DOI:
10.1039/B006482N
(Paper)
Analyst, 2001,
126, 66-70
Immunodetection of lactosylated proteins as a useful
tool to determine heat treatment in milk samples
Received 8th August 2000, Accepted 19th October 2000
First published on 1st December 2000
Abstract
This paper reports the optimisation of a competitive
immunoassay (ELISA) to detect lactosylated proteins in milk samples. The
assay employs monoclonal antibodies for lactosylated proteins produced in
our laboratory and requires no pre-treatment of the samples other than a
dilution step. Monoclonal antibodies were fully characterised in terms of
selectivity and cross-reactivity with structurally related molecules and
used in a competitive assay format with lactosylated standard proteins
(lactosylated ovalbumin). The detection limit for lactosylated ovalbumin
was 0.015 μg ml−1 and the working range was from 0.010
to 40 μg ml−1. The data obtained indicate that the
ELISA developed is applicable to diluted milk samples and is able to
distinguish between milk samples that have undergone different heat
treatments (UHT and pasteurised milk).
Introduction
Heat treatment of milk samples during pasteurisation processes leads to
the non enzymatic lactosylation of free amino groups of proteins due to the
Maillard reaction.1 The addition of lactose
to lysine ε-amino groups forms
N-substituted-1-amino-deoxy-2-ketoses, known as Amadori compounds,
subsequently leading to unavailable amino acid sugar reaction
products.2,3 The blocked lysine will
not be susceptible to further enzyme attack, becoming nutritionally
unavailable. Since lysine is an essential amino acid, it is not synthesised
by humans, and it must be present in the diet. Hence, the main consequence
of these heating processes is the modification of the nutritional values of
milk.The two main heat induced markers used as indicators of the severity of
heat treatment are furosine and lactulose. Furosine, namely
ε-N-(-2-furoylmethyl)-L-lysine, is an amino acid
obtained by acid hydrolysis of glycosylated proteins4 and is mainly detected by reversed-phase
HPLC5 or ion-exchange chromatography,6 although lately capillary electrophoresis has also
been reported.7 Hydrolysis of
lactulosyllysine, the lysine–lactose adduct formed in MR, yields
three different products, lysine (∼50%), furosine (∼30–40%)
and pyridosine (∼10–20%), but the conversion factor for the
calculation of the content of the Amadori product is uncertain since the
proportions of the hydrolysis products are variable. Microwave hydrolysis
of proteins has been applied to furosine determination, shortening the time
required for the whole procedure.8 The
furosine method has the advantage of being sensitive at very low levels of
blocked lysine, but the overall procedure, consisting of 24 h hydrolysis
and chromatographic determination, is cumbersome and time consuming,
requiring expensive equipment and skilled operators.
Lactulose (4-O-ß-galactopyranosyl-D-fructofuranose) is
formed by isomerisation of lactose
(4-O-ß-galactopyranosyl-D-glucopyranose) during the heat
treatment of milk, and has been proposed by the International Dairy
Federation (IDF)9 and by the European
Commission (EC)10 as an analytical index to
distinguish UHT milk from sterilised milk. The detection of lactulose is
mainly based on gas chromatography,11
liquid chromatography,9 spectrophotometric
methods12,13 and enzymatic methods
with either spectrophotometric14,15
or amperometric detection.16,17 Our
group has developed several methods for the determination of lactulose in
milk samples, aimed at producing much simpler and shorter protocols that do
not require expensive equipment.18–20
Immunological methods for the measurement of the extent of protein
lactosylation have been developed based on the well known antigenic
properties of lactose.21 Several groups
have reported the production of polyclonal antibodies for lactosylated
proteins21–27 using different immunogens such as lactosylated
proteins and peptides, but to date no-one has been able to set up a simple
ELISA procedure to distinguish among different classes of milk. All of the
immunoassays proposed so far require long pre-treatment of the samples,
such as protein precipitation and further purification.
The aim of this paper is to report the characterisation of monoclonal
antibodies for lactosylated proteins and their use in an immunoassay
[enzyme-linked immunosorbent assay (ELISA)] for the detection of
lactosylated proteins in milk samples. Two commercially available classes
of milk (UHT and pasteurised) treated with different heating procedures
were tested in order to assess the applicability of the monoclonal
antibodies. This assay does not need any pre-treatment of the milk samples
other than a dilution step and seems suitable for the measurement of the
extent of protein lactosylation in milk.
Experimental
Reagents and materials
All proteins, carbohydrates, chemicals for buffers and gels were
obtained from Sigma (St Louis, MO, USA). Anti-mouse HRP conjugated Ab was
purchased from Bio-Rad Laboratories (Richmond, CA, USA). ELISA plates were
Maxisorp from Nunc (Roslklde, Denmark). Protein A for antibody purification
was Protein A Sepharose 4 Fast Flow (Amersham Pharmacia Biotech, Uppsala,
Sweden). Culture media for hybridoma cells was GIBCO BRL (Life
Technologies, UK) and HAT medium was obtained from Flow Laboratories
(USA).Skimmed milk was purchased directly in shops; all the brands tested are
commercially available in Rome, Italy.
Apparatus
A Model 550 microplate reader (Bio-Rad) was used to read the absorbance
on ELISA plates at 490 nm. A model 238 Uvicord S II instrument from LKB
(Bromma, Sweden) at 280 nm was used for the detection of proteins during
the collection of antibodies in the purification process.Protein lactosylation
Lactosylated proteins were prepared according to the procedure described
by Matsuda et al.21,22 with some
modifications. Ovalbumin (Ova), casein (Cas), ß-lactoglobulin (LG)
and hemocyanin keyhole limpet (KLH) were lactosylated and used as standards
for the antiserum characterisation and milk tests and for animal
immunisation. The same procedure was used for lactosylation and the
modified protein was injected into the animals as antigen for the
production of antibodies. The proteins were dissolved in distilled water
(2% w/v) and mixed with D-lactose (30% of the protein dry
weight). The protein–sugar solutions were adjusted to pH 8.0 with
dilute NaOH and freeze-dried. The dried samples were kept at 50 °C and
65% relative humidity using a saturated KI solution for 72 h, and subjected
to gel filtration on a Sephadex G-50 column to eliminate any unreacted
lactose. The protein concentration was determined
spectrophotometrically.28 Lactosylated and
native proteins were both run on sodium dodecyl sulfate polyacrylamide
gel,29 as a control of the lactosylation
process, by comparing sample mobilities.Immunisation procedures
Ten mice (female Balb/c) were immunised by intraperitoneal injection of
lactosylated KLH (0.1 mg ml−1, 1 ml per mouse) emulsified
with complete Freund’s adjuvant (CFA, Sigma). Injections were
repeated several times every 4 weeks with phosphate-buffered saline
(PBS)–lactosylated KLH (1 mg ml−1). Blood was
collected and centrifuged and serum was stored at −20 °C until
use.Production of monoclonal antibodies (mAb)
Somatic hybridisation was performed by incubating for 1 min at 37 °C
108 immune spleen cells with 107 mouse myeloma cells
(NSO) in presence of 0.5 ml of 15% v/v dimethyl sulfoxide and 41% w/v
polyethylene glycol (PEG). A 0.5 ml volume of 25% w/v PEG was then added.
Three minutes later, fused cells were suspended slowly in 48 ml of complete
culture medium (CCM) supplemented with 20% fetal bovine serum (FBS),
(GIBCO) and plated on to 96-well tissue culture plates (0.2 ml per well).
HAT medium (0.15 ml per well) was added on days 2 and 8, after removing
0.15 ml of spent medium. Cells from positive wells were cloned twice to 0.1
and 0.3 cells per well by limiting dilution in 96-well plates screened for
the presence of antibody able to bind L-Ova or L-LG
by direct ELISA (see later). Selected clones were grown in 15 ml flasks and
the supernatants were used as the source of mAbs.Antibody purification
Antibodies were purified by affinity chromatography on a 10 ml protein A
column (Protein A Sepharose 4 Fast Flow, 5 ml gel), the whole system
consisting of the chromatographic column attached to a UV reader
(λ = 280 nm) and a chart recorder. The column was subjected
to sequential pre-washing with the following solutions: PBS (pH 7.4), 0.7%
NaCl, 1 M NH4SO4 (pH 9), 3.5 M MgCl2 and 1
M NH4SO4 (pH 9). The hybridoma supernatant diluted in
NH4SO4 (1 ml of supernatant in 9 ml of
NH4SO4) was allowed to run overnight with a
continuous flow system. The next day the antibodies were eluted with
MgCl2 and the protein fraction was collected. Antibodies were
dialysed in de-ionised water overnight and their titre was
determined28 spectrophotometrically.Antibody characterisation
The binding specificity of the sugar-specific antibody for the
lactosylated proteins was measured by competitive and non-competitive
ELISA.30 Both polyclonal and monoclonal
antibody screening were performed by coating the plates with all of the
lactosylated protein standards (1 μg ml−1 in 50 mmol
l−1 carbonate buffer (pH 9) overnight at 4 °C (100
μl per well)). The plate was washed three times with PBS, blocked for 1
h at 37 °C with 0.5% gelatine in PBS (200 μl per well) and then
washed three times with PBS containing 0.05% Tween 20 (polyoxyethylene
sorbitan monolaureate) (Sigma) (PBST).In direct ELISA, serial dilutions of serum or hybridoma cell-culture
supernatant or purified antibodies (100 μl per well) were incubated at 4
°C for 2 h. The wells were then washed three times (PBST), and further
incubated for 1 h at 4 °C with 0.1 ml of horseradish
peroxidase-conjugated goat anti-mouse IgG (Bio-Rad). After washing (PBS),
0.1 ml per well of o-phenylenediamine in 0.1 M
citrate–phosphate buffer (pH 4.5) containing 1 mM
H2O2 was added (20 min at room temperature). The
enzymatic reaction was stopped with 2 M HCl and the absorbance was read on
an ELISA plate reader at 495 nm.
Competition curves were obtained mixing 50 μl of sample (as stated)
with 50 μl of antiserum or hybridoma cell-culture supernatant or
purified antibodies at the appropriate dilution in the wells and incubated
for 1 h at 4 °C. The plates were then processed exactly as before.
Western immunoblot31 on lactosylated and
native proteins with purified antibodies was carried out to confirm
antibody specificity.
Competitive ELISA in milk samples
Pasteurised and UHT milk samples were tested in competitive ELISA and
the total milk protein concentration was determined
spectrophotometrically.28 Serial milk
dilutions from 1∶500 (∼60 μg ml−1,
considering that the average protein concentration is 3.1%) to
1∶500000 (∼0.06 μg ml−1) in PBS were
incubated with the appropriate mAb dilution (1 μg ml−1)
and added to the plate (incubation for 1 h at 4 °C). The ELISA plates
were then processed as before. The data obtained from each competition
curve were plotted and fitted using SigmaPlot software (SPSS) and a
regression analysis on the linear portion of the sigmoidal curves was
performed. The slopes obtained for the regression analysis were used as an
index of protein lactosylation.Each experiment was performed in triplicate and the mean of each value
was used for curve fitting.
Lactulose determination in milk samples
Lactulose was determined according to the procedure described by Amine
et al.20 using a
spectrophotometric assay.Results and discussion
Protein lactosylation
Lactosylated and native proteins were subjected to 15%
SDS–polyacrylamide gel electrophoresis (SDS-PAGE) to compare the
difference in mobility and to evaluate the molecular weight of lactosylated
samples. As expected, lactosylated proteins had a lower mobility than the
native proteins because of the higher molecular weight. The molecular
weights of Ova and L-Ova were calculated to be 45000 and 48000,
respectively, LG and L-LG 25000 and 30000, respectively, and Cas and
L-Cas 58000 and 62000, respectively; these are comparable to the
molecular weights reported by Matsuda et al.21Characterisation of the antibodies
Selectivity for lactosylated proteins.. Polyclonal (pAb) and monoclonal antibody (mAb) selectivity for
lactosylated proteins was tested in direct ELISA, using L-Ova,
L-Cas and L-LG as antigens. Both pAbs and mAbs
exhibited high selectivity for the lactosylated standard proteins as shown
in Fig. 1(a) and 1(b). The antibodies recognise all the lactosylated
proteins with different affinity; this can be explained by the difference
in the number and position of the epitopes (lactosylation sites) for each
of the proteic molecules. The results clearly indicate that the antibodies
are specific for the lactose–lysine adduct present on each of these
proteins, regardless of the structure of the protein itself. Western
immunoblot on both lactosylated and native proteins confirmed these data
(results not shown).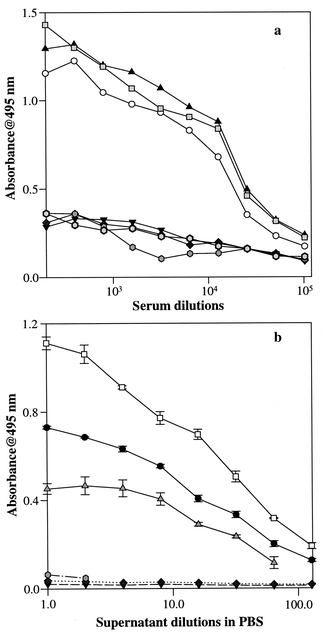 |
| Fig. 1 (a) Polyclonal antibody selectivity for lactosylated vs. native
proteins: L-Cas (○); L-LG ( );
L-Ova (▲); Cas (▼); LG (◆); Ova ( );
no coating (♦). (b) Monoclonal antibody selectivity for lactosylated
vs. native proteins: L-Cas (●); L-LG
(□); L-Ova ( ); Cas (▼); LG (♦); Ova
( ). | |
As expected, mAb and pAb present different responses to lactosylated
proteins; this is more evident by the competition curves reported in
Fig. 2. The main difference that should be
pointed out is in selectivity (Fig. 1) and
sensitivity (Fig. 2; different working
ranges for mAb and pAb). The positive hybridoma clone (mAb) was selected
for the assay because it gives major advantages for the standardisation of
the procedure. In fact, the analyst is able to operate with a reagent
(mAbs) exhibiting constant characteristics in terms of selectivity,
affinity and cross-reactivity with any structure related molecule.
Moreover, no further immunisation (or sacrifice) is needed, nor
re-optimisation of the assay conditions owing to the large variability in
the immunogenic responses of different animals.
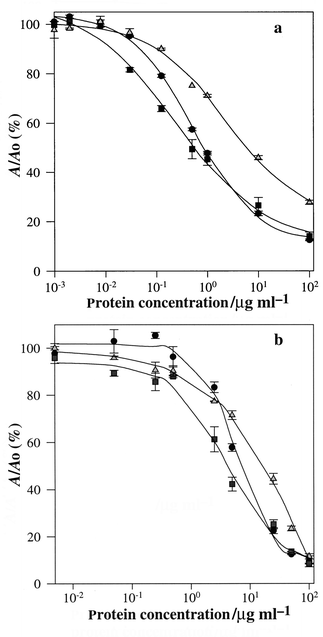 |
| Fig. 2 (a) Polyclonal antibody competition curves with lactosylated proteins
standards: L-Ova (●); L-Cas ( );
L-LG ( ). (b) Monoclonal antibody competition curves with
lactosylated proteins: L-Ova (●); L-Cas
( ); L-LG ( ). | |
The affinity constants for mAbs were determined from the competition
curves with lactosylated standards by the method of Friguet et
al.32 [Fig.
2(b)]. The values obtained were 10−8–5
× 10−9 for L-Ova, 5 ×
10−8–8 × 10−8 for
L-Cas and 10−8–5 ×
10−8 for L-LG.
The working range and the detection limit for L-Ova were also determined
from the competition curve in Fig. 2(b). The
detection limit, defined as the concentration of lactosylated standard
protein equivalent to three standard deviations at Ao (no
competition) was 0.015 μg l−1. The working range for
L-Ova, defined as the standard protein concentration range
between 90 and 10% of the maximum signal (A0)33 was 0.010–40 μg ml−1.
Since mAbs had the lowest affinity for L-Ova, the latter was
selected for all further experimental work. In fact, in a competitive assay
format with the antigen competing for a limiting amount of mAbs, the use of
a L-OVA is expected to increase the sensitivity of the assay; the majority
of milk proteins is composed of casein. The same analytical behaviour was
observed using a 1∶8 dilution of supernatant in PBS or 1 μg
ml−1 of purified mAbs.
Cross-reactivity with glycosylated-OVA adducts and
carbohydrates.. The cross-reactivity of mAb with some structurally related glycosylated
proteins and carbohydrates was investigated using lactosylated ovalbumin as
a protein standard. Lactose was tested because is the main source of sugars
in milk (∼5% w/w) and because it has been reported to be the major
interfering compound in previously developed immunoassays for lactosylated
proteins.21,25–27
Glucose and galactose as the product of lactose hydrolysis and lactulose
which is formed during milk heat treatment, although at low concentration
compared with lactose (below 50 ppm in pasteurised milk and ∼300 ppm in
UHT), were also tested. Other cross-reactivity tests were run with the
glycated adducts of Ova with glucose, galactose and lactulose prepared as
described in the Experimental section.Cross-reactivity (CR%) was expressed as the ratio of the concentration
of free L-Ova that binds to 50% of mAb used for the competition
(x) to the concentration of the competitor that binds the same
amount of antibody (y) (CR% = x/y).34 The results in Table
1 show that none of these molecules is able to interfere
significantly with the detection of lactosylated proteins. Galactose and
the galactose–ovalbumin adduct exhibited the highest
cross-reactivity, suggesting that the galactose residue is probably
recognised by the antibodies. Their effect is negligible considering both
their CR% (15 and 10%, respectively) and the very low concentration of
galactose in milk. The latter consideration applies also to lactulose. The
selectivity of the mAbs versus lactose was very high. However,
considering the concentration in milk samples, it was calculated that a
minimum dilution of 1∶100 was required in order to have a negligible
effect on the assay.
Table 1 Monoclonal antibody supernatant cross-reactivity (CR%) with glycosylated
proteins and free carbohydrates. CR% = x/y
× 100;
x = μg ml−1L-Ova that corresponds
to 50% A/A0, y = μg
ml−1 competitor that correspond to 50%
A/A0
Competitor | y/μg ml−1 | CR% |
---|
L-Ova | 0.88 | 100.0 |
Ovalbumin–glucose | 174.5 | 0.5 |
Ovalbumin–galactose | 8.64 | 10.2 |
Ovalbumin–lactulose | 1534 | <0.1 |
Glucose | 180.2 | 0.5 |
Galactose | 5.9 | 15 |
Lactose | 44 | 2 |
Lactulose | 17.2 | 5 |
Measurement in milk samples
Preliminary experiments performed on milk samples kept at 37 °C for
up to 4 d demonstrated that it was possible to follow the increase in the
extent of lactosylation by a direct ELISA using polyclonal
antibodies.35 A higher absorbance value was
registered for the heated milk samples during a 4 d period of time
versus the same samples kept at 4 °C.The purpose of this work was to produce antibodies and optimise an ELISA
competitive assay able to work in milk samples with no pre-treatment of the
sample other than dilution. The extent of lactosylation was evaluated by
running the competitive assay for different dilutions of milk and plotting
the absorbance against the total amount of proteins of the sample
calculated as reported in the Experimental section.28 The total milk protein concentration range
chosen for ELISA was from ∼120 to ∼0.06 μg ml−1
(corresponding to a dilution range of 1∶250 to 1∶500000).
Typical competition curves for pasteurised and UHT milk are shown in
Fig. 3.
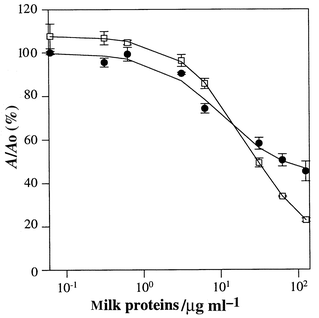 |
| Fig. 3 Competitive ELISA for pasteurised milk (●) and UHT milk
(□) samples. | |
Our main goal was to determine whether this immunoassay was able to
distinguish between commercial classes of milk. Five pasteurised and five
UHT commercial milk brands were analysed for this purpose. The increase in
the severity of heat treatment in milk pasteurisation processes enhances
the number of epitopes (lactosylation sites) on the milk protein. This
influences the shape of the ELISA competition curves, the two main
differences being the difference in the absorbance between the highest and
lowest competition values and the slope of the linear portion of the
sigmoidal curves. As expected, UHT milk presents a more negative slope
because of the larger amount of lactosylated protein (see Fig. 3). The difference in absorbance is larger
compared with fresh milk samples for the same reason, the greater amount of
antigen able to bind a larger amount of mAb used in the assay.
The ELISA lactosylation index chosen to compare the results obtained for
all of the samples was the slope of the competition curves between 60 and 3
μg ml−1 total protein concentration (corresponding to
1∶500 to 1∶10000 dilutions, respectively). Four different
dilutions in triplicate were considered for the regression analysis and
only r2
≥ 0.990 was considered acceptable. The
intra- and inter-day reproducibility of the slope were evaluated on sample
No. 8 (six replicates for five days); The RSD was 3% intra-day and 8%
inter-day. Data obtained for milk samples are reported in Table 2.
Table 2 Lactosylated protein and lactulose assay in pasteurised and UHT milk
samples. The slope of the regression analysis of the ELISA assay (sample
dilution from 1∶500 to 1∶10000) is reported for lactosylated
proteins
Pasteurised milk sample No. | Slope | Lactulose/ mg l−1 | UHT milk sample No. | Slope | Lactulose/ mg l−1 |
---|
1 | −0.21 | nd | 6 | −0.35 | 236 |
2 | −0.21 | nd | 7 | −0.43 | 321 |
3 | −0.19 | nd | 8 | −0.33 | 220 |
4 | −0.21 | nd | 9 | −0.34 | 215 |
5 | −0.23 | nd | 10 | −0.48 | 352 |
The lactulose content of the samples was also measured with a
spectrophotometric procedure. Lactulose was not detectable in pasteurised
milk samples since this method has a detection limit of 10 mg
l−1.20 The results
obtained for UHT milk show the same trend as for the lactosylated proteins:
the higher the amount of lactulose, the more negative is the slope of the
ELISA. To prove that the difference between the two classes of milk
reported in Table 2 was significant, a
t-test on the sample means was performed. The probability that
this difference is relevant was > 99% at the 95% confidence level,
meaning that this difference is statistically relevant.
Conclusions
Monoclonal antibodies for lactosylated proteins were produced,
characterised and used in a competitive assay format. The data obtained
indicate that the ELISA developed is applicable to diluted milk samples and
is able to distinguish between milk samples that have undergone different
heat treatments (UHT and pasteurised milk). Acknowledgement
This work was supported by the EU, project EU-FAIR CT 96-1095.References
- M. A. J. S. van Boekel, Food Chem., 1999, 62, 403 CrossRef.
- J. O’Brien and P. A. Morrisey, Crit. Rev. Food Sci. Nutr., 1989, 28, 5 Search PubMed.
- J. Mauron, Prog. Food Nutr. Sci., 1981, 5, 5 Search PubMed.
- P. A. Finot, R. Deutsch and E. Bujard, Prog. Food Nutr. Sci., 1981, 5, 345 Search PubMed.
- P. Resmini, L. Pellegrino and G. Battelli, Ital. J. Food Sci., 1990, 3, 173.
- J. Hartkporf and H. F. Ebersdobler, J. Chromatogr., 1993, 635, 151 CrossRef.
- A. Tirelli and L. Pellegrino, Ital. J. Food Sci., 1995, 7, 379 CAS.
- R. Acquistucci, G. Panfili and E. Marconi, J. Agric. Food Chem., 1996, 44, 3855 CrossRef CAS.
- IDF, Standard 147, International Dairy Federation,
Brussels, 1991..
- EC Commision, Dairy Chemists’ Group-Doc VI/5276,
European Commission, Brussels, 1992..
- A. Olano, M. M. Calvo and V. Corzo, Food Chem., 1989, 31, 259 CrossRef CAS.
- A. K. Adhikari, D. Sahai and O. N. Mathur, Lait, 1991, 71, 555 Search PubMed.
- F. W. Parish, K. Hicks and L. Donner, J. Dairy Sci., 1980, 63, 1809 Search PubMed.
- H. Geier and H. Klostermeyer, Z. Lebensm.-Unters. Forsch., 1980, 171, 443 Search PubMed.
- G. R. Andrews, J. Soc. Dairy Technol., 1984, 37, 92 Search PubMed.
- M. Mayer, M. Genrich, W. Kunnecke and U. Bilitewski, Anal. Chim. Acta, 1996, 324, 37 CrossRef CAS.
- Y. Sekine and E. A. H. Hall, Biosens. Bioelectron., 1998, 13, 995 CrossRef CAS.
- D. Moscone, R. A. Bernardo, E. Marconi, A. Amine and G. Palleschi, Analyst, 1999, 124, 325 RSC.
- A. Amine, D. Moscone and G. Palleschi, Anal. Lett., 2000, 33(1), 125.
- A. Amine, D. Moscone, R. A Bernardo, E. Marconi and G. Palleschi, Anal. Chim. Acta, 2000, 406, 217 CrossRef CAS.
- T. Matsuda, Y. Kato, K. Watanabe and R. Nakamura, J. Food Sci., 1985, 50, 618 Search PubMed.
- T. Matsuda, Y. Kato, K. Watanabe and R. Nakamura, J. Agric. Food Chem., 1985, 33, 1193 CrossRef CAS.
- T. Matsuda, Y. Kato, K. Watanabe and R. Nakamura, Mol. Immunol., 1987, 24, 421 CrossRef CAS.
- T. Matsuda, Y. Kato and R. Nakamura, J. Agric. Food Sci., 1991, 39, 1201 CrossRef CAS.
- T. Matsuda, H. Ishiguro, I. Ohkubo and R. Nakamura, J. Biochem., 1992, 111, 383 Search PubMed.
- V. Fogliano, S. M. Monti, A. Ritieni, C. Marchisano, G. Peluso and G. Randazzo, Food Chem., 1997, 58, 53 CrossRef CAS.
- R. Pizzano, M. A. Nicolai, R. Siciliano and F. Addeo, J. Agric. Food Sci., 1998, 46, 5373 CrossRef CAS.
- O. H. Lowry, N. J. Rosebrough, A. L. Farr and R. J. Randall, J. Biochem., 1951, 193, 265 Search PubMed.
- U. K. Laemmli, Nature (London), 1979, 227, 680.
- E. Engval and P. Perhann, Immunochemistry, 1971, 8, 871 Search PubMed.
- H. Towbin, T. Staehelin and J. Gordon, Proc. Natl. Acad. Sci. USA, 1974, 76, 4350.
- B. Friguet, A. F. Chaffotte, L. Djavadi-Ohaniance and M. J. Goldberg, J. Immunol. Methods, 1985, 77, 305 CrossRef CAS.
- G. Giraudi,
I. Rosso,
C. Baggiani,
C. Giovannoli,
A. Vanni and
G. Grassi,
Anal. Chim. Acta, 1999,
392, 85, and references cited
therein. Search PubMed.
- M. Pallini,
D. Compagnone,
S. Marini and
G. Palleschi, in
Proceedings of the 4th Italian Conference on Sensors and
Microsystems, ed. C. Di Natale, A D’Amico and F. Davide,
World Scientific, Singapore,
2000, pp. 63–68. Search PubMed.
- G. E. Abraham, J. Clin. Endocrinol. Metab., 1696, 29, 866 Search PubMed.
|
This journal is © The Royal Society of Chemistry 2001 |