DOI:
10.1039/B209149F
(Communication)
Chem. Commun., 2003, 58-59
Novel addition reactions of titanacycle phosphonates by tuning of Ti(O-i-Pr)4/2i-PrMgCl†
Received
(in Cambridge, UK)
19th September 2002
, Accepted 13th November 2002
First published on 25th November 2002
Abstract
Di- or tri-substituted vinylphosphonates, 2–5, can be obtained in a highly regio- and stereoselective manner from 1-alkynylphosphonates, by manipulation of Ti(O-i-Pr)4/2i-PrMgCl.
Vinylphosphonates are an exceedingly important group of compounds.1–7 Owing to their importance, there are various routes to the preparation of vinylphosphonates.8–13 Recently, two groups have reported protocols based on carbocupration reactions of 1-alkynylphosphonates.14 Since the cuprate reagents are derived from organolithium or Grignard reagents, they suffer from the same functional group incompatibility. Nevertheless, metallation of alkynylphosphonates is an attractive approach to the synthesis of highly substituted vinylphosphonates. In many instances, transition metal chemistry circumvents these problems. The Ti(O-i-Pr)4/2RMgCl reagent initially discovered by Kulinkovich15 and developed into a highly useful synthesis of cyclopropanols from esters,16 has been further modified by Sato to add to alkynes and alkenes from which a variety of useful reagents and products can be obtained.17 Its preparation from relatively inexpensive starting materials makes it an attractive alternative to divalent titanocene compounds. We have been interested in the chemistry of zirconacycle phosphonates as a means of preparing vinylphosphonates.18 However, the potentially enhanced reactivity of titanacycle isopropoxides tempted our curiosity. Herein we report some interesting new reactions involving divalent titanium isopropoxides, which greatly expand the usefulness of this reagent and provide compounds not attainable with zirconacycles, thus complementing the reactions we have developed with the latter.
When the intermediate titanacycle prepared from 1-alkynylphosphonate,191, and Ti(O-i-Pr)4/2i-PrMgCl, was reacted with an additional equivalent of RMgX, followed by aqueous workup, the vinylphosphonate, 2, was isolated (Scheme 1). This intrigued us. We assumed that two equiv. of i-PrMgCl went into the formation of the titanacycle. The other Grignard then attacked and inserted into the Ti–C bond (Scheme 1). The reaction did not proceed when the Grignard reagents were replaced by n-BuLi. Since the diisopropoxytitanacycle is a stable species at low temperature, it was reasonable to expect that replacement of the third equivalent of isopropylmagensium chloride by another Grignard would give the corresponding 1,4-addition product. This indeed proved to be the case. When phenyl- or ethylmagnesium bromide were used in step two (Scheme 1), aqueous workup provided the corresponding disubstituted vinyl phosphonates. Isopropyl derivatives were not detected. Yields were good to excellent.
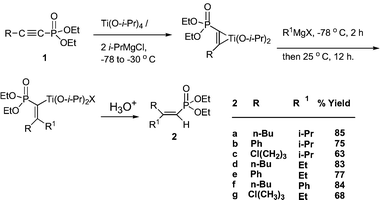 |
| Scheme 1 | |
The titanium reagent may be further modified. Reacting an alkynylphosphonate (1 mmol), Ti(O-i-Pr)4 (2 mmol), i-PrMgCl (4 mmol) to allow formation of intermediates followed by addition of another equivalent of i-PrMgCl and an acyl chloride (1 mmol) overnight with warming to room temperature provided in good yields the isopropyl derivatives 3a–c (Scheme 2).20 A possible mechanism involves the in-situ formation of a ketone that then reacts with the titanacycle to form the allylic alcohol. As was the case in Scheme 1, another Grignard may be substituted for isopropyl Grignard. For 3d, i-PrMgCl (4 mmol) was used followed by BnMgCl (1 mmol) and for 3ei-PrMgCl (4 mmol) and PhMgCl (1 mmol) was then added. The ability to select different Grignards and acyl chlorides makes it possible to synthesize a very large number of compounds. In products 2 and 3, coupling occurred on C2 (2JPH = 17.1–18.6 Hz). On the other hand the stereochemistry of compounds 2 and 3 was determined by the carbon–phosphorus coupling constant 3JPC. The large 3JPC of the allylic carbon in R1, 2, or the alcohol carbon in 3 (20.3–24.1 Hz) compared to the small 3JPC of the allylic carbon of R in 2 or the n-Bu carbon in 3 (6.3–8.0 Hz) is indicative that both R1 and the alcohol groups are trans to phosphorus, whereas R and n-Bu are cis. Although the above isomer 2 was the major product, in some cases another stereoisomer was detected, ∼10%, in which R1 is cis to phosphorus, and R is trans, 2b, 2f.
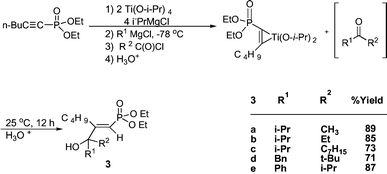 |
| Scheme 2 | |
Interesting C–C bond formations occurred when the initially formed titanacycle was allowed to react with stoichiometric amounts of PhMgBr and an acid chloride (Scheme 3). Products 4 were obtained in good yields along with 2f (∼30%). A possible mechanism involves attack by the Grignard on an intermediate cyclopropene oxide.21 The sequence is equivalent to syn addition of a Grignard followed by an aldehyde.22
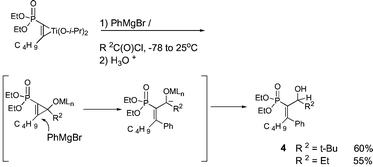 |
| Scheme 3 | |
The reaction takes a somewhat different course when a Cu(I) catalyst (10 mol%) is added, followed by an acyl chloride. Under these conditions, double insertion with C–C bond formation occurred to give 5 (Scheme 4).
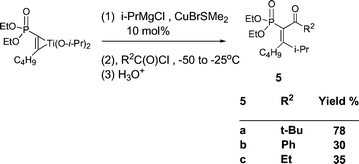 |
| Scheme 4 | |
The regioselectivity of compounds 4 and 5 was determined by 3JPC and 2JPC. Since 3JPC of the i-Pr carbon, 5, or the 3JPC of the Ph carbon, 4, (19.1–23.0 Hz) is larger than the 2JPC of the carbonyl in 5 or the alcohol carbon in 4, (12.4–15.8 Hz), coupling of the isopropyl group in compounds 5, or the phenyl group in compounds 4, of the Grignard reagents must have occurred on C2 while coupling of the acid chlorides occurred on C1. In addition, the stereochemistry of compounds 4 and 5 was also determined by 3JPC. The large 3JPC of the i-Pr carbon, 5, or Ph carbon, 4, (20.0–23.0 Hz), and the small 3JPC of the n-Bu carbon, 4 and 5 (6.3–8.3 Hz), is indicative that the i-Pr group in 5 and the Ph ring in 4 are trans to phosphorus while the n-Bu is cis. A series of labeling experiments with D2O were carried out to determine that the actual intermediates involved were titanacycles. Thus GCMS analysis of the product obtained by treatment of the intermediate titanacycle formed from diethyl 1-hexynylphosphonate gave M+ = 222, indicative of the cis-1,2-dideuteriovinylphosphonate (Scheme 5). D2O workup after addition of i-PrMgCl to the same titanacycle provided the monodeuterio product, M+ = 263.
 |
| Scheme 5 | |
We thank the Israel Science Foundation for support of this work.
Notes and references
-
(a)
J. Jin, II and J. C. H. Hua, US Patent 3925506, 1975 Search PubMed;
(b)
M. Fujimatsu and M. Miura, Jpn. Patent, 1988, 86-250155 Search PubMed; Chem. Abstr., 1989, 110, 9644.
-
(a)
A. J. Spak and A. J. Yu, US Patent 4088710, 1978 Search PubMed;
(b)
B. S. Fuller, W. D. Ellis and R. M. Rowell, US Patent 5683820, 1997 Search PubMed.
-
(a)
J. R. Shukla, US Patent 4241125, 1980 Search PubMed;
(b) C. M. Welch, E. J. Gonzalez and J. D. Guthrie, J. Org. Chem., 1961, 26, 3270 CrossRef CAS.
-
(a) M. R. Harnden, A. Parkin, M. J. Parratt and R. M. Perkin, J. Med. Chem., 1993, 36, 1343 CrossRef CAS;
(b) Y. G. Smeyers, F. J. Romero-Sanchez, A. Hernandez-Laguna, N. Fernandez-Ibanez, E. Galvez-Ruano and S. Arias-Perez, J. Pharm. Sci., 1987, 76, 753 CAS;
(c) S. Megati, S. Phadtare and J. Zemlicka, J. Org. Chem., 1992, 57, 2320 CrossRef CAS;
(d) H. B. Lazrek, A. Rochdi, H. Khaider, J. L. Barascut, J. L. Imbach, J. Balzarini, M. Witvrouw, C. Pannecouque and E. De Clerq, Tetrahedron, 1998, 54, 3807 CrossRef CAS;
(e) P. W. Smith, P. W. A. J. Chamiec, G. Chung, K. N. Cobley, K. Duncan, P. D Howes, A. R. Whittington and M. R. Wood, J. Antibiot. Tokyo, 1995, 48, 73 Search PubMed;
(f) S. A. Holstein, D. M. Cermak, D. F Wiemer, K. Lewis and R. J. Hohl, Bioorg. Med. Chem., 1998, 6, 687 CrossRef CAS.
-
L. H. Chance and J. P. Moreau, US Patent 3910886, 1975 Search PubMed.
- For a review:
(a) T. Minami and J. Motoyoshiya, Synlett, 1992, 333 CAS. Selected recent transformations
(b) aminohydroxylations: A. A. Thomas and K. B. Sharpless, J. Org. Chem., 1999, 64, 8379 Search PubMed;
(c) cycloaddition: S. Y. Lee, B. S. Lee, C. W Lee and D. Y. Oh, Synth. Commun., 1999, 29, 3621 Search PubMed;
(d) Mukaiyama–Michael reaction: L. A. Telan, C.-D. Poon and S. A. Evans, Jr., J. Org. Chem., 1996, 61, 7455 Search PubMed;
(e) iodocyclization: Y. F. Zhao, C. X. Pei, Z. Y. Wong and S. K. Xi, Phosphorus Sulfur Silicon, 1992, 66, 115 Search PubMed;
(f) aziridines: D. Y. Kim and D. Y. Rhie, Tetrahedron, 1997, 53, 13603 Search PubMed;
(g) Baylis–Hillman: Y. Nagaoka and K. Tomioka, J. Org. Chem., 1998, 63, 6428 Search PubMed;
(h) Diels–Alder: N. Defacqz, R. Touillaux and J. Marchand-Brynaert, J. Chem. Res. S, 1998, 512 Search PubMed.
- For a complete list, see the IBM patent data base at: www.delphion.com.
- Wittig-type:
(a) A. Gupta, K. Sacks, S. Khan, B. E Tropp and R. Engel, Synth. Commun., 1980, 10, 299 CrossRef CAS;
(b) E. E. Aboujaoude, S. Lietje and N. Collingnon, Tetrahedron Lett., 1985, 26, 4435 CrossRef CAS;
(c) C. R. Degenhardt, Synth. Commun., 1982, 12, 415 CAS;
(d) Y. Yamshita, M. Kojima, H. Yoshida, T. Ogata and S. Inokawa, Bull. Chem. Soc. Jpn., 1980, 53, 1625;
(e) Y.
B. Xu, M. T. Flavin and Z. Q. Xu, J. Org. Chem., 1996, 61, 7697 CrossRef CAS;
(f) Y. C. Shen and B. Z. Yang, Synth. Commun., 1993, 23, 3081 CAS;
(g) R.-Q. Liu and M. Schlosser, Synlett, 1966, 1197.
- Dehydration:
(a) M. F. Semmelhack, J. C. Tomesch, M. Czarney and S. Boettger, J. Org. Chem., 1978, 43, 1259 CrossRef CAS;
(b) C. R. Degenhardt and D. C. Burdsall, J. Org. Chem., 1986, 51, 3488 CrossRef CAS;
(c) W. Flitsch and W. Lubisch, Chem. Ber., 1984, 117, 1424 Search PubMed;
(d) T. Minami, T. Yamanouchi, S. Tokumasu and I. Hirao, Tetrahedron Lett., 1983, 24, 767 CrossRef CAS;
(e) M. T. Reetz, R. Peter and M. von Itzstein, Chem. Ber., 1987, 120, 121 Search PubMed.
- Oxidative elimination:
(a) T. Minami, H. Suganuma and T. Agawa, Chem. Lett., 1978, 285 CAS;
(b) W. A. Kleschick and C. H. Heathcock, J. Org. Chem., 1978, 43, 1256 CrossRef CAS;
(c) B. Venugopalan, A. B. Hamlet and T. Durst, Tetrahedron Lett., 1981, 22, 191 CrossRef CAS.
- Allylic rearrangements: B. Principato, M. Maffei, C. Siv, G. Buono and G. Peiffer, Tetrahedron, 1996, 52, 2087 Search PubMed.
- Peterson olefination:
(a) M. Mikolajczyk and P. Balczewski, Synthesis, 1989, 101 CrossRef CAS;
(b) H. Ahlbrecht, W. Farnung and H. Simon, Chem. Ber., 1984, 117, 2622 Search PubMed;
(c) R. Waschbusch, J. Carran and P. Savignac, Tetrehedron, 1996, 52, 14199 CrossRef CAS.
- Arbuzov: G. Axelrad, S. Laosooksathit and R. Engel, J. Org. Chem., 1981, 46, 5200 CrossRef.
-
(a) H.-J. Cristau, X. Y. Mbianda, Y. Beziat and M.-B. Gase, J. Organomet. Chem., 1997, 529, 301 CrossRef CAS;
(b) J. M. Gil and D. Y. Oh, J. Org. Chem., 1999, 64, 2950 CrossRef CAS.
-
(a) O. G. Kulinkovich, S. V. Sviridov, D. A. Vasilevskii and T. S. Pritytskaya, Zh. Org. Khim., 1989, 25,, 2244 Search PubMed;
(b) O. G. Kulinkovich, S. V. Sviridov and D. A. Vasilevskii, Synthesis, 1991, 234 CrossRef.
-
(a) O. G. Kulinkovich, A. I. Savchgenko, S. V. Sviridov and D. A. Vasilevskii, Mendeleev Commun., 1993, 192 Search PubMed;
(b) O. G. Kulinkovich and A. de Meijere, Chem. Rev., 2000, 100, 2789 CrossRef CAS.
-
(a) F. Sato, H. Urabe and S. Okamoto, Synlett, 2000, 753 CAS;
(b) F. Sato and S. Okamoto, Adv. Synth. Catal., 2000, 343, 759 CrossRef CAS.
-
(a) A. A. Quntar, A. Melman and M. Srebnik, Synlett, 2000, 61 CAS;
(b) A. A. Quntar and M. Srebnik, J. Org. Chem., 2001, 66, 6650 CrossRef CAS;
(c) A. A. Quntar and M. Srebnik, J. Org. Chem., 2002, 67, 3769 CrossRef CAS.
- For a review of the synthesis and chemistry of 1-alkynylphosphonnates: B. Iorga, F. Eymery, D. Carmichael and P. Savignac, Eur. J. Org. Chem., 2000, 3103 Search PubMed.
- While Scheme 2 is only a suggestion, we believe it is valid for
the following reasons: (1) one equiv. of Ti(O-i-Pr)4 and two equiv. of i-PrMgCl are used in the formation of the initial titanacycle. The remaining equiv. of Ti(O-i-Pr)4 and the two equiv. of i-PrMgCl are then utilized to form another divalent titanium species which inserts the acid chloride. The latter then reacts with the other equivalent of Grignard to form a ketone in-situ. Otherwise there would be no discernable difference between Schemes 2 and 3.
- L. K. Sydnes, Eur. J. Org. Chem., 2000, 3511 CrossRef CAS.
- In point of fact addition of an aldehyde gave a complex mixture of products.
|
This journal is © The Royal Society of Chemistry 2003 |