DOI:
10.1039/B109392D
(Paper)
J. Mater. Chem., 2002,
12, 47-52
Efficient electroluminescent material for light-emitting diodes from 1,4-distyrylbenzene derivatives
Received
16th October 2001
, Accepted 1st November 2001
First published on 26th November 2001
Abstract
A series of distyrylbenzene (DSB) derivatives, as the oligomeric analogue of poly-p-phenylenevinylene (PPV), were synthesized and assessed as the emitter in organic light emitting diode (OLED) fabrication. The presence of an electron-withdrawing cyano group and an electron-donating methoxy group was studied at various positions in the molecule to evaluate their influence on the photophysical properties and the electroluminescent behavior of these derivatives in OLED. Bright blue emissions were achieved with these materials, as dopant, in a device of structure ITO/NPB/CBP/TPBI ∶ DSB/TPBI/Mg ∶ Ag, where NPB, CBP, and TPBI stand for 4,4′-bis[N-(1-naphthyl)-N-phenylamino]biphenyl, 4,4′-dicarbazolyl-1,1′-biphenyl and 2,2′,2″-(benzene-1,3,5-triyl)tris-[1-phenyl-1H-benzimidazole], respectively.
There was not much difference in the absorption and emission spectra of the compounds containing n-hexyloxy and 2-ethylhexyloxy groups. However, 2-ethylhexyloxy groups produce a more saturated blue color in their EL. The compounds 1–6 with higher fluorescence quantum yield did not result in higher EL quantum efficiency in this multilayer OLED fabrication as that of 7–9 with lower fluorescence quantum yield.
Introduction
Recently, poly-p-phenylenevinylene (PPV) derivatives have drawn attention due to their applications in electroluminescent (EL) devices.1,2 A challenging task in this field is to optimize the photophysical properties of the PPVs for device fabrication. Introduction of various alkyl or alkoxy groups on the phenylene ring was achieved to improve the solubility and thus processability of the polymer during fabrication. Attachment of various substituents to improve photoluminescent quantum yield or tune the emission color is another important endeavour.3–9
The oligomers of the repeating unit of a polymer are often useful in understanding the properties of a polymer system. This is due to the close analogy of their physical properties, as well as the ease of characterization of the oligomeric system. Several papers have reported the synthesis of a series of short chain oligomeric analogues of PPVs.3–15 These can be used as a model system for understanding the relationship between luminescence and molecular structure and also may be used in fabricating multilayer-type EL devices. Such reports are limited, to our knowledge.8a In this paper, we report the synthesis and photophysical properties of a series of PPV oligomers, distyrylbenzenes (DSBs), possessing an electron-withdrawing cyano group or electron-donating methoxy group at various positions. The optical properties were compared. Devices were
fabricated based on these materials in an effort to explore the potential in EL application.
Experimental
Materials
E,E-1,4-Bis(hexyloxy)-2,5-bis[2-(2-cyanophenyl)ethenyl]benzene 1.
To a stirred suspension of NaH (0.15 g, 6 mmol) in THF (5 mL) was added 1,4-bis(hexyloxy)benzene-2,5-dicarbaldehyde (1.00 g, 3 mmol) under a nitrogen atmosphere. A solution of diethyl 2-cyanobenzylphosphonate (1.52 g, 6 mmol) in THF (2 mL) was added to the mixture at 0
°C. The reaction mixture was stirred at the same temperature for 3 h. It was quenched with water (20 mL). The residue was extracted with ethyl acetate (3 × 25 mL). The combined organic phase was washed with brine solution (2 × 15 mL), dried (MgSO4), filtered and evaporated under reduced pressure. The product was
recrystallized three times from MeOH to afford 1
(1.29 g, 81% yield) as a yellow solid: mp 104–105
°C; 1H-NMR (CDCl3, TMS) 0.91 (t, J = 7 Hz, 6 H), 1.33–1.70 (m, 12 H), 1.85–1.92 (m, 4 H), 4.09 (t, J = 6.4 Hz, 4 H), 7.18 (s, 2 H), 7.32 (t, J = 7.6 Hz, 2 H), 7.48–7.69 (m, 8 H), 7.82 (d, J = 8.0 Hz, 2 H) ppm; 13C-NMR (CDCl3, TMS) 13.99, 22.60, 25.92, 29.34, 31.57, 69.58, 110.92, 118.09, 124.52, 125.21, 126.80, 127.35, 128.13, 132.71, 133.11, 141.19, 151.51 ppm; IR (KBr) 2916, 2210, 1508, 1467, 1420, 1384, 1202, 958, 840, 749 cm−1; MS m/z 532 (M+),
434, 365, 307. HRMS calcd for C36H40N2O2 532.3090, found 532.3092; Anal. Calcd for C36H40N2O2: C, 81.17; H, 7.57; N, 5.26. Found: C, 81.32; H, 7.66; N, 5.39%.
E,E-1,4-Bis(hexyloxy)-2,5-bis[2-(3-cyanophenyl)ethenyl]benzene 2.
Following the procedure described above for the synthesis of 1, compound 2 was prepared from NaH (0.15 g, 6 mmol), 1,4-bis(hexyloxy)benzene-2,5-dicarbaldehyde (1.00 g, 3 mmol) and diethyl 3-cyanobenzylphosphonate (1.52 g, 6 mmol) in THF (6 mL) as a yellow solid (1.09 g, 68 % yield): mp 162–163
°C; 1H-NMR (CDCl3, TMS) 0.93 (t, J = 7 Hz, 6 H), 1.38–1.57 (m, 12 H), 1.85–1.94 (m, 4 H), 4.07 (t, J = 6.4 Hz, 4 H), 7.10 (s, 2 H), 7.11 (d, J = 16.4 Hz,
2 H, α-vinylic protons), 7.45–7.53 (m, 6 H), 7.73 (d, J = 7.7 Hz, 2 H), 7.78 (s, 2 H) ppm; 13C-NMR (CDCl3, TMS) 14.00, 22.62, 25.91, 29.37, 31.57, 69.50, 110.77, 112.92, 118.83, 126.04, 126.57, 126.76, 129.43, 129.90, 130.46, 130.54, 139.14, 151.25 ppm; IR (KBr) 2922, 2225, 1592, 1501, 1415, 1207, 1035, 958, 786 cm−1; MS m/z 532 (M+), 434, 307. HRMS calcd for C36H40N2O2 532.3090, found 532.3091; Anal. Calcd for C36H40N2O2: C, 81.17; H, 7.57; N, 5.26. Found: C, 81.35; H, 7.70; N, 5.32%.
E,E-1,4-Bis(hexyloxy)-2,5-bis[2-(4-cyanophenyl)ethenyl]benzene 3.
Following the procedure described above for the synthesis of 1, compound 3 was prepared from NaH (0.15 g, 6 mmol), 1,4-bis(hexyloxy)benzene-2,5-dicarbaldehyde (1.00 g, 3 mmol) and diethyl 4-cyanobenzylphosphonate (1.52 g, 6 mmol) in THF (6 mL) as a greenish yellow solid (1.15 g, 72% yield): mp 170–171
°C; 1H-NMR (CDCl3, TMS) 0.92 (t, J = 7 Hz, 6 H), 1.37–1.57 (m, 12 H), 1.84–1.93 (m, 4 H), 4.07 (t, J = 6.4 Hz, 4 H), 7.11 (s, 2 H), 7.14 (d, J = 16.5 Hz,
2 H, α-vinylic protons), 7.55–7.65 (m, 10 H) ppm; 13C-NMR (CDCl3, TMS) 14.00, 22.62, 25.91, 29.36, 31.57, 69.49, 110.40, 110.77, 119.07, 126.75, 126.84, 127.07, 127.34, 132.46, 142.35, 151.38 ppm; IR (KBr) 2940, 2222, 1598, 1491, 1424, 1341, 1209, 1031, 979, 867, 823 cm−1; MS m/z 532 (M+), 307, 289. HRMS calcd for C36H40N2O2 532.3090, found 532.3091; Anal. Calcd for C36H40N2O2: C, 81.17; H, 7.57; N, 5.26. Found: C, 81.36; H, 7.69; N, 5.38%.
E,E-1,4-Bis(2-ethylhexyloxy)-2,5-bis[2-(2-cyanophenyl)ethenyl]benzene 4.
Following the procedure described above for the synthesis of 1, compound 4 was prepared from NaH (0.15 g, 6 mmol), 1,4-bis(2-ethylhexyloxy)benzene-2,5-dicarbaldehyde (1.17 g, 3 mmol) and diethyl 2-cyanobenzylphosphonate (1.52 g, 6 mmol) in THF (6 mL). The crude product was recrystallized three times from MeOH to provide 4
(1.47 g, 83% yield) as a shining yellow solid: mp 88–89
°C; 1H-NMR (CDCl3, TMS) 0.90 (t, J = 7 Hz, 6 H), 0.99 (t, J = 7 Hz,
6 H), 1.33–1.65 (m, 16 H), 1.74–1.90 (m, 2H), 3.99 (d, J = 5.4 Hz, 4 H), 7.19–7.82 (m, 14 H) ppm; 13C-NMR (CDCl3, TMS) 11.30, 14.06, 23.07, 24.21, 29.21, 30.91, 39.65, 71.81, 110.35, 111.13, 118.11, 124.22, 125.20, 126.87, 127.34, 128.04, 132.75, 133.15, 141.22, 151.57 ppm; IR (CHCl3) 2927, 2338, 2223, 1626, 1449, 1466, 1421, 1203 cm−1; MS m/z 588 (M+), 476, 364. HRMS calcd for C40H48N2O2 588.3716, found 588.3718; Anal. Calcd for C40H48N2O2: C, 81.59; H, 8.22; N, 4.76. Found: C, 81.76; H, 8.42; N, 4.88%.
E,E-1,4-Bis(2-ethylhexyloxy)-2,5-bis[2-(3-cyanophenyl)ethenyl]benzene 5.
Following the procedure described above for the synthesis of 1, compound 5 was prepared from NaH (0.15 g, 6 mmol), 1,4-bis(2-ethylhexyloxy)benzene-2,5-dicarbaldehyde (1.17 g, 3 mmol) and diethyl 3-cyanobenzylphosphonate (1.52 g, 6 mmol) in THF (6 mL). The crude product was recrystallized three times from MeOH to provide 5 as a greenish yellow solid (1.13 g, 64% yield): mp 187–188
°C; 1H-NMR (CDCl3, TMS) 0.90 (t, J = 7 Hz, 6 H), 0.99 (t, J = 7 Hz,
6 H), 1.20–1.65 (m, 16 H), 1.74–1.90 (m, 2 H), 3.97 (d, J = 5.5 Hz, 4 H), 7.01 (s, 2 H), 7.13 (d, J = 16.5 Hz, 2 H, α-vinylic protons), 7.46 (t, J = 7.7 Hz, 2 H), 7.51 (d, J = 16.5 Hz, 2 H, β-vinylic protons), 7.52 (d, J = 7.7 Hz, 2 H), 7.73 (d, J = 7.9 Hz, 2 H), 7.76 (s, 2 H) ppm; 13C-NMR (CDCl3, TMS) 11.26, 14.06, 23.04, 24.19, 29.17, 30.88, 39.62, 71.70, 110.48, 112.86, 118.79, 125.96, 126.43, 126.67, 129.41, 129.83, 130.29, 130.48, 139.12, 151.30 ppm; IR (CHCl3) 2223, 1594, 1498, 1421, 1337, 1196, 1042 cm−1; MS m/z 588 (M+),
364, 219, 131. HRMS calcd for C40H48N2O2 588.3716, found 588.3717; Anal. Calcd for C40H48N2O2: C, 81.59; H, 8.22; N, 4.76. Found: C, 81.78; H, 8.45; N, 4.86%.
E,E-1,4-Bis(2-ethylhexyloxy)-2,5-bis[2-(4-cyanophenyl)ethenyl]benzene 6.
Following the general procedure described above for the synthesis of 1, compound 6 was prepared from NaH (0.15 g, 6 mmol), 1,4-bis(2-ethylhexyloxy)benzene-2,5-dicarbaldehyde (1.17 g, 3 mmol) and diethyl 4-cyanobenzylphosphonate (1.52 g, 6 mmol) in THF (5 mL). The crude compound was recrystallized three times from MeOH to provide 6 as a golden yellow solid (1.55 g, 88% yield): mp 158–159
°C; 1H-NMR (CDCl3, TMS) 0.90 (t, J = 7 Hz, 6 H), 0.99 (t, J = 7 Hz,
6 H), 1.20–1.65 (m, 16 H), 1.74–1.90 (m, 2 H), 3.97 (d, J = 5.4 Hz, 4 H), 7.12 ( s, 2 H), 7.16 (d, J = 16.5 Hz, 2 H, α-vinylic protons), 7.58 ( d, J = 16.5 Hz, 2 H, β-vinylic protons), 7.59 (d, J = 8.4 Hz, 4 H), 7.64 (d, J = 8.4 Hz, 4 H) ppm; 13C-NMR (CDCl3, TMS) 11.30, 14.09, 23.06, 24.22, 29.21, 30.92, 39.66, 71.66, 110.32, 110.43, 119.10, 126.63, 126.75, 127.01, 127.26, 132.48, 142.36, 151.46 ppm; IR (CHCl3) 2922, 2210, 1607, 1459, 1421, 1344, 1203, 1170 cm−1; MS m/z 588 (M+), 476, 364. HRMS calcd for C40H48N2O2 588.3716, found
588.3718; Anal. Calcd for C40H48N2O2: C, 81.59; H, 8.22; N, 4.76. Found: C, 81.80; H, 8.47; N, 4.92%.
1,4-Bis[(Z)-2-cyano-2-(2-methoxyphenyl)ethenyl]-2,5-bis(hexyloxy)benzene 7.
To a mixture of 1,4-bis(hexyloxy)benzene-2,5-dicarbaldehyde (0.84 g, 2.5 mmol) and KOH (0.28 g, 5 mmol) in 20 mL of EtOH was added (2-methoxyphenyl)acetonitrile (0.74 g, 5 mmol) under nitrogen atmosphere. The reaction mixture was stirred at room temperature. The reaction was monitored by TLC analysis. After the disappearance of the starting material, the reaction mixture was filtered and the crude product was washed with EtOH and recrystallized three times from ethyl acetate–dichloromethane (1 ∶ 2 ratio) to give pure product 7 as a greenish yellow solid (1.07 g, 72% yield): mp 106–107
°C; 1H-NMR (CDCl3,
TMS) 0.86 (t, J = 7 Hz, 6 H), 1.33–1.55 (m, 12 H), 1.77–1.85 (m, 4 H), 3.94 (s, 6 H), 4.11 (t, J = 6.4 Hz, 4 H), 6.97–7.06 (m, 4 H), 7.35–7.48 (m, 4 H), 7.92 (s, 2 H), 7.96 (s, 2 H) ppm; 13C-NMR (CDCl3, TMS) 13.93, 22.54, 25.78, 29.19, 31.51, 55.69, 69.33, 108.91, 111.43, 111.54, 118.49, 121.01, 124.83, 125.82, 130.01, 130.37, 140.12, 151.31, 157.06 ppm; IR (CHCl3) 2933, 2213, 1588, 1493, 1425, 1251, 1211, 1026 cm−1; MS m/z 593 (M+ + 1), 510, 425, 393. HRMS calcd for C38H44N2O4 592.3301, found 592.3303; Anal. Calcd for C38H44N2O4: C, 77.00; H, 7.48; N, 4.73. Found:
C, 77.23; H, 7.61; N, 4.84%.
1,4-Bis[(Z)-2-cyano-2-(3-methoxyphenyl)ethenyl]-2,5-bis(hexyloxy)benzene 8.
Following the general procedure as described above for the synthesis of 7, compound 8 was prepared from 1,4-bis(hexyloxy)benzene-2,5-dicarbaldehyde (0.84 g, 2.5 mmol) and (3-methoxyphenyl)acetonitrile (0.74 g, 5 mmol) using KOH (0.28 g, 5 mmol) as base in 20 mL of EtOH at 0
°C. The crude product was recrystallized three times from ethyl acetate–dichloromethane (1 ∶ 2 ratio) to give pure product 8 as an orange solid (0.77 g, 52% yield): mp 133–134
°C; 1H-NMR (CDCl3, TMS) 0.89 (t, J = 7 Hz,
6 H), 1.33–1.58 (m, 12 H), 1.80–1.87 (m, 4 H), 3.87 (s, 6 H), 4.12 (t, J = 6.3 Hz, 4 H), 6.94 (d, J = 7.8 Hz, 2 H), 7.21–7.40 (m, 6 H), 7.88 (s, 2 H), 8.03 (s, 2 H) ppm; 13C-NMR (CDCl3, TMS) 13.94, 22.57, 25.85, 29.14, 31.52, 55.34, 69.41, 111.35, 111.65, 114.84, 118.31, 118.52, 125.73, 130.06 (2 C), 136.07, 136.27, 151.53, 160.09 ppm; IR (CHCl3) 2926, 2206, 1602, 1493, 1425, 1269, 1201; MS m/z 592 (M+), 509, 425, 307. HRMS calcd for C38H44N2O4 592.3301, found 592.3303; Anal. Calcd for C38H44N2O4: C, 77.00; H, 7.48; N, 4.73. Found: C, 77.19; H, 7.59; N, 4.89%.
1,4-Bis[(Z)-2-cyano-2-(4-methoxyphenyl)ethenyl]-2,5-dihexyloxybenzene 9.
Following the general procedure as described above for the synthesis of 7, compound 9 was prepared from 1,4-bis(hexyloxy)benzene-2,5-dicarbaldehyde (0.84 g, 2.5 mmol) and (4-methoxyphenyl)acetonitrile (0.74 g, 5 mmol) using KOH as base at 0
°C. The crude product was recrystallized three times from ethyl acetate–dichloromethane (1 ∶ 2 ratio) to give pure product 9 as an orange solid (1.13 g, 76% yield): mp 152–153
°C; 1H-NMR (CDCl3, TMS) 0.89 (t, J = 7 Hz, 6 H), 1.35–1.62 (m,
12 H), 1.82–1.90 (m, 4 H), 3.88 (s, 6 H), 4.13 (t, J = 6.3 Hz, 4 H), 6.99 (d, J = 8.7 Hz, 4 H), 7.65 (d, J = 8.7 Hz, 4 H), 7.88 (s, 2 H), 7.93 (s, 2 H) ppm; 13C-NMR (CDCl3, TMS) 13.93, 22.55, 25.82, 29.14, 31.50, 55.39, 69.39, 111.09, 111.18, 114.42 (2 C), 118.49, 125.66, 127.27 (2 C), 127.36, 133.94, 151.34, 160.43 ppm; IR (CHCl3) 2926, 2207, 1602, 1507, 1297, 1249, 1181, 1024 cm−1; MS m/z 592 (M+), 530. HRMS calcd for C38H44N2O4 592.3301, found 592.3303; Anal. Calcd for C38H44N2O4: C, 77.00; H, 7.48; N, 4.73. Found: C, 77.21; H, 7.68; N, 4.94%.
The charge transporting materials NPB, CBP and TPBI were prepared according to literature procedures16 and purified by train-sublimation twice.
Instrumentation
Absorption and emission spectra were taken with a Hewlett Packard 8453 absorption spectrophotometer and Hitachi F-4500 fluorescence spectrophotometers, respectively. Cyclic voltammetric experiments were carried out using a BAS 100B electrochemical analyzer. A conventional three-electrode cell system was used, with glassy carbon, platinum wire and Ag/AgCl as the working, counter, and reference electrodes respectively. Degassed dichloromethane solution with 0.1 M tetra-n-butylammonium hexafluorophosphate was used as the electrolyte medium.
Device fabrication
Indium–tin-oxide (ITO)-coated glass with a sheet resistance of <50 Ω
□−1 was used as substrate. The substrate was pre-patterned by photolithography to give an effective device size of 3.14 mm2. Pre-treatment of ITO includes a routine chemical cleaning using detergent and alcohol in sequence, followed by oxygen plasma cleaning. The thermal evaporation of organic materials was carried out using ULVAC Cryogenics at a chamber pressure of 10−6 Torr. The devices with a configuration of ITO/NPB/CBP/TPBI ∶ DSBs/TPBI/Mg ∶ Ag were fabricated using 20, 20, 42 and 8 nm thickness of NPB, CBP, TPBI ∶ DSB (where the DSB was co-evaporated with TPBI at a concentration of 2% by weight) and TPBI respectively. The cathode Mg0.9Ag0.1 alloy was deposited (50 nm) by co-evaporation
and followed by a thick silver capping layer. Current voltage and light intensity measurements were made using a Keithley 2400 Source meter and a Newport 1835C Optical meter equipped with a Newport 818-ST silicon photodiode, respectively. The measurements were made at room temperature and ambient conditions. The electroluminescence was measured using a fluorescence spectrophotometer by blocking the incident light. Where necessary, a parallel deposition of the relevant organic materials was carried out on glass to measure solid PL spectra.
Results and discussion
The synthesis of the PPV oligomers used in this study is outlined in Scheme 1.
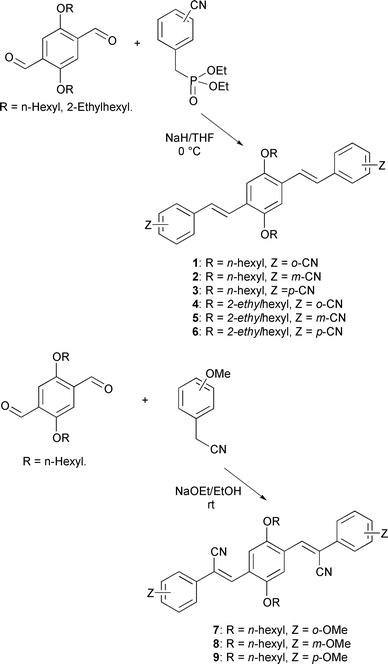 |
| Scheme 1
Synthesis of the cyano- or methoxy-substituted distyrylbenzene.
| |
The Horner–Emmons reaction of 1,4-dialkoxybenzene-2,5-dicarbaldehyde and cyano-substituted benzylphosphonate ester with sodium hydride led to cyano-substituted distyrylbenzene in 64–88% yields. Similarly, the Horner–Emmons reaction of 1,4-dialkoxybenzene-2,5-dicarbaldehyde and methoxy-substituted benzyl cyanide with sodium ethoxide as base gave methoxy-substituted distyrylbenzene in 52–76% yields.
Fig. 1 shows the UV absorption spectra of the three series 1–3, 4–6 and 7–9. The shapes and the absorption intensities are rather similar for these compounds. Two absorption bands were observed for each compound. The extinction coefficients, all of the order of 104, are always higher for the longer wavelength absorption. A comparison of the peak positions of the cyano-substituted derivatives 1–6 showed that for the n-hexyloxy or 2-ethylhexyloxy group, common groups introduced to moderate the solubility of a polymer chain, results exhibited little difference in the spectral maximum, which is expected as the two groups exert similar electronic effects on the molecule. On the other
hand, the cyano group exhibits different electronic effects in different positions. Thus ortho- and para-cyano substituents resulted in a red shift relative to the meta-cyano substituent in the DSB moiety. The meta-substituted DSB is in turn red-shifted relative to that without a cyano group. This may be due to the stabilization effect of para- and ortho-cyano groups to the π-electrons delocalized in the π system, while meta-cyano groups do not have this kind of stabilization effect. In contrast, within the series of methoxy-substituted derivatives 7–9, the ortho-methoxy-substituted compound 7 has an apparent blue shift effect relative to the meta- and para-substituted ones in the π → π* transition.17 The blue shift for 7 may come from the steric effect of the ortho-methoxy group with the β-cyano group, causing a torsion in the chains and reducing the degree of conjugation. The para-methoxy-substituted 9 is found to be red-shifted relative to the meta-substituted 8, presumably for the same aforementioned reason (delocalization stabilization at the para-position). The fluorescence spectra, which gave only one emission irrespective of the excitation wavelength, exhibited a similar trend to that in the UV spectra, that is, red-shifted for ortho- and para-substituted derivatives relative to meta-compounds, except for the ortho-methoxy-substituted 7. The quantum yields, determined with respect to Coumarin 1 (7-dimethylamino-4-methylcoumarin), are of similar order within
each series, with the exception of 7, which has a much reduced quantum yield. Cyclic voltammetry was used to determine the oxidation potentials of these derivatives. A reversible redox wave was observed for each compound. The series 7–9 have higher oxidation potentials, even with two electron-donating methoxy groups present. The two cyano groups outweigh the methoxy groups in influencing the oxidation potential. The HOMO energy levels were calculated from oxidation potentials according to literature reports.18,16a The LUMO levels were calculated using the absorption edge for estimation of band gaps. The HOMO/LUMO energy, as well as UV absorption λmax, extinction coefficients, fluorescent emission λmax, fluorescence quantum yield, and the apparent color of these PPV oligomers are summarized
in Table 1.
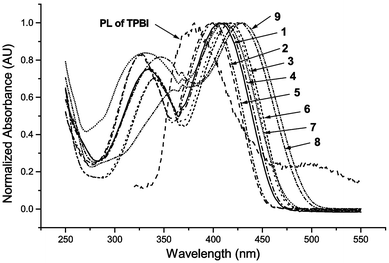 |
| Fig. 1
Normalized UV spectra of DSB derivatives and emission of TPBI.
| |
Table 1
The absorption λmax, extinction coefficiency, emission λmax, excitation λmax, fluorescence quantum yield, and the appearance color of PPV oligomers
Cpd |
UV λmax/nm |
ε × 10−4/dm3 mol−1 cm−1 |
Em λmax/nm |
Ex λmax/nm |
Φ
F
a
|
HOMO/eV |
LUMO/eV |
Band gap/eV |
Color |
Use Coumarin 1 (ΦF ≅ 1) to compare with in ethyl acetate.
|
1
|
334 |
2.2370 |
475 |
338 |
0.32 |
5.68 |
3.07 |
2.61 |
Yellow |
|
407 |
2.9823 |
|
418 |
|
|
|
|
|
2
|
327 |
2.5243 |
457 |
332 |
0.45 |
5.60 |
2.96 |
2.64 |
Yellow |
|
399 |
3.0545 |
|
407 |
|
|
|
|
|
3
|
340 |
2.8881 |
484 |
337 |
0.48 |
5.70 |
3.15 |
2.55 |
Greenish yellow |
|
414 |
4.1187 |
|
433 |
|
|
|
|
|
4
|
336 |
1.9637 |
473 |
337 |
0.44 |
5.72 |
3.12 |
2.60 |
Yellow |
|
411 |
2.6075 |
|
422 |
|
|
|
|
|
5
|
327 |
2.8192 |
460 |
327 |
0.44 |
5.66 |
3.03 |
2.63 |
Greenish yellow |
|
399 |
3.3860 |
|
413 |
|
|
|
|
|
6
|
341 |
2.7443 |
482 |
339 |
0.40 |
5.67 |
3.12 |
2.55 |
Yellow orange |
|
417 |
3.9046 |
|
436 |
|
|
|
|
|
7
|
341 |
1.3617 |
496 |
338 |
0.05 |
5.90 |
3.37 |
2.53 |
Greenish yellow |
|
414 |
1.6226 |
|
424 |
|
|
|
|
|
8
|
348 |
1.8407 |
512 |
352 |
0.15 |
5.94 |
3.50 |
2.44 |
Orange |
|
431 |
2.2532 |
|
448 |
|
|
|
|
|
9
|
369 |
1.9158 |
507 |
359 |
0.24 |
5.81 |
3.42 |
2.39 |
Orange |
|
431 |
2.8749 |
|
457 |
|
|
|
|
|
Attempts to fabricate a multilayer OLED with the DSB as emitting layer in a device structure ITO/NPB/DSB/TPBI/Mg ∶ Ag were not successful. However with DSB derivatives as a dopant in the device structure ITO/NPB/CBP/TPBI ∶ DSB/TPBI/Mg ∶ Ag, where the NPB, CBP, and TPBI stand for 4,4′-bis[N-(1-naphthyl)-N-phenylamino]biphenyl, 4,4′-dicarbazolyl-1,1′-biphenyl, and 2,2',2″-(benzene-1,3,5-triyl)tris-[1-phenyl-1H-benzimidazole], respectively, gave bright blue emission. The TPBI was chosen as a host material for DSB derivatives because it is a wide band gap material and emits strongly at around 376 nm, where most of the DSB derivatives have strong absorption (Fig. 1). Two layers of hole-transporting material NPB and CBP were used as it was demonstrated
previously that CBP serves to provide an intermediate HOMO level by which the holes can transport across to the TPBI layer.19 In the absence of the dopant, the ITO/NPB/CBP/TPBI/Mg ∶ Ag device gave a broad electroluminescence (EL) spectrum that has a major contribution from TPBI (∼380 nm) and a minor contribution from NPB(∼450 nm) and possible emission from CBP, which occurs at around 390 nm.16a This indicates that the recombination region is located mainly in the TPBI region, with some excitons occurring in the NPB area. In contrast, EL spectra of the same devices with 1%
(w/w) DSB doped into the TPBI are blue.
For the series of 1–3, the emission maximum depends on the position of the cyano group, with the ortho-substituted 1 giving the shortest wavelength at 446 nm and the para-substituted 3 giving the longest wavelength. This order is different from that in the PL in solution (λo,p > λm), due to different medium effect. The normalized spectra are given in Fig. 2. As a consequence of the small shift among different substitution, the Commission Internationale de L'Eclairage (CIE) coordinates are varying and the values are shown in Table 2. Among the three compounds, the ortho-derivative gave CIE coordinates (x,y) that
correspond to more nearly pure blue than the other two. Fig. 3 shows the I–V characteristics of the devices. The ortho-derivative gave a higher current density than the other two isomers. However the para-derivative gave a higher external quantum efficiency as well as the maximum brightness among the three. All devices start glowing between 4 and 5 V (Fig. 4). Nearly 7500 cd m−2 was obtained for 3 at a voltage of 15 V.
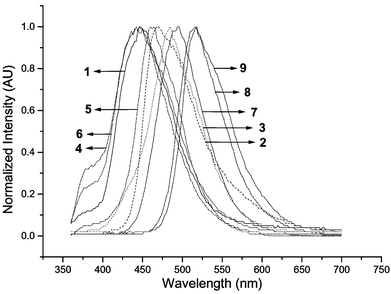 |
| Fig. 2
Normalized EL spectra of DSBs in ITO/NPB/CBP/TPBI + dopant(1%)/Mg ∶ Ag.
| |
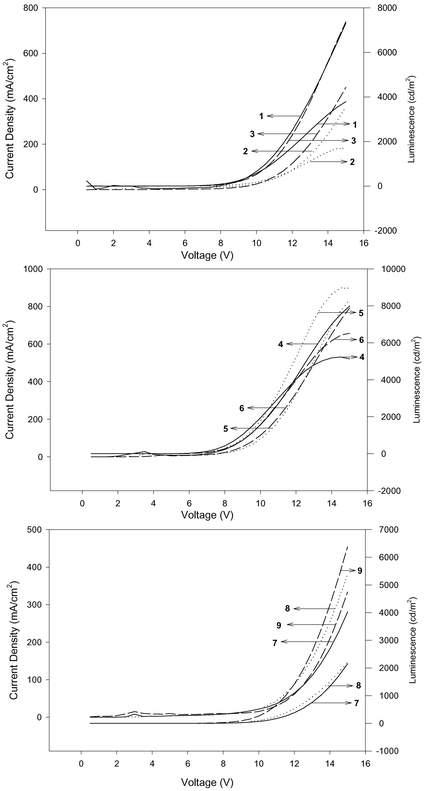 |
| Fig. 3
I–V curve and luminescence of DSB series.
| |
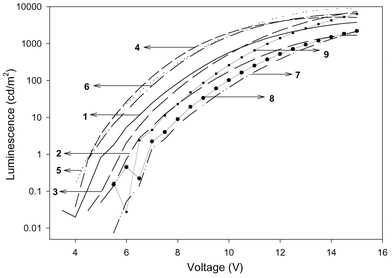 |
| Fig. 4
Turn-on voltage of DSB series.
| |
Table 2
Performance of the LEDs fabricated in this study
Cpd |
EL λmax/nm |
EL quantum efficiency (%) |
Luminance/cd m−2 at 15 V |
Turn-on voltage/V |
CIE coordinate (x,y) |
1
|
446 |
0.7 |
3786 |
4.0 |
(0.1652,0.1511) |
2
|
468 |
0.38 |
1688 |
5.0 |
(0.1957,0.3) |
3
|
484 |
1.06 |
7365 |
4.5 |
(0.1652,0.3056) |
4
|
446 |
1.14 |
4292 |
4.0 |
(0.1555,0.1044) |
5
|
464 |
1.15 |
6850 |
4.0 |
(0.1345,0.1484) |
6
|
442 |
0.93 |
3344 |
4.5 |
(0.1597,0.1319) |
7
|
494 |
0.51 |
6695 |
5.5 |
(0.1574,0.4) |
8
|
516 |
1.7 |
6499 |
6.5 |
(0.2553,0.61) |
9
|
518 |
0.78 |
9321 |
6.0 |
(0.2936,0.61) |
For the series 4–6, the EL spectra again depend on the location of the cyano substituent. At a doping concentration of 1%
(w/w), a shoulder emission of the host TPBI is present in the EL spectra (Fig. 3). The CIE coordinates showed that devices based on these three derivatives are all nearly saturated blue. The meta-derivative gave slightly better performance with a maximum luminance of ∼7000 cd m−2. The performance in respect to the EL quantum efficiency, turn-on voltage, and brightness of 5 with 2-ethylhexyloxy groups is much better than the analog 2 with n-hexyloxy groups. The turn-on voltages were between 4.0 and 4.5 V (Fig. 4).
For the series 7–9, the EL spectra shift to green or bluish-green. The ortho-derivative has shorter wavelength (494 nm) than the meta- and para-derivatives, which now give green emission (at ∼516 and 518 nm, respectively). While the current density is similar for the three compounds, the external quantum efficiency and the luminance are the highest for the para-derivative. A maximum brightness of ∼9300 cd m−2 for this device was obtained at 15 V. It is noted that although the fluorescence quantum yields of 7–9 are lower than those of 1–6, the EL quantum efficiency and brightness of 7–9 are similar or even higher than
those of 1–6. The turn-on voltages were between 5.5 and 6.5 V (Fig. 4).
In conclusion, we have demonstrated that the distyrylbenzene derivatives, which are oligomeric analogs of PPV polymer, can be used as a dopant in the multilayer (ITO/NPB/CBP/TPBI ∶ DSB/TPBI/Mg ∶ Ag) OLED fabrication. The position of the substituent on the phenyl ring of DSB can affect the absorption and emission wavelength and causes a red shift for ortho- and para-electron withdrawing groups as compared with the corresponding meta-isomer. The steric effect in 7 causes a blue shift in the corresponding spectra relative to the less sterically hindered 8 and 9. The n-hexyloxy and 2-ethylhexyloxy groups may not cause a great difference in the absorption and emission spectra. However, 2-ethylhexyloxy groups may give a more saturated blue color in their EL. The
compounds 1–6 with higher fluorescence quantum yield did not give relatively higher EL quantum efficiency in this multilayer OLED fabrication than those of 7–9 with lower fluorescence quantum yield.
Acknowledgements
We thank China Petroleum Corporation and Academia Sinica for the financial support of this work.
References
- N. C. Greenham, S. C. Moratti, D. D. C. Bradley, R. H. Friend and A. B. Holmes, Nature, 1993, 365, 628 CrossRef.
- H. E. Katz, S. F. Bent, W. L. Wilson, M. L. Schilling and S. B. Ungashe, J. Am. Chem. Soc., 1994, 116, 6631 CrossRef CAS.
-
(a) D. Oelkrug, A. Tompert, J. Gierschner, H. J. Egelhaaf, M. Hanack, M. Hohloch and E. Steinhuber, J. Phys. Chem. B, 1998, 102, 1902 CrossRef CAS;
(b) A. Heller, J. Chem. Phys., 1964, 40, 2839 CrossRef CAS.
- S. E. Döttinger, M. Hohloch, D. Hohnholz, J. L. Segura, E. Steinhuber and M. Hanack, Synth. Met., 1997, 84, 267 CrossRef.
- H. Ndayikengurukiye, S. Jacobs, W. Tachelet, J. van der Looy, A. Pollaris, H. J. Geise, M. Claeys, J. M. Kauffamnn and S. Janietz, Tetrahedron, 1997, 53, 13811 CrossRef CAS.
- J. Cornil, D. A. dos Santos, D. Beljoune and J. L. Brédas, J. Phys. Chem., 1995, 99, 5604 CrossRef CAS.
- D. Oelkrug, A. Tompert, H. J. Egelhaaf, M. Hanack, E. Steinhuber, M. Hohloch, H. Meier and U. Stalmach, Synth. Met., 1996, 83, 231 CrossRef CAS.
-
(a) K. H. Schweikart, M. Hanack, L. Lüer and D. Oelkrug, Eur. J. Org. Chem., 2001, 293 CrossRef CAS;
(b) S. Nakatsuji, K. Matsuda, Y. Uesugi, K. Nakashima, S. Akiyama, G. Katzer and W. Fabian, J. Chem. Soc., Perkin Trans. 2, 1991, 861 RSC.
- J. L. Segura and N. Martin, J. Mater. Chem., 2000, 10, 2403 RSC.
- S. E. Döttinger, M. Hohloch, J. L. Segura, E. Steinhuber, M. Hanack, A. Tompert and D. Oelkrug, Adv. Mater., 1997, 9, 233 CrossRef.
- M. Hennecke, T. Damerau and K. Mullen, Macromolecules, 1993, 26, 3411 CrossRef CAS.
- C. Hosokawa, H. Higashi, H. Nakamura and T. Kusumoto, Appl. Phys. Lett., 1995, 67, 3853 CrossRef CAS.
- N. N. Barashkov, D. J. Guerrero, H. J. Olivos and J. P. Ferraris, Synth. Met., 1995, 75, 153 CrossRef CAS.
- M. Hohloch, J. L. Segura, S. E. Döttinger, D. Hohnholz, E. Steinhuber, H. Spreitzer and M. Hanack, Synth. Met., 1997, 84, 319 CrossRef CAS.
- B. Strehmel, A. M. Sarker, J. H. Malpart, V. Strehmel, H. Seifert and D. C. Neckers, J. Am. Chem. Soc., 1999, 121, 1226 CrossRef CAS.
-
(a) B. E. Koene, D. E. Loy and M. E. Thompson, Chem. Mater., 1998, 10, 2235 CrossRef CAS;
(b)
J. Shi, C. W. Tang and C. H. Chen, U. S. Patent 5,645,948, 1997 Search PubMed;
(c) C. H. Chen and J. Shi, Coord. Chem. Rev., 1998, 171, 161 CrossRef CAS.
- The absorption λmax of the DSB derivatives increased while the polarity of the solvent was increased. For example, the longer absorption λmax of 3 in methylene chloride, benzene, and n-hexane is at 414, 413, and 407 nm, respectively..
-
(a) S. Janietz, D. D. C. Bradley, M. Grell, C. Giebeler, M. Inbasekaran and E. P. Woo, Appl. Phys. Lett., 1998, 73, 2453 CrossRef CAS;
(b) M. Thelakkat and H.-W. Schmidt, Adv. Mater., 1998, 10, 219 CrossRef CAS.
- Y. T. Tao, E. Balasubramaniam, A. Danel and P. Tomasik, Appl. Phys. Lett., 2000, 77, 933 CrossRef CAS.
|
This journal is © The Royal Society of Chemistry 2002 |
Click here to see how this site uses Cookies. View our privacy policy here.