DOI:
10.1039/B009190L
(Paper)
Green Chem., 2001,
3, 23-25
A novel Suzuki reaction system based on a supported
palladium catalyst
Received (in Cambridge, UK) 7th July 2000
First published on 18th January 2001
Abstract
A range of supported palladium complex-catalysed Suzuki
reactions is described with notable features including fast and efficient
reactions, excellent catalyst recyclability, and total catalyst stability
under the reaction conditions. We have achieved turnover numbers of several
thousand based on ten re-use experiments from batch reactions in air. Our
system not only solves the basic problems of catalyst separation and
recovery but also avoids the use of phosphine ligands.
Green ContextThese Suzuki reactions combine the efficiency and high turnover number
typical of palladium catalysis, with the recoverability and reusability
offered by heterogeneous systems, to give high yields in short times
without the need for phosphine ligands.ST |
Introduction
The Suzuki reaction is proving to be an increasingly popular method for
forming carbon–carbon bonds both in the laboratory and in commercial
manufacturing; it has quickly become an integral part of modern organic
synthesis.1,2 The reaction represents
an attractive alternative over other methods using organometallics because
organoboranes are air- and moisture-stable with relatively low
toxicity.1 However, like many organic
reactions using inorganic reagents and catalysts, the standard procedures
suffer from wasted inorganics which are too difficult to recover and are
lost in an aqueous work-up stage.3 For
Suzuki reactions soluble complex palladium catalysts are normally employed
alongside bases such as soluble amines; these are rarely recoverable
without elaborate and wasteful procedures that are commercially
unacceptable. Some progress has been made with the use of solid reagents,
for example the combination of alumina-supported fluorides and palladium
powder has been recently shown to be effective in the Suzuki coupling of
phenyl boronic acids with iodobenzenes.4,5 However, the reaction is much less effective with
bromobenzenes and there is no evidence for the recyclability of the
palladium, an essential aspect of the process on environmental and economic
grounds. The use of an efficient heterogeneously supported palladium
catalyst (Pd/C) in Suzuki cross-coupling has been used to produce
biphenylacetic acid.6 In other reported
cases supported palladium catalysts have suffered on application from
diffusion limitations and dissolution of the palladium complex in the
reaction medium. Here we describe an entirely novel heterogeneous
palladium-catalysed Suzuki reaction system that is very effective with the
less expensive bromobenzenes, uses only hydrocarbon solvents, and requires
very small amounts of the solid palladium catalysts which are entirely
recoverable and reusable; there is no contribution to the reaction due to
homogeneous catalysis from any leached metal complex. One salient feature
of our catalyst is that it does not require addition of phosphines
(commonly introduced into palladium-catalysed reactions) thus improving the
atom economy of the reaction. While reducing process costs, it also
eliminates side reactions that may occur between arylphosphines and
arylboronic acid.7 The overall outcome of
these improvements may lead to cost effective industrial processes and to
the reduction of unwanted wastes.Results and discussion
The organically modified silica (Merck Kieselgel 100) and micelle
templated silicas (MTS) materials used for preparing the catalysts were
prepared by a one-pot method.8 They were studied for the
presence and stability of bound organics by diffuse reflectance infrared
spectroscopy (DRIFTS) and simultaneous thermal analysis (STA). The DRIFTS
of 3-aminopropyl-MTS for example, displays the characteristic
C–H2 stretching bands at 2938 and 2867
cm−1 and aliphatic CH2 deformation bands at
1471 and 1439 cm−1. The IR spectrum of the chemically
modified 3-aminopropyl-MTS and 3-aminopropyl-silica prior to complexation
with the metal had a peak at 1647 cm−1 due to the
C
N stretching vibration of the imine. The peak disappears on
complexation with palladium, changing into bands at 1525 and 1593
cm−1 consistent with strong binding between the metal and
the ligand. Simultaneous thermal analysis shows a loss of residual solvent
at ca. 80 °C followed by a gradual minor weight loss of <
1% up to ca. 505 °C where a sudden major loss (ca.
10%) is observed. The major weight loss at such high temperatures in the
MTS material is characteristic of chemisorbed material and shows that the
3-aminopropyl is chemically bound. The thermal stability of the organically
modifed Kieselgel 100 is less, with decomposition occuring between 205 and
385 °C.The palladium catalysts bound on these materials have been tested in the
Suzuki reaction between phenylboronic acid and bromobenzene in xylene in
the presence of potassium carbonate as the base. Catalysts based on the
solid carboxylic acids9
[TMS–(CH2)nCO2H leading to
catalyst 1] are active but quickly lose activity (turnover number ArBr/Pd =
ca. 100) although washing the catalyst after use (with
dichloromethane and then water) restored some of the original activity,
giving up to 76% yields (Table 1, cycle
3). Catalysts 2 and 3 are based on reaction of aminopropyl–TMS
(leading to catalyst 2) and aminopropylsilica (leading to catalyst 3) with
pyridinecarbaldehyde,10 followed by
complexation of palladium acetate (Fig. 1)
are more active and have excellent recyclability (Table 1). With these catalysts, we have achieved
turnover numbers of several thousand based on ten filtrations and reuse
experiments from batch reactions. The other advantage of our systems,
compared to work up procedure,1a is
that high yields of the biaryls are obtained in air at the lower
temperature of 95 °C, at shorter times and without using phosphine
ligands.
Table 1 Comparison of the activities on reuse of different supported palladium
catalysts on the Suzuki reaction between phenylboronic acid and
bromobenzene at 95 °C in xylene and K2CO3 as
basea
Catalyst cycleb | Catalyst | t/min | GC Yieldb
(%)c |
---|
The reactions were carried out in xylene (13 ml) with 5 mmol of
bromobenzene, 7.3 mmol of phenylboronic acid, 10 mmol of
K2CO3 base and 0.2 g palladium catalyst (loading 0.3
mmol g−1) at 95 °C. The used catalysts were thoroughly washed with dichloromethane then
water before reuse. GC yields obtained using n-dodecane as an internal standard and
is based on the amount of haloarene employed in relation to authentic
standard biphenyl. |
---|
1 | 1 | 60 | 75 |
2 | 1 | 180 | 4.4 |
3 | 1 | 180 | 76 |
4 | 1 | 180 | 25 |
1 | 2 | 60 | 75 |
2 | 2 | 60 | 98 |
3 | 2 | 60 | 97 |
4 | 2 | 60 | 98 |
5 | 2 | 60 | 96 |
6 | 2 | 60 | 45 |
1 | 3 | 60 | 90 |
2 | 3 | 60 | 98 |
3 | 3 | 60 | 97 |
4 | 3 | 60 | 96 |
5 | 3 | 60 | 95 |
6 | 3 | 60 | 85 |
7 | 3 | 60 | 64 |
8 | 3 | 60 | 70 |
9 | 3 | 60 (120) | 36 (44) |
10 | 3 | 60 (180) | 17 (20) |
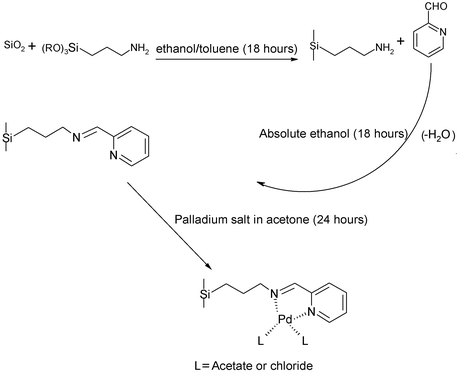 |
| Fig. 1 Preparation of the active and reusable supported palladium catalysts
(catalysts 2 and 3) based on chemically modified aminopropyl mesoporous
silicas. | |
The stability of the supported complex towards dissolution during the
reaction is a problem of great concern for most anchored catalysts.11 In view of the leaching problems observed with
palladium supported on polymer beads,12
quantitative analysis using atomic absorption spectroscopy (AAS) was
employed to determine the amount of metal in the final reaction solution.
We also performed the hot filtration test.13 No palladium could be detected in the liquid
reaction mixtures by AAS and more significantly, hot filtration of the
reaction mixture at an early stage of the reaction followed by taking the
mixture back to reaction temperature completely stopped the reaction,
effectively proving heterogeneous catalysis and no measurable contribution
from homogeneous catalysis (Fig. 2).13 Very good to excellent yields of biphenyl in
short reaction times for reactions run using catalysts 2 and 3 were
achieved with the latter having the better lifetime. The study was then
extended to substituted bromobenzenes involving electronically activating
and deactivating groups. A favourable effect of electron-withdrawing
substituents is normally observed in palladium catalysed reactions.14 With our catalysts however, electron withdrawing
groups have relatively little effect on the reaction rate or selectivity
(Table 2). Substituted chlorobenzenes
are inert under the standard conditions giving a cross-coupling product
(<2% yield) and a 4% yield of biphenyl from homocoupling of the
phenylboronic acid. To further illustrate that chloroarenes are inert in
the reaction system, p-chlorobiphenyl was selectivity produced in
the reaction of phenylboronic acid with p-bromochlorobenzene
(Table 2, entries 6, 14). The importance
of the base is illustrated by the lack of desired cross-coupling product
when the reaction is run in the absence of base.
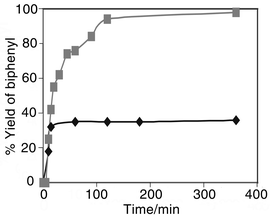 |
| Fig. 2 Effect of removing the supported palladium catalyst from the reaction of
phenylboronic acid and bromobenzene (hot filtration test, see text). | |
Table 2 Suzuki reactions of substituted bromobenzenes catalysed by supported
phosphine free palladium catalysts

Experimental
Chemicals were obtained from Aldrich and Merck and were used as
received. The detailed preparation schemes of the catalysts have already
been published.9,10 In this instance
however, the organically modified MTS was prepared by a one-pot synthesis
method8 using a long-chain neutral
templating amine (5.10 g n-dodecylamine in this case) in aqueous
ethanol (46 ml ethanol–53 ml water). To this solution, 8.36 g (40
mmol) of tetraethoxysilane (TEOS) and 1.79 g (10 mmol) of
3-aminopropyl(trimethoxy)silane were separately but simultaneously added
and stirred together at room temperature for 18 h. After this solution, the
mixture is initially turbid, then turns milky after about 10 min and
finally forms a thick white paste after 18 h. The thick white paste was
filtered off and the template was extracted from the white solid by Soxhlet
extraction with ethanol for 10 h. The template-extracted white
3-aminopropyl-MTS solid was dried in air at 90 °C overnight before
further reactions appropriate for metal complex anchoring were carried out.
The dried 3-aminopropyl-MTS (or silica) was reacted with an equivalent of
2-pyridinecarbaldehyde in ethanol at room temperature. The resulting
modified material was dried overnight in air at 90 °C before reacting
with a solution of palladium acetate in acetone. The mixture was
magnetically strirred for 24 h, and the catalyst paste was filtered off and
washed thoroughly with acetone until the washings were colourless. The
catalyst was dried overnight in air at 90 °C to result into a brown
palladium supported catalyst. The catalyst was conditioned for a total of
27 h by refluxing in ethanol, toluene and then acetonitrile before being
tested for activity. The condition was done to remove any physisorbed
palladium. The final conditioned catalyst was filtered off and dried
overnight in air at 90 °C to be ready for use.The IR spectra of the final materials were measured on a Bruker Equinox
55 spectrometer fitted with an environmental chamber diffuse reflectance
unit. Simultaneous thermal analysis (thermal analysis coupled with
differential thermal analysis, STA) was carried out to determine the
thermal stability of the bound organics. The pore size distribution and BET
surface areas were determined by using Coulter SA2100 porosimeter with
dinitrogen as an adsorbate.
A variety of silica supported palladium catalysts were evaluated in the
Suzuki cross-coupling reactions. In a typical reaction, phenylboronic acid
(914 mg, 7.3 mmol), bromobenzene (785 mg, 5 mmol), potassium carbonate
(1381 mg, 10 mmol), dodecane (1 ml, internal standard) and the supported
catalyst (0.2 g at 0.3 mmol Pd per gram) were stirred together in
o-xylene (or m-xylene) (13 ml) at 95 °C. Samples were
withdrawn periodically and analysed by GC/GC–MS. The isolated
products were analysed by GC/GC–MS and NMR and compared with
authentic samples.
Conclusion
These new supported palladium catalysts show remarkable versatility as
they are capable of catalysing Suzuki and Heck reactions to high yields of
coupling products at lower temperatures than conventional systems in air.
Further catalyst reuse studies on other Suzuki cross-coupling reactions are
being investigated. Acknowledgements
The financial support of the Norwegian Agency for International
Development (NORAD) through the University of Dar es Salaam, Tanzania (to
E. B. M.) is gratefully acknowledged. We also thank the Royal Academy of
Engineering and EPSRC for a Clean Technology Fellowship (to J. H. C.) and
the Royal Society for a University Research Fellowship (to D. J. M.).References
-
(a) N. Miyaura, T. Yanagi and A. Suzuki, Synth. Commun., 1981, 11, 513 CrossRef CAS;
(b) N. Miyaura and A. Suzuki, Chem. Rev., 1995, 95, 2457 CrossRef CAS.
- A. Suzuki, J. Organomet. Chem., 1999, 576, 147 CrossRef CAS.
- J. H. Clark, Green Chem., 1999, 1, 1 RSC.
- G. W. Kabalka, R. M. Pagni and C. M. Hair, Org. Lett., 1999, 1, 1423 CrossRef CAS.
- G. W. Kabalka, R. M. Pagni, L. Wang, V. Namboodiri and C. M. Hair, Green. Chem., 2000, 2, 120 RSC.
- D. Gala, A. Stamford, J. Jenkins and M. Kugelman, Org. Proc. Res. Dev., 1997, 1, 163 Search PubMed.
- D. O’Keefe, M. Dannock and S. Marcuccio, Tetrahedron Lett., 1992, 33, 6679 CrossRef CAS; K.-C. Kong and C.-H. Cheng, J. Am. Chem. Soc., 1991, 113, 6313 CrossRef CAS.
- D. J. Macquarrie, Chem. Commun., 1996, 1961 RSC; D. J. Macquarrie and D. B. Jackson, Chem. Commun., 1997, 1781 RSC.
- A. Butterworth, J. H. Clark, P. H. Walton and S. J. Barlow, Chem. Commun., 1996, 1859 RSC; J. A. Elings, R. Ait-Meddour, J. H. Clark and D. J. Macquarrie, Chem. Commun., 1998, 2707 RSC.
- J. H. Clark, D. J. Macquarrie and E. B. Mubofu, Green Chem., 2000, 2, 53 RSC.
- W. M. H. Sachtler, Acc. Chem. Res., 1993, 26, 383 CrossRef CAS; D. Brunel, Coord. Chem. Rev., 1998, 178–180, 1085 CrossRef.
- M. S. Anson, M. P. Leese, L. Tonks and J. M. J. Williams, J. Chem. Soc., Dalton Trans., 1998, 3529 RSC.
- H. E. B. Lempers and R. A. Sheldon, J. Catal., 1998, 175, 62 CrossRef CAS.
- V. V. Grushin and H. Alper, Chem. Rev., 1994, 94, 1047 CrossRef CAS.
|
This journal is © The Royal Society of Chemistry 2001 |