DOI:
10.1039/A906852J
(Paper)
Analyst, 2000,
125, 217-220
Determination of cyclamate in low-calorie foods by
high-performance liquid chromatography with indirect visible photometry
Received 23rd August 1999, Accepted 22nd November 1999
First published on UnassignedUnassigned7th January 2000
Abstract
A rapid and simple method using reversed-phase
high-performance liquid chromatography combined with indirect visible
photometry at 433 nm was developed to determine cyclamate in some food
samples. Cyclamate was not detected in these chosen samples as its use is
banned in Hong Kong. Cyclamate can easily be detected in spiked samples
using a mobile phase consisting of 30 μmol dm−3 Methyl
Red and 0.02 mol dm−3 phosphate buffer (pH
7.0)–methanol in a volume ratio of 3∶2. The column temperature
was set at 23 °C. The detection limit was 0.14 mmol
dm−3 and the relative standard deviation of the peak area
response was 0.58% for a solution containing 5.0 mmol dm−3
of cyclamate (n = 8). This method was successfully applied to the
analysis of eight spiked food samples and the cyclamate recoveries for
these samples ranged from 93 to 99%.
Artificial sweeteners play an important role in our society not
only for diabetic patients but also for people using low-calorie foods. To
date the most commonly used artificial sweeteners are saccharin, cyclamate,
aspartame and acesulfam. The sodium and calcium salts of cyclamic acid are
used extensively in many diet and medical products. It has been reported
that cyclamate is a potent carcinogen when it is converted into
cyclohexylamine in the gastrointestinal tract. Some countries such as
Canada, USA, European countries, India and Hong Kong have banned its use as
a food additive but its usage is still permitted in other countries
including mainland China.1,2 The
detection and quantification of cyclamate in diet foods is still regularly
performed in Hong Kong.Tremendous efforts over the years have been devoted to the development
of methods for the determination of cyclamate. There are many analytical
procedures for determining this sweetener, such as gravimetry,3 volumetric analysis,4 amperometry,5
ion-selective electrode,6 gas
chromatography,7 high-performance liquid
chromatography (HPLC),8–12 capillary electrophoresis13 and spectrophotometry or flow injection
spectrophotometry.14–17 Most of these methods require extensive or
laborious chemical reactions and extraction procedures. HPLC is one of the
most useful and popular techniques as it can separate target analytes from
a complex mixture in samples when an optimum mobile phase and stationary
phase are employed. Unfortunately, traditional HPLC is not suitable for the
determination of cyclamate as cyclamate does not absorb in the usable
ultraviolet/visible (UV/VIS) range. This shortcoming has been solved by
using indirect UV photometry,8,13
post-column ion-pair extraction,9,10
pre-column derivatisation11 and
conductivity detection.12 Indirect UV
photometry has been shown to be a very sensitive detection method and has
the advantage of being applicable to conventional HPLC instrumentation
without any cumbersome modification.18 In
this technique, a fixed concentration of a UV absorbing ion is added into
the mobile phase. The absorbance of this mobile phase is then monitored and
solute ions can easily be detected by the change in the baseline absorbance
as either negative or positive peaks.19,20 In most indirect photometric methods, UV absorbing
ions are normally used as they are compatible with the UV light sources
such as deuterium, mercury arc and xenon lamps used in HPLC. However, the
use of chromogenic dyes is seldom mentioned or realised. It is possible
that the principle of indirect photometry in conjunction with HPLC can also
be applied to a mobile phase containing a visible absorbing ion. The main
advantage of using visible light detection is that the latest
optoelectronics including super-bright light emitting diodes (LEDs),
photodiodes, charge-coupled devices (CCDs) and plastic optical fibres can
be used to construct a relatively cheap and compact detection unit for
HPLC.
In this paper, we demonstrate the use of a chromogenic dye,
o-Methyl Red, as a visible absorbing dye dissolved in a mobile
phase, combined with an octadecylsilica column to determine cyclamate in
various low-calorie foods.
Experimental
Materials
Aspartame, cyclamate sodium salt and saccharin sodium salt were obtained
from Sigma (St. Louis, MO, USA), o-Methyl Red sodium salt from
Aldrich (Milwaukee, WI, USA), methanol (HPLC grade) from Acros Organics
(Geel, Belgium) and disodium hydrogen phosphate and monosodium dihydrogen
phosphate from Farco Chemical Supplies (Beijing, China). All other reagents
were of analytical-reagent grade and mobile phase solutions were prepared
in de-ionised water.Instrumentation
The high-performance liquid chromatograph used was an HP1050 series
consisting of a pumping system, a vacuum degasser and a variable wavelength
detector (Hewlett-Packard, Wilmington, DE, USA). The column was an Inertsil
ODS-3, 5 μm particle size, 250 × 4.6 mm id (GL Sciences, Tokyo,
Japan). The column temperature was controlled by a Model CH-30 column
heater in conjunction with a Model TC-50 temperature controller (Eppendorf
Scientific, Westbury, NY, USA). pH measurements were taken on an Orion
(Chicago, IL, USA) combined pH glass electrode. UV/VIS absorption spectra
were measured on a Cary 100 Scan UV/VIS spectrophotometer (Varian
Australia, Mulgrave, Victoria, Australia) using 10 mm matched cuvettes.Chromatographic procedures
Mobile phases were prepared from solvent mixtures of methanol and
solutions of Methyl Red dissolved in phosphate buffers (pH 7.0). The mobile
phase compositions were varied with different volume ratios of methanol and
phosphate buffers and also concentrations of Methyl Red in the buffers. All
mobile phases were filtered through a 0.45 μm filter and degassed in an
ultrasonic bath prior to use. Normally, the column was pre-conditioned for
1–2 h by pumping through a mobile phase at a flow rate of 1.0
cm3 min−1 and the column effluent was monitored
at 433 nm. The chromatographic separation was then begun after a steady
baseline response of the detector had been attained. The calibration
standards were dissolved in the mobile phases and injected into a Rheodyne
sample injector having a sample loop volume of 20 mm3. Spiked
samples were prepared by either dissolving in or diluting with the mobile
phases, if not stated otherwise.Results and discussion
Selection of wavelength for detection
o-Methyl Red is a well known chromogenic dye which has a strong
UV/VIS absorption spectrum. The UV/VIS absorption spectrum of a chromogenic
dye may shift on changing the solvent system used. Therefore, the UV/VIS
absorption spectrum of Methyl Red in mobile phases of various compositions
were determined and are displayed in Fig. 1.
The UV/VIS absorption spectrum does not shift much when the volume ratio of
buffer to methanol is varied from 4∶1 to 1∶1. The absorption
peak maximum is at 433 nm and was used throughout the chromatographic
separation.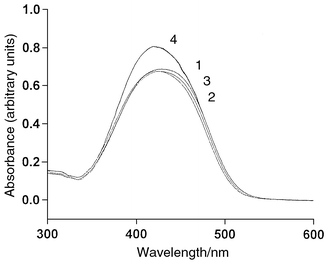 |
| Fig. 1 Absorption spectra of Methyl Red (30 μmol dm−3) in
various volume ratios of 0.02 mol dm−3 phosphate buffer
(pH 7.0)–methanol: (1) 4∶1; (2) 7:3; (3) 3∶2; (4)
1∶1. | |
Selection of eluent pH
The use of o-Methyl Red as an indicator for acid–base
titrations in aqueous solutions is based on the observation that the
indicator changes its colour in the pH range 4.2–6.3.21 The acid dissociation constant of
o-Methyl Red is pKa = 5.1.22 It is obvious that different forms of Methyl Red
exist in different pH ranges. In Fig. 1,
broad absorption bands at 350–500 nm are observed due to the
formation of the yellow anionic form of Methyl Red at pH 7.0. This dominant
anionic form does not change even on addition of methanol to the buffer and
is consistent with the results obtained by other researchers.23 An intense absorption band with a peak maximum
of 520 nm is observed if the pH is lowered to 3.8 because of the formation
of the red protonated form of Methyl Red.24
In principle, the red protonated form of Methyl Red can also be used as the
visible-absorbing ion in the mobile phase for indirect photometric
detection.25 Unfortunately, the light
intensity of our HPLC detection system at 520 nm is not strong enough for
photometric measurement. Therefore, the pH of the eluent was kept at 7.0 in
order to ensure that all the Methyl Red was in its anionic form. pH values
higher than 7.0 are not recommended as there is a possibility of the
dissolution of silica material in the column at high pH values.Selection of buffer and its concentration
The use of buffer is essential to keep the eluent pH constant as Methyl
Red can exist in different forms at different pH values. There are many
buffer systems that can be tried, such as sodium hydrogen
carbonate–carbonic acid, ammonium chloride–aqueous ammonia and
sodium phosphate buffers. However, the last one is the most appropriate
choice because phosphate buffer has the advantage of reducing any other
mixed mechanisms that lead to peak tailing. Phosphate salt can effectively
compete for the electrostatic sites by hindering the ionic interactions
between sample solutes and uncapped silanol groups of the packing
materials.26 Second, a wide pH range from 4
to 12 can easily be prepared when a phosphate buffer is used.Different concentrations of phosphate buffers (0–0.2 mol
dm−3) at pH 7.0 were used to prepare 3∶2
buffer–methanol. It was found that a lower concentration of buffer
gave a very unstable baseline for detection. However, too high a
concentration of buffer will build up a high column back-pressure, which
can damage the operation of an HPLC pump. There is also a risk of
precipitating the phosphate salt if a high concentration of buffer is used.
From our experience, an optimum concentration of phosphate buffer of 0.02
mol dm−3 was chosen throughout the remainder of the
experiments.
Composition of mobile phase
The volume ratio of a buffer–methanol mixture as the mobile phase
was investigated for chromatographic separation of artificial sweeteners.
When a volume ratio of 9∶1 of buffer to methanol was used as the
mobile phase, there was no background absorption signal on the detector.
Methyl Red was completely retained in the column since the mobile phase is
too hydrophilic. However, when 100% methanol was pumped through the column
again, all the Methyl Red was desorbed from the column, giving a very high
background absorption signal. Lower volume ratios of buffer–methanol
mixtures as mobile phases (4∶1 to 1∶1) were subsequently
tested. It was found that at 4∶1 and 7∶3 volume ratios of
buffer to methanol, the saccharin, cyclamate and aspartame peaks were not
satisfactorily separated. When the volume ratio was set at 3∶2, all
the solute peaks were completely separated within 12 min.Fig. 2 shows a typical chromatogram for
the separation of a mixture of saccharin, cyclamate and aspartame using
buffer–methanol (3∶2) as the mobile phase and o-Methyl
Red as the visible-absorbing anion. The first negative peak was assigned as
the injection peak arising from a decrease in absorbance because of all the
unretained solutes and the eluent dilution resulting from the injected
sample solvent. This observation was confirmed by the injection of a
solvent without cyclamate into the column. A similar negative peak at the
same retention time was found in the chromatogram. The second, third and
fourth positive peaks were identified as saccharin, cyclamate and
aspartame, respectively. The retention mechanisms for these sweeteners are
complicated processes. It has been mentioned that dynamic modification of a
hydrophobic surface of a reversed stationary phase to a charged double
layer can be achieved by the sorption of a hydrophobic ionic modifier from
the eluent on its hydrophobic surface.27 In
the present mobile phase system, it is probable that Methyl Red with its
counter-ion (Na+) is adsorbed on the octadecylsilica surface to
form a charged double layer. Below pH 7.0, cyclamate and aspartame are
present in anionic form whereas saccharin is in neutral form. The retention
of cyclamate and aspartame can be explained by the anion exchange mechanism
between the analyte and the surface adsorbed Methyl Red ions.28 However, the retention of saccharin is possibly
based on the displacement of the sodium–Methyl Red ion-pair from the
stationary phase.
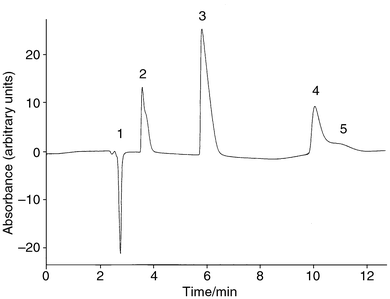 |
| Fig. 2 Chromatogram showing the separation of saccharin (4.9 mmol
dm−3), cyclamate (5.0 mmol dm−3) and
aspartame (3.4 mmol dm−3) using a mobile phase of
3∶2 buffer (pH 7.0)–methanol and 30 μmol
dm−3 Methyl Red at a column temperature of 23 °C. (1)
Injection peak; (2) saccharin; (3) cyclamate; (4) aspartame; (5) system
peak. | |
In general, the sample elution peaks can be either positive or negative
according to their charge and retention relative to the UV/VIS-absorbing
ion. The injection of a sample with the same charge as the
visible-absorbing ion (in the present system) gives a positive peak if the
capacity factor of the sample is smaller than the visible-absorbing
ion.25 In addition to the sample solute
peaks, a small positive system peak eluting close to the aspartame peak was
also observed. This system peak is considered to arise from the elution of
neutral eluent molecules desorbed from the column surface during sample
injection. The occurrence of this system peak can be explained by
reversed-phase and dye-displacement mechanisms.29 Apparently, the present eluent still contained a
very small amount of neutral molecules. If the eluent pH is raised to a
level where the eluent is completely ionised, then the system peak can be
eliminated.20 However, the pH of the
present mobile phase cannot be raised further as dissolution of the packing
material occurs, as mentioned above.
When the composition of the mobile phase was changed to 1∶1
buffer–methanol, a standard mixture of saccharin, cyclamate and
aspartame could also be completely separated within 7 min. However, the
sensitivity for detection of cyclamate is lower than that when using a
mobile phase of 3∶2 buffer–methanol. Hence the sample analysis
of low-calorie foods was only carried out using 3∶2
buffer–methanol.
Effect of Methyl Red concentration
The effect of Methyl Red concentration on the sensitivity for the
detection of cyclamate was investigated by varying its concentration in the
mobile phase from 10 to 50 μmol dm−3. A series of
cyclamate standards were injected into the column. The peak area was
plotted against the concentration of cyclamate and the results for the
regression analysis are given in Table
1. The higher the concentration of the Methyl Red in the mobile
phase, the higher was the sensitivity for the detection of cyclamate.
However, at a higher dye concentrations, it would generate a higher
background absorption signal, which in turn would increase the noise level
of the detection. It has been suggested that the photometric error would be
smaller if the absorbance range can lie between 0.2 and 0.8.30 Therefore, the eluent ion concentration
(10–30 μmol dm−3) chosen should have a background
absorption that lies within this range. The limit of detection can be
determined as three times the standard deviation of the peak area of the
blank and from results for the linear regression equations in Table 1.31 The
limits of detection for 10, 30 and 50 μmol dm−3 Methyl
Red solvent systems were 0.80, 0.14 and 0.30 mmol dm−3,
respectively. Consequently, a concentration of Methyl Red of 30 μmol
dm−3 was chosen for most chromatographic separations so as
to maximise the sensitivity of detection, minimise the photometric error
and lower the limit of detection for cyclamate.
Table 1 Linear regression analysis for concentration of cyclamate standard
against peak area using various Methyl Red concentrations in 3∶2
buffer–methanol as mobile phase at a column temperature of 23 °C
(n = 7)
Methyl Red concentration/μmol
dm−3 | Slope/area unit mmol−1
dm3 | y-Intercept/area unit | r2 |
---|
10 | 39.33 ± 0.60 | −4.73 ± 5.19 | 0.9988 |
30 | 101.64 ± 0.28 | 8.32 ± 2.40 | 0.9999 |
50 | 153.57 ± 0.91 | 22.52 ± 7.94 | 0.9998 |
Effect of column temperature
It has been mentioned that careful temperature control of the whole
chromatographic system is required for good stability of the mobile phase
absorbance when indirect photometry is applied.25 The effect of column temperature from 23 to 32
°C on the sensitivity for the detection of cyclamate was therefore
investigated. A series of cyclamate standards were injected into the HPLC
system at different column temperatures. The peak area was then plotted
against the concentration of cyclamate and the results for the regression
analysis are given in Table 2. The
sensitivity increases with increase in temperature up to 32 °C. The
sensitivities at 27 and 32 °C are very close to each other. Although
higher temperatures can improve the sensitivity slightly, it was found that
the limit of detection was worse at higher column temperatures (0.14, 0.35
and 0.40 mmol dm−3 cyclamate at 23, 27 and 32 °C,
respectively). Therefore, the column temperature was set at 23 °C for
the determination of cyclamate in food samples.
Table 2 Linear regression analysis for concentration of cyclamate standard
against peak area using various column temperatures with 3∶2
buffer–methanol containing 30 μmol dm−3 Methyl
Red as mobile phase (n = 7)
Column temperature/°C | Slope/area unit mmol−1
dm3) | y-Intercept/ area unit | r2 |
---|
23 | 101.64 ± 0.28 | 8.32 ± 2.40 | 0.9999 |
27 | 117.70 ± 0.79 | 9.05 ± 6.82 | 0.9998 |
32 | 115.92 ± 0.88 | 15.15 ± 7.62 | 0.9997 |
Repeatability
In order to investigate the repeatability of the proposed method, a 5.0
mmol dm−3 cyclamate standard was injected eight times into
the HPLC system using optimum conditions of 30 μmol
dm−3 Methyl Red, 3∶2 buffer–methanol and 23
°C. The relative standard deviation was found to be 0.28%. Our proposed
HPLC method demonstrates good repeatability.Analysis of food samples
Eight commercial samples were bought from a local supermarket in Hong
Kong and analysed using the optimum experimental conditions. No detectable
cyclamate was found in these samples. Recovery tests were also used to test
the validity of our method by adding a fixed amount of cyclamate to the
food samples. A typical chromatogram of a spiked sample (Diet Seven-up) is
shown in Fig. 3. A small positive peak that
eluted before the cyclamate peak was identified as benzoate. This is not
surprising since benzoic acid is a commonly used preservative added to soft
drinks. A small amount of aspartame was also found in the chromatogram, as
aspartame is added as a low-calorie sweetener in this type of sample.
Chromatograms for other spiked samples were very similar. The recovery
tests for all the spiked samples are summarised in Table 3 and the results were satisfactory.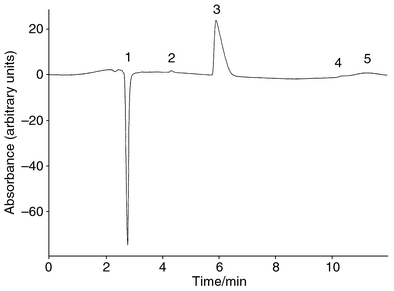 |
| Fig. 3 Chromatogram of Diet Seven-up spiked with 5.0 mmol dm−3
cyclamate using a mobile phase of 3∶2 buffer–methanol
containing 30 μmol dm−3 Methyl Red at pH 7.0 and 23
°C. (1) Injection peak; (2) benzoate; (3) cyclamate; (4) aspartame; (5)
system peak. | |
Table 3 Analysis of real samples and the recovery test
Sample | Content/ mmol dm−3 | Added/mg | Recovery (%) |
---|
ND = not detected. |
---|
Diet Coke | NDa | 10.1 | 98.1 |
Diet Pepsi | ND | 10.6 | 98.7 |
Diet Seven-up | ND | 10.9 | 99.2 |
Diet Sprite | ND | 10.4 | 96.2 |
Lucozade | ND | 11.2 | 99.4 |
Preserved sweet prune | ND | 21.1 | 93.2 |
Vita guava juice | ND | 10.3 | 97.2 |
Vita lemon tea | ND | 10.4 | 98.0 |
Conclusion
An HPLC method combined with indirect visible photometry for the
separation and determination of cyclamate was developed. The method was
applied to the analysis of real samples with satisfactory results. There is
great potential for employing indirect visible photometry in HPLC since the
latest optoelectronics, including super-bright LEDs, CCDs, photodiodes and
optical fibres, can easily be incorporated to assemble a compact and
inexpensive detection system for HPLC instrumentation.Acknowledgements
The research described in this paper was made possible in part by the
HKBU (project No. FRG/97-98/II-05).References
- Encyclopaedia of Food Science, Food Technology and
Nutrition, ed. R. Macrae, R. K. Robinson and M. J. Sadler,
Academic Press, London,
1993, vol. 2, p. 1287. Search PubMed.
- M. Mathlouthi and
C. Bressan, in
Low-Calorie Foods and Food Ingredients, ed. R. Khan,
Blackie, Glasgow, 1993,
ch. 8. Search PubMed.
- J. B. Wilson, J. Assoc. Off. Anal. Chem., 1955, 38, 559 Search PubMed.
- M. L. Richardson and P. E. Luton, Analyst, 1966, 91, 520 RSC.
- O. Fatibello-Filho, M. D. Capelato and S. A. Calafatti, Analyst, 1995, 120, 2407 RSC.
- D. Feng and C. Chen, Fenxi Huaxue, 1988, 16, 737 Search PubMed.
- J. A. W. Dalziel, R. M. Johnson and A. J. Shenton, Analyst, 1972, 97, 719 RSC.
- A. Herrmann, E. Damawandi and M. Wagmann, J. Chromatogr., 1983, 280, 85 CrossRef CAS.
- J. F. Lawrence, Analyst, 1987, 112, 879 RSC.
- J. F. Lawrence and C. F. Charbonneau, J. Assoc. Off. Anal. Chem., 1988, 71, 934 Search PubMed.
- I. Casals, M. Reixach, J. Amat, M. Fuentes and L. Serra-Majem, J. Chromatogr. A, 1996, 750, 397 CrossRef CAS.
- Q.-C. Chen, S.-F. Mou, K.-N. Liu, Z.-Y. Yang and Z.-M. Ni, J. Chromatogr. A, 1997, 771, 135 CrossRef CAS.
- C. O. Thompson, V. C. Trenerry and B. Kemmery, J. Chromatogr. A, 1995, 704, 203 CrossRef CAS.
- K. C. Güven, T. Özol, N. Ekiz and T. Güneri, Analyst, 1984, 109, 969 RSC.
- S. T. Gouveia, O. Fatibello-Filho and J. de A. Nóbrega, Analyst, 1995, 120, 2009 RSC.
- C. S. P. Sastry, K. R. Srinivas, K. M. M. K. Prasad and A. G. Krishnamacharyulu, Analyst, 1995, 120, 1793 RSC.
- C. Cabero, J. Saurina and S. Hernández-Cassou, Anal. Chim. Acta, 1999, 381, 307 CrossRef CAS.
- H. Small and T. E. Miller, Jr., Anal. Chem., 1982, 54, 462 CAS.
- M. Denkert, L. Hackzell, G. Schill and E. Sjsgren, J. Chromatogr., 1981, 218, 31 CrossRef CAS.
- P. E. Jackson and P. R. Haddad, J. Chromatogr., 1985, 346, 125 CrossRef CAS.
- A. I. Vogel,
Vogel’s Text-book of Quantitative Inorganic Analysis Including
Elementary Instrumental Analysis, Longman,
London, 4th edn., 1978, p.
761. Search PubMed.
- D. F. Boltz and
J. A. Howell,
Colorimetric Determination of Nonmetals, John Wiley
and Sons, New York, 2nd edn.,
1978, p. 60. Search PubMed.
- S. A. Tucker, H. C. Bates and W. E. Acree, Jr., Analyst, 1995, 120, 2277 RSC.
- K. M. Tawarah and H. M. Abu-Shamleh, Dye Pigm., 1991, 17, 203 Search PubMed.
- CRC Handbook of HPLC for the Separation of Amino Acids, Peptides,
and Proteins, ed. W. S. Hancock, CRC Press,
Boca Raton, FL, 1984, vol. 1,
pp. 182–183. Search PubMed.
- B. A. Bidlingmeyer,
Practical HPLC Methodology and Applications, John
Wiley and Sons, New York, 1992,
p. 147. Search PubMed.
- R. M. Cassidy and S. Elchuk, J. Chromatogr. Sci., 1983, 21, 454 CAS.
- B. B. Wheals, J. Chromatogr., 1987, 402, 115 CrossRef CAS.
- N. Chauret and J. Hubert, J. Chromatogr., 1989, 469, 329 CrossRef CAS.
- J. Weiss, Ion
Chromatography, VCH, New
York, 2nd edn., 1995, pp.
316–317. Search PubMed.
- J. C. Miller and
J. N. Miller,
Statistics for Analytical Chemistry, Ellis
Horwood, Chichester, 3rd edn.,
1993, pp. 115–118. Search PubMed.
|
This journal is © The Royal Society of Chemistry 2000 |