DOI:
10.1039/B008795P
(Paper)
Green Chem., 2001,
3, 26-29
Conjugate additions of heteronucleophiles to enones and
alkynoates. A ‘benign by design’ functionalization of
heteroaromatics
Received (in Cambridge, UK) 1st November 2000
First published on 17th January 2001
Abstract
A simple and convenient functionalization of pyrrole and
thiophene nuclei has been accomplished by one-step clay-catalyzed conjugate
addition reactions of these heteroaromatics with some enones and alkynoates
under very mild conditions. The experimental protocol can be easily
modified to accommodate one or two alkyl groups.
Green ContextPyrroles and thiophenes are extremely useful compounds with
applicability in numerous areas including bioactive compounds, organic
semiconductors and addressable genechips. Thus their functionalisation is
of considerable interest though traditionally reliant on hazardous and
wasteful procedures. Here novel facile functionalisation procedures are
described. These are solvent-free, employ safe and reusable solid acids and
subject to microwave acceleration. A variety of mono- and bis-alkylated
products have been prepared using this procedure.DJM |
Introduction
In a series of recent investigations we have disclosed that
homo-Diels–Alder reactions can be conducted under solvent-free
conditions using inexpensive and innocuous mineral solids as
catalysts.1 Furans undergo competitive
cycloaddition and Michael-type reactions, and the product distribution is
largely dependent on the stoichiometry and experimental conditions.2 The incessant demand for increasingly green
technologies is encouraging scientists to think more on environmentally
friendly chemical processes.3 In this
context, important generic areas of chemistry such as acid-catalyzed
reactions need to be rethought and modified. Besides furan, the
π-excessive heteroaromatics pyrrole and thiophene react with
electrophiles via Friedel–Crafts-type reactions to give
useful precursors for natural product syntheses. However, these reactions
often require acid catalysts and/or high temperatures to be
achieved,4–6 and oligomeric
side products are also obtained. Remarkably, polypyrrole and polythiophene
films are currently being explored in applications such as biosensores,
organic semiconductors, or addressable gene-chips.7,8 It may be possible to substitute the above-mentioned
forcing conditions for solid catalysts (e.g. clays, zeolites) or
supported reagents, thereby making unnecessary the use of solvents and
separation agents.9 We now report a facile
functionalization of these important heterocycles involving their
solventless condensation with enones adsorbed on montmorillonite K10 to
afford mono- and bis-alkylated products in moderate to good overall
yields.Results and discussion
Our results with pyrrole and thiophene have been collectively summarized
in Schemes 1 and 2, and Table 1.
Condensations with N-phenylmaleimide proceed slowly, presumably
due to steric reasons. Reactions can either be stirred or irradiated with
focused microwaves, thereby resulting in shorter reaction times especially
with thiophene derivatives (Scheme 2).
These fast reaction conditions also favor the formation of monoalkylated
products, although lower yields are often obtained owing to the volatility
of the products (Table 1, entry 6). It
should be noted that irradiated reactions can equally be conducted in a
domestic oven with similar results. Nevertheless, our reactions employing a
monomode reactor (see Experimental section) exhibited increased efficiency
and reproducibility. In addition, and unlike domestic ovens, the reaction
temperature can be controlled by power modulation within a wide range of
Watts and measured with accuracy by IR detection. Furthermore, this device
offers a homogeneous distribution of the electric field inside the
cavity.10 |
| Scheme 1 | |
 |
| Scheme 2 | |
Table 1 Pyrrole and thiophene functionization reactions
Entry | X | R | R1 | Substrates (Molar ratio) | Conditions | t | Yield (%)a |
---|
Isolated yields of flash-chromatographed products. Using monomode reactor with focused microwaves (2.45 GHz, 5% relative
intensity). |
---|
1 | NH | COMe | H | 1∶2 | 0 °C | 45 min | 2 (100) |
2 | NH | COMe | H | 1∶1 | 0 °C | 1 h | 1 (15), 2 (68) |
3 | NH | COMe | H | 2∶1 | 0 °C | 1 h | 1 (30), 2 (50) |
4 | NH | –CONPhCO– | 1∶1 | 25 °C | 12 h | 1 (16) |
5 | NH | –CONPhCO– | 2∶1 | 25 °C | 48 h | 1 (30) |
6 | NH | –CONPhCO– | 1∶1 | μν (300 W)b | 1 h | 1 (16) |
7 | S | COMe | H | 1∶1 | 25 °C | 3 d | 1 (14), 2 (64) |
The reaction of pyrrole with maleic anhydride gives rise to a mixture of
6 and the dicarboxylic derivative 7, the latter arising
from ring opening of the anhydride moiety at the catalytically active acid
sites of montmorillonite, and is the exclusive product after a prolonged
reaction time (Scheme 3). The
distinctive identification between compounds 6 and 7
could be accomplished on the basis of their IR data, as 7 exhibits
characteristic bands at ca. 3000–2500 cm−1
due to OH stretching. Likewise, and unlike 6, compound 7
was insoluble in chloroform. No reaction was observed between thiophene
derivatives and N-phenylmaleimide or maleic anhydride.
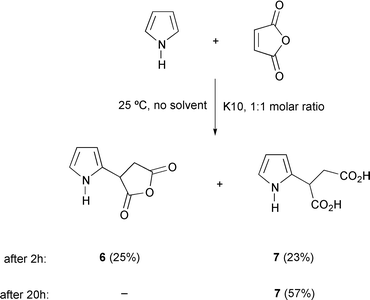 |
| Scheme 3 | |
This clay-catalyzed protocol could also be extended to alkynic
substrates such as dimethyl acetylenedicarboxylate providing an interesting
reaction mixture of double addition products (8 and 9)
accompanied by a Z/E mixture of alkenes (10, Scheme 4), which could be purified by flash
chromatography and identified by spectroscopic methods. It is well known
that simple pyrroles or thiophenes show little tendency to react as 4π
components in cycloadditions owing to their enhanced aromaticity. In fact,
Diels–Alder reaction between pyrrole and methyl vinyl ketone
catalyzed by Cr(III)-exchanged montmorillonite in organic
solvents has been observed, although the cycloadduct was obtained in 35%
yield, but no reaction could be detected for thiophene under these
Lewis-acid conditions.11 On the other hand,
conjugate addition reactions with electrophilic alkynes are accelerated by
high pressure or by Lewis-acid catalysts.4
 |
| Scheme 4 | |
Compound 9 was obtained as a single diastereomer as evidenced
by its NMR spectra which showed only one signal set. Likewise, it was
difficult to establish a spectroscopic distinction between isomers
10Z and 10E, because of the similarity
of their coupling pattern.
No alkene derivatives could be detected in the reaction between pyrrole
and methyl propiolate, but rather products 11 and 12
resulting from successive Michael-type additions (Scheme 5). The latter results are especially
attractive because they represent a facile entry to polypyrrolic fragments
and porphyrin precursors,12 which are
currently accessible via the classical reaction of pyrroles with
aldehydes13 or the self-condensation of
dipyrromethanes.14 It should also be
pointed out that compound 12, as well as the symmetrical compounds
2 (X = NH), show H-3 signals as doublets (J = 2.8 Hz) as
a consequence of a long range coupling between H-3 and the NH proton which
could further be determined by selective spin–spin decoupling. In
fact, it is well documented that the NH proton of a pyrrole or an amide is
coupled to vicinal protons, even though the NH absorption may be broad as
result of partial decoupling by the nitrogen electrical quadrupole
moment.15
 |
| Scheme 5 | |
With a few exceptions, such as 1 (X = NH, R = R1 =
CONPhCO), 8 and 9 which could be obtained as crystalline
solids and gave satisfactory combustion analyses, most samples were
homogeneous oils by TLC analysis. Finally, the mechanism associated with
these processes is a subject of an ongoing investigation, since it is
unclear if the conjugate addition follows a concerted16 or stepwise pathway,17 and a theoretical study is under way.
In conclusion, we have shown that a clay material drives conjugate
addition reactions of heteroaromatics to enones and alkynes in absence of
organic solvents. The protocol combines atom economy, efficiency and
provides access to mono- and di-alkylated products under mild conditions.
Although this procedure will require further exploration of its scope, it
is hoped that the above results will be of benefit to the scientific
community engaged in solvent-free condensations.
Experimental
All commercial reagents and solvents were used without further
purification. Montmorillonite K10 was purchased from Aldrich. Column
chromatography and TLC analyses were performed on Merck silica gel 60
(400–230 mesh) and GF254, respectively. NMR spectra were
recorded on a 400 MHz Bruker spectrometer in CDCl3.
Microwave-irradiated reactions were conducted in a
Synthewave® 402 reactor from Prolabo.Typical procedure
To commercially available montmorillonite K10 (2.5 g), cooled previously
at 0 °C, was added dropwise the corresponding heterocycle (5.0 mmol)
and methyl vinyl ketone (5.0 mmol) with vigorous stirring, and the reaction
mixture was kept at 0 °C (or 25 °C). Upon standing for an
approprite reaction time, the mixture was extracted with
CH2Cl2 (20 mL) and MeOH (20 mL), filtered, and
concentrated to give a residue, which was purified by flash chromatography
(diethyl ether–hexane or ethyl acetate–hexane eluent
systems).Reaction of pyrrole and methyl vinyl ketone
2-(3′-Oxobutyl)pyrrole:
δH(CDCl3) 8.51 (br s, NH),
6.62 (m, H-5), 6.06 (dd, J = 2.9, 2.3 Hz, H-4), 5.86 (br s, H-3),
2.83 (2H, t, J = 6.0 Hz, H-1′, H-1″), 2.76 (2H, t,
J = 6.0 Hz, H-2′, H-2″), 2.14 (3H, s, CH3),
δC(CDCl3) 209.7 (C
O), 131.4
(C-2), 116.7 (C-5), 107.8 (C-4), 105.2 (C-3), 44.1 (C-2′), 30.0
(CH3), 21.2 (C-1′). 2,3-Bis(3′-oxobutyl)pyrrole:
δH(CDCl3) 8.47 (1H, br s, NH), 5.71
(2H, d, J = 2.8 Hz, H-3, H-4). 2.73–2.81 (8H, m, H-1′,
H-1″, H-2′, H-2″), 2.16 (6H, s, 2 ×
CH3), δC(CDCl3) 209.1
(C
O), 130.4 (C-2), 104.7 (C-3), 43.9 (C-2′), 27.0
(CH3), 21.4 (C-1′).Reaction of pyrrole and N-phenylmaleimide
3-(2′-Pyrroyl)-N-phenylsuccinimide: Mp 176.5 °C.
δH(CDCl3) 9.11 (br s, NH), 7.48 (2H,
t, J = 7.2 Hz, Ar), 7.40 (1H, t, J = 7.2 Hz, Ar), 7.26
(2H, d, J = 7.2 Hz, Ar), 6.82 (br s, H-5′), 6.20
(dd, J = 3.2, 2.8 Hz, H-4′), 6.00 (m, H-3′). 4.24 (dd,
J = 9.2, 5.6 Hz, H-3), 3.35 (dd, J = 18.0, 9.2 Hz, H-4b),
3.20 (dd, J = 18.0, 5.6 Hz, H-4a).
δC(CDCl3) 176.7, 174.7, (C
O),
131.6, 129.2, 128.8, 126.4 (Ar), 125.2 (C-2′), 119.0 (C-5′),
108.5 (C-4′), 105.7 (C-3′), 38.5 (C-3), 34.2 (C-4). Anal. Calc.
for C14H12N2O2: C, 69.99; H,
5.03; N, 11.66. Found: C, 69.45; H, 4.84; N, 11.60%.Reaction of thiophene and methyl vinyl ketone
2-(3′-Oxobutyl)thiophene:
δH(CDCl3) 7.11 (dd, J = 5.2,
1.2 Hz, H-5), 6.90 (dd, J = 5.2, 3.6 Hz, H-4), 6.79 (dd,
J = 3.6, 1.2 Hz, H-3), 3.11 (2H, t, J = 7.6 Hz, H-1′,
H-1″), 2.82 (2H, t, J = 7.6 Hz, H-2′, H-2″),
2.16 (3H, s, CH3).
δC(CDCl3) 207.2 (C
O), 143.5
(C-2), 126.8 (C-5), 124.5 (C-4), 123.3 (C-3), 45.2 (C-1′), 30.0
(CH3), 23.8 (C-2′). 2,5-Bis(3′-oxobutyl)thiophene:
δH(CDCl3) 6.56 (2H, s, H-3, H-4), 3.01
(4H, t, J = 7.3 Hz, H-1′, H-1″), 2.78 (4H, t,
J = 7.3 Hz, H-2′, H-2″), 2.15 (6H, s, 2 ×
CH3). δC(CDCl3) 207.0
(C
O), 141.4 (C-2), 123.9 (C-3), 44.8 (C-1′), 29.8
(CH3), 23.8 (C-2′).Reaction of 2-methyl thiophene and methyl vinyl
ketone
The preparation of 3, 2-methyl-5-(3′-oxobutyl)thiophene,
constitutes a representative example: An equimolar mixture of
2-methylthiophene (10 mmol) and methyl vinyl ketone (10 mmol) was adsorbed
on montmorillonite K10 (5.0 g) with stirring for 5 min. The homogenized
mixture was then irradiated in a microwave reactor with focused
electromagnetic radiation (2.45 GHz, 300 W, 5% relative intensity) for 1 h.
Extraction with CH2Cl2, followed by filtration and
evaporation gave 3 as a yellowish oil in 85% yield.
δH(CDCl3) 6.53 (m, 2H, H-2, H-3), 3.01
(t, J = 7.3 Hz, 2H, CH2), 2.77 (t, J = 7.3 Hz,
2H, CH2), 2.41 (s, 3H, CH3CO), 2.12 (s, 3H,
CH3); δC(CDCl3) 207.2
(C
O), 141.2 (C-2), 137.6 (C-5), 124.6 (C-3), 124.1 (C-4), 45.1
(CH2), 29.9 (CH3CO), 23.9 (CH2),
15.1 (CH3).Reaction of 2,5-dimethyl thiophene and methyl vinyl
ketone
2,5-Dimethyl-3-(3′-oxobutyl)thiophene 4:
δH(CDCl3) 6.42 (1H, s, H-4), 2.65 (4H,
m, H-1′, H-1″, H-2′, H-2″), 2.36 [3H, s,
CH3(C-5)], 2.28 [3H, s, CH3(C-2)], 2.13 (3H, s,
COCH3). δC(CDCl3) 208.12
(C
O), 135.8 (C-5), 135.3 (C-2), 130.6 (C-3), 126.4 (C-4), 44.1
(C-1′), 30.0 [(C
O)CH3], 22.2 (C-2′), 12.7
[CH3(C-2)], 15.1 [CH3(C-5)].
2,5-Dimethyl-3,4-bis(3′-oxobutyl)thiophene 5:
δH(CDCl3) 2.67 (4H, t, J =
7.3 Hz, H-1′, H-1″), 2.54 (4H, t, J = 7.3 Hz,
H-2′, H-2″), 2.27 (6H, s, 2 × CH3), 2.16 [6H,
s, 2 × CH3(CO)].
δC(CDCl3) 207.9 (C
O), 135.2
(C-2), 129.8 (C-3), 43.8 (C-1′), 29.9 (CH3), 20.9
(C-2′), 12.9 [CH3(CO)].Reaction of pyrrole and maleic anhydride
2-(2′-Pyrroyl)succinic anhydride 6:
δH(CDCl3) 8.82 (br s, NH), 6.84 (m,
H-5′), 6.19 (dd, J = 2.9, 2.7 Hz, H-4′), 6.05 (br s,
H-3′), 4.39 (dd, J = 9.6, 7.2 Hz, H-2), 3.43 (dd, J
= 18.8, 9.6 Hz, H-3b), 3.30 (dd, J = 18.8, 7.2 Hz, H-3a).
δC(CDCl3) 171.6, 168.9 (C
O),
122.5 (C-2′), 119.6 (C-5′), 108.9 (C-4′), 106.5
(C-3′), 39.5 (C-2), 34.2 (C-3). 2-(2′-Pyrroyl)etanodioic acid
7: mp 126.5 °C.
δH(DMSO-d6) 10.72
(s, NH), 6.61 (m, H-5′), 5.90 (dd, J = 2.8, 2.4 Hz,
H-4′), 5.82 (br s, H-3′), 3.88 (dd, J = 10.4, 4.8 Hz,
H-2), 2.92 (dd, J = 17.2, 10.4 Hz, H-3b), 2.54 (dd, J =
17.2, 4.8 Hz, H-3a).
δC(DMSO-d6) 173.7,
173.0 (C
O), 128.1 (C-2′), 117.4 (C-5′), 107.5
(C-4′), 105.2 (C-3′), 40.5 (C-2), 36.5 (C-3).Reaction of pyrrole and dimethyl
acetylenedicarboxylate
Dimethyl 2,2-bis(2′-pyrroyl)butanodioate 8: mp 98 °C.
δH(CDCl3) 8.79 (2H, br s, NH), 6.71
(2H, m, H-5′), 6.12 (2H, m, H-4′), 5.92 (2H, m, H-3′),
3.78 (3H, s, CH3), 3.64 (3H, s, CH3), 3.43 (2H, s,
H-3). δC(CDCl3) 173.0, 172.0
(C
O), 130.3 (C-2′), 118.0 (C-5′), 108.1 (C-3′),
106.6 (C-4′), 53.0 (CH3), 52.0 (CH3), 48.5
(C-2), 43.1 (C-3). Anal. Calc. for
C14H16N2O4: C, 60.86; H, 5.84;
N, 10.14. Found: C, 61.16; H, 6.04; N, 9.68%. Dimethyl
2,3-bis(2′-pyrroyl)butanodioate 9: mp 216 °C.
δH(CDCl3): 8.62 (2H, br s, NH), 6.72
(2H, m, H-5′), 6.09 (2H, m, H-4′), 6.04 (2H, m, H-3′),
4.30 (2H, s, H-2), 3.56 (6H, s, 2 × CH3).
δC(CDCl3) 172.2 (C
O), 124.8
(C-2′), 118.4 (C-5′), 108.4 (C-3′), 108.2 (C-4′),
52.4 (CH3). 48.8 (C-2). Anal. Calc. for
C14H16N2O4: C, 60.86; H, 5.84;
N, 10.14. Found: C, 60.20; H, 5.66; N, 9.98%. trans-Dimethyl
2-(2′-pyrroyl)butenodioate E-10:
δH(CDCl3) 9.05 (br s, NH), 6.91 (dd,
J = 2.4, 1.2 Hz, H-5′), 6.48 (dd, J =
3.6, 2.4 Hz, H-4′), 6.25 (dd, J = 3.6, 1.2 Hz, H-3′),
6.00 (s, H-3), 3.93 (3H, s, CH3), 3.72 (3H, s, CH3).
δC(CDCl3) 168.2, 166.2 (C
O),
140.0 (C-2), 123.5 (C-3), 125.9 (C-2′), 113.7 (C-5′), 111.2
(C-3′), 108.8 (C-4′), 52.9 (CH3), 51.9
(CH3). cis-Dimethyl 2-(2′-pyrroyl)butenodioate
Z-10: δH(CDCl3) 8.78
(br s, NH), 7.05 (dd, J = 2.4, 1.2 Hz, H-5′),
6.73 (dd, J = 3.6, 2.4 Hz, H-4′), 6.30 (dd, J =
3.6, 1.2 Hz, H-3′), 5.96 (1H, s, H-3), 3.90 (3H, s, CH3),
3.80 (3H, s, CH3).
δC(CDCl3) 168.9, 168.1 (C
O),
138.7 (C-2), 125.8 (C-2′), 123.7 (C-3), 118.1 (C-5′), 110.5
(C-3′), 109.7 (C-4′), 52.8 (CH3), 52.1
(CH3).Reaction of pyrrole and methyl propiolate
Methyl 3,3-bis(2′-pyrroyl)propanoate 11:
δH(CDCl3) 8.24 (2H, br s, NH), 6.67
(2H, m, H-5′), 6.13 (2H, m, H-4′), 5.98 (2H, m, H-3′),
4.58 (1H, t, J = 7.2 Hz, H-3), 3.67 (3H, s, CH3), 3.01
(2H, d, J = 7.2 Hz, H-2).
δC(CDCl3) 173.1 (C
O), 131.7
(C-2′), 117.2 (C-5′), 108.1 (C-4′), 105.3 (C-3′),
51.9 (CH3), 40.0 (C-2), 33.8 (C-3).
2,5-Bis(1′-(2-pyrroyl)-2′-carbomethoxyethyl)pyrrole
12: δH(CDCl3) 8.27, 8.19 (3H,
m, NH), 6.64 (2H, m, H-5″), 6.08 (2H, m, H-4″), 5.91 (2H, br s,
H-3″), 5.85 (2H, d, J = 2.8 Hz, H-3), 4.48 (2H, t,
J = 7.2 Hz, H-1′), 3.64 (6H, s, 2 × CH3),
2.92 (4H, d, J = 7.2 Hz, H-2′).
δC(CDCl3) 173.1 (C
O), 132.1
(C-2), 131.7 (C-2″), 117.2 (C-5″), 108.2 (C-4″), 105.4
(C-3), 105.3 (C-3″), 51.9 (CH3), 40.0 (C-2′), 33.7
(C-1′). Acknowledgements
Financial support from the Spanish Ministry of Science and Technology
(DGICYT, PB98-0997 and AMB99-0591) and the Junta de Extremadura-Fondo
Social Europeo (IPR98-A065) is gratefully acknowledged.References
- M. Avalos, R. Babiano, J. L. Bravo, P. Cintas, J. L. Jiménez, J. C. Palacios and B. C. Ranu, Tetrahedron Lett., 1998, 39, 2013 CrossRef CAS.
- M. Avalos, R. Babiano, J. L. Bravo, P. Cintas, J. L. Jiménez and J. C. Palacios, Tetrahedron Lett., 1998, 39, 9301 CrossRef CAS.
- P. T. Anastas and
J. C. Warner,
Green Chemistry: Theory and Practice, Oxford
University Press, Oxford, 1998 Search PubMed; P. T. Anastas and
T. C. Williamson,
Green Chemistry: Frontiers in Benign Chemical Synthesis and
Processes, Oxford University Press,
Oxford, 1998. Search PubMed.
- J. A. Joule,
K. Mills and
G. F. Smith,
Heterocyclic Chemistry, Chapman & Hall,
London, 1995, pp.
231–242. Search PubMed.
- K. Hayakawa, M. Yodo, S. Ohsuki and K. Kanematsu, J. Am. Chem. Soc., 1984, 106, 6735 CrossRef CAS.
- Y. Kamitori, M. Hojo, R. Masuda, T. Izumi and S. Tsukamoto, J. Org. Chem., 1984, 49, 4161 CrossRef CAS.
- V. R. Shastri and
M. V. Pishko, in
Electrical and Optical Polymer Systems, ed. D. L. Wise, G.
E. Wnek, D. J. Trantolo, T. M. Cooper and J. D. Gresser, Marcel
Dekker, New York,
1998. Search PubMed.
- J. Roncali, Chem. Rev., 1992, 92, 711 CrossRef CAS; G. Schopf and
G. Kossmehl,
Polythiophenes—Electrically Conductive Polymers,
Springer, Berlin, 1997
R. D. McCullough, Adv. Mater., 1998, 10, 93. Search PubMed.
- J. H. Clark and
C. N. Rhodes,
Clean Synthesis Using Porous Inorganic Solid Catalysts and Supported
Reagents, Royal Society of Chemistry,
Cambridge, 2000. Search PubMed.
- A. Loupy, A. Petit, J. Hamelin, F. Texier-Boullet, P. Jacqualt and D. Mathé, Synthesis, 1998, 1213 CrossRef CAS.
- J. M. Adams, S. Dyer, K. Martin, W. A. Matear and R. W. McCabe, J. Chem. Soc., Perkin Trans. 1, 1994, 761 RSC.
- J. L. Sessler and
S. J. Weghorn,
Expanded, Contracted and Isomeric Porphyrins,
Elsevier, Oxford,
1997. Search PubMed.
- M. Onaka, T. Shinoda, Y. Izumi and E. Nolen, Tetrahedron Lett., 1993, 34, 2625 CrossRef CAS.
- C. Pichon and A. I. Scott, Tetrahedron Lett., 1994, 35, 4497 CrossRef CAS.
- R. M. Silverstein,
G. C. Bassler and
T. C. Morrill,
Spectrometric Identification of Organic Compounds,
Wiley, New York, 1974,
p. 192. Search PubMed.
- D. Niu and K. Zhao, J. Am. Chem. Soc., 1999, 121, 2456 CrossRef CAS.
- L. R. Domingo, M. T. Picher and R. J. Zaragoza, J. Org. Chem., 1998, 63, 9183 CrossRef CAS.
|
This journal is © The Royal Society of Chemistry 2001 |
Click here to see how this site uses Cookies. View our privacy policy here.