson, T. H. Sparks, P. Splatt, C. Woods and A. R. McLeod, UV-B effect on Quercus robur leaf litter decomposition persists over four years, Global Change Biol., 2001, 7, 479–483 Search PubMed.
- C. A. Mazza, J. Zavala, A. L. Scopel and C. L. Ballaré, Perception of solar UVB radiation by phytophagous insects: Behavioral responses and ecosystem implications, Proc. Natl. Acad. Sci., 1999, 96, 980–985 Search PubMed.
-
C. A. Mazza, M. M. Izaguirre, J. Zavala, A. L. Scopel, C. L. Ballaré, Insect perception of ambient ultraviolet-B radiation, Ecol. Lett., 2003, 6, in press Search PubMed.
- J. A. Zavala, A. L. Scopel and C. L. Ballaré, Effects of ambient UV-B radiation on soybean crops: Impact on leaf herbivory by Anticarsia gemmatalis, Plant Ecol., 2001, 156, 121–130 Search PubMed.
- A. R. McLeod, A. Rey, K. K. Newsham, G. C. Lewis and P. Wolferstan, Effects of elevated ultraviolet radiation and endophytic fungi on plant growth and insect feeding in Lolium perenne Festuca rubra F. arundinacea and F. pratensis, J. Photochem. Photobiol. B, 2001, 62, 97–107 Search PubMed.
- M. C. Rousseaux, A. L. Scopel, P. S. Searles, M. M. Caldwell, O. E. Sala and C. L. Ballaré, Responses to solar ultraviolet-B radiation in a shrub-dominated natural ecosystem of Tierra del Fuego (southern Argentina), Global Change Biol., 2001, 7, 467–478 Search PubMed.
- R. L. Lindroth, R. W. Hofmann, B. D. Campbell, W. C. McNabb and D. Y. Hunt, Population differences in Trifolium repens L. response to ultraviolet-B radiation: foliar chemistry and consequences for two lepidopteran herbivores, Oecologia, 2000, 122, 20–28.
- N. Buck and T. V. Callaghan, The direct and indirect effects of enhanced UV-B on the moth caterpillar Epirrita autumnata, Ecol. Bull., 1999, 47, 68–76 Search PubMed.
- E. S. McCloud and M. R. Berenbaum, Effects of enhanced UV-B radiation on a weedy forb (Plantago lanceolata) and its interactions with a generalist and specialist herbivore, Entomol. Exp. Appl., 1999, 93, 233–247 Search PubMed.
- K. K. Newsham, P. D. Greenslade and A. R. McLeod, Effects of elevated ultraviolet radiation on Quercus robur and its insect and ectomycorrhizal associates, Global Change Biol., 1999, 5, 881–890 Search PubMed.
- M. C. Rousseaux, C. L. Ballaré, A. L. Scopel, P. S. Searles and M. M. Caldwell, Solar ultraviolet-B radiation affects plant–insect interactions in a natural ecosystem of Tierra del Fuego (southern Argentina), Oecologia, 1998, 116, 528–535 CrossRef.
- D. T. Salt, S. A. Moody, J. B. Whittaker and N. D. Paul, Effects of enhanced UVB on populations of the phloem feeding insect Strophingia ericae
(Homoptera: Psylloidea) on heather (Calluna vulgaris), Global Change Biol., 1998, 4, 91–96 Search PubMed.
- A. Lavola, R. Julkunen-Tiitto, H. Roininen and P. J. Aphalo, Host-plant preference of an insect herbivore mediated by UV-B and CO2 in relation to plant secondary metabolites, Biochem. Syst. Ecol., 1998, 26, 1–12 CrossRef CAS.
- D. Gwynn-Jones, Enhanced UV-B radiation and herbivory, Ecol. Bull., 1999, 47, 77–83 Search PubMed.
- D. Gwynn-Jones, J. A. Lee and T. V. Callaghan, Effects of enhanced UV-B radiation and elevated carbon dioxide concentrations on a sub-arctic forest heath ecosystem, Plant Ecol., 1997, 128, 242–249 Search PubMed.
- J. Grant-Petersson and J. A. A. Renwick, Effects of ultraviolet-B exposure of Arabidopsis thaliana on herbivory by two crucifer-feeding insects (Lepidoptera), Environ. Entomol., 1996, 25, 135–142 Search PubMed.
- C. L. Ballaré, A. L. Scopel, A. E. Stapleton and M. J. Yanovsky, Solar ultraviolet-B radiation affects seeding emergence, DNA integrity, plant morphology, growth rate, and attractiveness to herbivore insects in Datura ferox, Plant Physiol., 1996, 112, 161–170 CAS.
- K. K. Newsham, A. R. McLeod, P. D. Greenslade and B. A. Emmett, Appropriate controls in outdoor UV-B supplementation experiments, Global Change Biol., 1996, 2, 319–324 Search PubMed.
- P. E. Hatcher and N. D. Paul, The effect of elevated UV-B radiation on herbivory of pea by Autographa gamma, Entomol. Exp. Appl., 1994, 71, 227–233 Search PubMed.
- D. J. Bergvinson, J. T. Arnason, R. I. Hamilton, S. Tachibana and G. H. N. Towers, Putative role of photodimerized phenolic acids in maize resistance to Ostrinia nubilalis
(Lepidoptera: Pyralidae), Environ. Entomol., 1994, 23, 1516–1523 Search PubMed.
- E. S. McCloud and M. R. Berenbaum, Stratospheric ozone depletion and plant–insect interactions: Effects of UVB radiation on foliage quality of Citrus jambhiri for Trichoplusia ni, J. Chem. Ecol., 1994, 20, 525–539 Search PubMed.
- P. S. Searles, B. R. Kropp, S. D. Flint and M. M. Caldwell, Influence of solar UV-B radiation on peatland microbial communities of Southern Argentina, New Phytol., 2001, 152, 213–221 Search PubMed.
- S. A. Moody, K. K. Newsham, P. G. Ayres and N. D. Paul, Variation in the responses of litter and phylloplane fungi to UV-B radiation (290–315 nm), Mycol. Res., 1999, 103, 1469–1477 Search PubMed.
- J. L. Jacobs and G. W. Sundin, Effect of solar UV-B radiation on a phyllosphere bacterial community, Appl. Environ. Microbiol., 2001, 67, 5488–5496 CrossRef CAS.
- D. Johnson, C. D. Campbell, D. Gwynn-Jones, J. A. Lee and T. V. Callaghan, Arctic soil microorganisms respond more to long-term ozone depletion than to atmospheric CO2, Nature, 2002, 416, 82–83 CrossRef CAS.
- J. W. M. van de Staaij, J. Rozema, A. van Beem and R. Aerts, Increased solar UV-B radiation may reduce infection by arbuscular mycorrhizal fungi (AMF) in dune grassland plants: evidence from five years of field exposure, Plant Ecol., 2001, 154, 171–177 Search PubMed.
- J. G. Zaller, M. M. Caldwell, S. D. Flint, A. L. Scopel, O. E. Sala and C. L. Ballaré, Solar UV-B radiation affects below-ground parameters in a fen ecosystem in Tierra del Fuego, Argentina: implications of stratospheric ozone depletion, Global Change Biol., 2002, 8, 867–871 Search PubMed.
- N. D. Paul, Stratospheric ozone depletion, UV-B radiation and crop disease, Environ. Poll., 2000, 108, 343–355 CrossRef CAS.
- K. K. Newsham, K. Oxborough, R. White, P. D. Greenslade and A. R. McLeod, UV-B radiation constrains the photosynthesis of Quercus robur through impacts on the abundance of Microsphaera alphitoides, For. Pathol., 2000, 30, 265–275 Search PubMed.
- K. K. Newsham, G. C. Lewis, P. D. Greenslade and A. R. McLeod,
Neotyphodium lolii, a fungal leaf endophyte, reduces fertility of Lolium perenne exposed to elevated UV-B radiation, Ann. Bot., 1998, 81, 397–403 CrossRef.
- N. D. Paul, S. Rasanayagam, S. A. Moody, P. E. Hatcher and P. G. Ayres, The role of interactions between trophic levels in determining the effects of UV-B on terrestrial ecosystems, Plant Ecol., 1997, 128, 296–308 Search PubMed.
- T. S. Gunasekera, N. D. Paul and P. G. Ayres, The effects of ultraviolet-B (UV-B: 290–320 nm) radiation on blister blight disease of tea (Camellia sinensis), Plant Pathol., 1997, 46, 179–185 CrossRef.
- K. K. Newsham, M. N. R. Low, A. R. McLeod, P. D. Greenslade and B. A. Emmett, Ultraviolet-B radiation influences the abundance and distribution of phylloplane fungi on pedunculate oak (Quercus robur), New Phytol., 1997, 136, 287–297 Search PubMed.
- Y. Naito, Y. Honda and T. Kumagai, Effects of supplementary UV-B radiation on development of damping-off in spinach caused by the soil-borne fungus Fusarium oxysporum, Mycoscience, 1996, 37, 15–19 Search PubMed.
-
P. G. Ayres, T. S. Gunasekera, M. S. Rasanayagam, N. D. Paul, in Fungi and environmental change, ed. G. M. Gadd, Cambridge University Press, 1996, pp. 32–50 Search PubMed.
- M. R. Finckh, A. Q. Chavez, Q. Dai and P. S. Teng, Effects of enhanced UV-B radiation on the growth of rice and its susceptibility to rice blast under glasshouse conditions, Agric. Ecosyt. Environ., 1995, 52, 223–233 Search PubMed.
- I. Panagopoulos, J. F. Bornman and L. O. Björn, Response of sugar beet plants to ultraviolet-B (280–320 nm) radiation and Cercospora leaf spot disease, Physiol. Plant., 1992, 84, 140–145 CrossRef CAS.
- A. B. Orth, A. H. Teramura and H. D. Sisler, Effects of ultraviolet-B radiation on fungal disease development in Cucumis sativus, Am. J. Bot., 1990, 77, 1188–1192 Search PubMed.
-
R. H. Biggs, P. G. Webb, in Stratospheric ozone reduction, solar ultraviolet radiation and plant life, ed. M. M. Caldwell, Springer-Verlag, Berlin, 1986, pp. 303–311 Search PubMed.
- P. Semeniuk and R. N. Stewart, Effect of ultraviolet (UV-B) irradiation on infection of roses by Diplocarpon rosae Wolf, Environ. Exp. Bot., 1981, 21, 45–50 CrossRef.
- P. Semeniuk and R. W. Goth, Effects of ultraviolet irradiation on local lesion development of potato virus S on Chenopodium quinoa
‘Valdivia’ leaves, Environ. Exp. Bot., 1980, 20, 95–98 CrossRef.
- S. Moody, N. D. Paul, L. O. Björn, T. V. Callaghan, J. A. Lee, Y. Manetas, J. Rozema, D. Gwynn-Jones, U. Johanson, A. Kyparissis and A. Oudejans, The direct effects of UVB radiation on Betula pubescens litter decomposing at four European field sites, Plant Ecol., 2001, 154, 29–36 Search PubMed.
- K. J. Duguay and J. N. Klironomos, Direct and indirect effects of enhanced UV-B radiation on the decomposing and competitive abilities of saprobic fungi, Appl. Soil Ecol., 2000, 14, 157–164 Search PubMed.
- K. K. Newsham, A. R. McLeod, J. D. Roberts, P. D. Greenslade and B. A. Emmet, Direct effects of elevated UV-B radiation on the decomposition of Quercus robur leaf litter, Oikos, 1997, 79, 592–602.
- C. Gehrke, U. Johanson, T. V. Callaghan, D. Chadwick and C. H. Robinson, The impact of enhanced ultraviolet-B radiation on litter quality and decomposition processes in Vaccinium leaves from the subarctic, Oikos, 1995, 72, 213–222.
- G. U. L. Braga, S. D. Flint, C. D. Miller, A. J. Anderson and D. W. Roberts, Variability in response to UV-B among species and strains of Metarhizium isolated from sites at latitudes from 61 °N to 54 °S, J. Invertebr. Pathol., 2001, 78, 98–108 CrossRef CAS.
- G. U. L. Braga, S. D. Flint, C. D. Miller, A. J. Anderson and D. W. Roberts, Both solar UVA and UVB radiation impair conidial culturability and delay germination in the entomopathogenic fungus Metarhizium anisopliae, Photochem. Photobiol., 2001, 74, 734–739 Search PubMed.
- M. Shapiro and J. Domek, Relative effects of ultraviolet and visible light on the activities of corn earworm and beet armyworm (Lepidoptera: Noctuidae) nucleopolyhedroviruses, J. Econ. Entomol., 2002, 95, 261–268 Search PubMed.
-
J. Rozema, G. M. Lenssen, J. W. M. van de Staaij, in The greenhouse effect and primary productivity in European agroecosystems, ed. H. H. VanLaar, Pudoc, Wageningen, 1990, pp. 68–71 Search PubMed.
- L. H. Ziska and A. H. Teramura, CO2 enhancement of growth and photosynthesis in rice (Oryza sativa). Modification by increased ultraviolet-B radiation, Plant Physiol., 1992, 99, 473–481 CAS.
- J. H. Sullivan and A. H. Teramura, The effects of UV-B radiation on loblolly pine. 3. Interaction with CO2 enhancement, Plant Cell Environ., 1994, 17, 311–317.
- A. J. Visser, M. Tosserams, M. W. Groen, G. W. H. Magendans and J. Rozema, The combined effects of CO2 concentration and solar UV-B radiation on faba bean grown in open-top chambers, Plant Cell Environ., 1997, 20, 189–199 CrossRef CAS.
- J. Rozema, G. M. Lenssen, J. W. M. van de Staaij, M. Tosserams, A. J. Visser and R. A. Broekman, Effects of UV-B radiation on terrestrial plants and ecosystems: interaction with CO2 enrichment, Plant Ecol., 1997, 128, 182–191 Search PubMed.
- J. H. Sullivan, Effects of increasing UV-B radiation and atmospheric CO2 on photosynthesis and growth: implications for terrestrial ecosystems, Plant Ecol., 1997, 128, 194–206 Search PubMed.
- X. Hao, B. A. Hale, D. P. Ormrod and A. P. Papadopoulos, Effects of pre-exposure to ultraviolet-B radiation on responses of tomato (Lycopersicon esculentum cv. New Yorker) to ozone in ambient and elevated carbon dioxide, Environ. Pollut., 2000, 110, 217–224 CrossRef CAS.
-
S. A. Moody, D. J. S. Coop, N. D. Paul, in Plants and UV-B. Responses to environmental change, ed. P. J. Lumsden, Cambridge University Press, 1997, pp. 283–304 Search PubMed.
- A. Lavola, R. Julkunen-Tiitto, T. M. de la Rosa, T. Lehto and P. J. Aphalo, Allocation of carbon to growth and secondary metabolites in birch seedlings under UV-B radiation and CO2 exposure, Physiol. Plant., 2000, 109, 260–267 CrossRef CAS.
- M. Tosserams, A. J. Visser, M. W. Groen, G. Kalis, E. Magendans and J. Rozema, Combined effects of CO2 concentration and enhanced UV-B radiation on faba bean, Plant Ecol., 2001, 154, 197–210 Search PubMed.
- A. Lavola, R. Julkunen-Tiitto, H. Roininen and P. Aphalo, Host-plant preference of an insect herbivore mediated by UV-B and CO2 in relation to plant secondarv metabolites, Biochem. Syst. Ecol., 1998, 26 CrossRef CAS.
- J. H. Sullivan and A. H. Teramura, Field study of the interaction between solar ultraviolet-B radiation and drought on photosynthesis and growth in soybean, Plant Physiol., 1990, 92, 141–146.
- T. Balakumar, V. H. B. Vincent and K. Paliwal, On the interaction of UV-B radiation (280–315 nm) with water stress in crop plants, Physiol. Plant., 1993, 87, 217–222 CrossRef CAS.
-
B. D. Campbell, R. W. Hofmann, C. L. Hunt, in Stratospheric ozone depletion: the effects of enhanced UV-B radiation on terrestrial ecosystems, ed. J. Rozema, Backhuys, Leiden, 1999, pp. 226–249 Search PubMed.
- Y. Manetas, Y. Petropoulou, K. Stamatakis, D. Nikolopoulos, E. Levizou, G. Psaras and G. Karabourniotis, Beneficial effects of enhanced UV-B radiation under field conditions: improvement of needle water relations and survival capacity of Pinus pinea L. seedlings during the dry Mediterranean summer, Plant Ecol., 1997, 128, 100–108 Search PubMed.
- J. E. Hunt and D. L. McNeil, Nitrogen status affects UV-B sensitivity of cucumber, Aust. J. Plant Physiol., 1998, 25, 79–86 Search PubMed.
- S. Li, M. Paulsson and L. O. Björn, Temperature-dependent formation and photorepair of DNA damage induced by UV-B radiation in suspension-cultured tobacco cells, J. Photochem. Photobiol. B, 2002, 66, 67–72 Search PubMed.
- Y. Takeuchi, M. Murakami, N. Nakajima, N. Kondo and O. Nikaido, Induction and repair of damage to DNA in cucumber cotyledons irradiated with UV-B, Plant Cell Physiol., 1997, 37, 181–187 Search PubMed.
- Q. Pang and J. B. Hays, UV-B-inducible and temperature-sensitive photoreactivation of cyclobutane pyrimidine dimers in Arabidopsis thaliana, Plant Physiol., 1991, 95, 536–543 CAS.
- D. J. Beerling, A. C. Terry, P. L. Mitchell, T. V. Callaghan, D. Gwynn-Jones and J. A. Lee, Time to chill: Effects of simulated global change on leaf ice nucleation temperatures of subarctic vegetation, Am. J. Bot., 2001, 88, 628–633 Search PubMed.
- T. A. Day, C. T. Ruhland, C. W. Grobe and F. Xiong, Growth and reproduction of Antarctic vascular plants in response to warming and UV radiation reductions in the field, Oecologia, 1999, 119, 24–35 CrossRef.
- D. Lud, A. H. L. Huiskes, T. C. W. Moerdijk and J. Rozema, The effects of altered levels of UV-B radiation on an Antarctic grass and lichen, Plant Ecol., 2001, 154, 87–99 Search PubMed.
- G. Kulandaivelu and N. Nedunchezhian, Synergistic effects of ultraviolet-B enhanced radiation and growth temperature on ribulose 1,5-bisphosphate carboxylase and 14CO2 fixation in Vigna sinensis L, Photosynthetica, 1993, 29, 377–383 Search PubMed.
- N. Nedunchezhian and G. Kulandaivelu, Effects of ultraviolet-B enhanced radiation and temperature on growth and photochemical activities in Vigna unguiculata, Biol. Plant., 1996, 38, 205–214 Search PubMed.
- J. S. Bale, G. J. Masters, I. D. Hodkinson, C. Awmack, T. M. Bezemer, V. K. Brown, J. Butterfield, A. Buse, J. C. Coulson, J. Farrar, J. E. G. Good, R. Harrington, S. Hartley, T. H. Jones, R. L. Lindroth, M. C. Press, I. Symrnioudis, A. D. Watt and J. B. Whittaker, Herbivory in global climate change research: direct effects of rising temperature on insect herbivores, Global Change Biol., 2002, 8, 1–16 Search PubMed.
- T. C. R. White, Weather, food and plagues of locusts, Oecologia, 1976, 22, 119–134 CrossRef.
- S. A. Moody, K. K. Newsham, P. G. Ayres and N. D. Paul, Variation in the responses of litter and phylloplane fungi to UV-B radiation (290–315 nm), Mycol. Res., 1999, 103, 1469–1477 Search PubMed.
-
B. Huntley, in Vegetation History, ed. T. I. Webb, Kluwer Academic Publishers, Dordrecht, 1988, pp. 341–383 Search PubMed.
-
M. T. Sykes, in Past and future rapid environmental changes: The spatial and evolutionary responses of terrestrial biota, ed. J. R. M. Allen, Springer-Verlag, Berlin, 1997, pp. 427–440 Search PubMed.
- M. T. Sykes, I. C. Prentice and W. Cramer, A bioclimatic model for the potential distributions of north European tree species under present and future climates, J. Biogeogr., 1996, 23, 203–233.
- M. Sturm, C. Racine and K. Tape, Increasing shrub abundance in the Arctic, Nature, 2001, 411, 546–547 CrossRef CAS.
Footnote |
† This article is published as part of the United Nations Environmental Programme: Environmental effects of ozone depletion and its interactions with climate change: 2002 assessment. |
|
This journal is © The Royal Society of Chemistry and Owner Societies 2003 |