DOI:
10.1039/D1RA01646F
(Paper)
RSC Adv., 2021,
11, 12249-12253
Transmembrane anion transport promoted by thioamides†
Received
2nd March 2021
, Accepted 19th March 2021
First published on 26th March 2021
Abstract
Thioamide groups represent useful hydrogen-bonding motifs for the development of active transmembrane anion transporters. Using a 1,8-di(thioamido)carbazole scaffold the superior performance of thioamides compared with the parent amides has been demonstrated.
Introduction
Small molecules capable of performing protein functions represent attractive minimalistic models to study cellular processes and are appealing for potential biological applications.1–3 In recent years, small molecules capable of facilitating the selective permeation of anions through lipid bilayers have emerged as promising candidates for the development of anticancer and antimicrobial agents as well as therapeutics for the treatment of channelopathies caused by faulty anion transport, such as cystic fibrosis, Dent's disease, and others.4–6
Transmembrane ion transport is a complex phenomenon influenced by a number of factors including binding energies and lipophilicity.7–9 Subtle changes in the molecular structure of anionophores often result in dramatic differences in their performance.10–12 Nevertheless, important insights into the design of this type of molecules have been obtained in recent years.13 Hydrogen bonding is the most frequently employed interaction in the design of synthetic anion transporters. Several hydrogen bond donors arranged in a convergent manner ensure effective binding between the transporter and the anion, thus forming a supramolecular complex capable of permeating the membrane. Ureas and thioureas are among the most successful anion binding motifs employed in this type of molecules.14,15 Thioureas usually outperform ureas as anion carriers because replacement of oxygen by sulfur improves the hydrogen bonding ability of the N–H groups, reduces tendency to self-assemble, and increases lipophilicity of the molecule. This strategy of using urea and thiourea derivatives has been extensively used to produce anionophores with increased activity.16 On the other hand, the potential of thioamides has remained largely unexplored despite a similar effect would be expected and amide groups are frequently employed in the construction of active anion receptors and transporters.17 In this paper, we explore the potential of the thioamide moiety in the development of active anionophores.
Results and discussion
We have recently reported a family of 1,8-diamidocarbazoles as anion receptors and identified compound 4A as a highly active anion carrier (Fig. 1).18 We envisaged the potential of this motif to prepare their thioamide derivatives and to explore their anion transport properties.
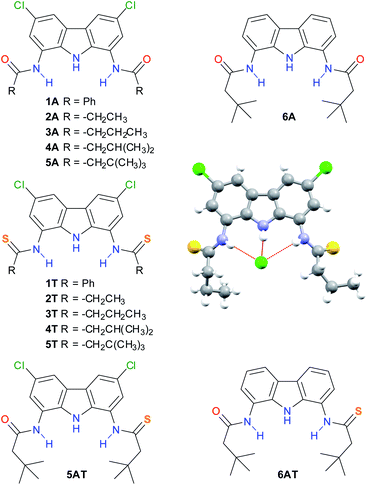 |
| Fig. 1 Compounds included in this study and solid-state X-ray structure of compound 3T complexing chloride (as TBACl; TBA cation omitted for clarity). | |
Thioamides 1T–5T as well as amido-thioamides 5AT and 6AT were synthesized from the corresponding 1,8-diamidocarbazoles by treatment with Lawesson's reagent and fully characterized (Fig. 1, see also ESI† for details).
1H NMR titrations of the thioamides with TBACl in DMSO-d6 + 0.5% H2O resulted in large downfield shifts of the carbazole NH protons (Δδmax from 1.83 to 2.55 ppm, except for the most weakly bonding 1T, for which Δδmax = 0.94 ppm was observed) as well as in much smaller downfield shifts of the thioamide NHs (0.42 < Δδmax < 0.65 ppm, again with the exception of 1T, where Δδmax = 0.89 ppm) (Fig. 2). In case of the amido-thioamides 5AT and 6AT, the CSNH protons shifted slightly upfield upon chloride binding (by 0.13–0.18 ppm), mirroring the behaviour of CONH protons of their parent diamide receptors.19 All these observations suggest that the hydrogen bonds formed with the anion by the central carbazole NHs are much stronger than the bonds formed by the (thio)amide side arms.
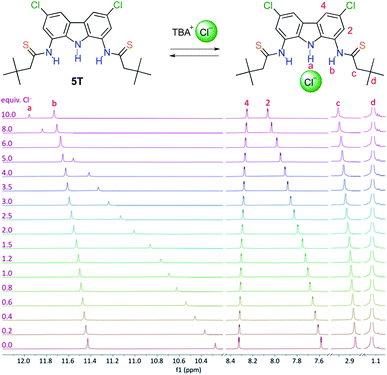 |
| Fig. 2 1H NMR titration of 5T (5 mM) with TBACl in DMSO-d6 + 0.5% H2O. T = 293 K. | |
This supposition was further corroborated by the X-ray crystal structure of the chloride complex of dithioamide 3T, in which the carbazole NH–Cl distance is much shorter (2.248 Å) than the two thioamide NH–Cl distances (2.581 Å and 2.830 Å), see Fig. 1. The inability of the thioamide arms to form shorter bonds with chloride might be related to the steric bulk of the sulfur atom, which enforces significant torsion of the thioamide arms with respect to the carbazole plane (44–46°) as well as to geometric mismatch between the relatively wide binding cavity of the receptor and the relatively small chloride anion.
Quantitative analysis of the titration data revealed that the association constants with chloride turned out to be 2–3 times smaller for thioamides in comparison to their amide precursors (Table 1). This is in contrast to what might have been expected based on the much higher acidity of thioamides,20 but in line with some literature precedents.21,22 Very likely, the beneficial effect of enhanced hydrogen bond donating ability of the thioamide moieties23 is counterbalanced here by unfavourable conformational preferences of the thioamides.24,25
Table 1 Association constants Ka for 1
:
1 complexes of compounds 1T–5T, 5AT and 6AT as well as their amide congeners (in brackets) with chloride (added as tetrabutylammonium salt) determined from 1H NMR titration experiments in DMSO-d6 + 0.5% H2O at 293 K and transport activities expressed as EC50 (nM)
Compound |
Ka (M−1) |
EC50 (nM) NO3−/Cl− |
EC50 (% molar) NO3−/Cl− |
EC50 (nM) HCO3−/Cl− |
EC50 (% molar) HCO3−/Cl− |
The association constants determined for parent bisamides were taken from ref. 18. The compound did not display enough activity to calculate an EC50 value. |
1T |
<10 (14)a |
—b |
—b |
—b |
—b |
2T |
49 (104)a |
297 |
0.059 |
4290 |
0.858 |
3T |
50 (109)a |
107 |
0.022 |
2910 |
0.582 |
4A |
123a |
184 |
0.037 |
—b |
—b |
4T |
46 |
66 |
0.013 |
385 |
0.077 |
5A |
159a |
—b |
—b |
—b |
—b |
5T |
52 |
93 |
0.019 |
465 |
0.093 |
5AT |
67 |
61 |
0.012 |
1719 |
0.344 |
6A |
48a |
—b |
—b |
—b |
—b |
6AT |
23 |
353 |
0.071 |
—b |
—b |
The anion transport properties of these compounds were investigated in POPC phospholipid vesicles using a chloride selective electrode.26 In these assays, the facilitated chloride efflux induced by aliquots of the compounds was monitored over time. All receptors were first screened at 2% carrier to POPC molar ratio and compounds eliciting more than 50% chloride efflux were further analysed at different concentrations. For these latter derivatives, the concentration-dependent chloride efflux activity was then fitted using the Hill equation to obtain the EC50 parameters representing the amount of compound needed to induce 50% efflux of the encapsulated chloride. All the experiments were repeated at least three times using different batches of vesicles (Fig. 3).
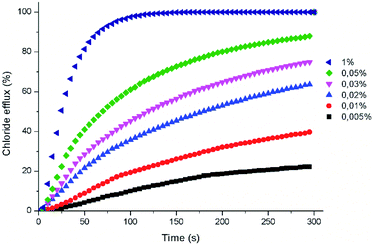 |
| Fig. 3 Chloride efflux promoted by 4T at different concentrations (1–0.005% carrier to POPC molar ratio, 5–0.025 μM) in unilamellar POPC vesicles. Vesicles loaded with 489 mM NaCl were buffered at pH 7.2 with 5 mM phosphate and dispersed in 489 mM NaNO3 buffered at pH 7.2. Each trace represents the average of at least three trials. | |
The positive effect of replacing the amide groups by the thioamide was evident with all 1,8-di(thioamido)carbazoles outperforming their parent 1,8-diamidocarbazoles. The least active derivative was found to be the 1,8-dibenzothioamido derivative 1T. For this compound, no EC50 could be calculated. The poor performance of transporters bearing aromatic substituents, such as 1T, is likely related to their much lower chloride affinity.18 Thioamides 2T–5T were found to be highly active in the chloride efflux assay with nitrate in the external solution, with EC50 values in the submicromolar range. The most active derivative was 4T, with a calculated EC50 of 67 nM. The positive effect of replacing even a single amide group by thioamide is demonstrated in the case of compounds 5AT and 6AT. Compound 5AT displayed transport efficacy rivalling the best performers identified in this study. Compound 6AT is an active transporter derived from a non-chlorinated carbazole core. None of the diamides derived from this core showed any chloride transport activity in our previous investigations.18
Experiments were also performed by suspending the vesicles in an external sulfate solution or an external mixture of sulfate and bicarbonate. These assays allow studying the impact of the anion composition of the external buffer on the observed chloride efflux. Bicarbonate is an anion of biological relevance and is significantly more hydrophilic than chloride and nitrate, thus more difficult to extract into the lipid bilayer. This was reflected in the higher calculated EC50 values. Only thioamides 4T and 5T displayed EC50 values in the submicromolar range under these conditions. No significant chloride efflux was detected in the presence of sulfate alone in the external milieu (see Fig. S56†). This result supports an exchange mechanism accounting for the transport activity displayed by these compounds, where the higher hydrophilicity of the doubly charged sulfate renders the compounds inactive as a result of their inability to facilitate the transport of this anion. This result also rules out any unspecific transport or membrane destabilization effect promoted by these compounds. The ability of some of these compounds to exchange chloride and bicarbonate bodes well for their potential biological applications.
Conclusions
In conclusion, we have demonstrated the usefulness of thioamides in the development of highly active transmembrane anion transporters.27 These compounds display enhanced transmembrane transport activity compared to the parent amide derivatives. Taking into account the widespread use of amides as hydrogen-bonding motifs in successful anionophores, it is expected that this strategy will allow the identification of new candidates with improved anion transport efficiency.
Experimental
N,N′-(3,6-Dichloro-9H-carbazole-1,8-diyl)dibenzothioamide (1T)
A 50 ml, 2-neck, round-bottomed flask was dried for 10 min with a stream of hot air (ca. 500 °C) from heat-gun and cooled down to rt in a desiccator. Then the flask was charged with diamide 1A (238 mg, 0.502 mmol), Lawesson's reagent (444 mg, 1.10 mmol) and a stir bar. Then the flask was equipped with a reflux condenser connected to a Schlenk line and its side neck was closed with a stopper. Air was removed by three pump/thaw cycles and then dry THF (30 ml) was added in a stream of argon through the side neck. The reaction mixture was intensively stirred and refluxed overnight. After this time the mixture was cooled down and 2.0 g of silica gel was added. Solvents were evaporated on a rotary evaporator and the solid residue was dried under high vacuum. Then the solid residue was suspended in hexane (40 ml) and put on top of a chromatographic column made of 110 g of silica suspended in CHCl3. The column was eluted with CHCl3 (ca. 500 ml), CHCl3
:
CH3CO2Et = 40
:
1 (ca. 500 ml) and CHCl3
:
CH3CO2Et = 30
:
1 until the desired product was completely washed out. Fractions containing pure product were combined and evaporated on a rotary evaporator yielding 293 mg (98.0%) of yellow solid. Mp: decomposition at 301 °C. 1H NMR (500 MHz, DMSO-d6) δDMSO: 11.69 (s, 2H, thioamide NHs), 11.23 (s, 1H, carbazole NH), 8.37 (bs, 2H, carbazole CH-4/5), 8.03 (d, J = 7.6 Hz, 4H, phenyl CH-ortho), 7.77 (bs, 2H, carbazole CH-2/7), 7.56 (t, J = 7.3 Hz, 2H, phenyl CH-para), 7.48 (t, J = 7.5 Hz, 4H, phenyl CH-meta). 13C NMR (126 MHz, DMSO-d6) δDMSO: 199.19, 141.09, 133.99, 131.30, 127.98, 125.66, 124.62, 124.46, 122.98, 119.23. Elemental analysis: calcd for C26H17Cl2N3S2: C, 61.66; H, 3.38; N, 8.30, found: C, 61.56; H, 3.52; N, 8.27. HR MS (TOF MS ES−) m/z calcd for C26H16Cl2N3S2−: 504.0163 found: 504.0168.
Compounds 2T–5T, 5AT and 6AT were prepared using similar procedures. Complete information is provided in the ESI.†
N,N′-(3,6-Dichloro-9H-carbazole-1,8-diyl)dipropanethioamide (2T)
Yellow solid, yield 79.7%. Mp: decomposition at 270 °C. 1H NMR (500 MHz, DMSO-d6) δDMSO: 11.53 (s, 2H, thioamide NH); 10.84 (s, 1H, carbazole NH); 8.31 (d, J = 2.0 Hz, 2H, carbazole CH); 7.75 (d, J = 2.0 Hz, 2H, carbazole CH); 2.88 (q, J = 7.5 Hz, 4H, methylene CH2); 1.35 (t, J = 7.5 Hz, 6H, methyl CH3). 13C NMR (126 MHz, DMSO-d6) δDMSO: 208.02, 133.48, 124.93, 124.47, 123.81, 122.99, 119.04, 38.84, 13.92. Elemental analysis: calcd for C18H17Cl2N3S2: C, 52.68; H, 4.18; N, 10.24, found: C, 52.64; H, 4.35; N, 10.09. HR MS (TOF MS ES−) m/z calculated for C18H16Cl2N3S2−: 408.0163 found: 408.0161.
N,N′-(3,6-Dichloro-9H-carbazole-1,8-diyl)dibutanethioamide (3T)
Yellow solid, yield 80.2%. M.p. decomposition at 282 °C. 1H NMR (500 MHz, DMSO-d6) δDMSO: 11.56 (s, 2H, thioamide NH), 10.64 (s, 1H, carbazole NH), 8.31 (d, J = 1.9 Hz, 2H, carbazole CH), 7.71 (d, J = 2.0 Hz, 2H, carbazole CH), 2.85 (t, 4H, J = 6.0 Hz, CH2C
S), 1.93–1.81 (m, 4H, CH2), 1.02 (t, J = 7.4 Hz, 6H, CH3). 13C NMR (126 MHz, DMSO-d6) δDMSO: 206.45, 133.45, 124.90, 124.54, 123.78, 123.06, 119.12, 47.69, 22.61, 13.27. Elemental analysis: calcd for C20H21Cl2N3S2: C, 54.79; H, 4.83; N, 9.58, found: C, 54.88; H, 5.06; N, 9.47. HR MS (TOF MS ES−) m/z calcd for C20H20Cl2N3S2−: 436.0476 found: 436.0472.
N,N′-(3,6-Dichloro-9H-carbazole-1,8-diyl)bis(3-methylbutanethioamide) (4T)
Yellow solid, yield 64.7%. 1H NMR (500 MHz, DMSO-d6) δDMSO: 11.57 (s, 2H, thioamide NH), 10.46 (s, 1H, carbazole NH), 8.33 (d, J = 1.5 Hz, 2H, carbazole CH-4), 7.66 (d, J = 1.5 Hz, 2H, carbazole CH-2), 2.75 (d, J = 7.2 Hz, 4H, CH2), 2.40–2.25 (m, 2H, CH), 1.03 (d, J = 6.6 Hz, 12H, CH3). 13C NMR (126 MHz, DMSO-d6) δDMSO 205.56, 133.46, 124.89, 124.66, 123.79, 123.15, 119.22, 54.88, 39.50, 29.07, 21.96. Elemental analysis: calcd for C22H25Cl2N3S2: C, 56.64; H, 5.40; N, 9.01, found: C, 56.75; H, 5.54; N, 8.94. HR MS (TOF MS ES−) m/z calcd for C22H24Cl2N3S2−: 464.0789 found: 464.0786.
N,N′-(3,6-Dichloro-9H-carbazole-1,8-diyl)bis(3,3-dimethylbutanethioamide) (5T)
Yellow solid, yield 95.1%. M.p. decomposition at 278 °C. 1H NMR (500 MHz, DMSO-d6) δDMSO: 11.43 (s; 2H; thioamide NH); 10.30 (s; 1H; carbazole NH); 8.33 (d; J = 2.0 Hz; 2H; carbazole CH-4/5); 7.59 (d; J = 2.0 Hz; 2H; carbazole CH-2/7); 2.85 (s; 4H; methylene CH2); 1.14 (s; 18H; t-butyl CH3). 13C NMR (126 MHz, DMSO-d6) δDMSO: 203.50, 133.43, 125.04, 124.75, 123.89, 123.17, 119.18, 59.12, 32.02, 29.77. Elemental analysis: calcd for C24H29Cl2N3S2: C, 58.29; H, 5.91; N, 8.50, found: C, 58.37; H, 6.06; N, 8.25. HR MS (TOF MS ES−) m/z calcd for C24H28Cl2N3S2−: 492.1102; found: 492.1113.
N-(3,6-Dichloro-8-(3,3-dimethylbutanethioamido)-9H-carbazol-1-yl)-3,3-dimethylbutanamide (5AT)
Yellow solid, yield 16.7%. 1H NMR (DMSO-d6) δDMSO: 11.55 (s, 1H, thioamides NH), 10.56 (s, 1H, carbazole NH), 10.00 (s, 1H, amide NH), 8.30 (d, J = 1.8 Hz, 1H, carbazole CH-5), 8.12 (d, J = 1.8 Hz, 1H, carbazole CH-4), 7.90 (d, J = 1.8 Hz, 1H, carbazole CH-2), 7.41 (d, J = 1.6 Hz, 1H, carbazole CH-7), 2.88 (s, 2H, CH2C
S), 2.35 (s, 2H, CH2C
O), 1.16 (s, 9H, t-Bu on the thioamide side), 1.08 (s, 9H, t-Bu on the amide side). 13C NMR (DMSO-d6) δDMSO: 203.85, 170.57, 133.40, 129.89, 125.06, 124.64, 124.59, 124.24, 124.00, 123.73, 122.96, 119.26, 117.74, 115.92, 58.90, 49.20, 32.07, 30.89, 29.78, 29.59. HR MS (TOF MS ES−): m/z calcd for C24H28Cl2N3OS−: 476.1330, found: 476.1331. Elemental analysis calcd for C24H29Cl2N3OS: C, 60.24; H, 6.11; N, 8.78; found: C, 60.61; H, 6.39; N, 8.39.
N-(8-(3,3-Dimethylbutanethioamido)-9H-carbazol-1-yl)-3,3-dimethylbutanamide (6AT)
Yellow solid, yield 40.3%, mp decomposition above 282 °C. 1H NMR (500 MHz, DMSO-d6) δDMSO: 11.47 (s; 1H; thioamide NH); 10.06 (s; 1H; carbazole NH); 9.95 (s; 1H; amide NH); 8.08 (d, J = 7.5 Hz, 1H; CH-5 on the thioamide side); 7.94 (d, J = 7.7 Hz, 1H; CH-4 on the amide side); 7.61 (dd; J1 = 7.8, J2 = 1.0 Hz; 1H; CH-2 on the amide side); 7.34 (d, J = 7.7, 1H; CH-7 on the thioamide side); 7.22 (t; J = 7.7 Hz; 1H; CH-6 on the thioamide side); 7.17 (t; J = 7.8 Hz; 1H; CH-3 on the amide side); 2.87 (s; 2H; methylene CH2 from the thioamide arm); 2.34 (s; 2H; methylene CH2 from the amide arm); 1.17 (s; 9H; t-butyl CH3 from the thioamide arm); 1.10 (s; 9H; t-butyl from the amide arm). 13C NMR (126 MHz, DMSO-d6) δDMSO: 202.76, 170.19, 134.29, 131.48, 124.60, 124.47, 124.10, 123.61, 123.40, 119.43, 119.07, 119.05, 118.28, 116.28, 58.89, 49.13, 31.91, 30.84, 29.85, 29.68. Elemental analysis: calcd for C24H31N3OS: C, 70.38; H, 7.63; N, 10.26, found: C, 70.07; H, 7.61; N, 10.05. HR MS (TOF MS ES−) m/z calcd for C24H30N3OS−: 408.2110 found: 408.2112.
Conflicts of interest
There are no conflicts to declare.
Acknowledgements
We thank the Polish National Science Centre for grant OPUS (2011/01/B/ST5/03900) and Consejería de Educación de la Junta de Castilla y León (project BU067P20). Part of this study was carried out at the Biological and Chemical Research Centre, University of Warsaw, established within a project co-financed by the European Union through the European Regional Development Fund under the Operational Programme Innovative Economy 2007–2013. The authors gratefully acknowledge Andrea Sancho-Medina and Óscar Fernández-Ramos for their contributions to transmembrane anion transport experiments.
Notes and references
- A. S. Grillo, A. M. SantaMaria, M. D. Kafina, A. G. Cioffi, N. C. Huston, M. Han, Y. A. Seo, Y. Y. Yien, C. Nardone, A. V. Menon, J. Fan, D. C. Svoboda, J. B. Anderson, J. D. Hong, B. G. Nicolau, K. Subedi, A. A. Gewirth, M. Wessling-Resnick, J. Kim, B. H. Paw and M. D. Burke, Science, 2017, 356, 608–616 CrossRef CAS PubMed.
- S. Zheng, L. Huang, Z. Sun and M. Barboiu, Angew. Chem., Int. Ed., 2021, 60, 566–597 CrossRef CAS PubMed.
- F.-F. Shen, S.-Y. Dai, N.-K. Wong, S. Deng, A. S.-T. Wong and D. Yang, J. Am. Chem. Soc., 2020, 142, 10769–10779 CrossRef CAS PubMed.
- V. Kaushik, J. Yakisich, A. Kumar, N. Azad and A. Iyer, Cancers, 2018, 10, 360 CrossRef CAS PubMed.
- J. T. Davis, P. A. Gale and R. Quesada, Chem. Soc. Rev., 2020, 49, 6056–6068 RSC.
- N. Akhtar, O. Biswas and D. Manna, Chem. Commun., 2020, 56, 14137–14153 RSC.
- H. Li, H. Valkenier, A. G. Thorne, C. M. Dias, J. A. Cooper, M. Kieffer, N. Busschaert, P. A. Gale, D. N. Sheppard and A. P. Davis, Chem. Sci., 2019, 10, 9663–9672 RSC.
- I. Marques, P. M. R. Costa, M. Q. Miranda, N. Busschaert, E. N. W. Howe, H. J. Clarke, C. J. E. Haynes, I. L. Kirby, A. M. Rodilla, R. Pérez-Tomás, P. A. Gale and V. Félix, Phys. Chem. Chem. Phys., 2018, 20, 20796–20811 RSC.
- N. J. Knight, E. Hernando, C. J. E. Haynes, N. Busschaert, H. J. Clarke, K. Takimoto, M. García-Valverde, J. G. Frey, R. Quesada and P. A. Gale, Chem. Sci., 2016, 7, 1600–1608 RSC.
- X. Wu, A. M. Gilchrist and P. A. Gale, Chem, 2020, 6, 1296–1309 CAS.
- L. Martínez-Crespo, J. L. Sun-Wang, P. Ferreira, C. F. M. Mirabella, G. Aragay and P. Ballester, Chem.–Eur. J., 2019, 25, 4775–4781 CrossRef PubMed.
- H. Valkenier, O. Akrawi, P. Jurček, K. Sleziaková, T. Lízal, K. Bartik and V. Šindelář, Chem, 2019, 5, 429–444 CAS.
- X. Wu, E. N. W. Howe and P. A. Gale, Acc. Chem. Res., 2018, 51, 1870–1879 CrossRef CAS PubMed.
- H. Valkenier, C. M. Dias, K. L. Porter Goff, O. Jurček, R. Puttreddy, K. Rissanen and A. P. Davis, Chem. Commun., 2015, 51, 14235–14238 RSC.
- M. J. Spooner, H. Li, I. Marques, P. M. R. Costa, X. Wu, E. N. W. Howe, N. Busschaert, S. J. Moore, M. E. Light, D. N. Sheppard, V. Félix and P. A. Gale, Chem. Sci., 2019, 10, 1976–1985 RSC.
- P. A. Gale, J. T. Davis and R. Quesada, Chem. Soc. Rev., 2017, 46, 2497–2519 RSC.
- N. J. Andrews, C. J. E. Haynes, M. E. Light, S. J. Moore, C. C. Tong, J. T. Davis, W. A. Harrell Jr and P. A. Gale, Chem. Sci., 2011, 2, 256–260 RSC.
- K. M. Bąk, K. Chabuda, H. Montes, R. Quesada and M. J. Chmielewski, Org. Biomol. Chem., 2018, 16, 5188–5196 RSC.
- The rather uncommon upfield shifts of the (thio)amide protons upon chloride binding might be rationalised in terms of their engagement in strong hydrogen bonding interactions with solvent molecules prior to the addition of chloride.
- F. G. Bordwell, J. Am. Chem. Soc., 1988, 110, 5903–5904 CrossRef CAS.
- S. J. Coles, P. A. Gale, M. B. Hursthouse, M. E. Light and C. N. Warrimer, Supramol. Chem., 2004, 16, 469–486 CrossRef CAS.
- T. Zieliński and J. Jurczak, Tetrahedron, 2005, 61, 4081–4089 CrossRef.
- H.-J. Lee, Y.-S. Choi, K.-B. Lee, J. Park and C.-J. Yoon, J. Phys. Chem. A, 2002, 106, 7010–7017 CrossRef CAS.
- T. Zieliński, M. Kędziorek and J. Jurczak, Chem.–Eur. J., 2008, 14, 838–846 Search PubMed.
- I. Sandler, F. A. Larik, N. Mallo, J. E. Beves and J. Ho, J. Org. Chem., 2020, 85, 8074–8084 CrossRef CAS PubMed.
- L. A. Jowett and P. A. Gale, Supramol. Chem., 2019, 31, 297–312 CrossRef CAS.
- K. M. Bąk, B. van Kolck, K. Maslowska-Jarzyna, P. Papadopoulou, A. Kros and M. J. Chmielewski, Chem. Commun., 2020, 56, 4910–4913 RSC.
Footnote |
† Electronic supplementary information (ESI) available: Compound characterization data and anion transport assays. CCDC 2027226. For ESI and crystallographic data in CIF or other electronic format see DOI: 10.1039/d1ra01646f |
|
This journal is © The Royal Society of Chemistry 2021 |
Click here to see how this site uses Cookies. View our privacy policy here.