Health effects from stratospheric ozone depletion and interactions with climate change†
First published on 10th January 2003
Abstract
The potential health effects of elevated levels of ambient UV-B radiation are diverse, and it is difficult to quantify the risks, especially as they are likely to be considerably modified by human behaviour. Nevertheless epidemiological and experimental studies have confirmed that UV radiation is a definite risk factor for certain types of cataract, with peak efficacy in the UV-B waveband. The causal link between squamous cell carcinoma and cumulative solar UV exposure has been well established. New findings regarding the genetic basis of skin cancer, including studies on genetically modified mice, have confirmed the epidemiological evidence that UV radiation contributes to the formation of basal cell carcinomas and cutaneous melanomas. For the latter, animal models have demonstrated that UV exposure at a very young age is more detrimental than exposure in adulthood. Although suppression of certain immune responses has been recognised following UV exposure, the impact of this suppression on the control of infectious and autoimmune diseases is largely unknown. However, studies on several microbial infections have indicated significant consequences in terms of symptoms or reactivation of disease. The possibility that the immune response to vaccination could be depressed by UV-B exposure is of considerable concern. Newly emerging possibilities regarding interactions between ozone depletion and global climate change further complicate the risk assessments for human health but might result in an increased incidence of cataracts and skin cancer, plus alterations in the patterns of certain categories of infectious and other diseases.
Introduction
The potential health effects of elevated levels of ambient UV radiation due to a depletion of stratospheric ozone have been under study for over 30 years, and the UNEP 1998 report1,2 presented an overall review. The present paper focuses on the main research developments from 1997 to the present.
From the outset it should be pointed out that human behaviour with regard to sun exposure is of decisive importance in considering the health risks. This includes such parameters as the popularity of tanning, the taking of holidays in the sun, and the wearing of minimal clothing as soon as the sun shines. Epidemiological and experimental studies show that proper protective measures, such as wearing appropriate glasses or sunglasses, and sunscreens, can offer some protection. In addition, some dietary and therapeutic ingredients are known to influence the risks both positively and negatively. These modifying factors are not included here because of the uncertainties surrounding their current and future impact.
Although UV- B radiation is effectively attenuated by the stratospheric ozone layer, it is not fully blocked.3 It is very strongly absorbed in tissues and penetrates only superficially into the body; thus it directly affects only the eye and the skin. Detrimental consequences for the eye could include impaired vision, since UV radiation has been reported to cause opacification of the lens (cataract). Effects of UV radiation on the eye are discussed in the first section below. The second section deals with the skin, summarising the acute effects of UV exposure and research developments with direct relevance to UV-induced skin cancers. Modulations in immune responses due to UV irradiation are then outlined, including the impact of these changes on the immunological control of certain diseases. In later sections, the potential effects of UV exposure on internal cancers and the possible health consequences of the substitutes for ozone depleting substances (ODS) are discussed. Finally, consideration is given to new research into environmental changes, in particular the interactions between global warming and stratospheric ozone depletion. Regarding health effects that could be caused by these interactions, little research has been done thus far, but an inventory of potential outcomes is attempted.
The eye
In recent years, it has become increasingly apparent that the effects of UV radiation are much more insidious and detrimental to the eye and vision than had been suspected previously. The effects may be acute (usually after a latent period), long-term following an acute exposure, or chronic due to extended or repeated exposure to levels of UV radiation below those required for acute effects. All anterior structures of the eye and those adjacent to it are potential targets for solar UV radiation. Whereas the skin and eyelids may be irradiated by direct sunlight, the outer layers of the eyeball (corneal and conjunctiva), iris and crystalline lens are mainly exposed to reflected and scattered UV radiation.4 Our earlier report2 considered the possible direct and indirect effects on the cornea and conjunctiva (photokeratitis, photoconjunctivitis, climatic droplet keratopathy, pinguecula, pterygium and squamous cell carcinoma), and the iris, ciliary body and choroid (anterior uveitis, ocular malignant melanoma). This report concentrates on the lens of the eye since cataract is the sunlight-related eye disease (ophthalmoheliosis) with the most serious public health implications.
Lens opacities
The lens of the human eye changes with increasing age: it loses its transparency, becomes opalescent and turns yellowish to brownish in hue. Its fluorescence increases,5 and the internal scattering of light increases6 resulting in reduced contrast, increased glare and decreased vision. In addition to these general changes in the nucleus of the lens, localized changes in the cortex (opaque spokes) may occur and may increase in size and extend toward the visual axis of the eye, ultimately impairing vision. Such an advanced stage of lens opacity forms a cataract requiring surgical intervention. As it is related to age, it is sometimes unfortunately referred to as ‘senile cataract’. The mechanisms underlying all of these age-related changes are not fully established, but in accordance with general views on aging, oxidation is involved, e.g. as a side effect of metabolic processes (see for example refs. 7,8). As UV radiation can generate reactive oxygen, it is also suspected to contribute to the deterioration of the lens by oxidation.9–11
Three main types of age-related cataract can be distinguished on the basis of location (Fig. 1): cortical cataract (CC) involving the cortex of the lens (Fig. 2a), nuclear (sclerotic) cataract (NC) at the nucleus of the lens (Fig. 2b), and posterior subcapsular cataract (PSC) at the extreme posterior cortex (Fig. 2c).
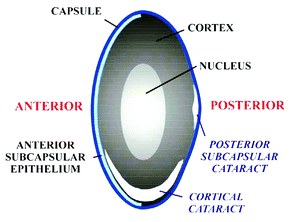 |
| Fig. 1 Schematic cross section of the lens demonstrating various zones and forms of age-related cataract. | |
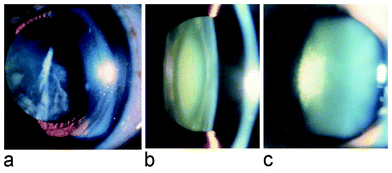 |
| Fig. 2 a) frontal view of a cortical cataract, b) cross sectional view (slit lamp) of a nuclear cataract, c) slit lamp view of a posterior subcapsular cataract. | |
Epidemiology
Globally cataract is a major cause of blindness. Cataract is adequately treated by surgery, but, where not treated, it often leads to permanent blindness with grave social/economic consequences; for instance, in 1998 an estimated 135 million people were visually impaired and 45 million people were blind worldwide with cataract as the leading cause [http://www.who.int/pbd/Vision2020/V2020slides/sld003.htm accessed 21 September 2002]. Epidemiological studies show that the aetiology of cataract is complicated and involves many risk factors. In considering all the risk factors, Taylor12 concludes that the only effective preventive interventions are to stop smoking and to reduce ocular UV-B exposure.
The reported risks from sun (UV) exposure in population-based studies are quite moderate: generally, less than 2 fold increases of risk.13 Assessments of personal, lifelong UV exposures in these studies (mainly from questionnaires) are, however, very inaccurate and inevitably lead to an underestimation of the relative risk. The best attempt made to record an accurate personal solar UV exposure history was in a study among watermen in Maryland,14 and a highly significant correlation was found between exposure and CC. The impact of solar UV radiation is population-wide, and therefore a small relative risk translates into a substantial number of cases. One alternative to assessing personal UV exposure is to study populations living at latitudes with differences in ambient UV exposure. For example, good correlations have been established between cataracts and ambient UV exposure in the Aboriginal population in Australia,15 and in the registered cataract operations in the USA.16 Although overall (ambient) UV exposures can be accurately assessed, these correlations can be affected by many confounding factors, such as behavioural, ethnic and environmental differences.
Of people older than 40 years in Victoria, Australia, 12% were found to have CC, 13% NC and 5% PSC.17 CC is relatively more prevalent than other types of cataract in populations living in temperate climates and the incidence increases toward lower latitudes. NC is more common in populations near the equator (tropical climates), e.g. comparing Iceland and Noto versus Singapore and Amami.18 Despite the high prevalence of NC in the tropics, there is no evidence that NC is related to solar UV exposure. The relationship between CC and sun exposure was re-affirmed in a population-based study in Salisbury, Maryland.19 A recent study in Iceland involving 1045 people older than 50 years reported a significantly increased risk for two different grades of cortical opacification in people who spend more than 4 hours per day outside on weekdays.20 In all, there is sufficient evidence of an increase in cortical opacities, including CC, with increasing UV exposure to warrant advising the public on measures to decrease their ocular exposure.21
Experimental evidence
The dependence of cataract formation on the wavelength of the UV radiation is important in establishing whether increases in ambient UV-B exposure due to ozone depletion will have an impact. Cataracts in humans develop slowly with age, and this process is difficult to investigate experimentally, especially in human subjects, but it can be done in isolated lenses and in animals. The problem with these experiments is that the cataracts are induced in a matter of hours, days or weeks. Careful comparisons show that the commonly UV-induced anterior subcapsular opacification in animals is not adequate to model the age-related cataracts observed in humans.22 The wavelength dependence of cataract formation can be inferred from the basic mechanism, but knowledge on this subject is inadequate.
Much in agreement with an older study,23 the wavelength dependence of cataract formation in rats was found to peak in the UV-B at 300 nm.24 Similarly, the efficacy of inducing CC in pig lenses in culture was found to be greatest for wavelengths shorter than 300 nm (UV-B), and these were about 2000 times more effective than wavelengths around 365 nm (UV-A);25 see Fig. 3. Hence, the experiments on isolated lenses and in animals provide the best data on the wavelength dependence, and reveal the increased potential hazard of ozone depletion to CC formation in human subjects.
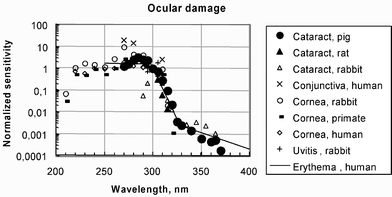 |
| Fig. 3 Wavelength dependencies measured for effects on the eye (symbols) compared to sunburn (lines); most recent data on cataract formation in solid symbols (cataract in pig25 and in rat24)
(courtesy of S. Madronich). | |
The skin
Although there are several types of molecules in the skin that absorb UV radiation resulting in their modification or damage, cellular DNA is the primary target for many UV-induced effects. These range from acute outcomes such as sunburn, to chronic outcomes such as skin cancer. DNA molecules are of central importance to the cell: sections make up the various genes that carry the basic information with which a cell forms its proteins and thus controls its behaviour.
Acute effects of UV exposure
Sunburn.
A unique study in Punta Arenas, a city located at the southern tip of Chile, has documented the relationship between episodes of ozone depletion, increased terrestrial UV-B radiation and sunburn during the spring months.26 The spring Antarctic ‘Ozone Hole’ passes over or near this city each spring, and local data of sudden ozone depletion and the corresponding increase in UV-B radiation are available. There was a dramatic rise in the number of sunburn cases after sudden severe ozone depletion, coinciding with Sunday outdoor recreational exposure. Further detrimental consequences for this population and others at similarly affected latitudes are currently unknown, but are worthy of further consideration.
DNA damage.
UV-B radiation causes very specific damage in a strand of DNA: chemically linking neighbouring pyrimidine bases to form pyrimidine dimers. The wavelength dependency of such damage closely follows that of inducing sunburn,27 and effective repair in active regions of DNA lowers the sunburn sensitivity.28 Focus on this type of alteration in DNA may have detracted from other, less direct, effects of UV radiation such as the generation of chemically reactive molecules, most notably those containing reactive oxygen, that cause oxidative damage to DNA. The oxidative damage is relatively more abundant at longer wavelengths, in the UV-A band, when compared to pyrimidine dimers which are more abundant at wavelengths around 300 nm.29 The wavelength dependence of these types of DNA damage combined may explain the wavelength dependence of squamous cell carcinomas, see Fig. 4.
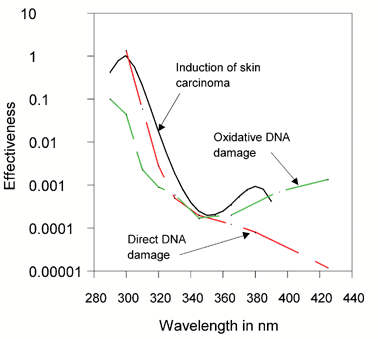 |
| Fig. 4 The wavelength dependence of induction of skin carcinomas30 compared to that of direct DNA damage (pyrimidine dimers) and indirect, oxidative DNA damage (e.g., 8-oxo-G).29 The DNA damage curves are shifted over the vertical axis by factors of 10 to coincide with the curve for skin carcinomas around 350 nm. Notice the correspondence between the curve for skin carcinomas and the upper contour for total DNA damage. | |
Recent research has revealed that, in addition to point mutations in the DNA, UV-B induced DNA damage also gives rise to changes in larger sections of DNA, such as by deletions31 and by faulty division leading to an excess of DNA in ‘micro-nuclei’ within cells.32,33 Because such defects in DNA can be caused by many other agents, it is not easy to establish a link between them and solar UV exposure.
DNA repair and defects.
The various types of DNA damage require an array of different mechanisms of DNA repair. The pyrimidine dimers induced by UV-B radiation are mainly removed by ‘Nucleotide Excision Repair’
(NER). This form of repair has been studied in depth using cells from Xeroderma pigmentosum (XP) patients who are defective in this form of DNA repair. XP patients are known to be very sensitive to UV-B radiation and develop skin cancers extremely early in life. That NER is not the only repair mechanism of importance is shown by the increased skin cancer risk in patients with a milder form of XP, the XP-variant. These patients have no defect in NER, but they lack a proper corrective mechanism in the replication of UV-B damaged DNA (caused by a lack of an enzyme that is capable of copying DNA across a cyclobutane pyrimidine dimer).34 This results in gross rearrangements in the genetic material of the cells (i.e., increases in ‘sister chromatid exchanges’ caused by yet another form of DNA repair, recombinational repair).35
After years of development using animal models, liposomes containing DNA repair enzymes have now been tested on the skin of human volunteers. There was some acceleration in the repair of pyrimidine dimers but the UV induction of sunburn (the skin ‘erythema’ or redness) and ‘sunburn cells’
(apoptotic cells, i.e. cells undergoing programmed cell death) was not affected. However, the UV-induced expression of genes important in suppressing immune responses was decreased.36 In another experiment using different, light-activated, repair enzymes, the enhanced repair prevented the UV-induced suppression of an allergic skin reaction.37 These latter experiments confirm that DNA is one very important UV target, and that DNA damage can initiate the immunosuppressive response to UV exposure (see section on UV-induced immunosuppression for further details).
Skin cancer
Epidemiology and trends.
Skin cancer is very common in people of Northern European descent and is by far the most frequent cancer in these people living in subtropical areas of the USA and Australia. In increasing order of malignancy and decreasing order of incidence, the three main types are basal cell carcinoma (BCC), squamous cell carcinoma (SCC), and cutaneous melanoma (CM)
(cancer of skin pigment cells called melanocytes). To give one example of the scale of the problem, in Finland about 50 new cases of BCC occur per 100,000 people per year38 whereas in Australia it is about 800 new cases per 100,000 people per year.39 Epidemiological studies have shown that all 3 types are related to sun (UV) exposure.40 The high incidences imply a large burden on health care systems. Except for CM, the mortality is generally low when compared to internal cancers, but successful therapy can leave a person disfigured, e.g. by surgery on the face where these tumours occur most frequently.
Reported trends with time in incidences of BCC and SCC vary substantially, but mainly increases are reported. For example, both BCC and SCC incidences rose by about 50% from 1988 to 1998 in the south of Wales,41 BCC by about 70% and SCC by about 15% from 1978 to 1995 in the Slovak Republic,42 BCC by 20% and SCC by 90% from 1985 to 1995 in Australia.39 In contrast, there have been no consistent increases in either BCC or SCC from 1985 to 1996 in Arizona, USA.43 Large increases, up to a doubling over 10 years, have occurred in the incidence of CM during the last century,40 but as reported earlier for CM mortality in the USA,44 age-standardised mortality and incidence are levelling off, especially in women, in Northern Europe, Australia and Canada.45–47
Intervention studies.
A recent randomised prospective cohort study in Queensland, Australia48 showed that the daily use of sunscreens for 4.5 years by adults decreased the appearance of new SCC, but not BCC. This is in accordance with the finding that UV radiation contributes to the development of SCC at all stages during a lifetime, whereas UV radiation mostly affects early stages of BCC development.2
In a randomised multi-centre study on 30 XP patients, it was found that enhanced DNA repair by regular application of liposomes containing DNA repair enzymes to the exposed skin over a period of 1 year was of significant benefit to these patients. It decreased the rate of newly occurring actinic keratoses (precursors of SCC) to one third of the number in control patients.49
Regulatory pathways of cell growth and carcinogenic changes in man and animal models.
With the rapid expansion of bio-molecular techniques, cancer research is making great advances in the understanding of how normal cells can become malignant, i.e., proliferate and spread uncontrollably. In general, a cancer cell arises from corrupted biochemical signalling pathways that control a cell's life cycle (i.e., division, maturation and death). The most permanent disruption stems from altered or lost genes that code for proteins involved in these signalling pathways. As discussed previously, UV-B radiation damages the DNA from which the genes are built up, and when repair fails to remove the damage, faulty copies of a gene may be passed to daughter cells. In general, a combination of genes has to be affected rather than a single gene in order for a cell to become malignant. Studies on oncogenic pathways indicate that the conversion of a normal cell to a cancer cell requires the modification of two or more pathways promoting cell survival and proliferation, on the one hand, versus growth suppression and cell death, on the other. After identifying such pathways, their targeting by UV radiation can be investigated.
Early changes in the genes involved in cancer (carcinogenic ‘events’) can be detected or even quantified in terms of frequency of occurrence. A good example is the early occurrence in human skin of microscopic clones of cells that carry UV-B specific point mutations (at potential pyrimidine dimer sites) in the P53 tumor suppressor gene;50,51 these mutations are also found in a large majority of skin carcinomas (BCC and SCC). In animal experiments the relationship between these early carcinogenic events and the ultimate SCC can be studied in detail, and the causal link between the P53-mutant clones and SCC has been substantiated recently.52 These developments open up new possibilities for improved quantitative risk models that incorporate such early events as predictors of future cancer risk.
There is epidemiological evidence and experimental proof in mice to demonstrate that chronic exposure to solar UV-B radiation causes SCC.2 Although there is epidemiological evidence that sun exposure contributes to the formation of BCC and CM, there was virtually no animal data for BCC, and only ambiguous animal data for CM until recently (see below). Details of the specific oncogenic pathways involved in BCC and CM have been revealed from studying familial forms of these cancers.53,54 Subsequently, non-familial (sporadic) skin cancers were investigated for abnormalities in these pathways and relevant genes. These aberrant genes have been introduced into mice which can then be studied for their tendency to develop these types of skin cancer, either with or without UV exposure.
BCC.
A particular proliferative pathway (SHH) is activated in the vast majority of BCC,55 often by mutations in the PTCH gene.56 Some of these mutations are characteristic of UV-B induced mutations. Transgenic mice with one functional copy of the homologue (Ptc) of the PTCH gene contract more BCC more rapidly when exposed to UV radiation.57 Most of these BCC lose the functional copy of the Ptc gene, and all BCC show activation of the SHH pathway. As in humans, a large fraction (40%) of the BCC also carry UV-B related mutations in the P53 tumor suppressor gene. These data show that UV-B radiation can play an important role in causing and enhancing the development of BCC, both by contributing to the activation of the proliferative SHH pathway (by loss of Ptc) and by rendering the P53 tumour suppressor protein dysfunctional.
CM.
The tumour suppressor pathway involving the p16/INK4a protein is important in the development of CM.58 The gene of p16/INK4a is mutated infrequently in CM, but the expression of the protein is aberrantly low in the majority of CM.59 Although CM is readily induced in mice lacking p16/Ink4a by neonatal exposure to chemical carcinogens,60–62 attempts using UV radiation have thus far not been successful.
The proliferative pathway in CM appears to involve RAS proteins. Earlier studies showed UV-B related activating mutations in RAS genes of CM from sun-exposed skin areas.63 Recently, Davies et al.64 reported that about 70% of CM carried an activating mutation in the gene that codes for BRAF, a protein which is activated by RAS. However, this mutation was not specific for UV-B radiation.
The hepatocyte growth factor stimulates proliferation of melanocytes (the normal pigment cells), and this protein also activates the RAS/BRAF pathway. Transgenic mice, overexpressing the hepatocyte growth factor in the skin, have melanocytes superficially in the skin (at the epidermal-dermal junction), a position at which they are found in human skin. In contrast, the melanocytes of normal mice (and other rodents) are located deeper in the skin (in the dermis and in hair follicles). Neonatal exposure of these transgenic mice to broadband UV radiation (including UV-B) was found to result in (metastatic) CM in adulthood. As in humans, these CM stemmed from superficial melanocytes.65 Remarkably, chronic exposure throughout adulthood did not induce CM, but mainly SCC.66 Similar results were obtained in a model using opossums (Monodelphis domestica) where 3 weeks of moderate UV-B exposure of sucklings resulted in metastatic CM in some of these animals later in life;67 some of these CM carried UV-B specific mutations in the Ink4a gene.68A 100-fold higher UV-A exposure of the neonates did not induce CM,69 and chronic UV-B exposure of adult animals induced melanocytic lesions, but no aggressive, metastatic CM.67
These data indicate that CM arise from a defective tumour suppressive pathway involving p16/INK4a and activation of a cell proliferation pathway involving RAS/BRAF. It is, however, not clear whether and to what extent UV-B radiation disrupts either or both of these pathways in the genesis of CM in humans. Evidence from these animal models support the hypothesis that UV exposure early in life is an important factor in the subsequent development of CM, consistent with epidemiological data, specifically those data obtained from migratory studies. Furthermore, studies in the two mammalian models support a role for UV-B radiation in CM, but provide no evidence in support of UV-A (E.C. De Fabo, personal communication). Thus, they do not support earlier studies in a fish model suggesting that UV-A radiation in full sunlight was more effective than UV-B radiation in inducing melanoma (for a review of UV-A and melanoma, see ref. 70)
UV-Induced immunosuppression
It is well established that UV radiation can suppress immunity (reviewed in ref. 71) The consequences for the pathogenesis of infectious diseases, for the effectiveness of vaccination, for tumour rejection and for autoimmune diseases are largely unknown but accumulating evidence indicates that the impact could be considerable.
Although the sequence of events leading to suppression of immune responses is known to be complex, it is clear that it begins with molecules in the skin which absorb UV radiation and change their structure as a result. At present major roles are indicated for DNA and trans-urocanic acid (trans-UCA) as the initiators, the former becoming damaged and the latter isomerising to cis-UCA. As a result of these changes, many molecules located in the skin are produced, some of which are called cytokines. They act on various cell populations in the skin and lymph nodes, with the end result being effects on the functions of the two major T lymphocyte subsets which represent part of the white blood cells. The first subset called T helper 1 lymphocytes, required to control tumour growth, responses to simple chemicals, and intracellular infections such as those caused by viruses, is severely depressed. In contrast, the second subset called T helper 2 lymphocytes, needed to control extracellular infections such as those caused by many bacteria, is not depressed to the same extent, in the majority of systems analysed thus far.
The activities of these two subsets of lymphocytes are orchestrated by regulatory cells, the precise identities of which have recently been reported.72,73 These cell types, which are present in very small numbers, could be enriched from lymphoid tissues of UV-irradiated mice that had subsequently been immunised: small numbers of these T cells were capable of suppressing the response to the immunising material when placed in untreated mice. The molecular controls of ‘unresponsiveness’ are of great interest currently and this work aids in our understanding of immunological regulatory pathways. In Fig. 5, details of the steps leading from UV exposure to immunosuppression can be found.
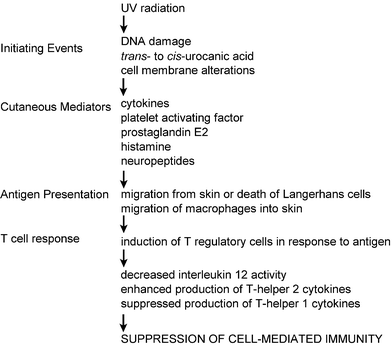 |
| Fig. 5 Details of the steps leading to UV-induced immunosuppression. | |
The impact of UV-induced immunosuppression on diseases
Herpes viruses.
For infectious diseases of human subjects, a role for UV radiation in affecting the control of a microorganism by altering the immune response of the host is most evident in the case of cold sores caused by herpes simplex virus (HSV). Here the evidence linking exposure to sunlight and reactivation of the virus from latency is very strong.74 It is interesting in the context of HSV infections to note a recent study75 reporting a seasonal variation in the incidence of shingles, caused by another herpes virus, namely herpes zoster which is also the virus causing chicken-pox. Following chicken-pox, the virus remains deep in the nervous tissue until it reactivates to give the lesions of shingles, often many years later. The frequency of shingles was found to be higher in the late spring/summer months than in the winter months. A similar study has been undertaken in Lodz, Poland.76 Again the number of cases of shingles was higher in the summer and showed a similar temporal pattern to ground level solar UV measurements. The number of zoster cases with lesions occurring on the exposed body sites (the face) varied significantly with season and peaked in July and August. In marked contrast, the majority of chicken-pox cases developed predominantly in the winter months, perhaps due to the more effective respiratory spread of the virus at this time of the year. For shingles, it could be speculated that the longer day and more sunlight in the summer lead to interference with the immune response to the virus and consequent reactivation. This hypothesis requires further testing.
Human immunodeficiency virus (HIV).
Various pieces of evidence indicate that UV exposure could affect the interaction of HIV with the host. A recent report adds further credence to this possibility.77 HIV-positive patients were given a suberythemal dose of UV-B radiation. Their skins were subsequently examined for activation of HIV which was shown to occur, most likely through the production of gene-activating factors by the host.77 An epidemiological study, in which viral load and various immunological parameters were followed over a period of 12 years in a cohort of HIV-infected homosexual men, showed a decreased number of CD4-positive T lymphocytes in the spring and summer months but no effect of seasonality on viral load.78 In addition, other immunological markers followed different seasonal courses. It should be noted, however, that to date, clinical studies involving HIV-infected patients have revealed no effect of sunlight exposure or of phototherapy on disease (reviewed in ref. 79).
Vaccination.
If UV radiation can modify immune responses to microbial antigens significantly, then various questions arise regarding vaccination. For example, does vaccination in the summer months generate less effective immunity than vaccination during the winter? Is vaccination of sun-exposed subjects less effective than vaccination of unexposed individuals? Should vaccination take place through sun-exposed body sites or should it be confined to sites which are normally covered? Is resistance to re-infection with a particular microbe lowered in the summer months? This aspect of UV-induced immunomodulation has not been investigated thoroughly as yet, although there has been interest for many years in the possible influence of season on sero-conversion in response to oral poliovirus vaccination. For example, one paper reported that, in Tel-Aviv, the rates of sero-conversion and the antibody titres generated were higher in children vaccinated in the winter compared with the summer.80 The reason for this difference was not found: exposure to sunlight was not considered. Furthermore, a decrease in the immunogenicity and replication in the upper respiratory tract of live influenza A and B vaccines in the summer compared with the winter has been reported in a study based in Leningrad.81
A Dutch group has assessed the effects of artificial UV-B irradiation on immune responses to hepatitis B virus.82 Volunteers were given whole body UV-B radiation with doses just below a sun-burning dose on each of 5 consecutive days, then vaccinated against hepatitis B virus. Although the UV-treated subjects demonstrated the typical immunosuppression observed following such exposures, such as suppression of the response to a simple chemical, the suppression did not extend to either antibody or T cell immunity specific for the virus. However, it should be noted that the vaccine used was given together with the adjuvant aluminium hydroxide, which directs the response towards the type of immunity (T helper 2) not likely to be affected by UV radiation. In addition the vaccine is administered at a high dose to encourage a protective outcome in subjects who respond poorly. This dose may have overcome any suppressive effect of UV radiation. Finally the degree of immune response to the hepatitis B vaccine is recognised to correlate with the genetic background of the individual, and small differences in, for example, the genes encoding the mediators involved in UV-induced immunosuppression were not taken into account. Preliminary results indicate that subjects with a particular polymorphism in the interleukin-1 beta gene may demonstrate UV-induced suppression of their immune responses to the hepatitis B vaccine (Sleijffers et al., personal communication).
A very interesting study examined the effect of UV radiation in subjects who had been immunised against tuberculosis at some time in the past, using a BCG vaccine.83 They were given suberythemal UV on a small area of their bodies and their immunological response to BCG tested in both this UV-exposed site and at a distant non-exposed site. Daily treatment with UV radiation for 5 consecutive days was sufficient to suppress the response at the exposed site but not at the distant site. It is not known if the depression in T cell responses induced by the UV radiation could lessen the protection offered by BCG vaccination significantly, and make individuals more prone to infection, or indeed to reactivation in the case of a persistent tuberculosis infection.
Knowledge of the immunological impact of UV radiation on vaccine effectiveness is lacking, in particular for vaccinations designed to stimulate T helper 1 responses.
Autoimmune diseases.
Several studies over the past 40 years have pointed out a marked positive correlation in the incidence of multiple sclerosis in the white Caucasian population with latitude. For example, in Australia, the prevalence of multiple sclerosis per 100,000 persons is 12 in North Queensland at latitudes of 12–23 degrees south, and 76 in Tasmania at a latitude of 43 degrees South. Recently, McMichael and Hall have suggested that this could be due to solar UV radiation levels that are negatively correlated with latitude.84 Multiple sclerosis is known to have an immunological basis with increased autoimmune T lymphocytes reactive with nerve-specific protein (myelin). Therefore increased UV exposure could perhaps ameliorate the disease by suppressing T cell function. This suggestion has gained support recently in a report where a negative association of deaths from multiple sclerosis and residential and occupational solar radiation in the United States was found.85 In contrast, deaths from non-melanoma skin cancer in the same population were positively associated with residential and occupational UV exposure. In the case of a second autoimmune disease, insulin-dependent diabetes mellitus, there are also reports of a latitude gradient in incidence. For example, in a large study of the Swedish population, there were 1.35 times more cases in the north of the country than in the south.86
Another autoimmune disease, systemic lupus erythematosus (SLE), is thought to involve a different type of T cell immunity, one which could be potentially enhanced by UV radiation causing exacerbations of symptoms. It has been reported that an increase in disease activity in 50% of photosensitive patients with SLE occurred following exposure to fluorescent lamps which emitted substantial levels of UV-B.87 A recent study in Puerto Rico examined the correlation between taking photoprotective measures and clinical outcome in patients with SLE.88 Although the wearing of a hat or long-sleeved clothes to protect from sunlight had no effect on the disease, individuals who regularly used sunblocks had lower renal involvement, thrombocytopoenia, hospitalisation and requirement for cyclophosphamide treatment than patients who had not used sunblocks. Thus the benefit of avoiding sun exposure in SLE is considerable, and it would be of great interest to monitor the immune response in such patients following sun exposure to confirm the suspected immunomodulatory effects on disease progression.
Respiratory allergy.
Concern has been expressed that UV exposure could exacerbate the symptoms of respiratory allergy, which is associated with enhanced T helper 2 responses. This hypothesis has not been tested in human subjects, but one study in mice indicated that UV irradiation did not enhance allergic reactions in the respiratory tract although it did modulate various systemic immune responses.89
Animal models.
To date approximately 15 animal models of infection have been developed, mainly using mice, in which the effects of UV radiation on immunity have been investigated (reviewed in ref. 90). The organisms range from viruses to worms, with some infections being skin-associated and others systemic with no skin involvement at any stage. In almost all cases the UV radiation caused suppression of resistance to the microorganism under investigation, the one exception being schistosomiasis.
Four models of particular interest have been described recently, all noting severe exacerbation of symptoms following UV exposure, with death ensuing in some instances. The first involved the malaria parasite. A single burning UV exposure one day before inoculation of mice resulted in the death of the infected animals, whereas, without the UV irradiation, the mice survived.91 The major immunological effect was attributed to a UV-induced alteration in the spectrum of cytokines normally induced in response to the parasite. The UNEP report in 198992 suggested that there may be a particular risk of greater incidence or severity of malaria if the terrestrial UV radiation exposure of human subjects increased. In the second model, prior exposure of mice to UV radiation was shown to enhance influenza virus infections.93 Mortality was increased up to 2-fold and was UV dose-dependent. The UV-irradiated animals demonstrated greater weight loss and degeneration of the thymus, raising concerns that sun exposure may also affect the course of human respiratory viral diseases. In the third model, rats were infected intranasally with HSV which leads to encephalitis, accompanied by severe clinical symptoms such as paralysis.94 UV-B treatment prior to such an infection increased the incidence and severity of the clinical signs. The viral load in the brain was greater and the specific immune response to the virus suppressed. Although HSV-encephalitis is rare in people, again the potential for UV exposure to have detrimental effects during the course of an infection is demonstrated. In the fourth model, mice were infected intradermally with Borrelia burgdorferi, the bacterium that causes Lyme disease. Exposure of the mice to UV radiation, either at the site of infection or at a distant site, decreased the immune response to the bacteria, increased the rate of dissemination from the skin to the bloodstream, and increased the severity of the arthritis that accompanies this infection.95 Again, this study illustrates the potential of UV irradiation to adversely affect an infectious disease of relevance to humans. In addition, the subtlety of the effects (reduced immunity and increased disease severity) points out the difficulty in identifying such effects in human populations.
Extrapolation from animal models to human subjects
It is of considerable interest to relate the observed immunological effects of UV radiation in the animal models to human subjects. The biologically effective dose for UV-induced suppression of contact hypersensitivity in a mouse model as a function of latitude and of ozone depletion was first calculated.96 Then, by using these data and by extrapolation from one animal model of infection, it has been suggested that exposure of people for about 100 minutes at mid-latitudes around noon on a clear day in summer would be sufficient to suppress their cellular immunity to a microbe significantly.97 This conclusion has yet to be verified for a natural human infection. However, the effective solar dose for the suppression of the immune response to a simple chemical in human subjects has been established recently and is equivalent to approximately 60 minutes of noonday summer sunlight at mid-latitudes98—of the same order as the theoretical extrapolation. Therefore it is likely that the doses of solar UV radiation required to suppress immunity to infectious agents are of the same magnitude as those experienced during common outdoor activities.
The impact of UV-induced immunosuppression on tumours
Several studies in the past have indicated that human subjects can be divided into those who are susceptible to the immunosuppressive effects of UV radiation and those who are resistant.99 The former were thought to be at higher risk of developing skin cancer than the latter. More recently Kelly et al have shown that simulated solar radiation is highly immunosuppressive in every person tested.98 A further report by the same group examined the relationship between sunburn and immunomodulation.100 Suppression occurred in all subjects by exposure to solar irradiation equivalent to 1 hour of noonday summer sunlight at mid-latitudes one day before application of the chemical at the irradiated site. A correlation was demonstrated between erythema (redness) and suppression of immunity. However, if the UV dose was reduced to below the minimum required to cause erythema of the skin, differences in immunomodulation were revealed, depending on the skin phototype of the individual. People who do not tan readily and are sun-sensitive (type I/II) were 2–3 fold more susceptible to immunosuppression than people who tan readily and are sun-tolerant (type III/IV). It is known that individuals with the former skin type are at greater risk of developing skin cancer than individuals with the latter skin type. Thus the greater sensitivity of pale skinned subjects for a given level of exposure may play a role in their increased risk of developing skin cancer.
Several studies have indicated an important role for mast cells in UV-induced systemic immunosuppression. Hart et al. used strains of mice with differing susceptibilities to immunomodulation following UV radiation, and showed a correlation of susceptibility with the number of mast cells in the skin.101 Thus a strain in which immunity was easily suppressed by UV exposure had a large number of mast cells, while another strain, in which immunity was only suppressed by large UV-B doses, had about half that number. Mast cells contain granules containing histamine and other pharmacological mediators, which can be released upon sun exposure or other stimulation, thus affecting local immunity and cell trafficking. Most interestingly, a subsequent publication by the same group showed that human subjects with a past history of BCC had higher numbers of cutaneous mast cells than matched controls, suggesting a link between UV-induced immunosuppression and skin cancer.102 There is preliminary evidence that cis-UCA, one of the initiators of UV-induced immunosuppression, may trigger mast cell degranulation.103 In fact recent evidence suggests that cis-UCA may cause mast cell degranulation by mimicking the action of serotonin (J. Walterscheid, personal communication). However, the relationship between UCA and the risk of developing skin cancer, if it exists, is not likely to be a straightforward one, as no difference in total UCA or the percentage of cis-UCA was found in subjects with a past history of CM and BCC compared with control subjects.104,105
Finally a study by Beissert et al., has revealed that cis-UCA may be an important factor in photocarcinogenesis.106 Mice chronically exposed to UV-B radiation were treated concurrently either with a monoclonal antibody effective in reversing the immunosuppressive effects of cis-UCA or a non-related monoclonal antibody. In the mice receiving the cis-UCA antibody, tumours took twice as long to develop as in mice receiving the unrelated antibody.
Other systemic effects from UV exposure
UV exposure of the skin is known to cause effects throughout the body by substances released into the circulation or cells migrating to and from the skin. Over the last decade data have been collected indicating that solar UV exposure may influence the development of internal cancers. UV radiation is unlikely to cause internal cancers by direct DNA damage, but it may stimulate or suppress the outgrowth of internal cancers.
Immune suppression (such as that induced by medication in renal transplant patients) is a known risk factor for both SCC and non-Hodgkin's lymphoma (NHL). Like skin cancers, the incidence of NHL has been increasing. BCC and SCC appear to be associated with increased risk of NHL and chronic lymphocytic leukaemia (CLL)107–110 suggestive of a common aetiological factor, presumably solar UV radiation. Similarly, NHL incidences were found to be higher in populations in sunnier areas,111–114 though this relationship was not demonstrated in the United States.115 Besides UV exposure, the impact of socio-economic factors on NHL risk needs to be taken into account (Langford et al., 1998, Schouten et al., 1996).113,116 Assessments of personal UV exposure have not revealed clear, significant associations with NHL,114 at most, they show trends of borderline significance with NHL and leukaemia.117,118 However, such exposure assessments may have been too crude or inadequate (e.g. assessing total instead of peak exposures) to reveal striking differences. An indication that sun (UV) exposure may affect lymphocytes adversely was found in the increase in mutation frequency in these blood-borne cells during the summer,119 presumably due to the UV-induced release of a factor that stimulated lymphocyte proliferation. Furthermore, UV exposure of certain genetically modified animals (missing one copy of the P53 tumor suppressor gene) greatly enhance the development of leukaemia in addition to causing SCC in the skin,120 suggesting that UV exposure may enhance the development of lymphoid tumours in genetically predisposed individuals.
UV-B radiation contributes to the formation of vitamin D in the skin. This vitamin is not biologically active, but the ‘vitamin D hormone’
(1,25-dihydroxyvitamin D) derived from it is. The vitamin D hormone can inhibit the growth of various cancer cells (e.g. breast cancer) in cell culture, and some studies have demonstrated that the risk of certain types of cancer (colon, prostate and breast) increases with low dietary intake of vitamin D or with low levels of vitamin D metabolites in the blood (see ref. 121 for an overview). It is possible that UV exposure could influence the blood level of the vitamin D hormone, but there is no simple direct relationship between vitamin D hormone and UV exposure because of the many regulatory feedback mechanisms. Following an earlier report of a very slight, but significant latitude gradient in prostate cancer across the USA,122 a recent case-control study123 showed that low levels of sun exposure are associated with a higher risk of prostate cancer. Within the group of prostate cancer patients, the individuals with the lowest level of UV exposure (within the lowest 25 percentile) developed their tumours at a median age of 68 years, whereas the others developed them at a median age of 72 years. It has also been suggested that a low level of vitamin D could contribute to the risk of developing insulin-dependent diabetes mellitus.124
Risks associated with the use of substitutes for ozone depleting substances
With the phase-out of ozone depleting substances (ODS) mandated under the Montreal Protocol and its amendments, a number of new chemical alternatives have been developed, and there has been increased usage of some older chemicals, often in venues different from their traditional usage. These increases in usage are likely to lead to increased human (and environmental) exposures that could have adverse consequences for both human health and the environment. Previous assessments have presented brief reviews of what was known about the toxicology of a number of these chemicals. As a consequence this review will focus on those chemicals not previously mentioned in earlier reports that are discussed in the most recent Technology and Economic Assessment Panel (TEAP) report.125 A list of these chemicals with their CAS (Chemical Abstracts Service) numbers and their likely use is presented in Table 1.
Table 1 CFC substitutes
Common name |
Chemical name |
CAS No |
Likely use |
Reference |
HFC 245fa |
1,1,1,2,3,3,3-Heptafluoropropane |
431-89-0 |
Medical aerosols
Fire-fighting agent
|
www.fluorocarbons.org |
HFC 365mfc |
1,1,13,3-Pentafluorobutane |
406-58-6 |
Foam blowing agent |
″ |
n-Propyl bromide |
|
|
|
|
HFC 43–10mee |
1,1,1,2,2,3,4,5,5,5-Decafluoropentane |
138495-42-8 |
Solvent |
|
HFC-236fa |
1,1,13,3,3-Hexafluoropropane |
290-39-1 |
Fire-fighting agent |
|
1,3-Dichloropropene |
|
542-75-6 |
Fumigant |
|
Chloropicrin |
Trichlornitromethane |
76-06-2 |
Fumigant |
|
Furfural |
|
98-01-1 |
Pesticide |
|
Methyl isocyanate |
|
624-83-9 |
Pesticide |
|
Methyl iodide |
|
74-88-4 |
Pesticide |
|
Fosthiazate |
Phosphonothioic acid, (2-oxo-3-thiazolidinyl)-,)-ethyl S-(1-methylpropyl) ester |
98886-44-3 |
Pesticide |
|
Enzone |
Sodium tetrathiocarbonate |
|
Pesticide |
|
Avermectin |
|
73989-17-0 |
Pesticide |
|
propargyl bromide |
|
106-96-7 |
Pesticide |
|
potassium azide |
|
20762-60-1 |
Pesticide |
|
Sulfuryl fluoride |
|
2699-79-8 |
Pesticide |
|
Ethyl formate |
|
109-94-4 |
Fumigant |
|
Propylene oxide |
|
75-56-9 |
Fumigant |
|
Carbonyl sulfide |
|
463-58-1 |
Fumigant |
|
R-407C |
Mixture of HFC 32, HFC-134a & HFC-125 |
|
Refrigerant |
|
R-410A |
Mixture of HFC-32, HFC-125 |
|
Refrigerant |
|
R-404A |
Mixture of HFC-125, HFC-134a & HCFC-143a |
|
Refrigerant |
|
R-500 |
|
|
Refrigerant |
|
R-1270 |
Propylene |
115-07-1 |
Refrigerant |
|
HC-600a |
Isobutane |
75-26-5 |
Refrigerant |
|
Ethylene dichloride |
|
107-6-2 |
|
|
Phosphine |
|
7803-51-2 |
Pesticide |
|
Currently ODS substitutes are being used in six major areas: 1) Aerosols, 2) Refrigerants, 3) Fire-suppressants, 4) Solvents, 5) Foam-blowing agents, and 6) Pesticides, including fumigants, all of which are represented in Table 1. All of these uses have the potential for human exposure, mainly for occupationally exposed populations, but also for the public in general.
The largest use of aerosols still requiring ODS is in the metered dose inhalers used to deliver pharmaceuticals to the respiratory system. Substitutes for use in these inhalers are being introduced; their production and use will continue to increase as the essential use exemption for the ODS is phased out. Of all the substitutes under consideration to replace ODS, those to be used in metered dose inhalers will be under the greatest scrutiny for adverse health effects, and it seems likely that any risks associated with their use will be correspondingly small.
Quite the reverse is likely to be true of the chemical pesticides, most of which will see increased use because of the phase-out of methyl bromide. Pesticides and fumigants have been designed to be very toxic. Thus, exposures to substitutes for these agents are likely to result in higher risks than exposure to the same levels of the substitutes used in the other areas. However, pesticides for the most part are closely regulated (and all of those identified in Table 1 have seen considerable use) so presumably these exposures and their associated risks will also be subjected to a higher degree of control than those chemicals not regulated as pesticides. It should be noted, however, that there are parts of the world where stringent pesticide regulation is lacking. In these locations, misuse of the substitutes (or for that matter any chemical pesticide) has the potential for significant adverse effects.
The remaining four groups of chemicals are much more likely to pose risks to occupationally exposed populations than the general public, and even then the risks from most of these chemicals appear to be low. Most of the chemicals listed below as substitutes for refrigerants, fire suppressants, solvents and foam-blowing agents have a low degree of acute toxicity. Furthermore, although the database on these chemicals is somewhat limited, what is available suggests that they will also demonstrate a low degree of activity as carcinogens. The one possible exception to this low degree of toxicity is n-propyl bromide, a bromine-containing compound that is being marketed as a replacement for many of the solvent uses of CFC. This chemical, which is becoming more and more widely used, is now recognized for its toxicity to the nervous and reproductive systems, as well as to the foetus.125
For a number of uses of ODS substitutes have not been identified.125 Although some of these involve the use of CFC, e.g., the use of CFC-113 as a solvent in the production of fluoropolymer resins, most relate to the use of carbon tetrachloride as a solvent in the production of various chemicals ranging from pharmaceuticals to a number of different kinds of polymers. Clearly when substitutes are found for these ODS they will need to be closely evaluated to ensure that one problem is not being substituted for another.
Possible interactions between climate change and ozone depletion
At the same time as the world is anticipating at least another 50 years of depleted ozone, another threat, namely increased atmospheric CO2 leading to global climate change (GCC), has been identified as having the potential to affect the world's environment for generations to come. For the time period when these two threats co-exist, the likelihood is great that interactive effects on human health will occur. This section will attempt to identify possible interactions between ozone depletion and GCC. It should be noted at the outset, however, that there are few experimental data from systems exploring the issue of how these threats may interact and how such interactions may affect human health. Thus most of the following discussion should be viewed as informed speculation. It should also be noted that one of the most likely impacts of both ozone depletion and GCC will be changes in human behaviour. Human behaviour will also change as nations develop economically. The inability to predict human behaviour adds a large degree of uncertainty to the prognostications provided below.
Impact of UV radiation and climate change on the eye
The prevalence of nuclear cataract is reported to be higher in tropical and subtropical regions where there is a high annual UV exposure rate coupled with high ambient temperature and infrared exposure. Unlike the skin, the temperature of the lens is more related to core body temperature than to external temperature, and little direct synergism of temperature with UV radiation would be anticipated. Other heat-induced systemic factors, which may indirectly affect lens nutrition and metabolism could, on the other hand, potentially accelerate cataract development.
A recent study evaluating the effect of environmental temperature on cataract progression in rats126 found no interaction between UV radiation and environmental temperature in UV-induced cortical cataract (including anterior subcapsular cataract). This suggests that the higher prevalence of nuclear cataract in regions near to the equator may be due primarily to other factors. The role of ambient temperature in influencing these factors has yet to be adequately investigated.
Impact of UV radiation and climate change on the skin
Results from one atmospheric model suggested that cooling of the stratosphere could delay the recovery of the ozone layer by a decade or more.127 Later models have led to a variety of conclusions, ranging from no delay at all to a resurgence of ozone depletion late in this century.3 The possibility of a delayed recovery gives reason for concern about the consequences, especially in the long-term. Before this problem was noted, a scenario study had been made for the increase in skin cancer incidence due to ozone depletion during the entire present century.2,128 Under the most optimistic conditions of full and worldwide compliance with the Montreal Protocol and all its amendments, the excess incidence would peak about the year 2055 at 9% above the baseline incidence, and then gradually decline. A similar scenario study has been performed recently, but now including a delay in the recovery of the ozone layer by 15–20 years,129Fig. 6. This showed that the excess incidence at the time of the peak would increase to 15%, and the peak itself would shift from 2055 to 2065.
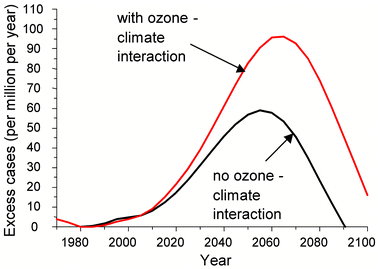 |
| Fig. 6 Increase in skin cancer incidence in North-Western Europe, with ozone–climate interaction causing 15–20 years delay (worst case scenario) in recovery of the ozone layer, and without any delay from an ozone-climate interaction.129 | |
Experiments performed many years ago130,131 demonstrated that mice exposed to UV radiation at increased room temperature developed skin cancers at an accelerated rate. From the data given in these papers, van der Leun and de Gruijl132 calculated an average increase in the carcinogenic effectiveness of the UV radiation with a 5% per degree C increase in temperature. This result was then applied to skin cancer in the human population—firstly, to the excess incidence caused by ozone depletion. The conclusion was that a 2 degree rise in temperature would increase the peak excess from the 9% calculated by Slaper et al.128 to 11%, and a 4 degree rise would increase it to 13%. Secondly, the effect on the baseline incidence itself was considered, without any ozone depletion. A persistent increase of temperature by 2 degrees C was calculated to increase the baseline incidence by 21%, and a 4 degree C rise by 46%.
It should be noted that, while UV carcinogenesis in mice and humans is basically similar, there are important quantitative differences, and the same may be true for the influence of higher temperatures. Direct data relating temperature and UV carcinogenesis in human populations are not yet available. The experimental mice were exposed to the higher temperatures constantly, a procedure which is rather different from normal human behaviour where people are unlikely to experience increased temperatures all the time. It is also unknown at what stage the temperature exerts its critical influence. This could be during the exposure to UV radiation, or during the time between exposures, or both. The calculation is also complicated by the fact that the observed temperature influence was non-linear: in the mouse experiment there was little effect below the normal room temperature of 23 degrees centigrade, and a marked effect at the higher temperatures. Despite all these uncertainties, the influence of increased temperatures on carcinogenesis in the human population merits further consideration and could be of importance.
Impact of UV radiation and climate change on the immune response
The potential consequences of GCC with regard to human health have been the subject of numerous reviews.133–135 For the most part it seems that any increases in such effects that might occur will be in those diseases whose incidences are increased in the tropics or with warm weather in other places. However, it is also clear that in most locations, there will only be an effect of GCC on disease if the disease already exists at that location or in a location that is geographically contiguous. Although there has been much concern that malaria may increase with GCC perhaps in the developed nations as well as the developing ones,135 it has been contended that most developed nations conquered the disease in their past and are unlikely to see a serious resurgence.
Longstreth136 examined the diseases found in the US that showed a seasonal variation and identified a number of endpoints that could potentially be affected by the changes in temperature and/or humidity likely to be associated with GCC. These included a variety of infectious diseases, e.g., viral encephalitis, Hanta virus pulmonary syndrome, as well as a number of other health endpoints such as increased asthma due to increased air pollution, shellfish poisonings, morbidity and mortality from extreme events, and heat stress.
To the extent that such diseases are increased by the changes in weather associated with GCC, it seems likely that many of them could be additionally exacerbated by elevated levels of UV radiation associated with a thinner stratospheric ozone layer. If climatic conditions become more favourable for the transmission of a particular endemic infectious disease, for example malaria, increased ambient UV radiation due to a depleted ozone layer may lead to more severe cases of the disease due to suppression of the immune system.
References
-
J. C. van der Leun, M. Tevini, X. Tang and R. C. Worrest, Environmental Effects of Ozone Depletion; 1998 assessment, United Nations Environment Programme, Nairobi, 1998, p. 192 Search PubMed.
- J. Longstreth, F. R. de Gruijl, M. L. Kripke, S. Abseck, F. Arnold, H. I. Slaper, G. Velders, Y. Takizawa and J. C. van der Leun, Health risks, J. Photochem. Photobiol. B, 1998, 46, 20–39 Search PubMed.
-
R. L. McKenzie, L. O. Bjorn, A. Bais and M. Ilyas, Changes in Biologically active ultraviolet radiation reaching the Earth's surface, Photochem. Photobiol. Sci., 2003, this issue (DOI:10.1039/b21155c) Search PubMed.
- D. H. Sliney, Geometrical assessment of ocular exposure to environmental UV radiation—implications for ophthalmic epidemiology, J. Epidemiol., 1999, 9, S22–32 Search PubMed.
- J. A. Van Best, J. L. Van Delft and J. E. Keunen, Long term follow-up of lenticular autofluorescence and transmittance in healthy volunteers, Exp. Eye. Res., 1998, 66, 117–123 CrossRef CAS.
- H. Sasaki, O. Hockwin, T. Kasuga, K. Nagai, Y. Sakamoto and K. Sasaki, An index for human lens transparency related to age and lens layer: comparison between normal volunteers and diabetic patients with still clear lenses, Ophthalmic Res., 1999, 31, 93–103 Search PubMed.
- S. Fu, R. Dean, M. Southan and R. Truscott, The hydroxyl radical in lens nuclear cataractogenesis, J. Biol. Chem., 1998, 273, 28603–28609 CrossRef CAS.
- Y. Berry and R. J. Truscott, The presence of a human UV filter within the lens represents an oxidative stress, Exp. Eye. Res., 2001, 72, 411–421 CrossRef CAS.
- J. W. Eaton, UV-mediated cataractogenesis: a radical perspective, Doc. Ophthalmol., 1994, 88, 233–242.
- J. Dillon, UV-B as a pro-aging and pro-cataract factor, Doc. Ophthalmol., 1994, 88, 339–344.
- K. W. Lee, N. Meyer and B. J. Ortwerth, Chromatographic comparison of the UVA sensitizers present in brunescent cataracts and in calf lens proteins ascorbylated in vitro, Exp. Eye. Res., 1999, 69, 375–384 CrossRef CAS.
- H. R. Taylor, Epidemiology of age-related cataract, Eye, 1999, 13, 445–448 Search PubMed.
- L. C. Hayashi, N. Tamiya and E. Yano, Correlation between UVB irradiation and the proportion of cataract–an epidemiological study based on a nationwide patient survey in Japan, Ind. Health, 1998, 36, 354–360 Search PubMed.
- H. R. Taylor, S. K. West, F. S. Rosenthal, B. Munoz, H. S. Newland, H. Abbey and E. A. Emmett, Effect of ultraviolet radiation on cataract formation, N. Engl. J. Med., 1988, 319, 1429–1433 CAS.
- F. Hollows and D. Moran, Cataract—the ultraviolet risk factor, Lancet, 1981, 2, 1249–1250 CrossRef CAS.
- R. Hiller, R. D. Sperduto and F. Ederer, Epidemiologic associations with cataract in the 1971–1972 National Health and Nutrition Examination Survey, Am. J. Epidemiol., 1983, 118, 239–249 CAS.
- C. A. McCarty, B. N. Mukesh, C. L. Fu and H. R. Taylor, The epidemiology of cataract in Australia, Am. J. Opthalmol., 1999, 128, 446–465 Search PubMed.
- K. Sasaki, H. Sasaki, M. Kojima, Y. B. Shui, O. Hockwin, F. Jonasson, H. M. Cheng, M. Ono and N. Katoh, Epidemiological studies on UV-related cataract in climatically different countries, J. Epidemiol., 1999, 9, S33–38 Search PubMed.
- S. K. West, D. D. Duncan, B. Munoz, G. S. Rubin, L. P. Fried, K. Bandeen-Roche and O. D. Schein, Sunlight exposure and risk of lens opacities in a population-based study: the Salisbury Eye Evaluation project, JAMA, 1998, 280, 714–718 Search PubMed.
- N. Katoh, F. Jonasson, H. Sasaki, M. Kojima, M. Ono, N. Takahashi and K. Sasaki, Cortical lens opacification in Iceland. Risk factor analysis—Reykjavik Eye Study, Acta Ophthalmol. Scand., 2001, 79, 154–159 CrossRef CAS.
- S. K. West, Ocular ultraviolet B exposure and lens opacities; a review, J. Epidemiol., 1999, 9, S97–101 Search PubMed.
- O. Hockwin, M. Kojima, Y. Sakamoto, A. Wegener, Y. B. Shui and K. Sasaki, UV damage to the eye lens: further results from animal model studies: a review, J. Epidemiol., 1999, 9, S39–47 Search PubMed.
- D. G. Pitts, A. P. Cullen and P. D. Hacker, Ocular effects of ultraviolet radiation from 295 to 365 nm, Invest. Ophthalmol. Vis. Sci., 1977, 16, 932–939 Search PubMed.
- J. C. Merriam, S. Lofgren, R. Michael, P. Soderberg, J. Dillon, L. Zheng and M. Ayala, An action spectrum for UV-B radiation and the rat lens, Invest. Ophthalmol. Vis. Sci., 2000, 41, 2642–2647 Search PubMed.
- O. M. Oriowo, A. P. Cullen, B. R. Chou and J. G. Sivak, Action spectrum and recovery for in vitro UV-induced cataract using whole lenses, Invest. Ophthalmol. Vis. Sci., 2001, 42, 2596–2602 Search PubMed.
- J. F. Abarca, C. C. Casiccia and F. D. Zamorano, Increase in sunburns and photosensitivity disorders at the edge of the Anartic ozone hole, Southern Chile, 1986–2000, J. Am. Acad. Dermatol., 2002, 46, 193–199 CrossRef.
- A. R. Young, C. A. Chadwick, G. I. Harrison, O. Nikaido, J. Ramsden and C. S. Potten, The similarity of action spectra for thymine dimers in human epidermis and erythema suggests that DNA is the chromophore for erythema, J. Invest. Dermatol., 1998, 111, 982–988 CrossRef CAS.
- R. J. Berg, H. J. Ruven, A. T. Sands, F. R. de Gruijl and L. H. Mullenders, Defective global genome repair in XPC mice is associated with skin cancer susceptibility but not with sensitivity to UVB induced erythema and edema, J. Invest. Dermatol., 1998, 110, 405–409 CrossRef CAS.
- C. Kielbassa, L. Roza and B. Epe, Wavelength dependence of oxidative DNA damage induced by UV and visible light, Carcinogenesis, 1997, 18, 811–816 CrossRef CAS.
- F. R. de Gruijl and J. C. van der Leun, Estimate of the wavelength dependency of ultraviolet carcinogenesis in humans and its relevance to the risk assessment of a stratospheric ozone depletion, Health Phys., 1994, 67, 319–325 Search PubMed.
- M. Horiguchi, K. I. Masumura, H. Ikehata, T. Ono, Y. Kanke and T. Nohmi, Molecular nature of ultraviolet B light-induced deletions in the murine epidermis, Cancer Res., 2001, 61, 3913–3918 Search PubMed.
- R. A. Keulers, A. R. de Roon, S. de Roode and A. D. Tates, The induction and analysis of micronuclei and cell killing by ultraviolet-B radiation in human peripheral blood lymphocytes, Photochem. Photobiol., 1998, 67, 426–432 Search PubMed.
- G. Emri, E. Wenczl, P. Van Erp, J. Jans, L. Roza, I. Horkay and A. A. Schothorst, Low doses of UVB or UVA induce chromosomal aberrations in cultured human skin cells, J. Invest. Dermatol., 2000, 115, 435–440 CrossRef CAS.
- C. Masutani, R. Kusumoto, A. Yamada, N. Dohmae, M. Yokoi, M. Yuasa, M. Araki, S. Iwai, K. Takio and F. Hanaoka, The XPV (xeroderma pigmentosum variant) gene encodes human DNA polymerase eta, Nature, 1999, 399, 700–704 CrossRef CAS.
- C. L. Limoli, E. Giedzinski, W. F. Morgan and J. E. Cleaver, Inaugural article: polymerase eta deficiency in the xeroderma pigmentosum variant uncovers an overlap between the S phase checkpoint and double-strand break repair, Proc. Natl. Acad. Sci. USA, 2000, 97, 7939–7946 CrossRef CAS.
- P. Wolf, H. Maier, R. R. Mullegger, C. A. Chadwick, R. Hofmann-Wellenhof, H. P. Soyer, A. Hofer, J. Smolle, M. Horn, L. Cerroni, D. Yarosh, J. Klein, C. Bucana, K. Dunner, Jr., C. S. Potten, H. Honigsmann, H. Kerl and M. L. Kripke, Topical treatment with liposomes containing T4 endonuclease V protects human skin in vivo from ultraviolet-induced upregulation of interleukin-10 and tumor necrosis factor-alpha, J. Invest. Dermatol., 2000, 114, 149–156 CrossRef CAS.
- H. Stege, L. Roza, A. A. Vink, M. Grewe, T. Ruzicka, S. Grether-Beck and J. Krutmann, Enzyme plus light therapy to repair DNA damage in ultraviolet-B-irradiated human skin, Proc. Natl. Acad. Sci. USA, 2000, 97, 1790–1795 CrossRef CAS.
- A. Hannuksela-Svahn, E. Pukkala and J. Karvonen, Basal cell skin carcinoma and other nonmelanoma skin cancers in Finland from 1956 through 1995, Arch. Dermatol., 1999, 135, 781–786 CAS.
- M. Staples, R. Marks and G. Giles, Trends in the incidence of non-melanocytic skin cancer (NMSC) treated in Australia 1985–1995: are primary prevention programs starting to have an effect?, Int. J. Cancer, 1998, 78, 144–148 CrossRef CAS.
-
IARC, Solar and ultraviolet radiation, Vol. 55, International Agency for Research on Cancer, Lyon, 1992 Search PubMed.
- S. A. Holme, K. Malinovszky and D. L. Roberts, Changing trends in non-melanoma skin cancer in South Wales, 1988–98, Brit. J. Dermatol., 2000, 143, 1224–1229 CrossRef CAS.
- I. Plesko, G. Severi, A. Obsitnikova and P. Boyle, Trends in the incidence of non-melanoma skin cancer in Slovakia, 1978–1995, Neoplasma, 2000, 47, 137–142 Search PubMed.
- R. B. Harris, K. Griffith and T. E. Moon, Trends in the incidence of nonmelanoma skin cancers in southeastern Arizona, 1985–1996, J. Am. Acad. Dermatol., 2001, 45, 528–536 CrossRef CAS.
- J. Scotto, H. Pitcher and J. A. Lee, Indications of future decreasing trends in skin-melanoma mortality among whites in the United States, Int. J. Cancer, 1991, 49, 490–497 CAS.
- R. M. MacKie, D. Hole, J. A. Hunter, R. Rankin, A. Evans, K. McLaren, M. Fallowfield, A. Hutcheon and A. Morris, Cutaneous malignant melanoma in Scotland: incidence, survival, and mortality, 1979–94. The Scottish Melanoma Group, Brit. Med. J., 1997, 315, 1117–1121 Search PubMed.
- G. G. Giles, B. K. Armstrong, R. C. Burton, M. P. Staples and V. J. Thursfield, Has mortality from melanoma stopped rising in Australia? Analysis of trends between 1931 and 1994, Brit. Med. J., 1996, 312, 1121–1125 Search PubMed.
- G. Severi, G. G. Giles, C. Robertson, P. Boyle and P. Autier, Mortality from cutaneous melanoma: evidence for contrasting trends between populations, Brit. J. Cancer., 2000, 82, 1887–1891 Search PubMed.
- A. Green, G. Williams, R. Neale, V. Hart, D. Leslie, P. Parsons, G. C. Marks, P. Gaffney, D. Battistutta, C. Frost, C. Lang and A. Russell, Daily sunscreen application and betacarotene supplementation in prevention of basal-cell and squamous-cell carcinomas of the skin: a randomised controlled trial, Lancet, 1999, 354, 723–729 CrossRef CAS.
- D. Yarosh, J. Klein, A. O'Connor, J. Hawk, E. Rafal and P. Wolf, Effect of topically applied T4 endonuclease V in liposomes on skin cancer in xeroderma pigmentosum: a randomised study. Xeroderma Pigmentosum Study Group, Lancet, 2001, 357, 926–929 CrossRef CAS.
- A. S. Jonason, S. Kunala, G. J. Price, R. J. Restifo, H. M. Spinelli, J. A. Persing, D. J. Leffell, R. E. Tarone and D. E. Brash, Frequent clones of p53-mutated keratinocytes in normal human skin, Proc. Natl. Acad. Sci. USA, 1996, 93, 14025–14029 CrossRef CAS.
- Z. P. Ren, F. Ponten, M. Nister and J. Ponten, Two distinct p53 immunohistochemical patterns in human squamous-cell skin cancer, precursors and normal epidermis, Int. J. Cancer, 1996, 69, 174–179 CrossRef CAS.
- H. Rebel, L. O. Mosnier, R. J. Berg, A. Westerman-de Vries, H. van Steeg, H. J. van Kranen and F. R. de Gruijl, Early p53-positive foci as indicators of tumor risk in ultraviolet-exposed hairless mice: kinetics of induction, effects of DNA repair deficiency, and p53 heterozygosity, Cancer Res., 2001, 61, 977–983 Search PubMed.
- H. Hahn, C. Wicking, P. G. Zaphiropoulous, M. R. Gailani, S. Shanley, A. Chidambaram, I. Vorechovsky, E. Holmberg, A. B. Unden, S. Gillies, K. Negus, I. Smyth, C. Pressman, D. J. Leffell, B. Gerrard, A. M. Goldstein, M. Dean, R. Toftgard, G. Chenevix-Trench, B. Wainwright and A. E. Bale, Mutations of the human homolog of Drosophila patched in the nevoid basal cell carcinoma syndrome, Cell, 1996, 85, 841–851 CrossRef CAS.
- A. Kamb, N. A. Gruis, J. Weaver-Feldhaus, Q. Liu, K. Harshman, S. V. Tavtigian, E. Stockert, R. S. Day, 3rd, B. E. Johnson and M. H. Skolnick, A cell cycle regulator potentially involved in genesis of many tumor types, Science, 1994, 264, 436–440 CAS.
- N. Dahmane, J. Lee, P. Robins, P. Heller and A. Ruiz i Altaba, Activation of the transcription factor Gli1 and the Sonic hedgehog signalling pathway in skin tumours, Nature, 1997, 389, 876–881 CrossRef CAS.
- M. R. Gailani, M. Stahle-Backdahl, D. J. Leffell, M. Glynn, P. G. Zaphiropoulos, C. Pressman, A. B. Unden, M. Dean, D. E. Brash, A. E. Bale and R. Toftgard, The role of the human homologue of Drosophila patched in sporadic basal cell carcinomas, Nat. Genet., 1996, 14, 78–81 CrossRef CAS.
- M. Aszterbaum, J. Epstein, A. Oro, V. Douglas, P. E. LeBoit, M. P. Scott and E. H. Epstein, Jr., Ultraviolet and ionizing radiation enhance the growth of BCCs and trichoblastomas in patched heterozygous knockout mice, Nat. Med., 1999, 5, 1285–1291 CrossRef CAS.
- M. Ruas and G. Peters, The p16INK4a/CDKN2A tumor suppressor and its relatives, Biochim. Biophys. Acta, 1998, 1378, F115–177 CAS.
- J. O. Funk, P. I. Schiller, M. T. Barrett, D. J. Wong, P. Kind and C. A. Sander, p16INK4a expression is frequently decreased and associated with 9p21 loss of heterozygosity in sporadic melanoma, J. Cutan. Pathol., 1998, 25, 291–296 CAS.
- M. Serrano, H. Lee, L. Chin, C. Cordon-Cardo, D. Beach and R. A. DePinho, Role of the INK4a locus in tumor suppression and cell mortality, Cell, 1996, 85, 27–37 CrossRef CAS.
- P. Krimpenfort, K. C. Quon, W. J. Mooi, A. Loonstra and A. Berns, Loss of p16Ink4a confers susceptibility to metastatic melanoma in mice, Nature, 2001, 413, 83–86 CrossRef CAS.
- N. E. Sharpless, N. Bardeesy, K. H. Lee, D. Carrasco, D. H. Castrillon, A. J. Aguirre, E. A. Wu, J. W. Horner and R. A. DePinho, Loss of p16Ink4a with retention of p19Arf predisposes mice to tumorigenesis, Nature, 2001, 413, 86–91 CrossRef CAS.
- A. van Elsas, S. F. Zerp, S. van der Flier, K. M. Kruse, C. Aarnoudse, N. K. Hayward, D. J. Ruiter and P. I. Schrier, Relevance of ultraviolet-induced N-ras oncogene point mutations in development of primary human cutaneous melanoma, Am. J. Pathol., 1996, 149, 883–893 Search PubMed.
- H. Davies, G. R. Bignell, C. Cox, P. Stephens, S. Edkins, S. Clegg, J. Teague, H. Woffendin, M. J. Garnett, W. Bottomley, N. Davis, E. Dicks, R. Ewing, Y. Floyd, K. Gray, S. Hall, R. Hawes, J. Hughes, V. Kosmidou, A. Menzies, C. Mould, A. Parker, C. Stevens, S. Watt, S. Hooper, R. Wilson, H. Jayatilake, B. A. Gusterson, C. Cooper, J. Shipley, D. Hargrave, K. Pritchard-Jones, N. Maitland, G. Chenevix-Trench, G. J. Riggins, D. D. Bigner, G. Palmieri, A. Cossu, A. Flanagan, A. Nicholson, J. W. Ho, S. Y. Leung, S. T. Yuen, B. L. Weber, H. F. Seigler, T. L. Darrow, H. Paterson, R. Marais, C. J. Marshall, R. Wooster, M. R. Stratton and P. A. Futreal, Mutations of the BRAF gene in human cancer, Nature, 2002, 417, 949–954 CrossRef CAS.
- F. P. Noonan, J. A. Recio, H. Takayama, P. Duray, M. R. Anver, W. L. Rush, E. C. De Fabo and G. Merlino, Neonatal sunburn and melanoma in mice, Nature, 2001, 413, 271–272 CrossRef CAS.
- F. P. Noonan, T. Otsuka, S. Bang, M. R. Anver and G. Merlino, Accelerated ultraviolet radiation-induced carcinogenesis in hepatocyte growth factor/scatter factor transgenic mice, Cancer Res., 2000, 60, 3738–3743 Search PubMed.
- E. S. Robinson, G. B. Hubbard, G. Colon and J. L. Vandeberg, Low-dose ultraviolet exposure early in development can lead to widespread melanoma in the opossum model, Int. J. Exp. Pathol., 1998, 79, 235–244 CAS.
- J. Chan, E. S. Robinson, J. Atencio, Z. Wang, S. Kazianis, L. D. Coletta, R. S. Nairn and J. R. McCarrey, Characterization of the CDKN2A and ARF genes in UV-induced melanocytic hyperplasias and melanomas of an opossum (Monodelphis domestica), Mol. Carcinog., 2001, 31, 16–26 CrossRef CAS.
- E. S. Robinson, R. H. Hill, Jr., M. L. Kripke and R. B. Setlow, The Monodelphis melanoma model: initial report on large ultraviolet A exposures of suckling young, Photochem. Photobiol., 2000, 71, 743–746 Search PubMed.
- S. Q. Wang, R. Setlow, M. Berwick, D. Polsky, A. A. Marghoob, A. W. Kopf and R. S. Bart, Ultraviolet A and melanoma: a review, J. Am. Acad. Dermatol., 2001, 44, 837–846 CrossRef CAS.
- G. J. Clydesdale, G. W. Dandie and H. K. Muller, Ultraviolet light induced injury: immunological and inflammatory effects, Immunol. Cell Biol., 2001, 79, 547–568 CrossRef CAS.
- A. M. Moodycliffe, D. Nghiem, G. Clydesdale and S. E. Ullrich, Immune suppression and skin cancer development: regulation by NKT cells, Nat. Immunol., 2000, 1, 521–525 CrossRef CAS.
- A. Schwarz, S. Beissert, K. Grosse-Heitmeyer, M. Gunzer, J. A. Bluestone, S. Grabbe and T. Schwarz, Evidence for functional relevance of CTLA-4 in ultraviolet-radiation-induced tolerance, J. Immunol., 2000, 165, 1824–1831 Search PubMed.
- J. F. Rooney, Y. Bryson, M. L. Mannix, M. Dillon, C. R. Wohlenberg, S. Banks, C. J. Wallington, A. L. Notkins and S. E. Straus, Prevention of ultraviolet-light-induced herpes labialis by sunscreen, Lancet, 1991, 338, 1419–1422 CrossRef CAS.
- M. Gallerani and R. Manfredini, Seasonal variation in herpes zoster infection, Brit. J. Dermatol., 2000, 142, 588–589 CrossRef CAS.
- M. Zak-Prelich, J. L. Borkowski, F. Alexander and M. Norval, The role of solar ultraviolet irradiation in zoster, Epidemiol. Inf., 2002, 129, 1–5 Search PubMed.
- J. Breuer-McHam, E. Simpson, I. Dougherty, M. Bonkobara, K. Ariizumi, D. E. Lewis, D. B. Dawson, M. Duvic and P. D. Cruz, Jr., Activation of HIV in human skin by ultraviolet B radiation and its inhibition by NFkappaB blocking agents, Photochem. Photobiol., 2001, 74, 805–810 Search PubMed.
- F. Termorshuizen, R. B. Geskus, M. T. Roos, R. A. Coutinho and H. Van Loveren, Seasonal influences on immunological parameters in HIV-infected homosexual men: searching for the immunomodulating effects of sunlight, Int. J. Hyg. Environ. Health, 2002, 205, 379–384 Search PubMed.
- R. Akaraphanth and H. W. Lim, HIV, UV and immunosuppression, Photodermatol. Photoimmunol. Photomed., 1999, 15, 28–31 CAS.
- T. A. Swartz, P. Skalska, C. G. Gerichter and W. C. Cockburn, Routine administration of oral polio vaccine in a subtropical area. Factors possibly influencing sero-conversion rates, J. Hyg. (Lond), 1972, 70, 719–726 Search PubMed.
- M. P. Zykov and A. V. Sosunov, Vaccination activity of live influenza vaccine in different seasons of the year, J. Hyg. Epidemiol. Microbiol. Immunol., 1987, 31, 453–459 Search PubMed.
- A. Sleijffers, J. Garssen, F. R. de Gruijl, G. J. Boland, J. van Hattum, W. A. van Vloten and H. van Loveren, Influence of ultraviolet B exposure on immune responses following hepatitis B vaccination in human volunteers, J. Invest. Dermatol., 2001, 117, 1144–1150 CrossRef CAS.
- D. L. Damian, G. M. Halliday, C. A. Taylor and R. S. Barnetson, Ultraviolet radiation induced suppression of Mantoux reactions in humans, J. Invest. Dermatol., 1998, 110, 824–827 CrossRef CAS.
- A. J. McMichael and A. J. Hall, Does immunosuppressive ultraviolet radiation explain the latitude gradient for multiple sclerosis?, Epidemiology, 1997, 8, 642–645 CrossRef CAS.
- D. M. Freedman, M. Dosemeci and M. C. Alavanja, Mortality from multiple sclerosis and exposure to residential and occupational solar radiation: a case-control study based on death certificates, Occup. Environ. Med., 2000, 57, 418–421 CrossRef CAS.
- L. Nystrom, G. Dahlquist, J. Ostman, S. Wall, H. Arnqvist, G. Blohme, F. Lithner, B. Littorin, B. Schersten and L. Wibell, Risk of developing insulin-dependent diabetes mellitus (IDDM) before 35 years of age: indications of climatological determinants for age at onset, Int. J. Epidemiol, 1992, 21, 352–358 Search PubMed.
- M. Rihner and H. McGrath, Jr., Fluorescent light photosensitivity in patients with systemic lupus erythematosus, Arthritis Rheum., 1992, 35, 949–952 CAS.
- L. M. Vila, A. M. Mayor, A. H. Valentin, S. I. Rodriguez, M. L. Reyes, E. Acosta and S. Vila, Association of sunlight exposure and photoprotection measures with clinical outcome in systemic lupus erythematosus, P. R. Health Sci. J., 1999, 18, 89–94 Search PubMed.
- H. Van Loveren, A. Boonstra, M. Van Dijk, A. Fluitman, H. F. Savelkoul and J. Garssen, UV exposure alters respiratory allergic responses in mice, Photochem. Photobiol., 2000, 72, 253–259 Search PubMed.
- M. Norval, J. Garssen, H. Van Loveren and A. A. el-Ghorr, UV-induced changes in the immune response to microbial infections in human subjects and animal models, J. Epidemiol., 1999, 9, S84–92 Search PubMed.
- K. Yamamoto, R. Ito, M. Koura and T. Kamiyama, UV-B irradiation increases susceptibility of mice to malarial infection, Infect. Immun., 2000, 68, 2353–2355 CrossRef CAS.
-
J. C. van der Leun, Y. Takizawa and J. Longstreth, in Environmental Effects Panel Report eds.: J. C. van der Leun and M. Tevini, United Nations Environmental Programme, Nairobi, 1989, pp. 11–24 Search PubMed.
- L. K. Ryan, D. L. Neldon, L. R. Bishop, M. I. Gilmour, M. J. Daniels, D. M. Sailstad and M. J. Selgrade, Exposure to ultraviolet radiation enhances mortality and pathology associated with influenza virus infection in mice, Photochem. Photobiol., 2000, 72, 497–507 Search PubMed.
- J. Garssen, R. van der Molen, A. de Klerk, M. Norval and H. van Loveren, Effects of UV irradiation on skin and nonskin-associated herpes simplex virus infections in rats, Photochem. Photobiol., 2000, 72, 645–651 Search PubMed.
- E. L. Brown, S. E. Ullrich, M. Pride and M. L. Kripke, The effect of UV irradiation on infection of mice with Borrelia burgdorferi, Photochem. Photobiol., 2001, 73, 537–544 Search PubMed.
- E. C. De Fabo, F. P. Noonan and J. E. Frederick, Biologically effective doses of sunlight for immune suppression at various latitudes and their relationship to changes in stratospheric ozone, Photochem. Photobiol., 1990, 52, 811–817 Search PubMed.
- J. Garssen, W. Goettsch, F. de Gruijl, W. Slob and H. van Loveren, Risk assessment of UVB effects on resistance to infectious diseases, Photochem. Photobiol., 1996, 64, 269–274 Search PubMed.
- D. A. Kelly, S. L. Walker, J. M. McGregor and A. R. Young, A single exposure of solar simulated radiation suppresses contact hypersensitivity responses both locally and systemically in humans: quantitative studies with high-frequency ultrasound, J. Photochem. Photobiol. B, 1998, 44, 130–142 Search PubMed.
- T. Yoshikawa, V. Rae, W. Bruins-Slot, J. W. Van den Berg, J. R. Taylor and J. W. Streilein, Susceptibility to effects of UVB radiation on induction of contact hypersensitivity as a risk factor for skin cancer in humans, J. Invest. Dermatol., 1990, 95, 530–536 CrossRef CAS.
- D. A. Kelly, A. R. Young, J. M. McGregor, P. T. Seed, C. S. Potten and S. L. Walker, Sensitivity to sunburn is associated with susceptibility to ultraviolet radiation-induced suppression of cutaneous cell-mediated immunity, J. Exp. Med., 2000, 191, 561–566 CrossRef CAS.
- P. H. Hart, M. A. Grimbaldeston, G. J. Swift, A. Jaksic, F. P. Noonan and J. J. Finlay-Jones, Dermal mast cells determine susceptibility to ultraviolet B-induced systemic suppression of contact hypersensitivity responses in mice, J. Exp. Med., 1998, 187, 2045–2053 CrossRef CAS.
- M. A. Grimbaldeston, L. Skov, O. Baadsgaard, B. G. Skov, G. Marshman, J. J. Finlay-Jones and P. H. Hart, Communications: high dermal mast cell prevalence is a predisposing factor for basal cell carcinoma in humans, J. Invest. Dermatol., 2000, 115, 317–320 CrossRef CAS.
- P. H. Hart, M. A. Grimbaldeston, G. J. Swift, E. K. Hosszu and J. J. Finlay-Jones, A critical role for dermal mast cells in cis-urocanic acid-induced systemic suppression of contact hypersensitivity responses in mice, Photochem. Photobiol., 1999, 70, 807–812 Search PubMed.
- F. De Fine Olivarius, J. Lock-Andersen, F. G. Larsen, H. C. Wulf, J. Crosby and M. Norval, Urocanic acid isomers in patients with basal cell carcinoma and cutaneous malignant melanoma, Brit. J. Dermatol., 1998, 138, 986–992 CrossRef CAS.
- E. Snellman, C. T. Jansen, T. Rantanen and P. Pasanen, Epidermal urocanic acid concentration and photoisomerization reactivity in patients with cutaneous malignant melanoma or basal cell carcinoma, Acta Derm. Venereol., 1999, 79, 200–203 CrossRef CAS.
- S. Beissert, D. Ruhlemann, T. Mohammad, S. Grabbe, A. El-Ghorr, M. Norval, H. Morrison, R. D. Granstein and T. Schwarz, IL-12 prevents the inhibitory effects of cis-urocanic acid on tumor antigen presentation by Langerhans cells: implications for photocarcinogenesis, J. Immunol., 2001, 167, 6232–6238 Search PubMed.
- F. Levi, L. Randimbison, C. La Vecchia, G. Erler and V. C. Te, Incidence of invasive cancers following squamous cell skin cancer, Am. J. Epidemiol., 1997, 146, 734–739 CAS.
- F. Levi, L. Randimbison, V. C. Te and C. La Vecchia, Non-Hodgkin's lymphomas, chronic lymphocytic leukaemias and skin cancers, Brit. J. Cancer., 1996, 74, 1847–1850 Search PubMed.
- M. Frisch, H. Hjalgrim, J. H. Olsen and M. Melbye, Risk for subsequent cancer after diagnosis of basal-cell carcinoma. A population-based, epidemiologic study, Ann. Intern. Med., 1996, 125, 815–821 Search PubMed.
- L. H. Goldberg, Basal-cell carcinoma as predictor for other cancers, Lancet, 1997, 349, 664–665 CAS.
- G. Bentham, Association between incidence of non-Hodgkin's lymphoma and solar ultraviolet radiation in England and Wales, Brit. Med. J., 1996, 312, 1128–1131 Search PubMed.
- A. J. McMichael and G. G. Giles, Have increases in solar ultraviolet exposure contributed to the rise in incidence of non-Hodgkin's lymphoma?, Brit. J. Cancer., 1996, 73, 945–950 Search PubMed.
- I. H. Langford, G. Bentham and A. L. McDonald, Mortality from non-Hodgkin lymphoma and UV exposure in the European Community, Health Place, 1998, 4, 355–364 CrossRef CAS.
- J. Adami, G. Gridley, O. Nyren, M. Dosemeci, M. Linet, B. Glimelius, A. Ekbom and S. H. Zahm, Sunlight and non-Hodgkin's lymphoma: a population-based cohort study in Sweden, Int. J. Cancer, 1999, 80, 641–645 CrossRef CAS.
- P. Hartge, S. S. Devesa, D. Grauman, T. R. Fears and J. F. Fraumeni, Jr., Non-Hodgkin's lymphoma and sunlight, J. Natl. Cancer. Inst., 1996, 88, 298–300 CAS.
- L. J. Schouten, H. Meijer, J. A. Huveneers and L. A. Kiemeney, Urban-rural differences in cancer incidence in The Netherlands 1989–1991, Int. J. Epidemiol., 1996, 25, 729–736 Search PubMed.
- N. Hakansson, B. Floderus, P. Gustavsson, M. Feychting and N. Hallin, Occupational sunlight exposure and cancer incidence among Swedish construction workers, Epidemiology, 2001, 12, 552–557 CrossRef CAS.
- M. Nordstrom, L. Hardell, A. Magnusson, H. Hagberg and A. Rask-Andersen, Occupation and occupational exposure to UV light as risk factors for hairy cell leukaemia evaluated in a case-control study, Eur. J. Cancer. Prev., 1997, 6, 467–472 CAS.
- G. Bentham, A. M. Wolfreys, Y. Liu, G. Cortopassi, M. H. Green, C. F. Arlett and J. Cole, Frequencies of hprt(−) mutations and bcl-2 translocations in circulating human lymphocytes are correlated with United Kingdom sunlight records, Mutagenesis, 1999, 14, 527–532 Search PubMed.
- W. Jiang, H. N. Ananthaswamy, H. K. Muller, A. Ouhtit, S. Bolshakov, S. E. Ullrich, A. K. El-Naggar and M. L. Kripke, UV irradiation augments lymphoid malignancies in mice with one functional copy of wild-type p53, Proc. Natl. Acad. Sci. USA, 2001, 98, 9790–9795 CrossRef CAS.
- G. P. Studzinski and D. C. Moore, Sunlight—can it prevent as well as cause cancer?, Cancer Res., 1995, 55, 4014–4022 Search PubMed.
- C. L. Hanchette and G. G. Schwartz, Geographic patterns of prostate cancer mortality. Evidence for a protective effect of ultraviolet radiation, Cancer, 1992, 70, 2861–2869 CAS.
- C. J. Luscombe, A. A. Fryer, M. E. French, S. Liu, M. F. Saxby, P. W. Jones and R. C. Strange, Exposure to ultraviolet radiation: association with susceptibility and age at presentation with prostate cancer, Lancet, 2001, 358, 641–642 CrossRef CAS.
- J. M. Norris, Can the sunshine vitamin shed light on type 1 diabetes?, Lancet, 2001, 358, 1476–1478 CrossRef CAS.
- TEAP, UNEP Report of Technology and Economic Assessment Panel, United Nations Environmental Programme Report, Nairobi.
-
M. Kojima, T. Okuno, M. Miyakoshi, K. Sasaki and N. Takahashi, in Progress in Lens and Cataract Research, Vol. 35 eds.: O. Hockwin, M. Kojima, N. Takahashi and D. H. Sliney, Karger, Basel, 2002, pp. 125–134 Search PubMed.
- D. T. Shindell, D. Rind and P. Lonergan, Increased polar stratospheric ozone losses and delayed eventual recovery owing to increased greenhouse-gas concentrations, Nature, 1998, 392, 589–592 CrossRef.
- H. Slaper, G. J. Velders, J. S. Daniel, F. R. de Gruijl and J. C. van der Leun, Estimates of ozone depletion and skin cancer incidence to examine the Vienna Convention achievements, Nature, 1996, 384, 256–258 CrossRef CAS.
- G. Kelfkens, A. Bergman, F. R. de Gruijl, J. C. Van der Leun, A. Piquet, T. van Oijen, W. W. C. Gieskens, H. van Loveren, G. J. M. Velders, P. Martens and H. Slaper, Ozone and climate change interactions; Influence on UV levels and UV-related effects, National Programme on Global Air Pollution and Climate Change Report No. Bilthoven, Netherlands.
- J. A. Bain, H. P. Rusch and B. E. Kline, The effect of temperature upon ultraviolet carcinogenesis with wavelengths 2,800–3,400 Å, Cancer Res., 1943, 3, 610–612 Search PubMed.
- R. G. Freeman and J. M. Knox, Influence of temperature on ultraviolet injury, Arch. Dermatol., 1964, 89, 858–864 CAS.
- J. C. van der Leun and F. R. de Gruijl, Climate change and skin cancer, Photochem. Photobiol. Sci., 2002, 1, 324–326 RSC.
- J. Longstreth, Anticipated public health consequences of global climate change, Environ. Health Perspect., 1991, 96, 139–144 Search PubMed.
- A. Leaf, Potential health effects of global climatic and environmental changes, N. Engl. J. Med., 1989, 321, 1577–1583 CAS.
- P. R. Epstein, Global warming and vector-borne disease, Lancet, 1998, 351, 1737; discussion 1738.
- J. Longstreth, Public health consequences of global climate change in the United States—some regions may suffer disproportionately, Environ. Health Perspect., 1999, 107(Suppl 1), 169–179 Search PubMed.
Footnote |
† This article is published as part of the United Nations Environmental Programme: Environmental effects of ozone depletion and its interactions with climate change: 2002 assessment. |
|
This journal is © The Royal Society of Chemistry and Owner Societies 2003 |