DOI:
10.1039/B005847P
(Paper)
Analyst, 2001,
126, 62-65
Electrochemical detection of sequence-specific DNA
using a DNA probe labeled with aminoferrocene and chitosan modified
electrode immobilized with ssDNA
Received 19th July 2000, Accepted 6th November 2000
First published on 18th December 2000
Abstract
The electrochemical detection of sequence-specific DNA using a
DNA probe labeled with aminoferrocene (AFC) is reported. Sample ssDNA was
immobilized on a chitosan modified glassy carbon electrode. A
sequence-known DNA with 256 bp [obtained by polymerase chain reaction
(PCR)] was successfully labeled with the electro-active reagent AFC by
1-ethyl-3-(3-dimethylaminopropyl) carbodiimide for the first time. This DNA
probe labeled with AFC was applied to hybridize with a sequence-unknown DNA
sample. Only the complementary sequence (cDNA) could form a double-stranded
DNA (dsDNA) with the DNA probe labeled with AFC. The anodic peak currents
(ipa) of the AFC bound to the dsDNA by differential
pulse voltammetry were used for the determination of cDNA. The
ipa of AFC was linearly related to the concentration of
cDNA sequence between 1.0 × 10−8 and 6.0 ×
10−6 mol L−1. The detection limit was 2.0
× 10−9 mol L−1 using 3σ
(where σ is the standard deviation of blank solution, n =
11). The probe showed high sensitivity and selectivity.
Introduction
DNA hybridization biosensors have been a very popular topic during the
past several years and they hold an enormous promise for the clinical
diagnosis of inherited diseases and the rapid detection of infectious
microorganisms. Various techniques of biotin,1 fluorescent dye,2
chemiluminophore,3 surface plasmon resonance sensor4 and electrochemical DNA probe,5–10 aiming at detecting the base
pair hybridization between a labeled probe and a target DNA, have been
developed. Since electrochemical techniques can offer the advantages of
being cheap, sensitive and rapid, many electrochemical DNA biosensors for
sequence-specific DNA detection have been reported.11–26 For example, Wang’s
group13 employed DNA probes for the detection of point mutations
in the p53 gene. The same group developed an electrochemical biosensor for
detecting the Mycobacterium tuberculosis DNA in connection to a
Co(phen)3 indicator.14 Hashimoto
et al.15,16 reported a DNA
electrochemical sensor based on an intercalator as a hybridization
indicator. Millan and Mikkelsen17,18
described a sequence-selective biosensor using a DNA-modified glassy carbon
electrode and Co(pby)33+ as electroactive
hybridization indicator. Ihara et al.19 employed ferrocene-mediated oligonucleotides for
a sandwich-based electrochemical detection of DNA hybridization.
Takenaka’s team20 reported on a
naphthalene–ferrocene redox indicator with a remarkable
discrimination between the probe and duplex. Our group has also reported
several electrochemical DNA biosensors in specific DNA sequence
detection21–24 and
damage and protection of DNA.25,26In this paper, aminoferrocene (AFC) was labeled with a sequence known
DNA with 256 bp (obtained by the polymerase chain reaction (PCR), marked as
PCR DNA) by 1-ethyl-3-(3-dimethylaminopropyl) carbodiimide (EDC) for the
sequence-specific DNA detection. The use of AFC was one of the novelties of
this work. Its advantages lie in that first, it avoids the use of toxic
anticarcinogens, such as daunomycin25 and
ethidium bromide21etc. Secondly,
the adsorption of the AFC labeled probe on the electrode is weak, so the
interference caused by the common intercalators which are used as
hybridization indicator15–18 could be eliminated. Thirdly, the labelling
procedure of this method is simpler than that of the previous published
work;23 AFC was directly labeled on the
5′ end of PCR DNA without using ethylenediamine as a linking reagent.
The other novelty was that the chitosan oligomer film was for the first
time used as an active coating for the immobilization of ssDNA at a glassy
carbon electrode. Chitosan oligomer is a kind of β-1,4-linked
glucosamine oligomer;27 it is a natural
cationic polymer and can form a stable complex with the polyanionic
phosphodiester backbones of DNA, either native or denatured.28 The main advantages of using chitosan were that
chitosan could form a tight complex with DNA which made the immobilization
very stable. Compared with the DNA immobilization methods using
self-assembly monolayer (SAM) and biotin, the use of chitosan for DNA
immobilization did not need the mercapto–DNA11 and biotin–DNA,1 which could greatly reduce the detection cost.
Further study of the electrochemical characterization of the chitosan
modified electrode for ssDNA immobilization is proceeding.
Experimental
Chemicals and solutions
The sequence-known DNA with 256 bp (obtained by the polymerase chain
reaction (PCR), A260/A280 = 1.96,
marked as PCR DNA) and DNA plasmid pNC3 (about 4000 bp,
containing a sequence complementary to the PCR DNA,
A260/A280 = 1.63; no further
purification) were obtained from the Molecular Biology Laboratory, Biology
Department, East China Normal University (Shanghai, China). Calf-thymus DNA
was purchased from Baitai Biochemical Technology Company (Beijing, China),
Sperm DNA and yeast RNA was purchased from Shanghai Institute of
Biochemistry (Chinese Academy of Sciences, Shanghai, China), λDNA
and pBR322 DNA were purchased from Huamei Biotechnology Company (Shanghai,
China). The DNA concentration was determined spectrophotometrically using
the known molar absorption coefficient 6600 (mol
L−1)−1 cm−1 at 260 nm
(per P or nucleotide unit).29 Denatured
single-stranded DNA (ssDNA) was produced by heating native double-stranded
DNA in a boiling water bath for about 5 min followed by rapid cooling in an
ice bath. Chitosan oligomer (1.0% solution in 1.0% acetic acid) was
purchased from Aldrich (USA). Aminoferrocene was obtained from Organic
Laboratory of Chemistry Department, East China Normal University (Shanghai,
China). 1-Ethyl-3-(3-dimethylaminopropyl) carbodiimide (EDC) and
hydroxymethylaminomethane (TRIS) were purchased from Sigma (USA),
N-methylimidazole and sodium dodecylsulfate (SDS) were obtained
from Guangyao Chemical Reagent Company (Jiangsu, China). The following
buffers were used: 2 × SSC buffer: 0.3 mol L−1 NaCl
+ 0.03 mol L−1 sodium citrate (pH 7.0) and TE buffer: 10
mmol L−1 TRIS–HCl + 1.0 mmol L−1
EDTA (pH 8.0).Other reagents were commercially available and were all of analytical
reagent grade. Solutions were prepared with distilled water.
Instrumentation
A CHI 630 Electrochemical Analyzer (CHI Instruments Inc. USA), a JB-1
stirring machine (Branson, Shanghai, China) and a TDL-16B centrifuge
(Anting Science Instrument Inc., Shanghai, China) were used.The three-electrode system consisted of a working electrode made of
glassy carbon (3 mm id), an Ag/AgCl (KCl, 3.0 mol L−1)
reference electrode and a counter electrode made of platinum. All
measurements were carried out in a 10 mL cell.
Procedure
The synthesis of DNA probe labeled with AFC. Denatured PCR DNA (1.0 mL of 0.1 mg mL−1) was added
into 10.0 mL of 0.1 mol L−1 imidazole solution (pH 6.8).
After 15 min, 1.0 mL of 2.5 × 10−4 mol
L−1 AFC and 40 μL of 0.1 mol L−1 EDC
were added to the solution. The solution was vigorously shaken at room
temperature (25 ± 0.5 °C) for 30 min, then left overnight.
Ethanol and 3.0 mol L−1 acetate buffer (pH 5.2) in the
ratio of 20 + 1 (v/v) were added and then the solution was placed in a
refrigerator (<−15 °C) for 20 min. After being centrifuged,
the settling was washed with ethanol solution (70%, 40 μL × 3) to
remove unreacted labeling reagents. This DNA probe labeled with AFC was
dissolved in a 100 μL TRIS–HCl buffer (0.01 mol
L−1, pH 7.0). It was stored in a refrigerator (<
−15 °C) prior to hybridization (The schematic diagram of
synthesis of the DNA probe labeled with AFC is shown in Fig. 1).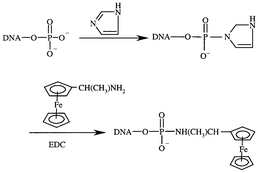 |
| Fig. 1 Synthesis of DNA probe labeled with aminoferrocene. | |
Immobilization of sample ssDNA at the chitosan modified
glassy carbon electrode (ssDNA electrode). A freshly smoothed electrode was uniformly coated with 2.0 μL 1.0%
chitosan solution. After it was naturally dried, the electrode was immersed
in 0.1 mol L−1 NaOH solution for 30 min to make the film
more stable. After further drying, a chitosan oligomer film was formed on
the electrode.The chitosan modified electrode was immersed in a 1.0 mL TE solution
containing 6.06 × 10−6 mol L−1
denatured sample DNA solution (ssDNA). The solution was stirred at room
temperature (25 ± 0.5 °C) for 120 min. Thus the ssDNA was
immobilized on the chitosan-modified electrode (the immobilization
schematic diagram of ssDNA on the chitosan modified glassy carbon electrode
is shown in Fig. 2). Then, the electrode was
washed with 0.1% (m/m) SDS phosphate buffer (pH 7.0) three times and
immersed in a 0.01 mol L−1 TE buffer (pH 8.0) prior to
use.
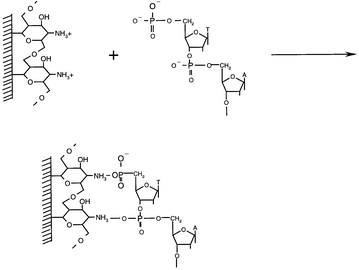 |
| Fig. 2 Immobilization schematic diagram of ssDNA on chitosan-modified glassy
carbon electrode. | |
Hybridization (dsDNA/AFC electrode). The electrodes immobilized with the denatured sample DNA (plasmid DNA,
λDNA, sperm DNA, calf-thymus DNA, pBR322 DNA and yeast RNA) were
immersed in a hybridization buffer (0.3 mol L−1 NaCl +
0.03 mol L−1 sodium citrate) containing the DNA probe
labeled with AFC. The solution was incubated in a water bath at 42 °C
for one hour with shaking to form a dsDNA/AFC system at the electrode
surface. After hybridization, the dsDNA/AFC electrode was washed three
times with a solution containing 0.4 mol L−1 NaOH and 0.1%
SDS to remove the adsorbed DNA probe labeled with AFC.
Electrochemical detection. Cyclic voltammetry (CV) and differential pulse voltammetry (DPV) were
carried out in a 10 ml electrochemical cell with the dsDNA/AFC electrode as
working electrode, an Ag/AgCl electrode as reference electrode and a
platinum wire as counter electrode. Voltammetries were performed in a 0.01
mol L−1 blank TE buffer at a scan rate of 100 mV
s−1. The scan range was from −0.20 to +0.60 V
(vs. Ag/AgCl) for CV and from 0.00 to +0.80 V (vs.
Ag/AgCl) for DPV, respectively.
Results and discussion
Cyclic voltammograms of the DNA probe labeled with AFC in
solution
Cyclic voltammograms of the DNA probe labeled with AFC in a 0.01 mol
L−1 TE solution using bare glassy carbon as working
electrode was recorded at a scan rate of 100 mV s−1 and
scan range from −0.20 to +0.60 V (vs. Ag/AgCl). A pair of
redox peaks was obtained at +0.29 V and +0.20 V (vs. Ag/AgCl),
respectively, which were the redox peaks of the aminoferrocene. With
increasing concentration of the DNA probe labeled with AFC, the anodic peak
current increased obviously (Fig.
3a, b and c). It indicated that the DNA
probe labeled with AFC had a good electrochemical response at the glassy
carbon electrode.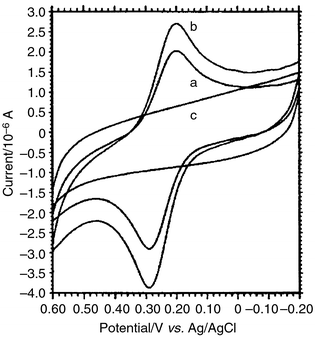 |
| Fig. 3 Cyclic voltammograms of the DNA probe labeled with aminoferrocene in
0.01 mol L−1 TE solution, scan range
−0.20–+0.60 V (vs. Ag/AgCl), scan rate 100 mV
s−1. The concentration of the aminoferrocene labeled DNA
probe was (a) 5.0 × 10−7 mol L−1
and (b) 1.0 × 10−6 mol L−1. (c) The
blank TE solution without the DNA probe labeled with aminoferrocene. | |
Sequence-specific DNA diagnosis
The principle of DNA probes labeled with electroactive reagents for
sequence-specific DNA detection is based on the fact that the sample ssDNA
is immobilized at an electrode surface before it is hybridized with the
sequence-known DNA probe, which is already labeled with an electroactive
reagent, i.e. AFC. Only the complementary sample DNA can form a
double stranded DNA with the electroactive reagent labeled probe, and be
diagnosed using electrochemical techniques.The proposed DNA probe labeled with AFC was applied to hybridize with
sample DNA sequences (all at a concentration of 2.5 ×
10−7 mol L−1) immobilized on the surface
of the chitosan modified glassy carbon electrode. Differential pulse
voltammograms were recorded using the dsDNA/AFC electrode as the working
electrode in a blank TE solution. The anodic wave derived from AFC bound to
the dsDNA on the electrode surface appeared only at the denatured plasmid
DNA electrode by DPV detection. As shown in Fig.
4a, the anodic peak current of AFC was obtained at +0.25 V
(vs. Ag/AgCl) and its value was 3.82 μA. Other noncomplementary
sequence electrodes, namely denatured calf-thymus DNA, denatured sperm DNA,
denatured λDNA, denatured pBR322 DNA and yeast RNA, showed no
electrochemical responses ranging from 0.00 to +0.80 V (vs.
Ag/AgCl) in the case of DPV detection (shown in Fig.
4b, the denatured calf-thymus DNA electrode was used as to
represent these noncomplementary DNA electrodes). These data indicated that
the DNA probe labeled with AFC could distinguish between the complementary
DNA sequence and the noncomplementary DNA sequences and it showed high
selectivity.
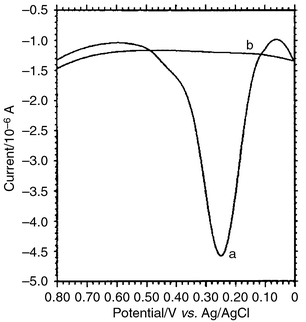 |
| Fig. 4 Differential pulse voltammograms of dsDNA/AFC electrode in a 0.01 mol
L−1 blank TE solution, scan range, 0.00–+0.80 V
(vs. Ag/AgCl), scan rate, 100 mV s−1. Chitosan
amount (1.0% solution in 1.0% acetic acid), 2.0 μL; immobilization time,
120 min; hybridization time, 60 min. The DNA probe labeled with AFC
hybridizes with (a) denatured plasmid DNA electrode, (b) noncomplementary
DNA electrode. | |
Condition optimization
Factors affecting the immobilization of denatured sample DNA and
hybridization were optimized aiming at maximizing the sensitivity and
shortening the detection time. As shown in Fig.
5, using the AFC labeled DNA as an indicator, the peak current of
chitosan-modified electrode increased rapidly with increase of the applied
amount of chitosan at the electrode. When chitosan was over 2.0 μL, the
increase of the peak current leveled off (Fig.
5A), so the appropriate amount of chitosan was 2.0 μL. The
influence of immobilization time was examined from 10 to 150 min. The
current of AFC increased gradually with the increase of the immobilization
period up to 120 min, and then it leveled off (Fig.
5B). This showed that the surface was saturated with denatured
sample DNA. Fig. 5C demonstrates the effect
of the pH in the hybridization solution. As can be seen, the pH value has
no pronounced effect on the hybridization (in the range of pH 5–8).
The effect of the hybridization time is displayed in Fig. 5D. As the hybridization time increased, the
current of AFC increased rapidly at beginning (up to 60 min) and then
leveled off slightly. Based on the data of Fig.
5, as the best compromise between sensitivity and speed, most work
employed a 120 min immobilization time, a 60 min hybridization time and a 2
× SSC buffer (pH 7.0).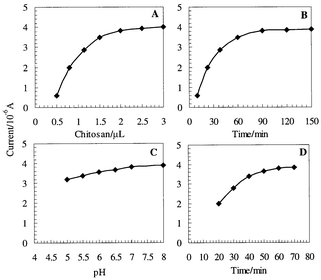 |
| Fig. 5 Effect of (A) chitosan amount, (B) immobilization time, (C) pH value and
(D) hybridization time in a 0.01 mol L−1 blank TE
solution. The DNA probe labeled with AFC was used as an indicator. Other
conditions were the same as in Fig. 4. Each
value was the mean of at least seven replicate measurements. | |
Linearity and detection limits
The linearity of the denatured plasmid DNA was investigated by varying
its concentration over the range of 1.0 ×
10−8–6.0 × 10−6 mol
L−1 (TE buffer, 120 min immobilization and 60 min
hybridization). The well-defined DPV peak of AFC increased linearly with
increasing concentration of the denatured plasmid DNA. The regression
equation was Y = 1.2173 × 107X + 1.080
(X was the concentration of denatured plasmid DNA from 1.0 ×
10−8 to 6.0 × 10−6 mol
L−1, Y was the peak current; the unit was μA)
and regression coefficient (r2) of the linear curve was
0.9971. The detection limit based on 3σ (where σ is the
standard deviation of a blank solution, n = 11) was 2.0 ×
10−9 mol L−1.Reproducibility and stability
The reproducibility was estimated by making repetitive hybridizations
with denatured plasmid DNA according to the specified procedure. The
relative standard deviation (RSD) based on 11 measurements for the
denatured plasmid DNA was 5.3%. Only 4.2% deterioration of anodic peak
currents was found during three months storage of the AFC labeled probe in
a refrigerator.Conclusions
In this paper, a DNA probe labeled with aminoferrocene (AFC) was
synthesized using a carbodiimide (EDC) for the first time. Meanwhile, the
sample ssDNA was successfully immobilized onto a chitosan-modified glassy
carbon electrode. The proposed DNA probe labeled with AFC was successfully
used for the sequence-specific DNA detection. Only the complementary
sequence (cDNA) could form a double-stranded DNA (dsDNA) with the DNA probe
labeled with AFC. The probe showed high sensitivity and selectivity. Our
future work will be focused on the further study of the electrochemical
characterization of chitosan-modified electrode for ssDNA immobilization
and the applications of the electrochemical DNA probe. Acknowledgements
We thank the National Nature Science Foundation of China (NSFC) which
financially supports this work (No. 29875008).References
- J. Olejnik, E. Krzymanska-Olejnik and K. J. Rothschild, Nucleic Acids Res, 1998, 26, 3572 CrossRef CAS.
- T. Livache, B. Fouque, A. Roget, J. Marchand, G. Bidan, R. Teoule and G. Mathis, Anal. Biochem, 1998, 255, 188 CrossRef CAS.
- D. K. Xu, L. R. Ma, Y. Q. Liu and Z. H. Jing, Analyst, 1999, 124, 533 RSC.
- G. Marrazza, I. Chiane and M. Mascini, Biosens. Bioelectron, 1999, 14, 43 CrossRef CAS.
- E. Palecek, M. Fojta, M. Tomschik and J. Wang, Biosens. Bioelectron, 1998, 13, 621 CrossRef CAS.
- M. Fojta, V. Stankova, E. Palecek, P. Koscielniak and J. Mitas, Talanta, 1998, 46, 115 CrossRef CAS.
- S. O. Kelley, E. M. Boon, J. K. Barton, N. M. Jackson and M. G. Hill, Nucleic Acids Res, 1999, 27, 4830 CrossRef CAS.
- T. Ihara, Y. Maruo, S. Takenaka and M. Takagi, Nucleic Acids Res, 1996, 24, 4273 CrossRef CAS.
- M. T. Carter, M. Rodriguez and A. J. Bard, J. Am. Chem. Soc, 1989, 111, 8902.
- M. Rodriguez and M. T. Carter, Anal. Chem, 1990, 62, 2658 CrossRef CAS.
- S. Takenaka, K. Yakenaka, M. Takagi, Y. Uto and H. Kondo, Anal. Chem, 2000, 72, 1334 CrossRef CAS.
- K. Yamashita, S. Takenaka and M. Takagi, Nucleic Acids Symp. Ser, 1999, 42, 185 Search PubMed.
- J. Wang, G. Rivas, X. Cai, M. Chicharro, C. Parrado, N. Dontha, A. Begleiter, M. Mowat, E. Palecek and P. Nielsen, Anal. Chim. Acta, 1997, 344, 111 CrossRef CAS.
- J. Wang, G. Rivas, X. Cai, N. Dontha, H. Shiraishi, D. Luo and F. Valera, Anal. Chim. Acta, 1997, 337, 41 CrossRef CAS.
- K. Hashimoto, K. Miwa and Y. Ishimori, Superamol. Chem, 1993, 2, 265 Search PubMed.
- K. Hashimoto, K. Itoh and Y. Ishimori, Anal. Chim. Acta, 1994, 286, 219 CrossRef CAS.
- K. M. Millan and S. R. Mikkelsen, Anal. Chem, 1993, 65, 2317 CrossRef CAS.
- K. M. Millan, A. Saraullo and S. R. Mikkelsen, Anal. Chem, 1994, 66, 2943 CrossRef CAS.
- T. Ihara, M. Nakama, M. Nakano and M. Maeda, Chem. Commun, 1997, 1609 RSC.
- S. Takenaka, Y. Uto, H. Saita, M. Yokayama, H. Kondo and D. Wilson, Chem. Commun, 1998, 1111 RSC.
- S. H. Liu, J. N. Ye, P. G. He and Y. Z. Fang, Anal. Chim. Acta, 1996, 335, 239 CrossRef CAS.
- X. Y. Sun, P. G. He, S. H. Liu, J. N. Ye and Y. Z. Fang, Talanta, 1998, 47, 487 CrossRef CAS.
- C. Xu, P. G. He and Y. Z. Fang, Anal. Chim. Acta, 2000, 411, 31 CrossRef CAS.
- C. Xu, H. Cai, P. G. He and Y. Z. Fang, Chem. J. Chin. Univ, 2000, 21, 1187 Search PubMed.
- X. Y. Sun, C. Xu, S. H. Liu, P. G. He and Y. Z. Fang, Chem. J. Chin. Univ, 1998, 19, 1393 Search PubMed.
- C. Xu, H. Cai, P. G. He and Y. Z. Fang, Fresenius’ J. Anal. Chem, 2000, 367, 593 CrossRef CAS.
- Y. H. Yu and B. L. He, Polym. Bull, 1997, 12, 232.
- H. Hayatsu, Y. Tanaka and K. Negishi, Nucleic Acids Symp. Ser, 1997, 37, 139 Search PubMed.
- T. Motomura and Y. Aoyama, Bull. Chem. Soc. Jpn, 1992, 65, 1755 CAS.
|
This journal is © The Royal Society of Chemistry 2001 |
Click here to see how this site uses Cookies. View our privacy policy here.