DOI:
10.1039/D4RA04310C
(Review Article)
RSC Adv., 2024,
14, 25256-25272
Water-soluble Schiff base ligands and metal complexes: an overview considering green solvent
Received
12th June 2024
, Accepted 30th July 2024
First published on 13th August 2024
Abstract
The water-soluble metal complexes with Schiff base (SB) ligands are of great interest to green chemistry researchers due to their stability, cost-effectiveness, eco-friendly, electron-donating ability, and various applications. They have high potential to express their biological activity including anti-inflammatory, anticancer, antibacterial, antifungal, antioxidant, and DNA binding and cleavage. In the recent era, transition metal complexes have played a significant role in different processes such as hydrogenation, carbonylation, oxidation, reduction, epoxidation, hydrolysis, decomposition, and polymerization reactions in industry. However, their limited aqueous solubility may be the major limitation to their potential catalytic, industrial, and clinical applications. In industrial catalytic processes, it has been proven that water can be used as a solvent to minimize the environmental effect of different reactions as well as simple and complete separation. Water is a green solvent, flexible, non-toxic, safe, readily available, environmentally harmless, and inexpensive. Attaching different substituents on Schiff bases enhances the water solubility and catalytic activity. Studies on water-soluble SB complexes will explore these aspects and their prospects for the future evolution of their diverse applications.
1. Introduction
Schiff bases (SB) are still being used as ligands in the field of coordination chemistry even a century after their discovery.1,2 The current study of SB metal complexes has been greatly expanded to incorporate many elements of bio-coordination and bio-inorganic chemistry. Although this issue has been extensively explored, SB is an important family of organic ligands, and their transition metal complexes are still an interesting topic in inorganic chemistry.3–5 The multifunctional ligands generated from the condensation of amino compounds such as groups NH2, NH2OH, NH2–NH2, etc. with carbonyl compounds (aldehydes or ketones) are known as SB and were first discovered in 1864 by a German chemist named Hugo Schiff.6–9 The SB compounds have a common structural characteristic with the formula RHC = N–R′ where R and R′ are alkyl, aryl, cycloalkyl, or heterocyclic groups. The carbon–nitrogen double bond is commonly called an azomethine group in Schiff bases, which is generated by substituting the carbonyl group in aldehydes or ketones.10 The SB ligands are specially adjusted to bond with different metal ions via a lone pair of electrons of N atoms to form the metal complexes. A Schiff base is commonly made in the presence of acid or base catalysts or with the use of heat.3–6 SB are crystalline solids that have low basicity, but some Schiff bases are reacted with strong acids to form insoluble salts.8,10,11 Scheme 1 depicts the general reaction for the synthesis of SB.
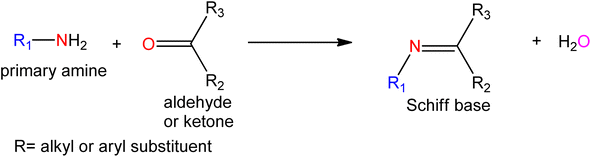 |
| Scheme 1 General methods of preparation of Schiff base ligands. | |
The chemistry of SBs and their metal complexes containing nitrogen, oxygen, and sulfur donor atoms has changed dramatically over the last two decades and has been rewarded recognition.12–16 The formation of carbon-nitrogen bonds in metal complexes aided by SB reactions is due to the presence of the amine group, which is responsible for their biological properties.17,18 It is the root of many organic compounds and a useful intermediate in many organic syntheses. According to large-scale biochemical and medical investigations19–22 these compounds are more efficient against numerous strains of bacteria. It is the class of chemical precursors used in medical and pharmaceutical chemistry that have antibacterial, antifungal, anticancer, antioxidant, and anti-inflammatory properties. They also lead to a variety of binuclear complexes with important implications in magnetochemistry, binuclear reactivity, food, dyes, analytical chemistry, catalysis, pigments, catalysts, organic synthesis intermediates, polymer stabilizers, molecular memory storage, imaging systems, corrosion inhibitors, and the non-enzymatic model for metabolic reactions.23–31 In the presence of electronic features of metal ions such as charge and oxidation number, which take part in a biological system, binding to electron-rich biological components, the function of metal complexes as biological drugs increases largely.32 The special advantages of SB ligands come from the easy access under a simple reaction, and the involvement of additional functional groups regulating their structures and chemo-physical properties. Importantly, introducing an anionic or an extremely polar substituent such as carboxylate, sulfonate, amino, ammonium, methoxy, imidazolium, or phosphonium derivatives generally influences an improved solubility in water. This review focused on various water-soluble SB complexes and their catalytic application including hydrogenation, carbonylation, oxidation, reduction, epoxidation, hydrolysis, decomposition, polymerization reaction, and biological applications including antimicrobial, DNA binding and cleavage, antioxidant, and anticancer activities.
2. Approaches to water-soluble Schiff-base chemistry
Along with conventional methods,3–6 Schiff bases have recently been synthesized using energy-efficient and environmentally benign methods such as microwave irradiation, ultrasound-assisted synthesis, photo-catalysis (UV-visible, IR irradiation), grinding, and milling procedures, etc.33–40 However, the versatility of a compound greatly depends on its ability to dissolve in an aqueous solution. Water-soluble ligands have attracted a lot of attention in recent years because they can easily form stable coordination compounds with a variety of transition metals and exhibit a variety of fascinating features.41 Scientists have recently focused their attention on the biological activities of SB ligands and their transition metal complexes.42–44 However, the poor aqueous solubility of such compounds hinders their probable evolution for their diverse uses. That aqueous solubility is much needed and regarded as a key factor in the selection of different biological drugs.45 SB with no substituents, as well as their metal complexes are water insoluble. The insolubility of such compounds in water is a major stumbling block to their use as medications and biological reagents.46 The synthesis and development of water-soluble SB metal complexes is an important focus of current research for this aim. Usually, the appearance of anionic or an extremely polar substituent or groups in the Schiff-base's backbone promotes increased solubility of their derivatives in an aqueous medium.47 Presently, the main strategy for the researcher to increase the aqueous solubility of SB complexes is to introduce different substituents to their structure. The presence of different substituents on the SB ligand and their metal complexes, such as the sulfonate group,48–58 hydroxyl group,59–64 carboxylate group,65–68 phosphonium group,47,69–72 ammonium or imidazole group,73,74 and methoxy group75,76 increases the water solubility and is a striking feature both for biological and catalytic uses. Murugan Theetharappan et al. have reported that acid group-containing Schiff base complexes were applicable in numerous fields for their water-solubility and slight cytotoxicity in nature.77 A typical review based on water-soluble SBs and their metal complexes provides a wide range of applications including catalytic and biological activities viz. antimicrobial activity, anticancer activity, DNA binding and cleavage, and antioxidant activity.
3. Catalytic activity
SB metal complexes act as catalysts in coordination chemistry, and they have been used to boost yield and product sensitivity in a variety of processes. The key benefits of water-soluble catalysts that make these complexes particularly appealing for commercial applications include simple and complete separation of product and catalyst as well as exceptional long-term stability.78 As a shred of evidence, 300
000 tons of n-butyraldehyde are produced annually from the homogeneous hydroformylation of propene with syngas in a two-phase system by using the water-soluble rhodium complex as a catalyst. The potential applications of metal complexes in various industrial processes such as hydrogenation, carbonylation, oxidation, reduction, hydroformylation, decomposition, epoxidation, and low-pressure polymerization reactions have been facilitated by their proper synthetic routes and their thermal stability.79–83 On the other hand, the function and reactivity of a metal complex as a catalyst very much depends on its ability to dissolve in aqueous solvent.47 Water solubility of metal complexes can be achieved by incorporating water-soluble groups into the SB ligands, which influence the catalytic activities of the active site and, idealy, remain coordinated to the metal site throughout the catalytic reaction. Because of ecologically friendly process, facile product separation, and pH-dependent selectivity in aqueous media, water-soluble transition-metal complexes are effective catalysts in both homogeneous and heterogeneous processes, and consequently there is a growing interest in catalytic applications.84–87 The coordination center of SB metal complexes can be altered by the involvement of diverse substituents in the ligands and is capable of providing electronic characteristics for fine-tuning structures and improving catalytic activity.88–90 The Ni(II)–methyl complex-containing water-soluble ligands were used as catalyst precursors for the olefin polymerization process (Fig. 1). In this case, various water-soluble ligands such as hexamethylenetetramine (urotropine) attached to the metal site enhance the catalytic activity for olefinic polymerization.74
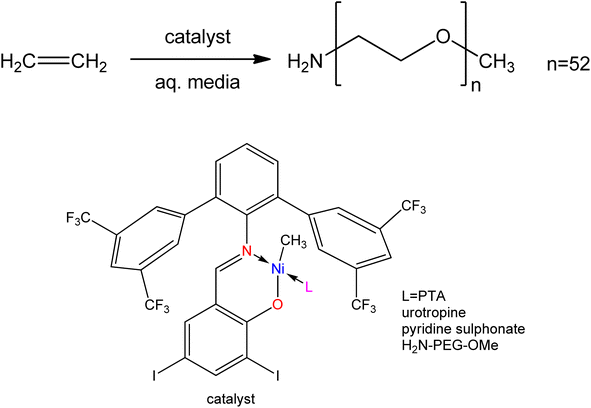 |
| Fig. 1 Catalytic olefin polymerization by water-soluble Ni(II)–methyl complex. | |
Somayeh Azizi Talouki et al.47 synthesized a novel water-soluble Ru(II)–p-cymene complexes having phosphonium and the imidazolium group. Under the right conditions, ruthenium(II) complexes were found as good catalysts for reducing acetophenone, cyclohexanone, cyclopentanone, and 2-butanone to alcohols in high yield (Fig. 2).
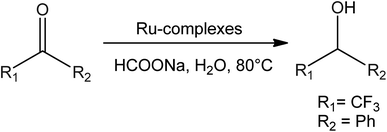 |
| Fig. 2 Catalytic hydrogenation of different carbonyl compounds. | |
Bahram Bahramian et al.71 produced the water-soluble [Mn(III)(salen)]OAc–montmorillonite complex. In the presence of sodium periodate, 4-methoxybenzyl alcohol is oxidized to 4-methoxybenzaldehyde. In this process, water-soluble Mn(III) salen complexes are used as catalysts in the aqueous medium. This catalytic process is applied for the oxidation of primary and secondary alcohols to corresponding aldehydes and ketones (Fig. 3). The water-soluble Mn(III) salen catalyst containing substituted phosphonium groups exhibited more advanced activity than free Mn(III) salen complexes in the oxidation of 4-methoxybenzyl alcohol.
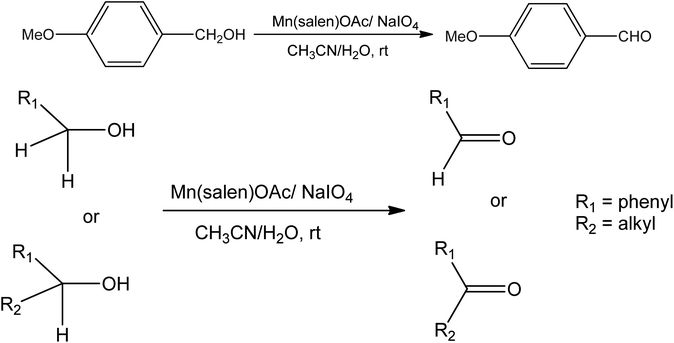 |
| Fig. 3 Oxidation of different alcohol by Mn(III) salen type complexes. | |
A series of 3-indole derivatives were synthesized from indole, aldehyde, and malononitrile in water (Fig. 4) using copper sulfonate salen catalyst by Yanyang Qu et al.91 In the catalytic process, copper(II) complex shows greater catalytic activity than other complexes including Mn(II), Ni(II), and Co(II). The efficiency of the derivatives ranges from good to excellent and can reach up to 97%.
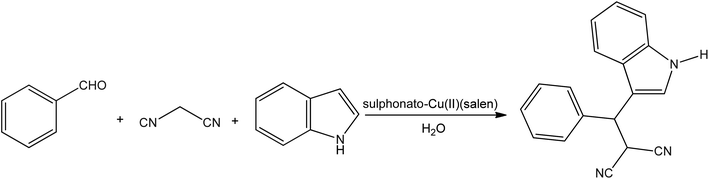 |
| Fig. 4 The catalytic three-component reaction by Cu(II) sulphonato salen catalyst. | |
The water-soluble oxovanadium(V) complexes were synthesized by Ali Hasnaoui et al.75 The oxovanadium complexes exhibited the highest catalytic activity. The oxidation of styrene with tert-butyl hydroperoxide (tBHP) as an oxidant in water produces different products such as epoxide, benzaldehyde, acetophenone, benzoic acid, and 1-phenylethane-1,2-diol (Fig. 5). The catalyst with methoxy group (–OCH3) has higher catalytic activity than the free catalyst.
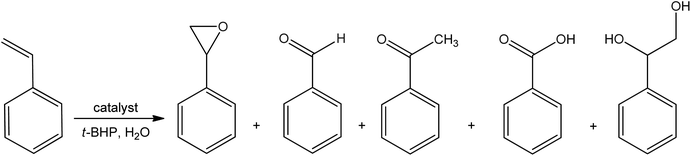 |
| Fig. 5 Catalytic oxidation of styrene by oxovanadium(V) complexes. | |
Kai Zhang et al. synthesized a series of water-soluble Co(II), Mn(II) and Cu(II) complexes from the ligand 3,5-bis[(2-hydroxy-4′-sodium sulphatoethylsulphonyl)azobenzene-methyneimino]-benzoic acid (BHSABA).66 They evaluated those synthesized complexes, and found them to be effective catalysts for H2O2 decomposition (Fig. 6).
 |
| Fig. 6 Catalytic decomposition of H2O2 by BHSABA metal complexes. | |
Claudia Palopoli et al.57,58 reported that a water-soluble bridged di-Mn(III) complex showed catalytic activity for the decomposition of H2O2 and evolution of O2. The relative catalytic activity of synthesized complexes was measured by counting O2 evolved during the volumetric titration in different solvents like DMF, methanol, and water. The rate of O2 evolution gradually decreases when a surplus of H2O2 is added to the solution successively. Maxime Dauchy et al.92 prepared water-soluble rhodium(I) salicylaldiminato and salicylhydrazonic complexes. The rhodium(I) complexes act as catalysts for the hydroformylation of 1-decene in aqueous biphasic solutions (Fig. 7). In the catalysis of 1-decene under 50 bar of CO/H2 pressure and at 80 °C to obtain 81% olefin, 72% aldehydes, and a low linear-to-branched aldehyde.
 |
| Fig. 7 Hydroformylation of 1-decene by rhodium(I) complexes. | |
Lintao Yu et al.93 synthesized phenazines from 2-halogen anilines by homocoupling in an aqueous medium (Fig. 8). In this process, a water-soluble Schiff base Cu(II) complex acts as a catalyst. The reactions were carried out with 2-iodoaniline (0.5 mmol), Cu(II) complex (10 mol%), PTC (phenylthiocarbamide) (20 mol%), K2CO3 (1 mmol) in water (10 mL) at 120 °C.
 |
| Fig. 8 Preparation of phenazines by catalytic homocoupling of sulfonato-Cu(II) salen complex. | |
A recent study reported that water-soluble Cu(II)-complexes of N-salicylidene amino acid Schiff base act as a homogeneous catalyst for the oxidation of alkenes and alcohols.94,95 The catalytic activity of cyclic alkenes and alcohols was higher than acyclic alkene or aromatics alcohols. Water-soluble complex catalysts are becoming increasingly popular because they combine the benefits of homogeneous and heterogeneous catalysis: easy and full separation of the product from the catalyst, high activity, priority, and selectivity.96 From suitable water-soluble ligands, effective catalysts can be constructed for a variety of processes.97
4. Biological activity of Schiff base ligand and metal complexes
4.1 Antimicrobial activity
Antibiotics are material of a small amount of concentration that is used to hinder the growth and replication of both bacteria and fungi. Nowadays without the application of antibiotics, the treatment of contagious diseases would be unbelievable.98 The amino acid Schiff base ligands (Fig. 9) obtained from the condensation of sodium-5-sulfonate-2-hydroxybenzaldehyde with D,L-leucine, and D,L-phenylalanine were synthesized by A. D. M. Mohamad et al.99 and sequentially metal complexes (Fig. 10) were obtained with synthesized ligands. The ligand and metal complexes were found as water-soluble. Those compounds were screened for their antibacterial and antifungal activity against Gram-negative bacteria (Escherichia coli, Serratia marcescens), Gram-positive bacteria (Staphylococcus aureus), and fungal strains (Aspergillus flavus, Candida albicans, and Trichophyton rubrum).
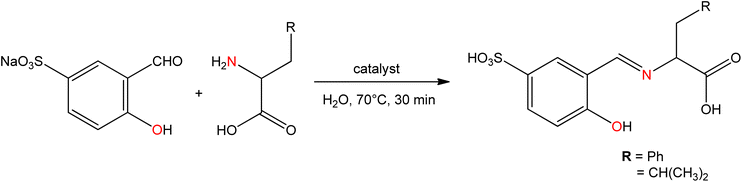 |
| Fig. 9 Synthesis of water-soluble amino acid Schiff base ligand. | |
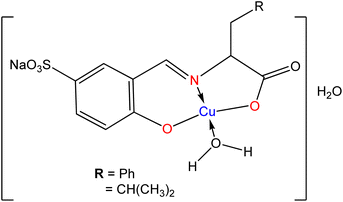 |
| Fig. 10 Structure of copper(II) complexes of N-salicylidene Schiff base amino acid ligand. | |
Seyed Abolfazl Hosseini-Yazdi et al.100 prepared Ni(II), Cu(II), and Zn(II) complexes with SB ligand 3-{[(aminocarbonothioyl)hydrazono]methyl}-4-hydroxybenzenesulfonate, derived by the condensation of 5-sulfonate salicylaldehyde with thiosemicarbazide and 4-phenyl thiosemicarbazide (Fig. 11). The synthesized complexes of copper and nickel evaluated their antifungal and antibacterial activity. The results revealed that the copper(II) complex showed greater efficiency against Gram-positive bacteria than the Gram-negative ones and fungi, while the nickel(II) complex showed superior activity on the Gram-negative E. coli, Gram-positive B. cereus and fungi C. albicans.
 |
| Fig. 11 Synthesis of water-soluble 4-phenylthiosemicarbazone SB ligand. | |
C. Shiju et al.101 prepared a water-soluble SB from the condensation of 4-nitrobenzaldehyde (4-NBA) with glycylglycine (GG) and its metal complexes were synthesized with (4-NBA–GG) ligand (Fig. 12). All the coordinated compounds were found as water soluble. The synthesized compounds were appeared for antibacterial test against four bacteria: Staphylococcus aureus, Bacillus subtilis, Pseudomonas aeruginosa, and Escherichia coli, and antifungal activity against three fungal: Candida albicans, Aspergillus flavus, and Aspergillus niger by the common disc diffusion method. Ciprofloxacin and nystatin were utilized as excellent marketable drugs concerning antibacterial and antifungal activity. The copper(II) complexes exhibited greater activity against Gram-negative bacteria such as Escherichia coli.
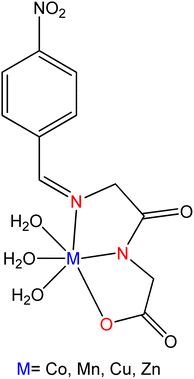 |
| Fig. 12 Structure of metal complexes of (4-NBA–GG) SB ligand. | |
Sohan Lal et al. introduced the water-soluble SB of carboxymethyl chitosan was amalgamated by the condensation reaction of o-carboxymethyl chitosan with vanillin. The prepared SB ligand combined with different salts of metal ions like Cu(II), Ni(II), Fe(II), and Zn(II) to produce metal complexes.102 The complexes were screened for their antibacterial activity against Gram-positive bacteria Bacillus subtilis and Gram-negative bacteria Escherichia coli by using the agar well diffusion method and anti-fungal activity against Aspergillus niger by using poisoned food technique. The activity of the anti-microbial of chitosan SB may be increased due to a significant amount of positive charge on nitrogen. A series of chitosan SB (Fig. 13) derived from the condensation of benzohydrazide with chitosan, pentane 2,4-dione and 1,3-diphenyl-1,3-propanedione were synthesized by Manimohan Murugaiyan et al.103 The studies of anti-microbial activity were directed for the hydrazone O-carboxymethyl chitosan SB and its Zn(II) complexes using the agar plate method. The appearance of the hydrazone functional group on the Schiff bases and their metal complexes showed greater hunting activity in the bacterial field. The prepared chitosan SB and complexes appeared as an excellent drug for bacterial strains E. coli, P. aeruginosa, S. aureus, and B. subtilis, which were grown in Mueller–Hinton agar plates at 37 °C. The coordinated carboxymethylated chitosan SB ligands and their Zn(II) complexes were estimated against fungi A. niger, C. albicans, and A. clavatus. The antifungal activity of the zinc(II) metal complexes was found to be very effective against Candida albicans compared to others drugs those are similar to ampicillin. The order of fungi according to the growth of hindrance was as follows: A. clavatus < A. niger < C. albicans.
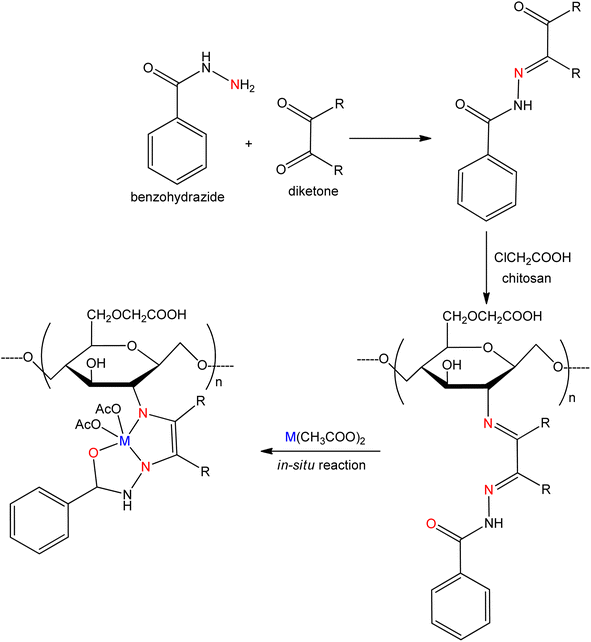 |
| Fig. 13 Synthesis of water-soluble hydrazide based O-carboxymethyl chitosan SB and its metal complexes (M = Cu, Zn). | |
The novel SB ligand synthesized from the distillation of meso-1,2-diphenyl-1,2-ethylenediamine with 3-formyl-4-hydroxy-benzyltriphenylphosphonium chloride was reported by Zeinab Mokhtarzadeh et al.104 They have synthesized three cobalt(III) complexes from prepared ligand along with pyridine, imidazole, and methylimidazole, respectively (Fig. 14). The prepared ligand and their complexes were tested for antibacterial activity against Gram-positive bacteria Bacillus subtilis, Staphylococcus aureus, and Gram-negative bacteria Salmonella typhi, Escherichia coli. The antibacterial activity for free ligands was found lower than the metal complexes.
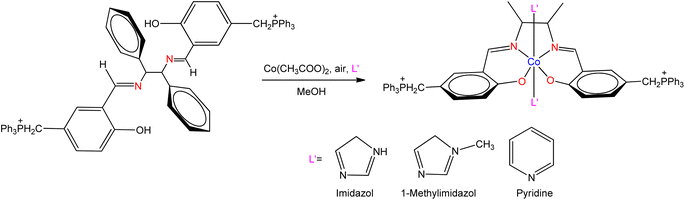 |
| Fig. 14 Schematic representation of SB and its Co(III) complexes containing phosphonium ion. | |
4.2 Anticancer activity
Cancer is one of the major health issues, a destructive, composite, antagonistic, miscellaneous, and alarming disease in which a group of cells exhibits abnormal growth, cause of death of millions of people worldwide and it is the most fatal disease after a cardiovascular one. Over the past two decades, the development of more successful drugs for treating cancer patients has been regarded as the main goal. The various transition metal SB complexes have been recognized in interrelation with anticancer properties.105 The water-soluble SB ligands were synthesized by the condensation of pyruvic acid with diaminoguanidine and triaminoguanidine as reported by S. Parveen et al.106 Furthermore, metal complexes were obtained from the hydrated salts of cobalt with the prepared SB ligand. The synthesized compounds exhibited anticancer activity against HeLa (human cervical carcinoma), and MCF-7 (human breast adenocarcinoma) using the MTT assay. For MCF-7 cells, the rate of cell hindrance rose with the increasing concentration from 0.25 μM to 100 μM with respective IC50 (half-maximal inhibitory concentration) values of 19.65 ± 0.56 and 12.86 ± 0.89 μM. The two obtained compounds were not poisonous to normal cells.
Narayanaperumal Pravin et al.107 derived water-soluble SB ligand from the condensation reaction of sodium 4-aminobenzenesulfonate with dibenzoylmethane, and also metal complexes were synthesized from the prepared ligand with Cu(II)/Zn(II) chloride in ethanol (Fig. 15). The derived complexes showed anticancer activity against in vitro cancer cell lines, specifically HeLa (human cervical carcinoma), MCF-7 (human breast adenocarcinoma), and HepG-2 (human liver carcinoma), along with NHDF (normal human dermal fibroblasts) operating colorimetric (MTT) assay. The Cu(II) complexes showed greater activity compared to the Zn(II) complexes.
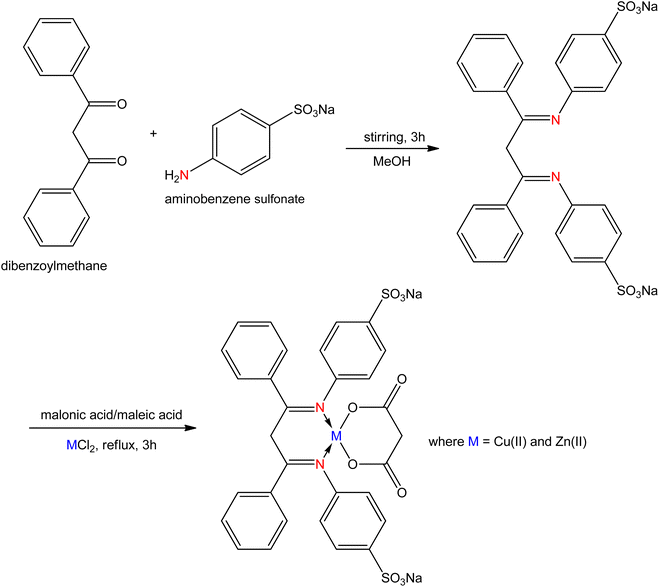 |
| Fig. 15 Synthetic route for water-soluble SB and their complexes containing sulfonate group. | |
Zahra Asadi et al.108 have synthesized a novel water-soluble SB ligand from the condensation reaction of (3-formyl-4-hydroxybenzyl)triphenylphosphonium chloride with phenylenediamine and zinc(II) and cobalt(II) complexes were derived from the obtained ligand (Fig. 16). In these cases, water solubility of the metal complexes greatly enhanced by introducing a lipophilic triphenylphosphonium cation to the aromatic portion of Schiff base. All the synthesized SB compounds appeared anticancer activity against three cancer cell lines, namely Jurkat human T cell leukemia, Raji Burkitt's lymphoma, and A549 lung carcinoma. This study uncovered that PPh3+ groups within the ligand are considered as important parts in binding with the nucleotide phosphate unit of the DNA backbone.
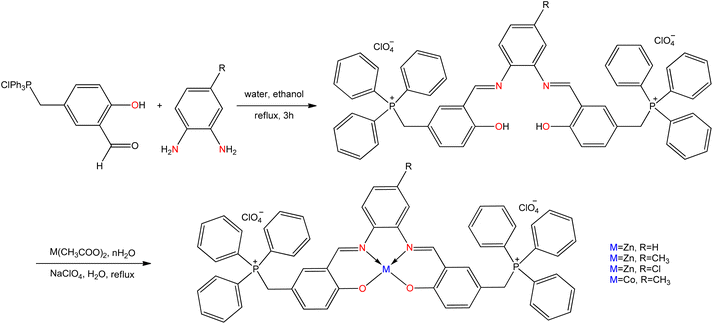 |
| Fig. 16 Synthesis of Schiff base ligand and their metal complexes containing PPh3+ ion. | |
Zn(II), Cu(II), Ni(II), and Mn(II) complexes were synthesized from a Schiff base ligand (Fig. 17) obtained from the condensation reaction of 3,4-diaminobenzophenone with sodium salicylaldehyde-5-sulfonate by M. Asadi et al.109 These compounds were found to be water soluble and the hydrophilic group like (–SO3Na) was identified for this solubility. All the coordinated compounds exhibited anticancer activity against the K562 leukemia cell line. The greater concentration of the metal complexes disclosed remarkable anti-proliferation activity against the cell line. The results for cytotoxicity of metal complexes were presented in the following order [Mn] > [Cu] > [Zn] > [Ni] using the IC50 values.
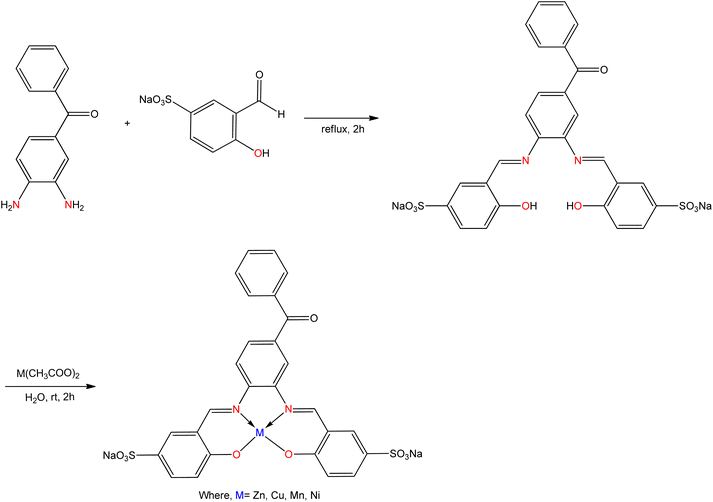 |
| Fig. 17 Structure of water-soluble SB complexes containing sulphonate group. | |
The water-soluble thiosemicarbazone ligand, 4-hydroxyl-3-({[(methylamino)carbonothioyl]hydrazono}methyl)benzoic acid was prepared from the condensation reaction of 3-formyl-4-hydroxy-benzoic acid with 4-methyl-3-thiosemicarbazide in methanol by Soheila Jenabi Sardroud et al.110 Also, metal complexes were synthesized from the prepared ligand and different salts of Cu(II), Mn(II), Ni(II), Fe(II), and Zn(II). The synthesized metal complexes were exhibited anticancer activity against two cancer cell lines, namely MCF-7 (human breast carcinoma cell line) and K562 (human chronic myelogenous leukemia) cells. All the complexes exhibited greater anticancer activity than the ligands. Among the complexes, the copper(II) complexes were evaluated for the most cytotoxicity against both cell lines (Fig. 18).
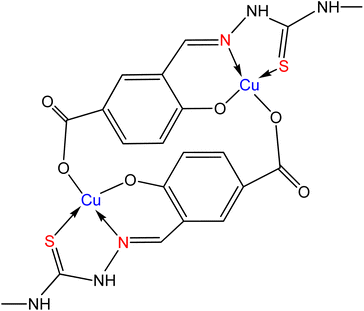 |
| Fig. 18 Cu(II) complex of water-soluble thiosemicarbazone ligand. | |
Azadeh Mirzaahmadi et al.111 have synthesized water-soluble SB metal complexes from the synthesized ligand and Cu(II) salts (Fig. 19). Here, the ligands were derived from the condensation reaction of 3-(3-formyl-4-hydroxybenzyl)-1-methyl-1H-imidazole-3-ium chloride with thiosemicarbazide, 4-methyl-3-thiosemicarbazide, and 4-phenyl-3-thiosemicarbazide. All the synthesized compounds exhibited anticancer activity against the MCF-7 cell line. The Cu(II) complexes exhibited the highest anticancer activity. The anticancer activity of this complex was due to the presence of imidazolium functional group on the synthesized ligand.
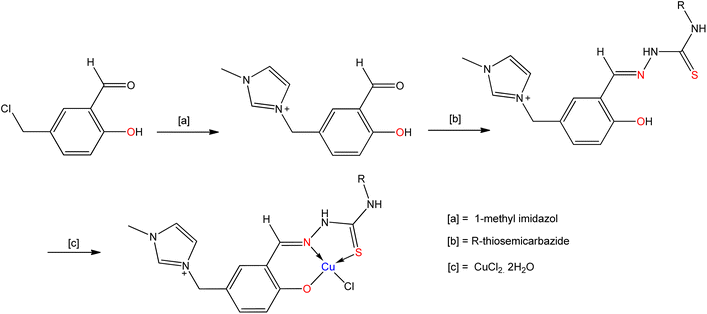 |
| Fig. 19 Reaction pathway of water-soluble thiosemicarbazone ligand and its metal complexes. | |
Burcu Saygideğer Demir et al.112 reported a water-soluble SB ligand derived from the condensation of 4-amino-3-hydroxynaphthalene-1-sulfonic acid with 2-hydroxy-3-methoxybenzaldehyde, and their Cu(II), Ni(II), and Zn(II) metal complexes (Fig. 20) were synthesized. The synthesized compounds and ligands were exhibited anticancer activity against A549 cancer cells. According to IC50 values, both Cu(II) complex (12 μM) and Zn(II) complex (80 μM) were found to be very effective on A549 cells. Among these complexes, Cu(II) complex showed the best cytotoxic effect on A549 cells, although the prepared ligand and Ni(II) complex were not evaluated the cytotoxicity effect on A549. In the toxicity study, the water solubility of the synthesized compounds was shown to have an outstanding advantage over the other solvents. The greater anticancer activities of these compounds are due to their higher solubilities.
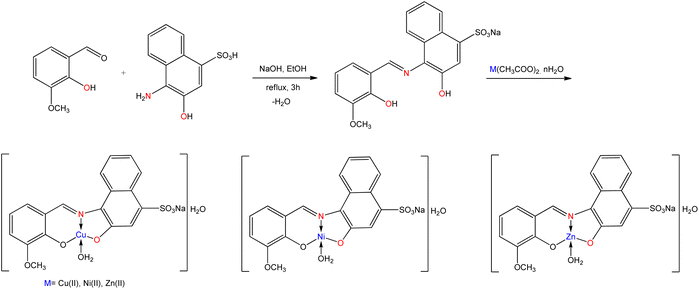 |
| Fig. 20 Synthetic pathway of water-soluble Schiff base ligand and metal complexes containing sulfonate group. | |
Li-Jun Li et al.113 have synthesized a series of water-soluble SB ligands and their Pt(II) metal complexes (Fig. 21). Here, the ligands were synthesized from the condensation reaction of salicylaldehyde and 5-bromosalicylaldehyde with L-amino acid: glycine, phenylalanine, serine, threonine, and leucine. All the coordinated complexes were exhibited their cytotoxic activity against cell lines of HL-60, KB, BGC-823, and Bel-7402 according to the MTT assay. It was observed that, water-soluble complexes showed the better cytotoxicity and the greater antitumor activity compared with cisplatin.
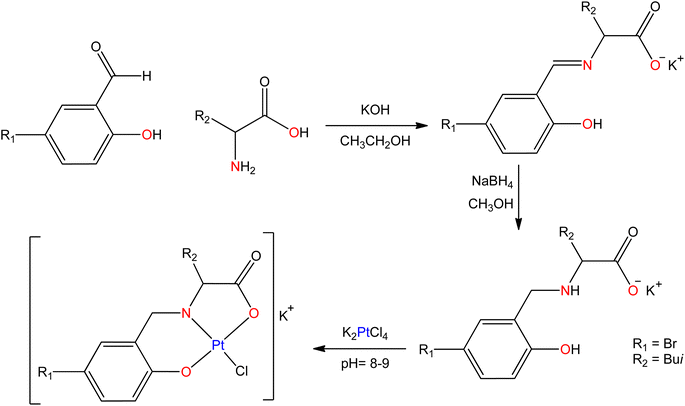 |
| Fig. 21 Synthetic pathway of water-soluble Pt(II) complexes of amino acid SB. | |
The two-water soluble copper(II) complexes were prepared and characterized by Jing Lu et al.114 The prepared complexes (Fig. 22) exhibited anticancer activity against a 3-cell line panel consisting of HeLa (human cervical carcinoma), MCF-7 (human breast adenocarcinoma), and A549 (lung adenocarcinoma carcinoma), respectively. The IC50 values (0.43–1.85 μM) also indicated that both complexes showed the highest cytotoxicity in all three cell lines.
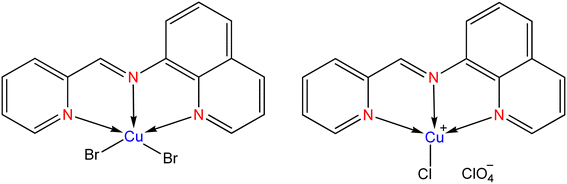 |
| Fig. 22 Water-soluble copper(II) complexes of 2-((quinolin-8-ylimino)methyl)pyridine ligand. | |
4.3 DNA binding and cleavage
DNA (deoxyribonucleic acid) is a self-replicating material that exists in all living organisms and is a storage site of cellular information. It is accessed continuously for storing and dispensing information required for existence. DNA is the main target molecule for most anticancer and antiviral therapies. The researcher inquired how DNA interacts with small molecules to give a product, which is important in the design of new types of pharmacological drugs. In the past decades, the binding and cleavage activities of transition metal complexes were discovered and are now widely studied in bioengineering. Literature exploration reveals that a huge number of Schiff base complexes were regarded as excellent DNA cleavage and binding.115,116 Several research groups are interested in taking part in developing new therapeutic reagents and DNA probes from water-soluble SB metal complexes. Three water-soluble mixed ligand Cu(II) complexes (Fig. 23) obtained from pyridoxal (vitamin B6 component), ethanol amine (1), bipyridine (2), and 1,10-phenanthroline (3) were synthesized by B. Annaraj et al.117 These copper complexes were analyzed by microanalytical, spectroscopic, and single-crystal X-ray diffraction studies. The derivative of pyridoxal in the coordination of copper complexes exhibited the binding constants (Kb) and quenching constants (Kq) for the interaction of complexes (a), (b), and (c) under the physiological environment with calf thymus (CT) DNA and BSA. The binding of metal complexes with CT DNA was examined using the absorption spectroscopic method. The cleavage properties of metal complexes and the distillation of plasmid DNA, pUC19 were studied by agarose gel electrophoresis. In the absence of additives, the synthesized complexes were upgraded to DNA cleavage in the following order: (b) > (a) > (c).
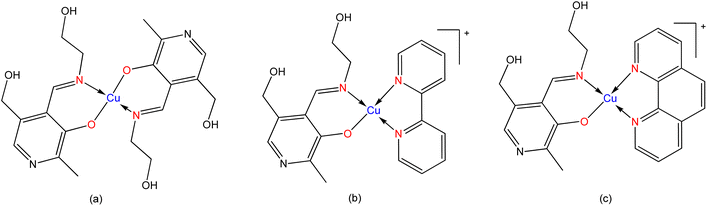 |
| Fig. 23 The general structure of water-soluble copper(II) complexes. | |
The water-soluble SB metal complexes were synthesized from the ligand and nitrate salt of nickel and copper by Dong-Dong Qin et al.118 Here, the ligand was derived from the condensation reaction of phenol and diethylene-triamine (Fig. 24), and sequentially its Cu(II) and Ni(II) complexes were synthesized. The interaction of copper and nickel complexes with DNA (biopolymer) has been investigated by fluorescence spectrometry and UV-vis spectroscopy. In the addition of DNA, the expanded emission intensities of the Ni(II) and Cu(II) complexes are about 1.76 and 1.23 times greater, respectively, than those in the free of DNA. The binding constant data indicated that the hydrophobic part of the DNA helix lessens the mobility and vibration of both complexes. Both complexes with calf thymus DNA exhibited different hypochromic responses. The different spectral variations of both complexes containing aromatic chromophores interacted with the nucleic acid bases.
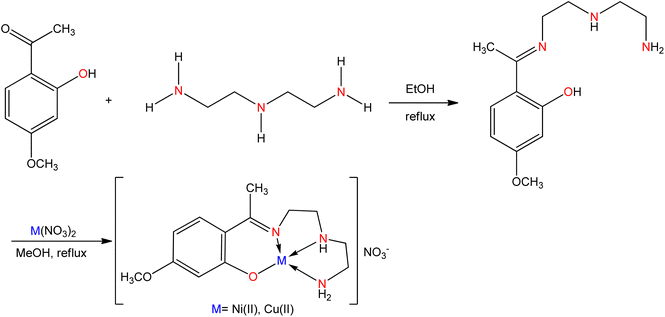 |
| Fig. 24 Synthetic pathway of water-soluble SB ligands and their Ni(II) and Cu(II) complexes. | |
The interaction of water-soluble SB cobalt(II) complex (Fig. 25) from with calf thymus DNA has been observed by Nahid Shahabadi et al.119 where the SB ligand was derived from the condensation of 3-formyl-4-hydroxybenzyl-triphenyl phosphonium chloride with o-phenylenediamine. The Schiff base ligand and cobalt(II) complex were able to execute DNA cleavage (pUC18 plasmid DNA) which was detected by agarose gel electrophoresis. With the increasing concentration of SB and its cobalt complex, the intensity band of the complex also increased.
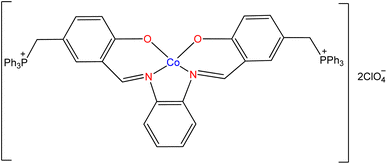 |
| Fig. 25 The structure of the water-soluble cobalt(II) SB complex containing PPh3 group. | |
Alessio Terenzi et al.120 observed that the nickel(II) and a copper(II) Schiff base salen-like cationic complexes (Fig. 26) interacted toward duplex and G-quadruplex DNA. Those were also compared with reference nickel(II) compound containing the naphthalene group, and their affinity was decreased toward G-quadruplex DNA.
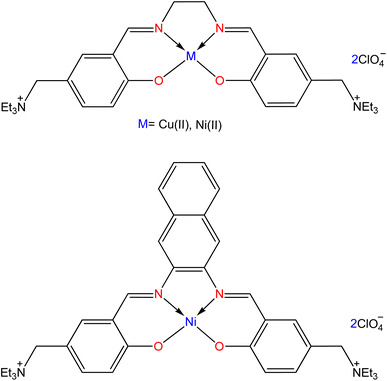 |
| Fig. 26 Synthetic route of Cu(II) and Ni(II) complexes containing salen type SB ligand. | |
4.4 Antioxidant activity
An antioxidant is a substance, which is used to deactivate or preserve free radicals before they attack cells. The radical of DPPH (2,2-diphenyl-1-picrylhydrazyl) is widely used for studying radical scavenging activity in the chemical analysis.121 A series of water-soluble SB complexes were synthesized by Zhao-Guo Hong et al.122 where the ligands were derived from the condensation of 5-sulfosalicylaldehyde with the (R/S)-1,2-diaminopropane-(+)-tartrate (Fig. 27).
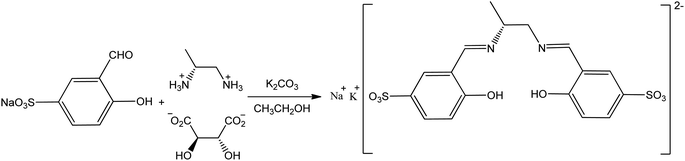 |
| Fig. 27 Synthesis of water soluble chiral/achiral Schiff base ligand. | |
All the synthesized compounds were exhibited antioxidant activity. The scavenging activity of the complexes and a hybrid biopolymer protein were assessed using the 2,2′-azino-bis(3-ethylbenzothiazoline-6-sulfonic acid) (ABTS) and a modified indirect Fridovich method. The analyzed data exhibited that all the complexes possess scavenging activity. Among them, the complexes of S configuration with hybrid protein were somewhat greater than the R configuration containing metal complexes with hybrid protein.
Duraisamy Senthil Raja et al.123 prepared the SB ligand from the condensation reaction of isonicotinic acid hydrazide with 2-oxo-1,2-dihydroquinoline-3-carbaldehyde, and its cobalt(II) complexes (Fig. 28) were derived from the prepared ligand. The synthesized compounds were exhibited strong antioxidant activity. The free radical scavenging activity of cobalt(II) complexes have been tested for hydroxyl radicals (OH˙), DPPH radicals (DPPH˙), superoxide anion radicals (O2−˙) and nitric oxide (NO) as well as standards such as butylated hydroxyanisole (BHA) and butylated hydroxytoluene (BHT) in a cell-free system. According to the IC50 value, the order of free radical scavenging activity of the complexes is DPPH˙ > O2−˙> OH˙ > NO˙.
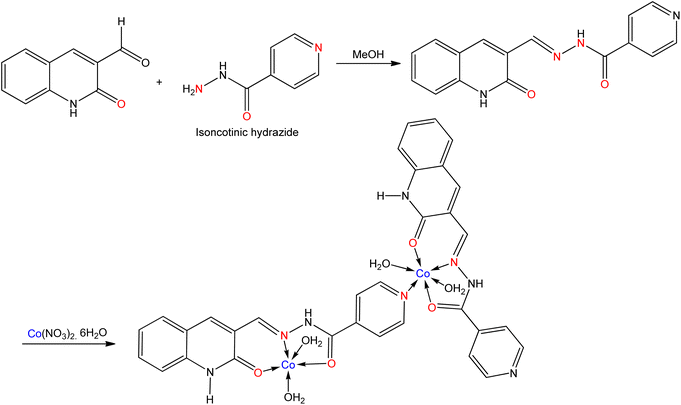 |
| Fig. 28 Synthetic route for water-soluble ligand-bridged cobalt(II) complex. | |
Zeng-Chen Liu et al.124 synthesized water-soluble SB ligands from 2-oxo-quinoline-3-carbaldehyde(4-hydroxybenzoyl)hydrazone, thiosemicarbazone, and two copper complexes (Fig. 29) were derived from the corresponding ligands and the structures of those complexes were characterized using single-crystal X-ray diffraction. Both complexes exhibited strong antioxidant activity and were determined in vitro by hydroxyl and superoxide radical scavenging methods. The measured antioxidant values of complexes including complex 1 (IC50 = 4 μM) and complex 2 (IC50 = 5 μM) were much better than standard antioxidants such as mannitol and vitamin C.
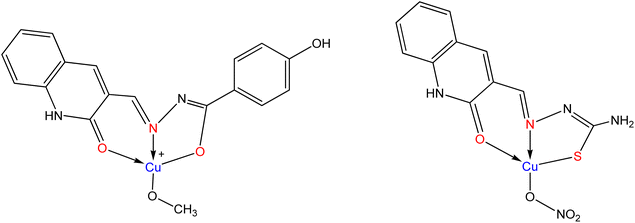 |
| Fig. 29 Structure of water-soluble Cu(II) complexes of 2-oxo-quinoline-3-carbaldehyde SB. | |
Jeyaraj Dhaveethu Raja et al.125 synthesized a Schiff base ligand (Fig. 30) from the condensation of salicylaldehyde with 4-(2-aminoethyl)-morpholine, and at least five metal complexes were synthesized from the ligands. The synthesized complexes exhibited antioxidant activity and their activities were determined in vitro by the 2,2-diphenyl-1-(2,4,6-trinitrophenyl)hydrazyl (DPPH) free radical scavenging assay. While DPPH reacts with the prepared compounds, it is reduced and the color changes (deep violet to light yellow) occur to form a saturated radical. The absorbance of each complex was determined using a UV-visible spectrophotometer at 517 nm.
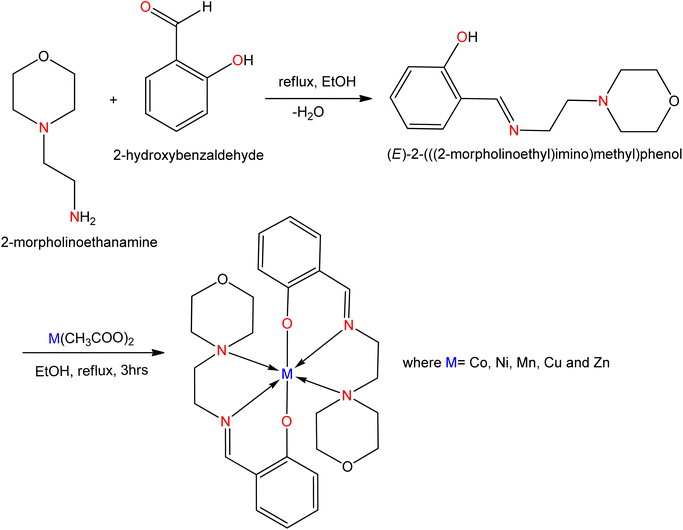 |
| Fig. 30 Synthesis of 2-[(2-morpholinoethylimino)methyl]phenol Schiff base and its metal complexes. | |
Water-soluble mixed-ligand nickel(II) complexes (Fig. 31) were synthesized by Gurumoorthy et al.126 in which the ligands were prepared from the condensation reaction of 2-((2-(piperazin-1-yl)ethylimino)methyl)-4-substituted phenols with diimine, 2,2′-bipyridyl (bpy) or 1,10-phenanthroline (phen). All the complexes were possessed to have greater scavenging activity against DPPH radicals compared to standard antioxidants like vitamin C and BHT.
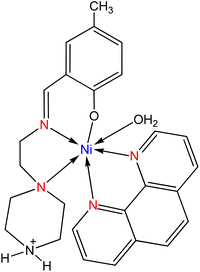 |
| Fig. 31 Water-soluble mixed-ligand nickel(II) complexes. | |
5. Conclusion and perspectives
Schiff base (SB) metal complexes are a significant class of compounds due to their structural diversity and various important biological properties. The major challenge for Schiff base ligands and their metal complexes to be used in various applications is their limited aqueous solubilities. Ligands and metal complexes having the ability to dissolve in an aqueous solvent greatly increase their applicability in therapeutic, medicinal, and catalytic activities. In addition, the incorporation of some cationic and anionic functional groups enhances their solubility. This review mainly focuses on some previously reported synthesis methods to produce water-soluble Schiff base ligands and metal complexes and the investigation of their catalytic and biological activities. The water-soluble ligands and metal complexes constantly attract interested researchers to achieve suggestive and incontestable access to various fields. The information provided in this review can be useful in the study of water-soluble Schiff base and metal complexes as well as their biological and catalytic activities with an update of recent research. Developing Schiff base chemistry could lead to the development of future research that produces Schiff-base modified nanoparticles or compounds.
Conflicts of interest
The authors have declared there exist no competing interests.
Acknowledgements
The authors are grateful to the Department of Chemistry, Rajshahi University, Rajshahi, Bangladesh. Thanks are also due for the financial aid funded by UGC project, Begum Rokeya University, Rangpur, Bangladesh.
References
- A. Kanwal, B. Parveen, R. Ashraf, N. Haider and K. G. Ali, J. Coord. Chem., 2022, 75, 2533–2556 CrossRef CAS.
- A. A. A. Abu-Hussen, J. Coord. Chem., 2006, 59(2), 157–176 CrossRef CAS.
- M. T. H. Tarafder and M. A. Ali, Can. J. Chem., 1980, 58, 727–732 CrossRef.
- N. H. Patel, H. M. Parekh and M. N. Patel, Transit. Met. Chem., 2005, 30(1), 13–17 CrossRef CAS.
- M. T. H. Tarafder, M. A. Ali and D. J. Wee, et al., Transit. Met. Chem., 2000, 25, 456–460 CrossRef CAS.
- V. Ambike, S. Adsule, F. Ahmed, Z. Wang, Z. Afrasiabi, E. Sinn, F. Sarkar and S. Padhye, J. Inorg. Biochem., 2007, 101(10), 1517–1524 CrossRef CAS.
- R. Katwal, H. Kaur and B. K. Kapur, Sci. Rev. Chem. Commun., 2013, 3(1), 1–15 CAS.
- S. Omidi and A. Kakanejadifard, RSC Adv., 2020, 10, 30186–30202 RSC.
- O. Gungor and P. Gurkan, Spectrochim. Acta, Part A, 2010, 77, 304–311 CrossRef.
- N. R. Bader, Rasayan J. Chem., 2010, 3(4), 660–670 CAS.
- K. J. Devi, M. Yadav, D. Kumar, L. S. Naik and D. K. Jindal, Appl. Organomet. Chem., 2019, 33(10), e5154 CrossRef.
- K. C. Gupta and K. Sutar, Coord. Chem. Rev., 2008, 252, 1420–1450 CrossRef CAS.
- M. A. Latif, T. Ahmed, M. S. Hossain, B. M. Chaki, A. Abdou and M. Kudrat-E-Zahan, Russ. J. Gen. Chem., 2023, 93, 389–397 CrossRef CAS.
- M. S. Hossain, K. A. Khushy, M. A. Latif and M. Kudrat-E-Zahan, et al., Russ. J. Gen. Chem., 2022, 92, 2723–2733 CrossRef CAS.
- M. A. Latif, T. Tofaz, B. M. Chaki, M. S. Hossain, H. M. T. Islam and M. Kudrat-E-Zahan, Russ. J. Gen. Chem., 2019, 89(6), 1197–1203 CrossRef CAS.
- H. Molaee, M. Sahihi, M. Moghadam, V. Mirkhani, S. Tangestaninejad, I. Mohammadpoor-Baltork and H. A. Rudbari, J. Biomol. Struct. Dyn., 2019, 37(3), 641–648 CrossRef CAS.
- Z. Guo, R. Xing, S. Liu, Z. Zhong, X. Ji, L. Wang and P. Li, Carbohydr. Res., 2007, 342, 1329–1332 CrossRef CAS PubMed.
- E. Yousif, A. Majeed, K. Al-Sammarrae, N. Salih, J. Salimon and B. Abdullah, Arab. J. Chem., 2017, 10(2), S1639–S1644 CrossRef CAS.
- Y.-W. Dong, R.-Q. Fan, P. Wang, L. G. Wei, X. M. Wang, H. J. Zhang, S. Gao, Y. L. Yang and Y. L. Wang, Dalton Trans., 2015, 44, 5306 RSC.
- A. Charles and K. Sivaraj, Res. J. Life Sci., Bioinf., Pharm. Chem. Sci., 2019, 5(2), 982–992 CAS.
- H. Naeimi, Z. S. Nazifi, S. M. Amininezhad and M. Amouheidari, J. Antibiot., 2013, 66, 687–689 CrossRef CAS.
- S. Arulmurugan, H. P. Kavitha and B. R. Venkatramanet, Rasayan J. Chem., 2010, 3(3), 385–410 Search PubMed.
- R. Antony, T. Arun and S. T. D. Manickam, Int. J. Biol. Macromol., 2019, 129, 615–633 CrossRef CAS PubMed.
- A. Nagajothi, A. Kiruthika, S. Chitra and K. Parameswari, Res. J. Chem. Sci., 2013, 3(2), 35–43 CAS.
- S. Kumar, M. S. Niranjan, K. C. Chaluvaraju, C. M. Jamakhandi and D. Kadadevar, Int. J. Curr. Pharm. Res., 2010, 1, 39–42 Search PubMed.
- A. M. Channa, A. N. Siyal, S. Q. Memon and S. Parveen, Desalin. Water Treat., 2014, 57(8), 3664–3673 CrossRef.
- F. K. Soomro, S. Q. Memon, N. Memon and M. Y. Khuhawar, Polym. Bull., 2019, 77, 2367–2383 CrossRef.
- M. T. Kaczmarek, M. Zabiszak, M. Nowak and R. Jastrzab, Coord. Chem. Rev., 2018, 370, 42–54 CrossRef CAS.
- P. Das and W. Linert, Coord. Chem. Rev., 2015, 311, 1–23 CrossRef.
- D. M. Boghaei and M. Gharagozlou, J. Coord. Chem., 2007, 60(3), 339–346 CrossRef CAS.
- I. Turel, Molecules, 2015, 20, 7951–7956 CrossRef CAS.
- W. A. Zoubi, S. G. Mohamed, A. A. S. Al-Hamdani, A. P. Mahendradhany and Y. G. Ko, RSC Adv., 2018, 8, 23294 RSC.
- L. H. Abdel-Rahman, A. M. Abu-Dief, R. M. El-Khatib and S. M. Abdel-Fatah, Bioorg. Chem., 2016, 69, 140–152 CrossRef CAS PubMed.
- S. Nagar, S. Raizada and N. Tripathee, Results Chem., 2023, 6, 101153 CrossRef CAS.
- A. de Moura, J. B. Júnior and A. C. S. Carvalho, et al., J. Therm. Anal. Calorim., 2022, 147, 11093–11106 CrossRef CAS.
- J. J. Boruah, Z. S. Bhatt, C. R. Nathani, V. J. Bambhaniya, A. K. Guha and S. P. Das, J. Coord. Chem., 2021, 74, 2055–2068 CrossRef CAS.
- S. S. Jawoor, S. A. Patil, M. Kumbar and P. B. Ramawadagi, J. Mol. Struct., 2018, 1164, 378–385 CrossRef CAS.
- V. K. Rao, S. S. Reddy, B. S. Krishna, K. R. M. Naidu, C. N. Raju and S. K. Ghosh, Green Chem. Lett. Rev., 2010, 3(3), 217–223 CrossRef CAS.
- T. A. Yousef, J. Mol. Struct., 2020, 1215(1–10), 128180 CrossRef CAS.
- A. Jain, S. De and P. Barman, Res. Chem. Intermed., 2022, 48, 2199–2251 CrossRef CAS.
- B. W. A. Herrmann and C. W. Kohlpaintner, Angew. Chem., Int. Ed. Engl., 1993, 32(11), 1524–1544 CrossRef.
- M. Fleck, D. Karmakar, M. Ghosh, A. Ghosh, R. Saha and D. Bandyopadhyay, Polyhedron, 2012, 34, 157–162 CrossRef CAS.
- K. Ghosh, A. Banerjee, A. Bauza, A. Frontera and S. Chattopadhyay, RSC Adv., 2018, 8, 28216–28237 RSC.
- L. C. Matsinha, J. Mao, S. F. Mapolie and G. S. Smith, Eur. J. Chem., 2015, 24, 4088–4094 Search PubMed.
- M. Tulu, N. M. Aghatabay, M. Senel, C. Dizman, T. Parali and B. Dulger, Eur. J. Med. Chem., 2009, 44(3), 1093–1099 CrossRef CAS PubMed.
- T. Akitsu and Y. Einaga, Polyhedron, 2005, 24(18), 2933–2943 CrossRef CAS.
- S. A. Talouki, G. Grivani, P. Crochet and V. Cadierno, Inorg. Chim. Acta, 2017, 456, 142–148 CrossRef.
- L. Zhou, P. Cai, Y. Fenzg, J. Cheng, H. Xiang, J. Liu, D. Wu and X. Zhou, Anal. Chim. Acta, 2012, 735, 96–106 CrossRef CAS PubMed.
- L. M. Wu, H. B. Teng, X. C. Feng, X. B. Ke, Q. F. Zhu, J. T. Su, W. J. Xu and X. M. Hu, Cryst. Growth Des., 2007, 7(7), 1337–1342 CrossRef CAS.
- S. A. H. Yazdi, A. Mirzaahmadi, A. A. Khandar, V. Eigner, M. Dušek, F. Lotfipour, M. Mahdavi, S. Soltani and G. Dehghan, Inorg. Chim. Acta, 2017, 458, 171–180 CrossRef.
- M. Ebrahimpour and M. Behzad, J. Coord. Chem., 2011, 68(8), 1426–1435 CrossRef.
- D. F. Evans and D. A. Jakubovic, Polyhedron, 1988, 7, 1881–1889 CrossRef CAS.
- D. M. Boghaei and M. Gharagozlou, J. Coord. Chem., 2007, 60(3), 339–346 CrossRef CAS.
- Z. Asadi, M. Asadi and M. R. Shorkaei, J. Iran. Chem. Soc., 2016, 13, 429–442 CrossRef CAS.
- M. Reimann, D. R. Salmon, J. T. Horton, E. C. Gier and L. R. Jefferies, ACS Omega, 2019, 4(2), 2874–2882 CrossRef CAS PubMed.
- D. Moreno, V. Daier, C. Palopoli, J. Tuchagues and S. Signorella, J. Inorg. Biochem., 2010, 104(5), 496–502 CrossRef CAS PubMed.
- C. Palopoli, N. Bruzzo, C. Hureau, S. Ladeira, D. Murgida and S. Signorella, Inorg. Chem., 2011, 50(18), 8973–8983 CrossRef CAS PubMed.
- C. Palopoli, C. Duhayon, J. P. Tuchagues and S. Signorella, Dalton Trans., 2014, 43, 17145–17155 RSC.
- L. Bai, F. Tao, L. Li, A. Deng, C. Yan, G. Li and L. Wang, Spectrochim. Acta, Part A, 2019, 214, 436–444 CrossRef CAS PubMed.
- T. Fang, L. Z. Fu, L. L. Zhou, S. Z. Zhan and S. Chen, Electrochim. Acta, 2015, 178, 368–373 CrossRef CAS.
- D. F. Evans and P. H. Missen, J. Chem. Soc., Dalton Trans., 1985, 7, 1451–1454 RSC.
- R. F. M. Elshaarawy, R. Ali, S. M. Saleh and C. Janiak, J. Mol. Liq., 2017, 241, 308–315 CrossRef CAS.
- Y. N. Xiao and C. X. Zhan, J. Appl. Polym. Sci., 2002, 84(5), 887–893 CrossRef CAS.
- G. J. Colpas, B. J. Hamstra, J. W. Kampf and V. L. Pecoraro, Inorg. Chem., 1994, 33(21), 4669–4675 CrossRef CAS.
- M. Manimohon, S. Pugalmani and M. A. Sithique, J. Inorg. Organomet. Polym. Mater., 2020, 30, 4481–4495 CrossRef.
- K. Zhang, C. Zhang and K. Xie, Text. Res. J., 2015, 85(16), 1704–1711 CrossRef CAS.
- O. Acet, T. Baran, D. Erdonmez, N. H. Aksoy, I. Alacabey, A. Mentes and M. Odabasi, J. Chromatogr. A, 2018, 1550, 21–27 CrossRef CAS.
- T. Baran, A. Mentes and H. Arslan, Int. J. Biol. Macromol., 2015, 72, 94–103 CrossRef CAS PubMed.
- N. Shahabadi, Z. Ghasemian and S. Hadidi, Bioinorg. Chem. Appl., 2012, 126451 Search PubMed.
- D. M. Boghaei, E. Askarizadeh and A. Bezaatpour, Spectrochim. Acta, Part A, 2008, 69(2), 624–628 CrossRef.
- B. Bahramian, V. Mirkhani, M. Moghadam and A. H. Amin, Appl. Catal., A, 2006, 315, 52–57 CrossRef CAS.
- N. Shahabadi, S. Kashanian and F. Darabi, DNA Cell Biol., 2009, 28(11), 589–596 CrossRef CAS.
- Y. P. Wang, Y. N. Xiao, C. X. Zhang and R. M. Wang, J. Macromol. Sci., Part A: Pure Appl.Chem., 2007, 38(11), 1099–1104 CrossRef.
- B. Korthals, I. Göttker-Schnetmann and S. Mecking, Organometallics, 2007, 26(6), 1311–1316 CrossRef CAS.
- A. Hasnaoui, R. Idouhli, A. Nayad, H. Ouahine, M. Khadiri, A. Abouelfida, L. Elfirdoussi and M. A. Alia, Inorg. Chem. Commun., 2020, 119, 108134 CrossRef CAS.
- D. Osypiuk, B. Cristóvão and A. Bartyzel, Crystals, 2020, 10(11), 1004 CrossRef CAS.
- M. Theetharappan and M. A. Neelakantan, J. Fluoresc., 2021, 31(5), 1277–1290 CrossRef CAS PubMed.
- W. A. Herrmann and C. W. Kohlpaintner, Angew Chem. Int. Ed. Engl., 1993, 32, 1524–1544 CrossRef.
- T. Katsuki, RSC Adv., 2004, 33(7), 437–444 CAS.
- I. Iwakura, T. Ikeno and T. Yamada, Org. Lett., 2004, 6(6), 949–952 CrossRef CAS PubMed.
- S. Jammi, P. Saha, S. Sanyashi, S. Sakthivel and T. Punniyamurthy, Tetrahedron, 2008, 64(51), 11724–11731 CrossRef CAS.
- A. M. Abu-Dief and I. M. A. Mohamed, Beni-Suef Univ. J. Basic Appl. Sci., 2015, 4(2), 119–133 Search PubMed.
- R. M. Wang, C. J. Hao, Y. P. Wang and S. B. Li, J. Mol. Catal. A: Chem., 1999, 147, 173–178 CrossRef CAS.
- T. Ohkuma, N. Utsumi, K. Tsutsumi, K. Murata, C. Sandoval and R. Noyori, J. Am. Chem. Soc., 2006, 128(27), 8724–8725 CrossRef CAS.
- J. S. M. Samec, J. E. Backvall, P. G. Andersson and P. Brandtb, Chem. Soc. Rev., 2006, 35(3), 237–248 RSC.
- C. Li, Chem. Rev., 2005, 105(8), 3095–3165 CrossRef CAS.
- U. Koelle, Coord. Chem. Rev., 1994, 135, 623–650 CrossRef.
- L. Canali and D. C. Sherrington, Chem. Soc. Rev., 1999, 28(2), 85–93 RSC.
- V. C. Gibson and S. K. Spitzmesser, Chem. Rev., 2003, 103(1), 283–315 CrossRef CAS.
- C. M. Che and J. S. Huang, Coord. Chem. Rev., 2003, 242, 97–113 CrossRef CAS.
- Y. Qu, F. Ke, L. Zhou, Z. Li, H. Xiang, D. Wuab and X. Zhou, Chem. Commun., 2011, 47(13), 3912–3914 RSC.
- M. Dauchy, M. Ferreira, J. Leblond, H. Bricout, S. Tilloy, G. S. Smith and E. Monflier, Pure Appl. Chem., 2018, 90(5), 845–855 CrossRef CAS.
- L. Yu, X. Zhou, D. Wu and H. Xiang, J. Organomet. Chem., 2012, 705, 75–78 CrossRef CAS.
- M. F. I. Al-Hussein and M. S. S. Adam, Appl. Organomet. Chem., 2020, 34(6), 1–22 CrossRef.
- O. Das and T. K. Paine, Dalton Trans., 2012, 41, 11476–11481 RSC.
- E. G. Percástegui, J. Mosquera, T. K. Ronson, A. J. Plajer, M. Kieffer and J. R. Nitschke, Chem. Sci., 2019, 10, 2006–2018 RSC.
- L. M. D. R. S. Martins and A. J. L. Pomberio, Eur. J. Inorg. Chem., 2016, 2016, 2236–2252 CrossRef CAS.
- E. G. Percástegui, T. K. Ronson and J. R. Nitschke, Chem. Rev., 2020, 120(24), 13480–13544 CrossRef PubMed.
- A. D. M. Mohamad, E. R. Shrkawya, M. Al-Husseind and M. S. S. Adam, J. Taiwan Inst. Chem. Eng., 2020, 113, 27–45 CrossRef CAS.
- S. A. H. Yazdi, A. Mirzaahmadi, A. A. Khandar, V. Eigner, M. Dušek, M. Mahdavi, S. Soltani, F. Lotfipour and J. White, Polyhedron, 2017, 124, 156–165 CrossRef.
- C. Shiju, D. Arish and S. Kumaresan, J. Mol. Struct., 2020, 1221, 128770 CrossRef CAS.
- S. Lal, S. Arora, S. Rani, P. Kumar, P. Dabas and J. Malik, J. Macromol. Sci., Part A: Pure Appl.Chem., 2020, 57(12), 791–799 CrossRef CAS.
- M. Murugaiyan, S. P. Manib and M. A. Sithique, New J. Chem., 2019, 43(24), 9540–9554 RSC.
- Z. Mokhtarzadeh, M. Behzad, A. Khaleghian and A. Bezaatpour, ChemXpress, 2013, 4(2), 225–232 Search PubMed.
- A. Arunadevi and N. Raman, J. Coord. Chem., 2020, 73(15), 2095–2116 CrossRef CAS.
- S. Parveen, G. Velmurugan, E. Sinn, P. Venuvanalingam and S. Govindarajan, J. Photochem. Photobiol., B, 2018, 189, 152–164 CrossRef CAS.
- N. Pravin, G. Kumaravel, R. S. kumar and N. Raman, Appl. Organomet. Chem., 2017, 31(10), 1–17 CrossRef.
- Z. Asadi, E. Haddadi and M. Sedaghat, J. Photochem. Photobiol., A, 2017, 337, 140–150 CrossRef CAS.
- M. Asadi, Z. Asadi, S. B. Said, L. Zarei, F. M. Baig and Z. Amirghofran, Spectrochim. Acta, Part A, 2014, 122, 118–129 CrossRef CAS PubMed.
- S. J. Sardroud, S. A. Hosseini-Yazdi, M. Mahdavi, M. Poupon and E. Skorepova, Polyhedron, 2020, 175, 114218 CrossRef.
- A. Mirzaahmadi, S. A. Hosseini-Yazdi, E. Safarzadeh, B. Baradaran, E. Samolov and M. Dusek, J. Mol. Liq., 2019, 293, 111412 CrossRef CAS.
- B. S. Demiri, I. Gonul, G. G. Çelik, S. İpekbayrak and Y. Saygideger, ADYU J. Sci., 2020, 10(2), 441–454 Search PubMed.
- L. J. Li, C. Wang, C. Tian, X. Y. Yang, X. X. Hua and J. L. Du, Res. Chem. Intermed., 2013, 39(2), 733–746 CrossRef CAS.
- J. Lu, Q. Sun, J. L. Li, L. Jiang, W. Gu, X. Liu, J. L. Tian and S. P. Yan, J. Inorg. Biochem., 2014, 137, 46–56 CrossRef CAS PubMed.
- T. Rosu, E. Pahontu, C. Maxim, R. Georgescu, N. Stanica, G. L. Almajan and A. Gulea, Polyhedron, 2010, 29(2), 757–766 CrossRef CAS.
- B. Dede, I. Ozmen and F. Karipcin, Polyhedron, 2009, 28(18), 3967–3974 CrossRef CAS.
- B. Annaraj and M. A. Neelakantan, Eur. J. Med. Chem., 2015, 106, 1–8 CrossRef.
- D. D. Qin, Z. Y. Yang, G. F. Qi and T. R. Li, Transit. Met. Chem., 2009, 34(5), 499–505 CrossRef CAS.
- N. Shahabadi, S. Kashanian and F. Darabi, Eur. J. Med. Chem., 2010, 45(9), 4239–4245 CrossRef CAS.
- A. Terenzi, D. Lötsch, S. Schoonhoven, A. Roller, C. R. Kowol, W. Berger, B. K. Kepplera and G. Barone, Dalton Trans., 2016, 45(18), 7758–7767 RSC.
- J. Xie and K. M. Schaich, J. Agric. Food Chem., 2014, 62(19), 4251–4260 CrossRef CAS PubMed.
- Z. G. Hong, X. M. Zhang, T. X. Wu, C. Zheng, B. Luo, J. Tang, F.-P. Huang, D. Yao and H. D. Bian, Polyhedron, 2019, 159, 355–364 CrossRef CAS.
- D. S. Raja, N. S. P. Bhuvaneshb and K. Natarajan, Dalton Trans., 2012, 41(15), 4365–4377 RSC.
- Z. C. Liu, B. D. Wang, Z. Y. Yang, Y. Li, D. D. Qin and T. Li, Eur. J. Med. Chem., 2009, 44(11), 4477–4484 CrossRef CAS.
- J. D. Raja and K. Sakthikumar, J. Chem. Pharm. Res., 2015, 7(10), 23–34 CAS.
- P. Gurumoorthy, D. Mahendiran and A. K. Rahiman, Chem. Biol. Interact., 2016, 248, 21–35 CrossRef CAS.
|
This journal is © The Royal Society of Chemistry 2024 |
Click here to see how this site uses Cookies. View our privacy policy here.