DOI:
10.1039/B106282B
(Paper)
Analyst, 2002,
127, 178-182
A new technique to determine organic and inorganic acid contamination†
Received
13th July 2001
, Accepted 1st October 2001
First published on 12th December 2001
Abstract
A new acid indicator pad was developed for the detection of acid breakthrough of gloves and chemical protective clothing. The pad carries a reagent which responds to acid contaminant by producing a color change. The pad was used to detect both organic and inorganic acids permeating through glove materials using the modified ASTM F-739 and direct permeability testing procedures. Breakthrough times for each type of glove were determined, and found to range from 4 min to >4 h for propionic acid, from 3 min to >4 h for acrylic acid, and from 26 min to >4 h for HCl. A quantification was performed for propionic and acrylic acids following solvent desorption and gas chromatography. Both acids exhibited >99% adsorption {the acid and its reactivity (the acid reacted with an indicator to contribute the color change)} on the pads at a spiking level of 1.8 μL for each acid. Acid recovery during quantification was calculated for each acid, ranging from 52–72%
(RSD ⩽ 4.0%) for both acids over the spiking range 0.2–1.8 μL. The quantitative mass of the acids on the pads at the time of breakthrough detection ranged from 260–282 and 270–296 μg cm−2 for propionic acid and acrylic acid, respectively. The new colorimetric indicator pad should be useful in detecting and collecting acid permeation samples through gloves and chemical protective clothing in both laboratory and field studies, for quantitative analysis.
Introduction
The use of chemical resistant gloves and protective clothing is the primary method employed to prevent skin exposure to toxic chemicals in the workplace.1 Chemical-resistant gloves are typically selected based on laboratory permeation testing, following the procedure known as the ASTM F-739 method or a modification of this method.2 This method provides breakthrough times and permeation rates for volatile chemicals so that one can choose the most appropriate glove types for use in a particular application. However, many workplace variables influence glove performance, including flexing, increased temperature, and differences between glove manufacturers and production lots. Moreover, the absorption of chemicals through the skin varies widely among different chemicals and cannot be fully predicted from knowledge concerning the physical and chemical properties of the chemicals. Limited laboratory-test data cannot address all these
variables.3–6
A new acid indicator was developed and used for the detection of organic and inorganic acids which are widely used and are thought to be causing significant workplace injury by the skin route. Propionic acid is used in synthesis of fungicides, herbicides, flavorings, perfumes, pharmaceuticals, and in the production of cellulose propionate plastics. The 2001 ACGIH threshold-limit-value time-weighted-average (TLV-TWA) for propionic acid is 10 ppm (30 mg m−3) based on irritation effects.7 Acrylic acid is used in plastics, water purification, paper and cloth coatings, and in medical and dental materials. The 2001 American Conference of Government Industrial Hygienists (ACGIH) TLV-TWA for acrylic acid is 2 ppm (5.9 mg m−3) with a notation for skin.8 Hydrochloric acid is used in the production of chlorinated organic chemicals, the production of dyes, steel pickling, and clean metal products. The
1995 ACGIH short-term exposure limit (STEL)/ceiling for hydrochloric acid is 5 ppm (7.5 mg m−3) based on irritation and corrosion effects.9
Indicator pads produce a color change if contamination occurs; therefore, if workers wear the indicator pads, they are able to detect contamination and learn to protect themselves from skin exposure to toxic chemicals. This approach has been used successfully as described by Vo et al.10–12 Although an ‘Acid/Base Permea-Tec’ indicator has been developed and is available from Colorimetric Laboratories, Inc (Des Plaines, IL), this indicator is not successful in detecting organic acids or weak acids. We report here a new development of rapid and reliable acid indicator pads to detect both organic and inorganic acids and to provide a method to determine the mass of permeating acids at timed intervals.
Materials and methods
Chemicals
Unless otherwise noted, all starting materials, all acids used for this study, and solvents were obtained from a commercial supplier (Aldrich Chemical, Milwaukee, WI) and used as neat standard chemicals without further purification.
Selected gloves, pad materials, and other apparatus
The six types of gloves selected for this study are identified in Table 1. Pad materials (Whatman Benchkote Plus, Catalog No.: 2301-6150) were purchased from Fisher Scientific (Pittsburgh, PA).
Table 1
Glove materials used in chemical permeation study
Glove type |
Material of glove |
Model or Log No. |
Manufacturer |
Guardian |
Butyl rubber |
Butyl-std model No. 1-196 |
Guardian Company (Willard, OH) |
Dura-Touch |
PVC (disposable vinyl) |
Model No. 34-100 |
Ansell-Edmont (Coshocton, OH) |
Pylox |
PVC |
Model No. V-10 |
MAPA Pioneer (Buffalo, NY) |
Neoprene |
Neoprene |
Model No. 29-845 |
Ansell-Edmont (Coshocton, OH) |
Micro-Grip |
Nitrile |
Model No. 40101-350 |
VWR Scientific Products (West Chester, PA) |
Sol-Vex |
Nitrile rubber |
Model No. 37-14510 |
Ansell-Edmont (Coshocton, OH) |
An Ames (Waltham, MA), 214-10 micrometer with a pressure foot of 1 cm was used to determine the thickness of each glove. A Miran-IA (Miniature Infrared Analyzer) closed-loop configuration (Fig. 1) which consisted of a metal bellows pump (Model MB-41, Metal Bellows, Sharon, MA), a 2.5 cm chemical permeation cell (2.5 cm in diameter, AMK Glass Company, Vineland, NJ), and a Miran (Foxboro, Norwalk, CT) was used to determine time to breakthrough for the glove material. A CEM (Matthews, NC) MES-100 microwave-extraction (ME) system was used to extract acids in pads. Savillex vials (3 mL vials, part number 221253; these vials could be used under heat condition while the vials were covered to prevent evaporation loss) were purchased from CEM. A Perkin-Elmer (Norwalk, CT) gas chromatographic system, which consisted of a PE Nelson Model 1022 Personal Integrator and an AutoSystem gas chromatograph with flame ionization detector (FID), was used to analyze chemicals.
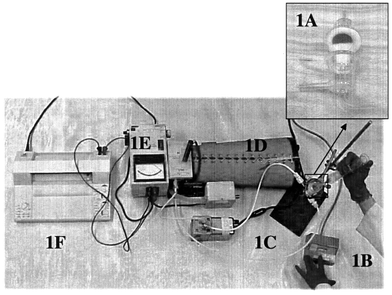 |
| Fig. 1
A Miran (Miniature Infrared Analyzer) closed-loop configuration: a 2.5 cm chemical permeation cell (1A), timer (1B), metal bellows pump (1C), Miran (1D), detector (1E), and charter recorder (1F).
| |
Acid indicator synthesis and pad fabrication
2-[4-(dimethylamino)phenylazo]benzoic acid, sodium salt was added slowly to a freshly prepared solution of 40 mM NaOH to a final concentration of 2 mM. The solution immediately adopted an orange color appearance, and the reaction was allowed to proceed at room temperature with continuous stirring for 2 h. The solution was then purified to separate the final product from excess reagents.
New pad materials were removed from sealed packages, and cut into 2.0 cm diameter circles or 1.8 cm side squares using a puncher. The minimum concentration of the indicator solution was applied into the pads. The pads were then dried at 38 ± 1 °C (conduct stability tests of the pads at temperature designed, 38 ± 1 °C, were based on three main factors to approximate within-glove temperatures under in-use conditions: the hand’s skin temperature is approximately 34 °C, hand movements will increase temperatures slightly, and conditions of high relative humidity inside gloves will also increase temperatures slightly) for 4 h using Isotemp Oven (Model 615F, Fisher Scientific) before being used.
Evaluation of sorption properties of pads
Spiking pads.
New indicator pads were tested to determine if there was any pad-media interference. Known volumes of each acid (0.2–1.8 μL) were added directly to the surface of the pads [half-circles of 2.0 cm diameter pads (Fig. 1, 1A) or 1.8 cm side square pads]. The pads were then inserted into 300 μL of extraction solvent (cyclohexane) in 3 mL Savillex vials and the vials were covered with the vial caps.
Efficiency of adsorption during acid spiking.
In order to assess whether complete adsorption of each acid (including its reactivity) is accomplished during spiking on pads, two Savillex vials were used. In the first vial, 1.8 μL of each acid was added to a pad and the vial was covered with the vial cap. The vial was left at room temperature for 20 min before the pad was inserted in 300 μL of extraction solvent in the second vial. Then, 300 μL of the extraction solvent was added directly to the first vial to extract excess acid which did not adsorb or react on the spiked pad. These vials were then used for the extraction process and GC analysis.
The ME process and GC analysis.
The ME-GC procedure was run according to the method of Vo et al.7 as follows. The closed vials were immersed in 25 mL of water in extraction vessels, then, the vessels were placed in the MES-100 system and extracted for 15 min at 100 °C, 70 psi, and 60% power. The extracted solutions were allowed to cool to room temperature for 20 min before opening the vial caps for GC analysis in order to obtain maximum recovery of these chemicals without evaporation loss. The optimum GC conditions were as follows: column, 3.05 m × 3.18 mm id, 3% SP-1500 on 80-120-mesh Carbopack B (Supelco, Bellefonte, PA); helium flow rate, 25 mL min−1; oven temperature, 180 °C; column temperature, 180 °C; temperature of the flame ionization detector (FID), 240 °C; data collection time, 0–15 min; main plot time, 0–6 min; and y-maximum, 50 mV. Volumes of 5 μL of
extracted samples of spiked acids in extracted solutions were injected into the GC column using a syringe (0.1–5.0 μL), up to four times for each sample. The areas of the spiked acid peaks in the resulting gas chromatograms were used for spiked acid determinations.
Acid recovery.
Standard acid determinations were performed using the same spiked acid procedure, but without using pads. Known volumes of each acid (0.2–1.8 μL) were added directly to 300 μL of extraction solvent in the vials. These vials were then used for the extraction process and GC analysis. The areas of the standard peaks in the resulting gas chromatograms were used for standard acid determinations. Acid recovery was calculated as the percentage of spiked acid peak area divided by standard acid peak area.
Glove thickness test
The thickness test was performed at three positions on each glove: the palm, the entire middle finger, and the cuff (3 cm from the open end), as these represent the areas of highest contact and glove abrasion, which enhances chemical penetration. Five thickness measurements for each position on the glove were recorded and the mean thickness and variation were calculated. All measurements were made to the nearest ±0.01 mm.
Glove breakthrough time determination
A modified ASTM F-739 method was used to determine breakthrough time (Fig. 1). The Miran conditions were as follows: slit, 1.0 mm; wavelength, 8.8 μm (for propionic acid experiment), 8.9 μm (for acrylic acid experiment), and 3.4 μm (for HCl experiment); pathlength, 20.25 m (for propionic, acrylic, and HCl experiments). The 2.5 cm permeation cell is divided into a ‘challenge side’ which contains the chemicals, and a ‘collection side’ which contains the sweep gas (flow rate, 11.33 L min−1). A glove membrane (sections from the palm and the cuff of gloves) separated the two sides of the permeation cell, with the outer surface toward the challenge side of the permeation cell. A half circle of a pad was attached to half of the inner surface of the glove section and covered with parafilm, then clear plastic tape, so the half circle of the pad was in direct contact with the glove surface and easily removed from the
glove. The other half of the glove section was left unobstructed so that permeating chemicals could reach the analyzer detector. This system was operated in the closed-loop mode and the experiment was conducted at room temperature (22 ± 1 °C). 15 mL of each acid was injected into the challenge side of the cell using a 30 mL glass syringe, and a timer and the circulation pump were immediately started. Permeation of each acid through the glove was detected by the change in color of the pads (a visible color indication of the pads changed immediately from yellow to dark brown when contamination of an acid or acids occurred) or the analyzer-detected response. A direct permeability testing procedure12 was also performed on the finger position of gloves. A finger of the gloves was turned inside out and a pad was attached to the finger. The glove finger was then fastened to a glass cylinder (2 × 15 cm) by using duct tape at the open end of the glove
finger as the attachment area. The glass cylinder served as a fill tube. A 10 mL volume of challenge chemical was injected into the glass cylinder and a timer was immediately started. Permeation of each acid through the glove finger was detected by the change in color of the pads. Breakthrough time was recorded and the pads were immediately removed from the glove and inserted into 300 μL of extraction solvent in the vials for the extraction process.
Quantitative determination of acids
The closed vials of each organic acid were extracted, and 5 μL of extracted samples were subjected to GC analysis as described above. The areas of the peaks in the resulting gas chromatograms were used for challenge acid quantification. The quantitative concentration of challenge acids was determined against a known concentration of spiked acids based on its linear equation and the degree of each organic acid recovery.
HCl (an inorganic acid) can be quantified using solvent desorption and HPLC analysis; however, HCl is a very strong irritant of the eyes, mucous membranes, and skin, therefore, in order to limit exposure to HCl, we only used the indicator pad to detect HCl permeation through gloves to determine breakthrough times, but not for quantification.
Results
Pad sensitivity
Permeation of each acid through the gloves was detected by the change in color of the pads before the IR analyzer detector responded. It was shown that a new acid indicator pad is very sensitive to detect the presence of both organic and inorganic acids with a small amount of 0.2 μL of each acid applied to the pads. Acids caused the pads to change from yellow to dark brown {the pad which carries thereon a predetermined reagent was designed to be responsive to contact by an acid or acids to produce a visible color indication (a positive indication results in a color change from yellow to dark brown)}.
Efficiency of adsorption during each acid spiking
Excess acids which did not adsorb or react on the spiked pads in the first vial were low, being <1% for all acids (the area peaks were 0.16% and 0.19% of the area peaks found in vial No. 2 for propionic and acrylic acids, respectively). Generally, all acids exhibited >99% adsorption (including acid reactivity) on the pads at a spiking level of 1.8 μL for each acid.
Resolution
Good resolution (peaks did not overlap) of propionic acid, acrylic acid, and cyclohexane (extraction solvent) was achieved by setting the optimum GC conditions as described above. None of the blanks (unexposed pads in the extraction solvent) produced chromatograms containing peaks corresponding to these acids used in this study. The GC retention times for the acids and the extraction solvent obtained under these conditions are given in Table 2.
Table 2
Recoveries of two commonly used organic acids (0.2–1.8 μL of each acid added to pads). 300 μL of cyclohexane (retention time, 6.92 min) was used to extract propionic and acrylic acids. 5 μL of the extracted solutions was analyzed by GC
Acids (retention time) |
Volumes of acid applied to filter/μL |
Peak areas (×100) |
Recovery (%) |
Propionic acid (4.48 min) |
0.2 |
11.6 |
56 ± 3.8 |
0.6 |
35.8 |
61 ± 3.7 |
1.0 |
58.4 |
65 ± 3.6 |
1.4 |
87.2 |
69 ± 3.2 |
1.8 |
107.5 |
72 ± 2.9 |
Acrylic acid (5.08 min) |
0.2 |
4.9 |
52 ± 4.0 |
0.6 |
25.3 |
56 ± 2.9 |
1.0 |
41.1 |
59 ± 3.5 |
1.4 |
60.6 |
62 ± 3.7 |
1.8 |
76.4 |
65 ± 2.3 |
Calibrations of pads
The relationship between signals (peak area) on the gas chromatograms and volumes over the range 0.2–1.8 μL (Table 2) of each acid applied to pads was analyzed using Microsoft Excel software. Fig. 2 shows a linear correlation for two organic acids over the range 0.2–1.8 μL (n = 5) of each acid added to the pads (2a, acrylic acid, r2 = 0.997 and 2b, propionic acid, r2 = 0.998).
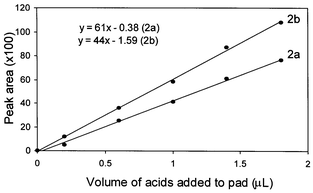 |
| Fig. 2
Calibration plots of peak areas against volumes of the two acids added to pads following the extraction process (the source of the data in Table 2). 5.0 μL of the extracted solution was applied to GC analysis. The best linear correlation for each acid was obtained over the range 0.2–1.8 μL (n = 5). The best straight-line fit from linear analysis of the data is also shown (2a, acrylic acid, r2 = 0.997 and 2b, propionic acid, r2 = 0.998).
| |
Acid recovery
For experiments performed with a repeated extraction process and GC measurements, recoveries from 52 to 72% (RSD ⩽4%) were obtained over the range 0.2–1.8 μL of each acid applied to the pads (Table 2).
Glove thickness test
Table 3, 4 and 5 summarize the results obtained for the mean thickness of five thickness measurements for each type of gloves.
Table 3
Results of the glove permeation tests (n = 3) for propionic acid
Glove type |
Glove material |
Thicknessa
{finger}/mm |
Breakthroughb time of acrylic acid {finger} |
Thicknessa
{palm & cuff}/mm |
Breakthroughc time of propionic acid {palm & cuff} |
Mass of propionic acid/μg (½-circle pad) |
Mean thickness ±
s (n = 4).
Breakthrough time detected by the change in color of indicator pads (the direct permeability testing procedure).
Breakthrough time detected by the change in color of indicator pads (the ASTM F739 testing procedure).
|
Guardian |
Butyl rubber |
0.25 ± 0.01 |
>4 h |
0.22 ± 0.01 |
232 ± 1 min |
408 ± 3 (260 μg cm−2) |
Dura-Touch |
PVC (disposable vinyl) |
0.17 ± 0.01 |
10 ± 1 min |
0.18 ± 0.01 |
9 ± 1 min |
434 ± 4 (276 μg cm−2) |
Pylox |
PVC |
0.24 ± 0.01 |
18 ± 1 min |
0.23 ± 0.01 |
16 ± 1 min |
428 ± 4 (273 μg cm−2) |
Neoprene |
Neoprene |
0.39 ± 0.01 |
140 ± 2 min |
0.39 ± 0.01 |
135 ± 2 min |
412 ± 3 (262 μg cm−2) |
Micro-Grip |
Nitrile |
0.13 ± 0.01 |
5 ± 1 min (swelled) |
0.12 ± 0.01 |
4 ± 1 min |
443 ± 5 (282 μg cm−2) |
Sol-Vex |
Nitrile rubber |
0.30 ± 0.01 |
66 ± 1 min (swelled) |
0.29 ± 0.01 |
59 ± 1 min |
417 ± 3 (266 μg cm−2) |
Table 4
Results of the glove permeation tests (n = 3) for acrylic acid
Glove type |
Glove material |
Thicknessa
{finger}/mm |
Breakthroughb time of acrylic acid {finger} |
Thicknessa
{palm & cuff}/mm |
Breakthroughc time of acrylic acid {palm & cuff} |
Mass of acrylic acid/μg (½-circle pad) |
Mean thickness ±
s (n = 4).
Breakthrough time detected by the change in color of indicator pads (the direct permeability testing procedure).
Breakthrough time detected by the change in color of indicator pads (the ASTM F739 testing procedure).
|
Guardian |
Butyl rubber |
0.25 ± 0.01 |
>4 h |
0.22 ± 0.01 |
229 ± 1 min |
424 ± 3 (270 μg cm−2) |
Dura-Touch |
PVC (disposable vinyl) |
0.17 ± 0.01 |
8 ± 1 min |
0.18 ± 0.01 |
7 ± 1 min |
452 ± 4 (288 μg cm−2) |
Pylox |
PVC |
0.24 ± 0.01 |
16 ± 1 min |
0.23 ± 0.01 |
15 ± 1 min |
441 ± 4 (281 μg cm−2) |
Neoprene |
Neoprene |
0.39 ± 0.01 |
139 ± 2 min |
0.39 ± 0.01 |
131 ± 2 min |
429 ± 3 (273 μg cm−2) |
Micro-Grip |
Nitrile |
0.13 ± 0.01 |
4 ± 1 min |
0.12 ± 0.01 |
3 ± 1 min |
464 ± 5 (296 μg cm−2) |
Sol-Vex |
Nitrile rubber |
0.30 ± 0.01 |
65 ± 1 min |
0.29 ± 0.01 |
59 ± 1 min |
433 ± 3 (276 μg cm−2) |
Table 5
Results of the glove permeation tests (n = 3) for HCl
Glove type |
Glove material |
Thickness a{finger}/mm |
Breakthroughb time of HCl acid {finger} |
Thickness a{palm & cuff}/mm |
Breakthroughc time of HCl acid {palm & cuff} |
Mean thickness ±
s (n = 4).
Breakthrough time detected by the change in color of indicator pads (the direct permeability testing procedure).
Breakthrough time detected by the change in color of indicator pads (the ASTM F739 testing procedure).
|
Guardian |
Butyl rubber |
0.25 ± 0.01 |
>4 h |
0.22 ± 0.01 |
>4 h |
Dura-Touch |
PVC (disposable vinyl) |
0.17 ± 0.01 |
30 ± 1 min |
0.18 ± 0.01 |
26 ± 1 min |
Pylox |
PVC |
0.24 ± 0.01 |
60 ± 1 min |
0.23 ± 0.01 |
56 ± 1 min |
Neoprene |
Neoprene |
0.39 ± 0.01 |
>4 h |
0.39 ± 0.01 |
>4 h |
Micro-Grip |
Nitrile |
0.13 ± 0.01 |
54 ± 1 min |
0.12 ± 0.01 |
34 ± 1 min |
Sol-Vex |
Nitrile rubber |
0.30 ± 0.01 |
>4 h |
0.29 ± 0.01 |
>4 h |
Glove breakthrough time determination
Table 3, 4 and 5 show the breakthrough times for all of the gloves tested with the ASTM F-739 standard and direct permeability testing procedures. Permeation of each acid through the gloves was detected by the change in color of the pads, before the analyzer detector responded. It was found that the change in color of the pads was detected 30 s to 5 min before the IR analyzer detector responded for three acids studied. Slow permeation by propionic and acrylic acids was observed for butyl rubber material, while rapid permeation for polyvinyl chloride (PVC, Dura-Touch and Pylox), nitrile (Micro-Grip) materials was observed (Table 3 and 4). For HCl, slow permeation was observed for polyvinyl chloride (PVC, Dura-Touch and Pylox), nitrile (Micro-Grip) materials, while no permeation was observed for butyl rubber, neoprene,
and nitrile rubber (Sol-Vex) materials after 4 h (Table 5).
Quantitative determination of each acid
The volumes of each acid on a half-circle pad at the point of permeation detection was determined, ranging from 0.23–0.25 and 0.21–0.23 μL for propionic and acrylic acids, respectively (based on acid recoveries and their linear equations from Fig. 2). These volumes represent 260–282 and 270–296 μg cm−2 for propionic and acrylic acids, respectively [area of a half-circle pad = ½
{π
× (½ diameter)2} = ½
{π
× (2.0 cm/2)2}].
Discussion
Pad material is very stable at 60 °C for 24 h. It has a high absorption of toxic chemicals or solvents (absorb >750 mL m−2 as described in Fisher Catalog, 2000/01, p. 172). Indicator solutions applied to pads are very low vapor pressure and low concentration, and the reverse side of the pad is covered with a barrier layer, polyethylene, which is impermeable; therefore, these pads are less likely to give respiratory and dermal exposures. A new acid indicator pad was very sensitive to detect the presence of both organic and inorganic acids with a small amount of 0.2 μL of each acid applied to the pads. The new pad is very stable at temperature ranges from 22–39 °C.
Although the results obtained with the solvent desorption-GC procedure were consistent for reproducible recovery of each acid, the recovery of each acid from pads was low, ranging from 52 to 72% over the range 0.2–1.8 μL. It indicates that the acid reacted with the indicator to contribute the color change and to give another product. Recovery was dependent on the volume of each acid applied to the pads, with increasing recovery observed with increasing volume. Excellent linear correlations were obtained for these acids over the spiking range 0.2–1.8 μL on pads using 5.0 μL of the extracted solutions for GC analysis.
The results of the permeation experiments demonstrated that within a given material type of the gloves tested, the thicker gloves had the longer breakthrough times for the test acids. Differences in glove materials or test acids yielded different breakthrough times.
The quantitative mass data of test acids on the indicator pads, ranging from 260–282 and 270–296 μg cm−2 for propionic and acrylic acids, respectively, indicated that these acids can be detected on the pads at the breakthrough times. The fact that the amounts of permeant detected in each glove test for each acid were consistent indicates that the pads performed independent of the glove type in consistently collecting amounts of acids during the time before breakthrough was detected by the ASTM method. The new indicator pad is therefore more sensitive to detecting breakthrough, by the amounts reported, than the ASTM method. Further evidence that the method is consistent and reliable was seen in the very small replicate glove result variations. The results also show that the new pad has high sensitivity to weak acids or other organic acids that may be comparable to an indicator from the Colorimetric Laboratory, Inc.
In future field studies, the high-efficiency pads may be suitable for use in detecting and collecting acid permeation samples through gloves and chemical protective clothing for quantitative analysis. The workers could carry out their tasks in accordance with normal work practices. When contamination occurs, indicator pads produce a color change; therefore, the workers will need to stop work and remove the outer glove.
Conclusion
The results presented herein indicate that the indicator pads evaluated as a glove performance sampler performed effectively for both organic and inorganic acids used as test chemicals. The results indicate that pads exposed to the acids studied can be successfully analyzed under the conditions for solvent desorption and GC analysis as described. These pads can be easily affixed to the inside of gloves while in use in the workplace, and a permeating chemical can be detected and quantitatively determined. The mass of acids detected on the inside of gloves can be used to determine adequacy of protection, duration of acceptable use, or need for substitution of a more effective glove type.
Acknowledgement
The author thanks Dr. Sidney Soderholm and Mr. Paul Keane (NIOSH, Morgantown, WV), for their valuable assistance in the preparation of the manuscript.
References
- OSHA, U.S. Occupational Safety and Health Administration: Personal Protective Equipment for General Industry, Final Rule. CFR 59, No. 66, 1994, pp. 16334–16364 Search PubMed.
- S. Berardinelli and E. Moyer, Am. Ind. Hyg. Assoc. J., 1987, 48(4), 324 CrossRef CAS.
-
D. Ehntholt, I. Bodek and J. R. Valentine, Chemical Protective Clothing Performance in Chemical Emergency Response ASTM STP 1037, ed. J. L. Perkins
and J. O. Stull, American Society of Testing and Materials, Philadelphia, PA, 1989 Search PubMed.
- E. Zellers and R. Sulewski, Am. Ind. Hyg. Assoc. J., 1993, 54, 465 CrossRef CAS.
- J. L. Perkins and K. Vesical, Appl. Occup. Environ. Hyg., 1997, 12, 206 CAS.
- J. L. Perkins and B. Pool, Am. Ind. Hyg. Assoc. J., 1997, 58, 474 CrossRef CAS.
-
G. J. Hathaway, N. H. Proctor and J. P. Hughes, Proctor and Hughes’ Chemical Hazards of the Workplace, John Wiley and Sons, New York, 4th edn., 1996, p. 535 Search PubMed.
-
G. J. Hathaway, N. H. Proctor and J. P. Hughes, Proctor and Hughes’ Chemical Hazards of the Workplace, John Wiley and Sons, New York, 4th edn., 1996, pp. 25–26 Search PubMed.
-
G. J. Hathaway, N. H. Proctor and J. P. Hughes, Proctor and Hughes’ Chemical Hazards of the Workplace, John Wiley and Sons, New York, 4th edn., 1996, pp. 344–345 Search PubMed.
- E. Vo, S. Berardinelli and R. Hall, Analyst, 1999, 124, 941 RSC.
- E. Vo and S. Berardinelli, J. Environ. Monit., 1999, 1(6), 545 RSC.
- E. Vo, S. Berardinelli, R. Hall and N. Ayouby, Am. Ind. Hyg. Assoc. J., 2000, 61(6), 847 CrossRef.
Footnote |
† ©US Government. |
|
This journal is © The Royal Society of Chemistry 2002 |