DOI:
10.1039/B107844E
(Paper)
Analyst, 2002,
127, 163-168
Validation of the Willems badge diffusive sampler for nitrogen dioxide determinations in occupational environments
Received
31st August 2001
, Accepted 11th October 2001
First published on 6th December 2001
Abstract
The Willems badge, a diffusive sampler for nitrogen dioxide, has previously been validated for ambient air measurements. This paper describes the laboratory and field validation of the Willems badge for personal sampling under working environment conditions. The mean sampling rate in the laboratory tests was 46 ml min−1, with an RSD of 12%. No statistically significant effects on sampling rate of the sampling time, concentration of NO2 or relative humidity were found. A slightly decreased sampling rate was observed at low wind velocity. This was also confirmed during static sampling, which makes the sampler less appropriate for static sampling indoors. No back diffusion was observed. Storage of the samplers for two weeks before or after exposure did not affect the sampling rate.Our analysis is based on a modified colorimetric method, performed by FIA (flow injection analysis). This technique was compared to ion chromatography analysis. The use of
ion chromatography lowered the detection limit from 11 to 2 μg m−3 for an 8 h sample, and furthermore enabled the detection of other anions. In conclusion, the diffusive sampler was found to perform well for personal measurements in industrial environments.
Introduction
Nitrogen oxides (NOx) are a large and complex group of compounds, and key components of two of the most important pollution problems: acid rain and photochemical smog. Nitrogen dioxide (NO2) is considered the most toxic form of NOx,1 and is therefore the most important compound from the viewpoint of human exposure. NO2 is a highly reactive, poorly water-soluble gas and therefore has a greater effect on distal airways when compared, for example, to the highly water soluble gas sulfur dioxide.2 Because of its low solubility, the effects and symptoms of the gas are delayed and exposure is often not noticed early by the subject, which leads to prolonged and more damaging exposure.2 The toxicity of nitrogen dioxide probably depends on its free radical and oxidative properties, which can cause alterations in the mammalian lung. The ability
of nitrogen dioxide to form nitrous acid and nitric acid on the moist surfaces of the air spaces also contributes to its toxicity.3 Several studies have revealed an increase in respiratory illness in people exposed to nitrogen dioxide, as well as increased susceptibility to airway infections.4
The main sources of nitrogen dioxide in outdoor air are emissions from motor vehicles and burning of fossil fuels such as coal, oil and natural gas. Indoor sources of NO2 include combustion appliances such as gas stoves, pilot lights, wood stoves, fireplaces, gas- and kerosene-fueled space heaters and gasoline engines.5 Nitrogen dioxide exposure occurs in many occupations, including bus garage workers, agricultural workers (silo filler’s disease), welders, firefighters, street cleaners,6 crews on car ferries, miners, glass craftsmen, bridge and tunnel workers, and bus drivers. Industries associated with nitrogen dioxide are explosives factories, chemical factories, dye/lacquer factories, aerospace, metal cleaning/engraving/electroplating, diesel and jet engines industries.2 Exposure to high levels of NO2 may also occur in military combat situations.7
The Swedish occupational exposure limit (OEL) for NO2 for an 8 h workshift is 4 mg m−3 and 2 mg m−3 if the source is motor exhaust. The ceiling value is 10 mg m−3 for 15 min exposure.
There are several sensitive methods for measuring nitrogen dioxide, including the chemiluminescence technique in which emission from excited NO2* is detected photoelectrically. The chemiluminescence analyzer has the advantage of offering time-weighted averages of the NO2 concentration. However the analyzers are heavy and expensive, need electricity, calibration and specialist maintenance, and are therefore not suitable for assessing personal exposure.
To enable self-assessment of worker exposure in the occupational environment, it is important to have cheap, lightweight, easy-to-use devices that cause minimal inconvenience to the wearer. Diffusive (passive) samplers, which are based on molecular diffusion of the gas to a collector medium, fufill these requirements, and are therefore ideal for the purpose of monitoring personal exposure. Diffusive samplers, however, are more or less dependent on temperature, sampler geometry and wind speed, which may lead to higher variations in results compared to active sampling methods. It is therefore important to determine the effective sampling rate of the actual sampler.8 Badge-type samplers (short samplers with large cross-sectional area) are more sensitive to air velocities than tube samplers. At low wind speed, badge samplers have a lower sampling rate.8 Hofschreuder et al.9 made efforts to
reduce the impact of wind velocities on the Willems badge, and stated that with proper sampler design and optimum choice of draught shield the influence of wind speed can be reduced to less than 10%.
We have previously validated a diffusive sampler for NO2, the Willems badge, for ambient air measurements.10,12 Our conclusion was that the sampler is suitable for measuring background levels of NO2 in ambient air. The concentration of nitrogen dioxide in occupational environments may, however, be considerably higher than outdoor levels, and it is therefore important to validate the sampler under these conditions. This paper describes the laboratory and field validation of the Willems badge for personal measurements of NO2 in working environments. Our aim was to determine the sampling rate of the sampler in laboratory studies at occupational environment concentrations and to investigate the effects of relative humidity, sampling time and concentration of nitrogen dioxide on sampling rate. Another objective was to test the sampler for personal measurements in working environment conditions in field tests.
Gair et al.11 made some recommendations for the optimized use of NO2 diffusion tube samplers, one of which was the use of ion chromatography analysis in order to reduce the detection limit. In the present study the analysis of nitrite based on a modified colorimetric method, performed by FIA (flow injection analysis), was compared to ion chromatography analysis.
Experimental
Diffusive sampler
The Willems badge diffusive sampler (Fig. 1) consists of a cylinder of polystyrene. A Whatman GF-A glass fibre filter placed at the bottom of the cylinder serves as the absorption medium. A Teflon filter (Schleicher & Schuell TE 38, 5μm) on a distance ring of polystyrene (6 mm) serves as a draught shield. A polystyrene ring of 3 mm keeps the Teflon filter in place, and the badge is closed after exposure by means of a polyethylene cap. The absorption filter is impregnated with a solution of triethanolamine–acetone as a collector reagent for NO2. The diffusion length in the badge is 6 mm. After a sampling period, the absorption filter is removed from the badge, extracted and analysed for nitrite.
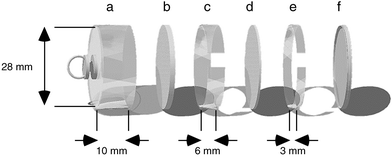 |
| Fig. 1
Expanded view of the Willems badge: a, sampler base of polystyrene; b, absorption filter; c, spacer ring; d, Teflon filter; e, fixation ring; f, cap of polyethylene.
| |
Analysis
The extraction step was the same for both methods of analysis. After sampling, the filter was transferred to a vessel containing 5 ml of 0.005 M NaOH (Eka Nobel, Göteborg, Sweden), shaken for 30 min and centrifuged at 4000 rpm for 10 min.
Spectrophotometric analysis.
Spectrophotometric analysis was used in the validation study. The analysis of nitrite based on a modified colorimetric method, the Salzman method, performed by an FIA (flow injection analysis) technique, has been described in detail previously.12
Ion chromatography analysis.
Ion chromatography analysis was used only for samples in the comparison study. After centrifugation and filtering through a 0.45μm Lida Ny (Lida Manufacturing Corp, Rochester, US) disposable filter assembly, 50μl of the the extract was injected into an ion chromatography system for detection of the nitrite concentration. The system consisted of an autosampler ‘Triathlon’ type 900 (Spark, The Netherlands), an ICSep AN1 column (CETAC, Omaha, US), a SCX membrane suppressor column (SeQuant, Umeå, Sweden) and a JD-21 conductivity detector (Costech Microanalytical Ltd.,Tallinn, Estonia). The eluent was aqueous sodium hydroxide (10 mM NaOH) with a flow rate of 1.0 ml min−1. The regenerant solution was 5 mM sulfuric acid delivered at a flow rate of approximately 5 ml min−1. Calibration was conducted using nitrite standards in the range 0.01 to 5 mg l−1. Control samples
of two nitrite concentrations (0.7, 3.5 mg l−1) and at least two blank samples were run together with samples in each run. (The day-to-day reproducibility of the method expressed as the relative standard deviation of the lowest control sample was 1.6% (n = 8).) The blank signal in each run was subtracted from the samples. The repeatability of the method was determined as the mean relative standard deviation of a control sample run in 6 replicates at two different occasions and was found to be 1.5%.
Laboratory validation
Generation of standard atmospheres of nitrogen dioxide.
Known concentrations of nitrogen dioxide were generated in an exposure chamber (70 × 48 × 900 mm) as described in detail previously.12,13 Nitrogen dioxide (23.5 ppm ± 3% NO2, 137 ppm ± 3% NO2 in nitrogen, AGA, Stockholm, Sweden) from a gas cylinder regulated by a mass flow controller (0–500 ml min−1, 0.2–10 l min−1) was diluted by clean, humidified air, controlled by a mass flow controller (0–200 l min−1), and mixed before the entrance to the exposure box. In order to vary the relative humidity, the air was passed through one to four gas-dispersion bottles containing water. By means of valves the number of bottles was modified and the relative humidity of the air could be varied between 20 and 80%. The air velocity in the box chamber was 0.3 m s−1
by keeping the airflow through the exposure box at 40 l min−1. The relative humidity and the temperature were monitored with Vaisala RH&T indicator HMI 14 (Helsinki, Finland).
Effects on sampling rate.
To experimentally determine the sampling rate at nitrogen dioxide concentrations representative of working conditions, the previously described exposure box chamber with NO2 generation system was used. The influence of sampling time, concentration of nitrogen dioxide and relative humidity on sampling rate was determined in the exposure box chamber by an eight run test with factorial design. Six samplers were exposed to nitrogen dioxide levels between 0.1 and 10 ppm, from 15 min to 8 h. The humidity was varied between 20 and 80%, and the temperature was 20 °C. Two laboratory blanks were collected in each run and the mean mass on these filters was subtracted from the amount of nitrite in the exposed samples.
Wind velocity tests.
The effects of air velocity and sampler orientation on sampling rate were studied in another exposure box (Fig. 2) connected to the above-mentioned NO2 generation system. The box (250 × 250 × 800 mm) was made of glass and equipped with a fan, which enabled wind speeds up to 2 m s−1. To make the air evenly distributed through the box chamber and to avoid turbulence, honeycomb gratings were placed on each side of the sampling site. The samplers (six at a time) were exposed in horizontal and perpendicular position respectively to 1 ppm NO2 for 60 min at air velocities of 0.1, 0.3, 0.5, 0.7, 1 and 2 m s−1.
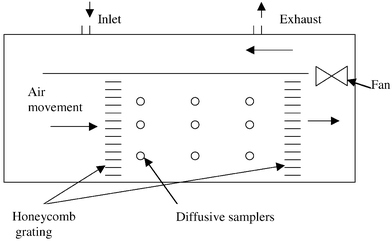 |
| Fig. 2
Exposure chamber.
| |
In both studies, measurements of NO2 were performed at the center of the exposure box chamber with a chemiluminescence instrument (ECO PHYSICS CLD 700 AL med, Dürnten, Switzerland) as a reference method. The analyser was calibrated daily with a certified gas of NO in nitrogen (AGA).
Desorption efficiency test.
Known amounts of nitrite were applied to samplers by adding a 10 μl solution of nitrite with a Hamilton syringe directly to the filter. Four levels of nitrite were used (0.7 μg, 7 μg, 14 μg, 70 μg) corresponding to exposure doses from 10 min exposure at the OEL (occupational exposure limit), to 8 h exposure at 2 × OEL, 3 parallel samples at each level. The analyte was desorbed by adding 5 ml of 0.005 M NaOH and the filters were shaken for 30 min. As a comparison 10 μl of each level of nitrite was applied directly in 5 ml of 0.005 M NaOH. Analysis was performed directly.
Storage stability test.
Two sets of samplers, with six samplers in each set, were exposed to 0.1 ppm NO2 for 8 h (1/10 × OEL). One set was analysed the next day and the other was kept in a refrigerator for two weeks before analysis. The storage test at room temperature included two levels of NO2 exposure (1/10 × OEL and 2× the ceiling value). Six samplers for each level of nitrogen dioxide were analysed within one day after exposure and six samplers were stored in room temperature (20 °C) for two weeks before analysis.
To examine the shelf life (stability before exposure) two sets of samplers were kept in room temperature for two weeks before they were exposed. These were compared with sets of freshly prepared samplers.
Back-diffusion test.
Six samplers in each set were exposed to 1.7 ppm NO2 for 40 min, (RH = 80%). One set was capped after exposure and the other was exposed to clean air for a further 7.5 h before analysis.
Comparison of two analytical methods
The two techniques for analysis of nitrite were compared by exposing 6 samplers at a time in the exposure box at four different nitrogen dioxide concentrations (0.088, 0.33, 2.0 and 10 ppm). Nine other samples were exposed in different office environments. Each sample was extracted in 6 ml of 5 mM NaOH. Half of the extract was analysed by the spectrophotometric method, and the other half by ion chromatography.
Field validation
Static and personal sampling was performed in an environmental exposure chamber where subjects were exposed to diesel exhaust. The chamber (2.5 × 2.5 × 2.4 m) is depicted schematically in Fig. 3. The walls consist of varnished steel panel with a glass window, and the floor is made of smooth galvanized steel panel. Diesel exhaust from an idling Volvo diesel engine (Volvo-BM TD45; Volvo, Gothenburg, Sweden; 4.5 L, 4 cylinders, 1991, 680 rpm) was pumped to a gasblender and diluted with fresh, warmed, filtered outdoor air regulated by a flow meter, before entering the chamber. The flow of diesel exhaust was controlled by regulating the back pressure in the exhaust pipe. By regulating the rotation speed of the fan motor the flow of fresh air was kept between 5 and 100 m3 h−1. The diesel exhaust to the exposure chamber was standardized to give a concentration of particulate matter of 100 μg m−3 and 300 μg
m−3 respectively, corresponding to steady state NO2 concentrations of about 0.5 and 1.3 ppm. Waste air from the chamber was drawn through a tube in the ceiling. Three studies of 32 simultaneous measurements with diffusive samplers and the chemiluminescence instrument were performed on 25 persons at two different NO2 concentrations. The samplers were attached to the clothes close to the breathing zone (one on each shoulder), and the measuring time was 15, 120 and 160 min, respectively. For the 120 and 160 min samples, the chemiluminescence concentrations were recorded on a datalogger (AAC-2, Intab interface teknik AB, Sweden).
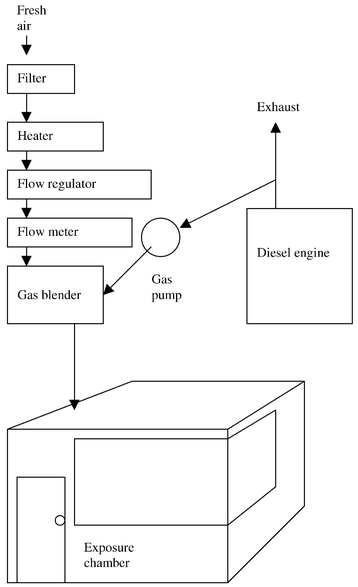 |
| Fig. 3
Schematic of the exposure chamber along with diesel exhaust generation system.
| |
For static sampling six diffusive samplers were mounted closely together. Two sets of 2.5 h measurements at a concentration of about 2.5 mg m−3 were carried out, with the on-line chemiluminescence instrument as a reference method.
For the statistics the Student’s t-test was used. Differences with a p-value <0.05 were considered significant.
Results and discussion
Laboratory validation
Sampling rate and wind velocity tests.
The sampler was evaluated according to the European standard for the validation of diffusive samplers for measurements in workplace atmospheres (CEN EN 838).14Table 1 shows the results obtained from the eight run factorial test. The mean sampling rate was 46 ml min−1 with an RSD of 12% for the 48 experiments. A statistical multiple regression analysis was performed to study the effects of relative humidity, concentration and sampling time on sampling rate.15 The parameters were scaled from −1 to +1, which makes it possible to directly compare the estimated model parameters. The numerical value of each parameter is a direct measure of the influence on the sampling rate.15 No statistically significant effects on badge sampling rate of the sampling time, concentration of NO2,
or relative humidity was found (Table 2). The results from the wind velocity tests are shown in Table 3 and Fig. 4. As shown in Table 3, perpendicular orientation results in higher sampling rates than parallel orientation for all wind speeds. In Fig. 4 the mean sampling rate (including confidence interval) for the different face velocities, is compared to the mean sampling rate determined in the factorial study. It is shown that the only wind velocity that has a mean sampling rate not included within the experimentally determined sampling rate range (mean ± standard deviation) is that of 0.1 m s−1. There is a slight reduction of the sampling rate at 0.1 m s−1 with the parallel orientation, which is the normal method of using this sampler. At 0.3 m s−1, the sampling rate
(45 ml min−1) is in accordance with the mean sampling rate obtained in the factorial study.
Table 1
Sampling rates of Willems badge (n = 6) at different sampling times, relative humidities and NO2 concentrations in laboratory tests with chemiluminescence as reference method.
Sampling time/min |
Relative humidity (%) |
Mean reference concentration/mg m−3 |
RSD(%) |
Sampling rate/ml min−1 |
16 |
80 |
2.2 |
9 |
50 |
15 |
20 |
2.2 |
8 |
48 |
485 |
20 |
0.2 |
4 |
47 |
15 |
20 |
17.7 |
8 |
43 |
468 |
80 |
0.2 |
9 |
55 |
15 |
80 |
17.8 |
7 |
46 |
479 |
20 |
3.6 |
9 |
40 |
475 |
80 |
3.7 |
9 |
42 |
|
|
Mean |
|
46
|
|
|
RSD(%) |
|
12 |
|
|
n
|
|
48 |
Table 2
Multiple regression analysis of the influence of sampling time, concentration and relative humidity. NS, not significant; RH, relative humidity
Variable |
Parameter estimate |
Standard error |
|
Sampling rate |
45.8 |
1.7 |
|
Time |
−2.3 |
1.9 |
NS |
Concentration |
−3.6 |
2.4 |
NS |
RH |
1.9 |
1.5 |
NS |
Table 3
Sampling rates of Willems badge (n = 6) at different orientations and face velocities in laboratory tests with chemiluminescence as reference method. NO2 concentration was approximately 1 ppm
Face velocity/m s−1 |
Orientation |
Sampling rate/ml min−1 |
RSD(%) |
0.1 |
Parallel |
35.5 |
11 |
0.3 |
Parallel |
44.6 |
11 |
0.5 |
Parallel |
51.5 |
12 |
0.7 |
Parallel |
54.2 |
7 |
1.0 |
Parallel |
45.7 |
16 |
2.0 |
Parallel |
51.7 |
5 |
0.1 |
Perpendicular |
54.3 |
10 |
0.3 |
Perpendicular |
56.4 |
9 |
0.5 |
Perpendicular |
51.7 |
10 |
0.7 |
Perpendicular |
57.9 |
9 |
1.0 |
Perpendicular |
58.4 |
11 |
2.0 |
Perpendicular |
67.2 |
4 |
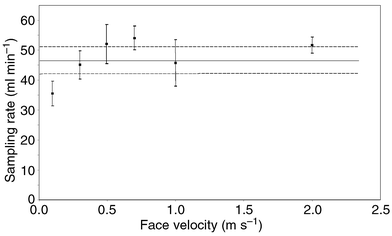 |
| Fig. 4
Effects of face velocity on sampling rate (parallel orientation) of the diffusive sampler (n = 6). The concentration of nitrogen dioxide was 1 ppm, temperature 20 °C, relative humidity 20%, and sampling time 1 h Mean sampling rate ± confidence interval for the mean at different wind velocities. ——— Mean sampling rate determined in the factorial study and - - - - - - ±
s (=4.66) for the sampling rate means (n = 8).
| |
Storage stability, desorption efficiency, and back-diffusion tests.
Exposed samplers were stable for at least 14 days at room temperature (+20 °C) and in a refrigerator (+8 °C), as shown in Table 4. The sampling rate was not affected by 14 days storage at room temperature before exposure (shelf life), as shown in Table 4. The recoveries in the desorption efficiency tests were 98–103% at the four different amount levels. The RSD for the samplers was below 6% for all amounts except for the lowest (0.7 μg), which had a RSD of 21%. The back-diffusion test showed that the sampling rate was not affected by further exposure to clean air after nitrogen dioxide exposure. There is no statistical difference between the sampling rate for samplers exposed to NO2 (51.5 ml min−1) and samplers exposed to further 7.5 h clean air (52.5 ml min−1). The
RSD was below 9% for both groups of samplers.
Table 4
Results from storage stability testsa and shelf life testsb (n = 6)
|
Storage |
Time/min |
Relative humidity(%) |
Mean reference concentration/mg m−3 |
RSD(%) |
Sampling rate/ml min−1 |
Calculated sampling rate with Willems badge analysed directly after exposure or after storage (14 days) in refrigerator or room temperature.
Calculated sampling rate with freshly prepared diffusive samplers or samplers stored 14 days at room temperature before exposure.
|
Storage stability tests |
No |
485 |
20 |
0.2 |
4 |
46.5 |
Refrigerator |
485 |
20 |
0.2 |
13 |
46.7 |
Room temp |
485 |
20 |
0.2 |
8 |
46.4 |
No |
15 |
20 |
17.7 |
8 |
43.0 |
Room temp |
15 |
20 |
17.7 |
11 |
42.6 |
Shelf life tests |
No |
468 |
80 |
0.23 |
8 |
54.5 |
Room temp |
468 |
80 |
0.23 |
9 |
54.3 |
No |
15 |
20 |
18.4 |
8 |
36.8 |
Room temp |
15 |
20 |
18.4 |
6 |
37.8 |
Comparison of two analytical methods
The detection limit for the spectrophotometric method was previously determined to be 11 μg m−3 for 8 h and 4 μg m−3 for 24 h.12 For the ion chromatography analysis the detection limit (defined as three times the mean standard deviation for the mass on filters of eight samples of low concentration (0.01mg l−1) ) was 2.3 μg m−3 for 8 h and 0.75 μg m−3 for 24 h. The day-to-day reproducibility of the method expressed as the relative standard deviation of the lowest control sample was 1.6% (n = 8). The blank signal in each run was subtracted from the samples. The repeatability of the method was determined as the mean relative standard deviation of a control sample run in 6 replicates at two different occasions and was found to be 1.5%.
The correlation between the two analytical methods is illustrated in Fig. 5. The equation for the line is IC = −0.0132 + (0.9172 × FIA), where IC is the result obtained with ion chromatography and FIA is the result obtained with the spectrophotometric method. The correlation between FIA and IC is good, with a correlation coefficient (r) of 0.998 for the 33 exposures.
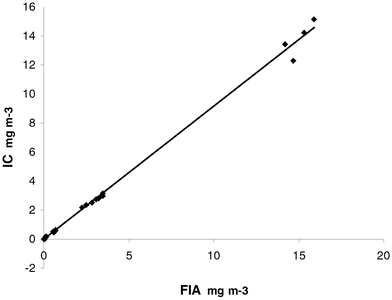 |
| Fig. 5
Correlation between the nitrite concentration analysed by FIA (flow injection analysis) and the concentration analysed by IC (ion chromatography). y = 0.9172x
− 0.0132.
| |
Field validation
Static sampling.
The ratio between diffusive sampling and reference method was 0.93 and 0.85 respectively, and the RSD for the diffusive samplers was below 4% in both experiments. The wind velocity in the exposure chamber during this experiment was very low (0.03 m s−1), which could explain the lower concentration on diffusive samplers, and confirms the laboratory test results of decreased sampling rate with low face velocity.
Personal sampling.
Results are shown in Table 5. No significant differences between concentrations on left and right shoulders were found at the two concentrations (p = 0.84; 0.58). The mean sampling rate at 2 h sampling time (0.9 mg m−3 NO2) was 49.4 ml min−1 , with a RSD for the 22 measurements of 11%, and is well in accordance with the sampling rate determined in the laboratory study. The sampling rate was considerably higher with short sampling time (15 min) and higher concentration of NO2 (2.4 mg m−3 NO2): 57 ml min−1 (RSD = 15%) for 27 measurements. This effect of higher sampling rate at short sampling time could not be seen in the laboratory tests. The 15 min sampling results are uncertain because of the short sampling time, and the fact that they were not recorded on the datalogger, but with an analogue recorder.
The ratio between concentrations on diffusive sampler and reference method was consistently higher (1.06–1.19) with personal sampling compared to static sampling. Subject movement, with the consequence of higher wind speeds in the chamber, could explain the higher sampling rate and therefore higher concentrations on the diffusive sampler.
Table 5
Personal sampling with Willems badge compared to chemiluminescence in the diesel exhaust chamber
Sampling time/min |
n
|
Chemiluminescence |
Willems badge |
Ratio between Willems badge and reference method |
Mean reference concentration/mg m−3 |
Mean concentration/mg m−3 |
Sampling rate/ml min−1 |
RSD(%) |
120 |
22 |
0.87 |
0.92 |
49 |
11 |
1.06 |
60 |
28 |
2.44 |
2.84 |
55 |
13 |
1.16 |
15 |
27 |
2.38 |
2.84 |
57 |
15 |
1.19 |
Conclusions
The Willems Badge diffusive sampler was validated for nitrogen dioxide measurements in laboratory studies as well as in field experiments.
The experimental sampling rate was determined to be 46 ml min−1 with an RSD of 12%. There was no influence on sampling rate of sampling time, concentration of NO2 or relative humidity. The ion chromatography analysis lowers the sensitivity by a factor of about 5. The sampler was found to perform well at wind velocities down to 0.3 m s−1 which makes it suitable for personal measurements. The sensitivity allows it to be used with a sampling time of 1 h for NO2 concentrations down to 0.018 mg m−3. In an 8 h sample the detection limit is 0.002 mg m−3. However at stationary sampling indoors where the wind velocity is less than 0.3 m s−1 the sampler has a lower sampling rate which makes it less suitable for these measurements.
References
- T. Sandström, Eur. Respir. J., 1995, 8, 976 CAS
.
- G. Ainslie, Respir. Med., 1993, 87, 169 CrossRef CAS
.
-
T. Sandström, PhD Thesis, Umeå University, 1989, ISBN 91-7045-036-6
.
-
R. Helleday, PhD Thesis, Umeå University, 1995, ISBN 91-7191-017-4
.
- W. M. Alberts, J. Allergy Clin. Immunol., 1994, 94, 289 CrossRef CAS
.
- O. Raaschou-Nielsen, M. L. Nielsen and J. Gehl, Arch. Environ. Health, 1995, 50, 207 Search PubMed
.
- N. M. Elsayed, Toxicology, 1994, 89, 161 CrossRef CAS
.
- R. H. Brown, J. Environ. Monit., 2000, 2, 1 RSC
.
- P. Hofschreuder, W. van der Meulen, P. Heeres and S. Slanina, J. Environ. Monit., 1999, 1, 143 RSC
.
- A. Hagenbjörk-Gustafsson, B. Forsberg, G. Hestvik, D. Karlsson, S. Wahlberg and T. Sandström, Analyst, 1996, 121, 1261 RSC
.
- A. J. Gair and S. A. Penkett, Atmos. Environ., 1995, 29, 2529 CrossRef CAS
.
- A. Hagenbjörk-Gustafsson, R. Lindahl, J.-O. Levin and D. Karlsson, J. Environ. Monit., 1999, 1, 349 RSC
.
-
R. Lindahl, PhD Thesis, Umeå University, 1997, ISBN 91-7191-355-6
.
- CEN, European Committee for Standardization, Workplace atmospheres—Diffusive samplers for the determination of gases and vapours—Requirements and test methods. EN 838, Brussels, 1995 Search PubMed
.
-
R. Carlson, Design and Optimization in Organic Synthesis (Data Handling in Science and Technology), Elsevier, Amsterdam, vol. 8, 1992 Search PubMed
.
|
This journal is © The Royal Society of Chemistry 2002 |