DOI:
10.1039/D1RA04887B
(Review Article)
RSC Adv., 2021,
11, 29130-29155
A review on convenient synthesis of substituted coumarins using reuseable solid acid catalysts
Received
23rd June 2021
, Accepted 19th August 2021
First published on 1st September 2021
Abstract
Due to growing concern about chemicals and their impact on the environment, cleaner reaction conditions are needed to be incorporated into chemical synthetic procedures. Recently, the use of heteropolyacid catalysts, mainly reuseable solid acid catalysts, has gained a leading role in organic synthesis due to their environmental and economic considerations and industrial utilization. The high catalytic activity, moisture sensitivity, reusability and inexpensive makes solid supported reagents attractive substituents to conventional Lewis acids. Nowadays synthesis of coumarins and their derivatives has attracted considerable attention from organic and medicinal chemists for many years as a large number of natural products contain this heterocyclic nucleus. In continuation with our investigations into the synthesis of substituted coumarins and due to several advantages of heterogeneous catalysts viz. cost-effective, no side products, high yield of desired products and no toxic waste material, here we report a new approach for the synthesis of substituted coumarins using solid acid catalysts.
Introduction
Coumarins as a major class of natural and synthetic products exhibit a variety of pharmacological and biological activities.1–3 There is growing curiosity for coumarins and their derivatives due to their anti-HIV, anti-oxidant, anti-fungal, antihelmintic and antibacterial properties.4–8 They are used in food and cosmetic industries as additive and also found applications as insecticides, optical brighteners, fluorescent and laser dyes.9–13 Masesane et al.14 reported the synthesis of chromane derivatives through the reaction of salicylaldehyde and enolates and they found that reactions of salicylaldehyde and enolates give nearly optically pure chromane derivatives. Coumarins can also be prepared by various methods viz. Pechmann condensation, Perkin, Knoevenagel and Reformatsky reactions.15 Pechmann condensation has been most popularly method for coumarin synthesis, since it proceeds from simple substrate viz. phenol and β-ketoester and gives excellent yields of coumarins. Pechmann condensation utilizes various catalysts viz. sulphuric acid, trifluoroacetic acid, phosphorous pentaoxide, ZrCl4, TiCl4 and ionic liquids, which have many drawbacks such as long reaction time, use of hazardous solvents, creates side products and salt waste due to acid neutralization.16 There has been some effort to find alternative, eco-friendly synthetic methods. Nowadays, the use of heterogeneous solid acid catalysts has fascinated significant attention. These catalysts have some advantages such as ease of product work-up, recyclability, strong safety and tolerance for wide range of temperature and pressures.17–21 Naikwadi and his coworkers22 reported the catalytic reaction of active methylene compounds with cyclic enol ethers and aryl acetals through oxonium intermediate under solvent-free conditions using heterogeneous solid acid catalysts and they found that Amberlyst-15 gave excellent yields of alkylated products. Therefore, there is a propensity to replace the classic homogeneous catalysts by heterogeneous solid acid catalysts. Due to several benefits of heterogeneous catalysts, in this review we encapsulate synthesis of substituted coumarins using solid acid catalyst.
Synthesis of substituted coumarins using solid acid catalysts
An efficient and facile synthesis of novel class of coumarin-containing secondary benzamide derivatives (4) has been developed via one-pot condensation of 5,7-dihydroxy coumarins (1), substituted aldehydes (2) and benzamide (3) using tungstate sulphuric acid by Karami and his coworkers (Scheme 1).23 To standardize the reaction conditions, a reaction between 5,7-dihydroxy-4-methylcoumarin, benzaldehyde and benzamide were chosen as a model reaction. The model reaction was screened under various conditions. After conducting several experiments, they found that the desired reaction took place efficiently using 1 mol% of tungstate sulphuric acid (TSA) at 120 °C under solvent-free conditions. The proposed mechanism of the formation of desired products is shown in Fig. 1. According to proposed mechanism, first there is formation of adduct (I) by the condensation reaction of substituted aldehyde and benzamide in the presence of TSA as an efficient proton source. Then C-8 of coumarin attacks on adduct (I) and gives intermediate (II). Finally by tautomerization desired product obtained. They also found that tungstate sulphuric acid is reuseable heterogeneous catalyst, which make this procedure mild, convenient and eco-friendly. Simplicity of procedure, use of safe and recyclable catalysts, high yields and short reaction times are some beauties of present methodology.
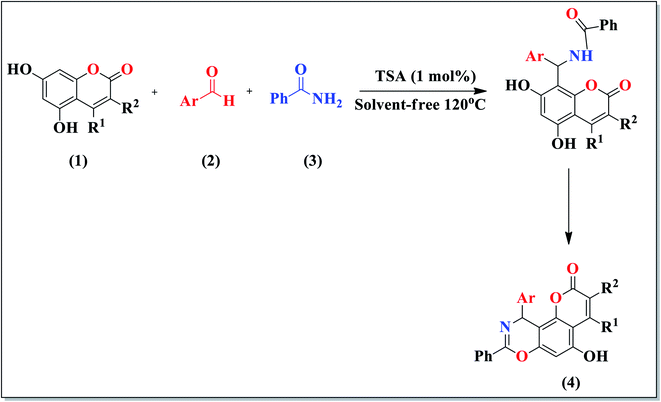 |
| Scheme 1 TSA-catalyzed synthesis of coumarin-containing secondary benzamides. | |
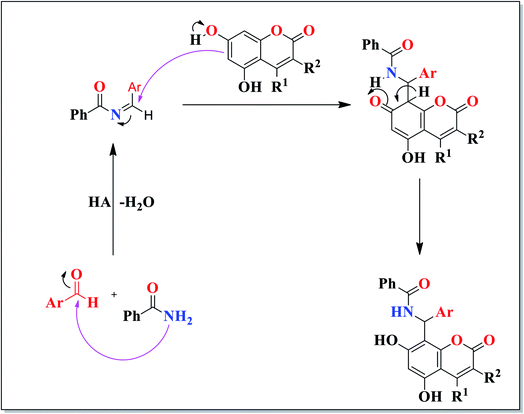 |
| Fig. 1 Possible mechanism for synthesis of coumarin-containing secondary benzamides. | |
Khaligh et al. found that poly(4-vinylpyridinium) hydrogen sulfate solid acid was efficient catalyst for the synthesis of substituted coumarins (7) via Pechmann condensation reaction between substituted phenols (5) and β-ketoester (6) using ultrasound irradiation at ambient temperature. Simplicity in operation, avoid use of toxic catalysts and solvents, excellent yield of desired products, reuse of catalyst are some merits of present methodology. First they standardized the reaction conditions by exploring model reaction between resorcinol and ethylacetoacetate (Scheme 2)24 in presence of different solvents viz. toluene, methanol, ethanol and dichloromethane under reflux reaction conditions as well as solvent-free medium at variety of temperature with PVPHS as the catalyst. The results are presented in Table 1.
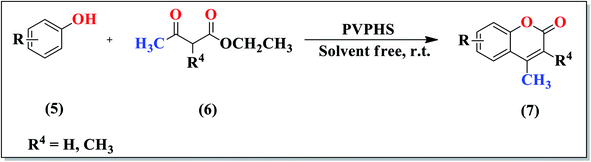 |
| Scheme 2 The synthesis of substituted coumarins in presence PVPHS at room temperature under ultrasound irradiation and solvent-free conditions. | |
Table 1 Effect of temperature, solvent, amount of catalyst on the synthesis of substituted coumarins
Entry |
Amount of catalyst (mg) |
Temperature (°C) |
Solvent |
Time (min) |
Yield (%) |
1 |
— |
60 |
Clean |
360 |
Nil |
2 |
10 |
Reflux |
C6H5CH3 |
60 |
72 |
3 |
10 |
Reflux |
CH3OH |
60 |
66 |
4 |
10 |
Reflux |
C2H5OH |
60 |
68 |
5 |
10 |
Reflux |
CH2Cl2 |
60 |
70 |
6 |
10 |
60 |
Clean |
60 |
88 |
7 |
10 |
70 |
Clean |
60 |
92 |
8 |
10 |
80 |
Clean |
60 |
94 |
9 |
5 |
70 |
Clean |
60 |
69 |
From Table 1 it was observed that resorcinol conversion increased with increase in temperature up to 80 °C. There was no significant difference in conversion between 70 and 80 °C (Table 1, entries 6–8). The yield of desired product decreased with decreasing of catalyst amount (Table 1, entry 9) and no reaction took place in the absence of catalyst after 6 h of reaction time (Table 1, entry 1).
Further, they also observed that PVPHS employed under ultrasonic irradiation showed a more effective catalytic activity in comparison with the stirring at room temperature in terms of yield and reaction time (Table 2, entries 2 and 3).
Table 2 Reaction of resorcinol and ethylacetoacetate in the presence of different amount of PVPHS
Entry |
Amount of catalyst (mg) |
Room temperature |
Ultrasonic irradiation |
Time (h) |
Yield (%) |
Time (min) |
Yield (%) |
1 |
— |
6 Trace |
|
60 44 |
|
2 |
5 |
2 32 |
|
15 86 |
|
3 |
10 |
2 48 |
|
5 96 |
|
The plausible mechanism for the synthesis of substituted coumarins in the presence of 7-hydroxy-4-methylcoumarin in the presence of PVPHS as a promoter under ultrasound irradiation is shown in Fig. 2.
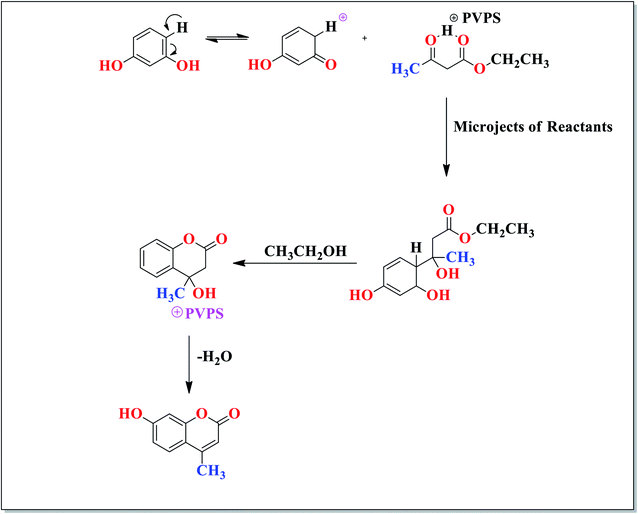 |
| Fig. 2 Proposed mechanism for Pechmann reaction of resorcinol with ethyl acetoacetate at room temperature under ultrasonic irradiation. | |
Akbari et al. reported the synthesis of bis-coumarin (9) in excellent yield via reaction between substituted aldehydes (2) and 4-hydroxycoumarin (8) in water under microwave irradiation in the presence of Fe3O4@sulfosalicylic acid magnetic nanoparticles as solid acid catalyst (Scheme 3).25 Less reaction time, excellent yields of desired products, avoid the use of hazardous or toxic reagent and solvents, thermal durability, easy separation and high reusability are main attractive characteristics of current methodology. First, they explored the model reaction between benzaldehyde and 4-hydroxycoumarin and studied the effect of different reaction conditions. The results are summarized in Table 3. The results show that the highest yield and lowest time of reaction were obtained when the reaction was performed in the presence of 0.05 g of sulfosalicylic acid magnetic nanoparticles under microwave irradiation at 180 W in water as green solvent (Table 3, entry 9).
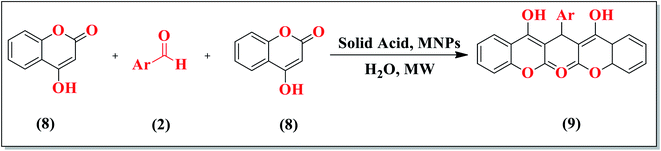 |
| Scheme 3 Synthesis of bis-coumarin derivatives in Fe3O4 @sulfosalicylic acid MNPs as catalyst under microwave irradiation in water. | |
Table 3 Optimization of the model reaction
Entry |
Catalyst (g) |
Power |
Time (min) |
Yield (%) |
1 |
Sulfosalicylic acid (0.01) |
180 |
15 |
75 |
2 |
FeCl3·6H2O (0.05) |
180 |
15 |
43 |
3 |
Bulk-Fe3O4 (0.05) |
180 |
15 |
50 |
4 |
Nano-Fe3O4 (0.05) |
180 |
15 |
68 |
5 |
Fe3O4@sulfosalicylic acid (0.03) |
180 |
20 |
89 |
6 |
Fe3O4@sulfosalicylic acid (0.03) |
300 |
10 |
92 |
7 |
Fe3O4@sulfosalicylic acid (0.05) |
100 |
10 |
89 |
8 |
Fe3O4@sulfosalicylic acid (0.05) |
180 |
10 |
96 |
9 |
Fe3O4@sulfosalicylic acid (0.08) |
180 |
10 |
96 |
10 |
Fe3O4@sulfosalicylic acid (0.015) |
180 |
10 |
80 |
11 |
— |
180 |
20 |
30 |
The possible reaction mechanism for the synthesis of bis-coumarin via Knoevenagel condensation is depicted in Fig. 3. First there is activation of substituted aldehyde by the acid catalyst and after that activated aldehyde react with 4-hydroxycoumarin to give an α,β-unsaturated intermediate. Then, there is Michael addition of the 4-hydroxycoumarin with an α,β-unsaturated intermediate to give the final polyhydroquinoline product. Finally, a tautomeric proton shift produces the desired product. Table 4 presented the results from the synthesis of bis-coumarin by reaction of benzaldehyde and 4 hydroxycoumarin in the presence of Fe3O4@sulfosalicylic acid magnetic nanoparticles which has been compared with the other methods reported in literature. The results show that the present method is preferable because of its reaction times and efficiency.
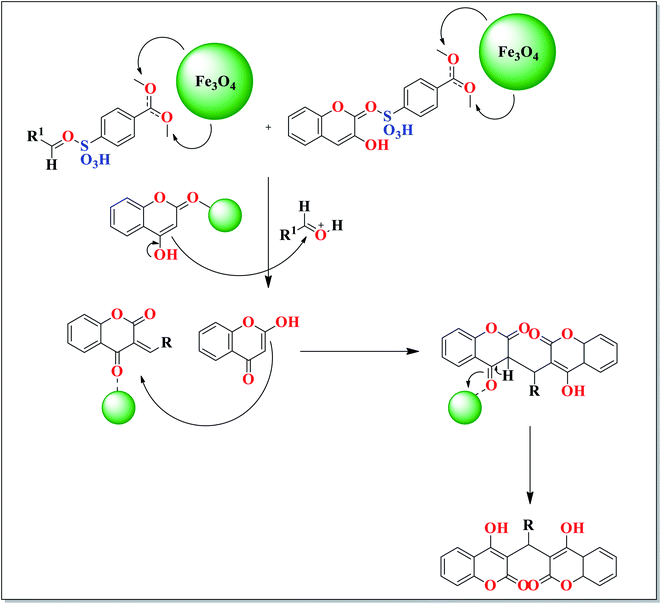 |
| Fig. 3 Proposed mechanism for the synthesis of biscoumarin derivatives in the presence of Fe3O4@sulfosalicylic acid magnetic nanoparticles. | |
Table 4 Comparison of efficiency of present catalyst with other catalysts reported in literature
Entry |
Catalyst/condition |
Time (min) |
Yield |
References |
1 |
Ionic liquids, reflux |
260 |
84 |
26 |
2 |
Choline hydroxide, reflux |
240 |
86 |
27 |
3 |
No catalyst/trifluoroethanol, reflux |
360 |
80 |
28 |
4 |
Fe3O4@sulfosalicylic acid/H2O, MW |
10 |
96 |
25 |
Samiei et al. reported the green synthesis coumarin derivatives (7) via Pechmann condensation reaction between substituted phenols (5) and β-ketoesters (6) in excellent yield under solvent-free conditions in presence of novel sulfonated carbon-coated magnetic nanoparticles (Scheme 4).29
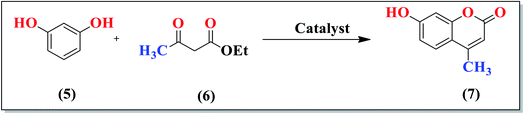 |
| Scheme 4 Synthesis of substituted coumarins. | |
For optimization of reaction conditions, first the model reaction was explored between resorcinol and ethyl acetoacetate to produce 7-hydroxy-4-methylcoumarin. The reaction was also optimized with respect to various parameters viz. catalyst loading, different temperatures and various solvents as shown in Table 5. It was observed from Table 5 that lack of catalyst and also with a catalyst loading of Fe3O4 NPs, CCMNPs (Fe3O4@C) led to no product even after 6 h, while the use of SCCMNPs (Fe3O4@C@OSO3H) could produce related 4H-coumarin in a good yield during the short time. Hence, SCCMNP with the sulfonic acid moiety on the surface of MNP was introduced as an effective catalyst in the Pechmann condensation. They also found that 6.5 mol% catalyst loading was identified as an optimized concentration in the model reaction at 120 °C under solvent-free condition.
Table 5 The effect of various solvents, temperature and catalyst loadings for the synthesis of substituted coumarins through Pechmann condensation
Entry |
Catalyst loading |
Solvent |
T (°C) |
Time (min) |
Yield (%) |
1 |
— |
— |
120 |
360 |
No reaction |
2 |
Fe3O4 NPs (6.5 mol%) |
— |
120 |
360 |
No reaction |
3 |
CCMNPs (Fe3O4@C) (6.5 mol%) |
— |
120 |
360 |
No reaction |
4 |
SCCMNPs (Fe3O4@C@OSO3H) (3.25 mol%) |
— |
120 |
30 |
86 |
5 |
SCCMNPs (Fe3O4@C@OSO3H) (6.5 mol%) |
— |
120 |
20 |
98 |
6 |
SCCMNPs (Fe3O4@C@OSO3H) (13 mol%) |
— |
120 |
20 |
98 |
7 |
SCCMNPs (Fe3O4@C@OSO3H) (6.5 mol%) |
— |
100 |
30 |
87 |
8 |
SCCMNPs (Fe3O4@C@OSO3H) (6.5 mol%) |
— |
90 |
40 |
83 |
9 |
SCCMNPs (Fe3O4@C@OSO3H) (6.5 mol%) |
H2O |
Reflux |
360 |
Trace |
10 |
SCCMNPs (Fe3O4@C@OSO3H) (6.5 mol%) |
Toluene |
Reflux |
360 |
No reaction |
11 |
SCCMNPs (Fe3O4@C@OSO3H) (6.5 mol%) |
CH2Cl2 |
Reflux |
360 |
No reaction |
12 |
SCCMNPs (Fe3O4@C@OSO3H) (6.5 mol%) |
EtOH |
Reflux |
360 |
No reaction |
13 |
SCCMNPs (Fe3O4@C@OSO3H) (6.5 mol%) |
CH3CN |
Reflux |
360 |
No reaction |
The comparison of catalytic activity of present catalyst with other catalysts reported in literature was shown in Table 6.
Table 6 Comparison of activity of some heterogeneous solid acid catalysts for the synthesis of substituted coumarins
Entry |
Catalyst |
Catalyst (mol%) |
T (°C) |
Time (min) |
Yield (%) |
References |
1 |
Fe3O4-DABCO |
1 |
100 |
40 |
93 |
30 |
2 |
γ-Fe3O4@HAp–Ag |
10 |
80 |
20 |
95 |
31 |
3 |
Fe3O4@SiO2@PrSO3H |
2 |
130 |
25 |
96 |
32 |
4 |
CMK-15-SO3H |
3 |
130 |
20 |
95 |
33 |
5 |
Random pore carbon-SO3H |
7 |
130 |
60 |
90 |
34 |
6 |
Fe3O4@SiO2@EtSO3H |
75 |
90 |
90 |
93 |
35 |
7 |
SnClx–SiO2 |
5 |
120 |
35 |
90 |
36 |
8 |
SBA-15-Ph-Pr–SO3H |
7 |
130 |
60 |
90 |
36 |
9 |
ZrW2 |
20 |
12 |
120 |
94 |
37 |
10 |
SnW2 |
20 |
120 |
120 |
88 |
37 |
11 |
Nanosponge MFI zeolite |
0.5 |
130 |
120 |
94 |
38 |
12 |
TiZnO |
10 |
110 |
180 |
85 |
39 |
13 |
Fe3O4@Boehmite–NH2–CoII |
6.6 |
90 |
30 |
95 |
40 |
14 |
SCCMNPs |
6.5 |
120 |
20 |
98 |
29 |
Khan and his coworkers reported the synthesis of coumarins (7) via Pechmann condensation reaction between substituted phenols (5) and β-ketoesters (6) in presence of zirconia-based heterogeneous catalyst (Scheme 5).41 First of all model reaction was carried out between resorcinol and ethyl acetoacetate without a catalyst at 80 °C, but there will be no formation of product as shown in Table 7. They also observed that excellent yield of product was obtained when electron releasing group linked with substituted phenols, while poor yield of product was obtained when electron withdrawing group linked with substituted phenols. They also studied reaction between resorcinol and ethyl acetoacetate with 50 mg of the catalyst ZrO2–TiO2 in polar solvent viz. ethanol and non-polar solvent viz. toluene by varying the temperature condition as shown in Table 8. The plausible mechanism for the reaction is depicted in Fig. 4.
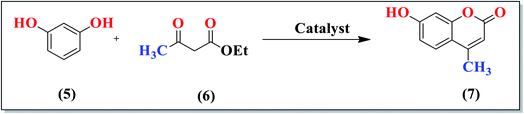 |
| Scheme 5 Synthesis of substituted coumarins. | |
Table 7 The reaction for synthesis of substituted coumarins in solvent-free condition at room temperature
Entry |
Reactant |
Catalyst |
Temperature (°C) |
Time (min) |
%Yield |
1 |
Resorcinol + ethylacetoacetate |
ZrO2–TiO2 |
RT |
180 |
97 |
2 |
Resorcinol + ethylacetoacetate |
ZrO2–ZnO |
RT |
240 |
63 |
3 |
Resorcinol + ethylacetoacetate |
ZrO2/cellulose |
RT |
180 |
Nil |
4 |
Catechol + ethylacetoacetate |
ZrO2–TiO2 |
80 |
240 |
55 |
5 |
o-Nitrophenol + ethylacetoacetate |
ZrO2–TiO2 |
80 |
240 |
Nil |
6 |
Resorcinol + ethylacetoacetate |
Without catalyst |
80 |
240 |
Nil |
Table 8 Comparison of efficiency ZrO2–TiO2 with reported catalysts
Entry |
Catalyst |
Time (min) |
Temperature (°C) |
Solvent |
Yield |
References |
1 |
Zeolite BEA |
240 |
130 |
PhNO2 |
63 |
42 |
2 |
PFPAT |
180 |
110 |
Toluene |
90 |
43 |
3 |
MFRH |
50 |
80 |
Solvent-free |
65 |
43 |
4 |
Nanoreactors |
60 |
130 |
Solvent-free |
30 |
43 |
5 |
CMK-5-SO3H |
20 |
130 |
Solvent-free |
95 |
44 |
6 |
CMK-5 |
60 |
130 |
Solvent-free |
10 |
44 |
7 |
ZrO2–TiO2 |
180 |
RT |
Solvent-free |
97 |
41 |
8 |
ZrO2–TiO2 |
110 |
60 |
Toluene |
95 |
41 |
9 |
ZrO2–TiO2 |
150 |
60 |
Ethanol |
92 |
41 |
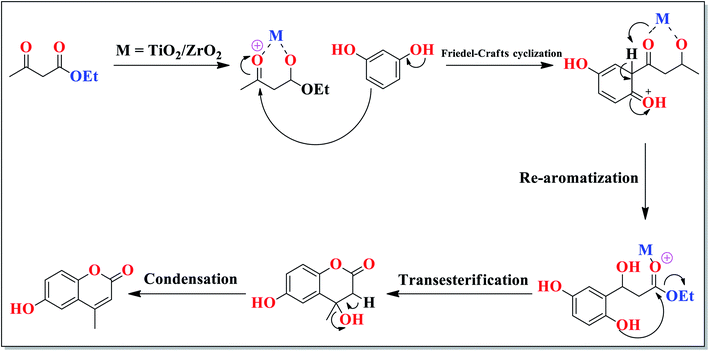 |
| Fig. 4 Plausible mechanism for the synthesis of substituted coumarins in presence of zirconia-based heterogeneous catalyst. | |
Kumbar and his coworkers developed efficient and facile methodology for synthesis of class of chromeno-3-substituted derivatives (10a–10l) in excellent yields in presence of solid-supported heterogeneous silica sulphuric acid as a reuseable catalyst (Scheme 6).45
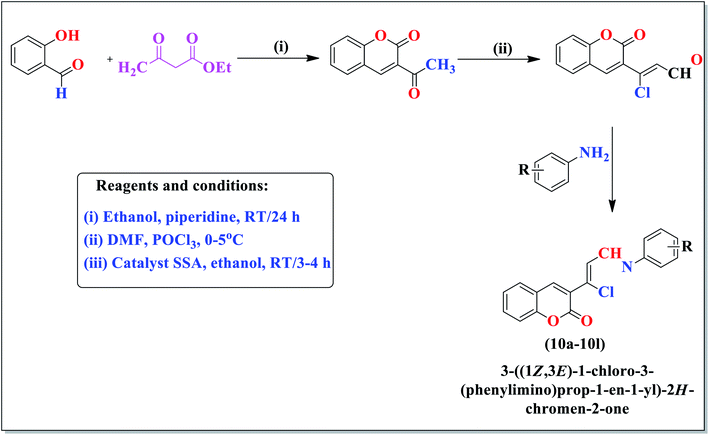 |
| Scheme 6 Synthesis of novel coumarin Schiff bases in presence of silica sulphuric acid as reuseable catalyst. | |
They found that use of silica sulphuric acid as catalyst provide good to excellent yields of desired products as shown in Table 9. The reaction was also optimized with respect to polar protic and aprotic solvents viz. acetonitrile, ethanol, DMF, dioxane, THF and DMSO as summarized in Table 10. The plausible mechanism of reaction was presented in Fig. 5. First there is nucleophilic attack of aniline on the carbonyl carbon of coumarin. Then in next step protonation occurs from silica sulphuric acid, forming itself as a nucleophile in the reaction mixture. Then nucleophilic SSA abstracts protons from nitrogen and gains stability by the formation of double bond between C and N and subsequent dehydration give desired product.
Table 9 Physical and analytical data of synthesized coumarin derivatives
Products |
R |
Yield (%) |
Time (min) |
Melting point (°C) |
10a |
H |
78 |
180 |
165–167 |
10b |
p-Cl |
62 |
210 |
193–195 |
10c |
p-Br |
61 |
190 |
182–184 |
10d |
p-OH |
67 |
195 |
198–200 |
10e |
p-OCH3 |
62 |
210 |
205–208 |
10f |
p-CH3 |
71 |
240 |
202–204 |
10g |
2,6-Dimethyl |
58 |
220 |
188–190 |
10h |
m-Cl |
68 |
210 |
197–200 |
10i |
m-Br |
69 |
190 |
178–181 |
10j |
m-OH |
62 |
195 |
184–186 |
10k |
m-OCH3 |
59 |
200 |
208–210 |
10l |
m-CH3 |
73 |
225 |
212–214 |
Table 10 Optimization of reaction conditions
Entry |
Solvent |
SSA |
Time (h) |
Temperature (°C) |
Yield (%) |
1 |
Acetonitrile |
1.0 |
4 |
25 |
35 |
2 |
Ethanol |
1.0 |
3 |
25 |
78 |
3 |
DMF |
1.0 |
12 |
25 |
Nil |
4 |
Dioxane |
1.0 |
6 |
25 |
38 |
5 |
THF |
1.0 |
12 |
25 |
Trace |
6 |
DMSO |
1.0 |
12 |
25 |
Nil |
7 |
Acetone |
1.0 |
12 |
25 |
Nil |
8 |
Acetonitrile |
2.0 |
12 |
40 |
42 |
9 |
Ethanol |
0.0 |
12 |
25 |
Nil |
10 |
Ethanol |
Silica |
12 |
25 |
45 |
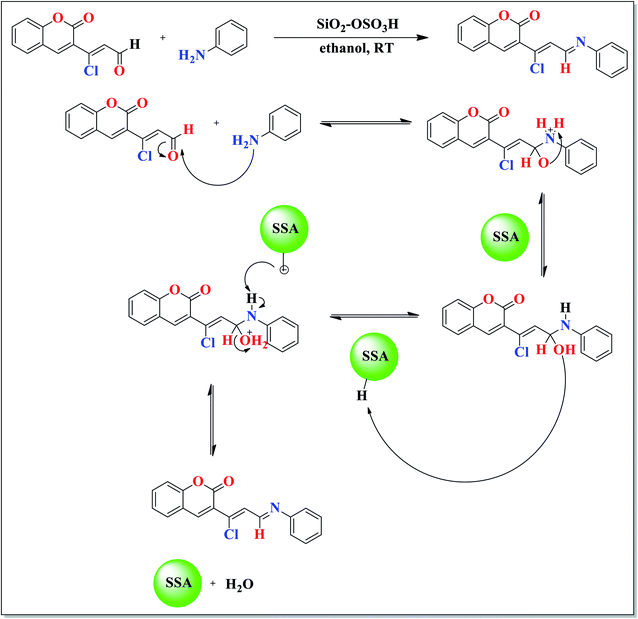 |
| Fig. 5 Proposed reaction pathway for the synthesis of substituted coumarins. | |
Moghaddam and Hoda designed magnetic graphene oxide coated with cysteic acid as an efficient and reuseable catalyst for the synthesis of 4H-chromene derivatives (13) via one-pot multicomponent reaction between enolizable compound (11), malononitrile (12), substituted aldehydes (2) or isatin and a mixture of water–ethanol as a green solvent (Scheme 7).46 Excellent yield of desired products, less reaction time, mild reaction conditions and eco-friendly approach are some merits of present methodology.
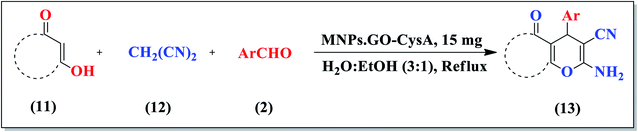 |
| Scheme 7 One-pot three-component reaction of enolizable compound, active methylene nitriles, and aldehydes catalyzed by MNPs·GO–CysA in water : ethanol. | |
An efficient and facile method for the one-pot synthesis of dihydropyrano[3,2-c]chromene derivatives (14) have been reported via reaction between substituted aldehydes (2), 4-hydroxycoumarin (8) and malononitrile (12) in presence of poly(4-vinyl-pyridine) as a cheap, efficient and recyclable catalyst (Scheme 8). They also reported the synthesis of biscoumarin derivatives (9) via one-pot reaction between substituted aldehydes (2) and 4-hydroxycoumarin (8) in presence of same catalyst (Scheme 9).47
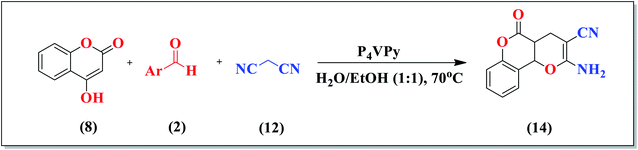 |
| Scheme 8 Synthesis of dihydropyrano[3,2-c] chromene derivatives. | |
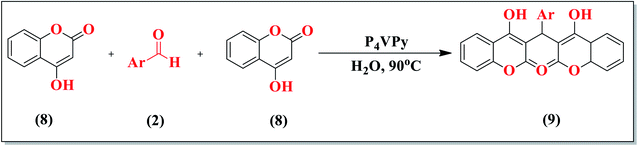 |
| Scheme 9 Synthesis of biscoumarin derivatives. | |
To optimize the reaction conditions, a model reaction was explored between 4-chlorobenzaldehyde, malononitrile and 4-hydroxycoumarin in presence of different concentration of P4VPy. The effect of different solvents viz. CH3CN, CH2Cl2, H2O and EtOH and temperature in the synthesis of dihydropyrano[3,2-c] chromene derivatives in the presence of P4VPy summarized in Table 11. They found that best result was obtained using 20 mg of P4VPy at 70 °C in a mixture of H2O and ethanol. They also observed that aldehydes containing electron-withdrawing as well as electron-donating groups such as Cl, Br, CH3, OCH3, NO2 and OH in the ortho, meta and para positions can be easily converted to the corresponding dihydropyrano[3,2-c] chromenes in less reaction times with excellent yield.
Table 11 The effect of different reaction conditions for the synthesis of dihydropyrano[3,2-c] chromene derivatives in the presence of P4VPy
Entry |
Catalyst (mg) |
Solvent |
Temperature (°C) |
Time (min) |
Yield (%) |
1 |
— |
No solvent |
RT |
120 |
Nil |
2 |
— |
No solvent |
100 |
120 |
Nil |
3 |
20 |
CH3CN |
RT |
120 |
Nil |
4 |
20 |
CH3CN |
Reflux |
120 |
Mixture of products |
5 |
20 |
CH2Cl2 |
RT |
120 |
Nil |
6 |
20 |
CH2Cl2 |
Reflux |
120 |
Mixture of products |
7 |
20 |
H2O |
90 |
180 |
50 |
8 |
24 |
H2O |
90 |
120 |
50 |
9 |
24 |
EtOH |
RT |
150 |
60 |
10 |
20 |
EtOH |
50 |
120 |
60 |
11 |
20 |
EtOH |
70 |
120 |
60 |
12 |
20 |
H2O/EtOH |
70 |
5 |
95 |
13 |
24 |
H2O/EtOH |
70 |
5 |
95 |
After most favourable results of P4VPy in the synthesis of dihydropyrano[3,2-c]chromene derivatives, they were interested to study the efficiency of this polymeric reagent in the synthesis of biscoumarins. For standardization of reaction conditions, first model reaction was carried out between 4-chlorobenzaldehyde and 4-hydroxycoumarin in the presence of P4VPy at different reaction conditions as shown in Table 12. They observed that best reaction conditions for the synthesis of the biscoumarin derivatives are use of 20 mg of the P4VPy in water at 90 °C. They also found that aldehydes containing electron-withdrawing or electron donating substituents converting to desired products in less time. The plausible mechanism for the synthesis of substituted pyrazoles given in Fig. 6. The comparison of catalytic activity and reaction conditions of present catalyst P4VPy for the synthesis of dihydropyrano[3,2-c]chromene derivatives and biscoumarin derivatives are summarized in Table 13 and Table 14. This comparison shows disadvantages of the other procedures such as long reaction times, toxic reagents, high temperature, organic solvents, excess reagents and low yields.
Table 12 Optimization of the reaction conditions for the synthesis of biscoumarin derivatives catalyzed by P4VPy
Entry |
Catalyst (mg) |
Solvent |
Temperature (°C) |
Time (min) |
Yield (%) |
1 |
— |
No solvent |
RT |
120 |
Nil |
2 |
— |
No solvent |
100 |
120 |
Nil |
3 |
20 |
CH3CN |
RT |
120 |
Nil |
4 |
20 |
CH3CN |
Reflux |
120 |
Mixture of products |
5 |
20 |
CH2Cl2 |
RT |
120 |
Nil |
6 |
20 |
CH2Cl2 |
Reflux |
120 |
Mixture of products |
7 |
20 |
EtOH |
RT |
120 |
30 |
8 |
20 |
EtOH |
Reflux |
120 |
60 |
9 |
10 |
H2O |
RT |
90 |
40 |
10 |
15 |
H2O |
RT |
90 |
50 |
11 |
20 |
H2O |
RT |
90 |
75 |
12 |
20 |
H2O |
90 |
5 |
96 |
13 |
24 |
H2O/EtOH |
90 |
5 |
96 |
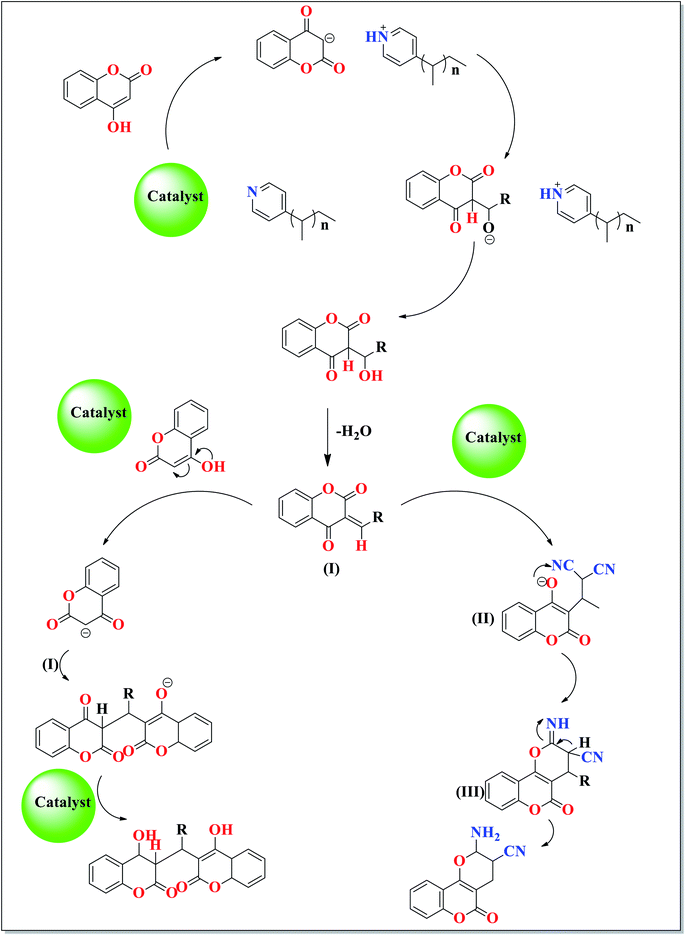 |
| Fig. 6 Proposed mechanism for the synthesis of dihydropyrano[3,2-c]chromene and biscoumarin derivatives in the presence of P4VPy as catalyst. | |
Table 13 Comparison of different catalysts for the synthesis of dihydropyrano[3,2-c]chromene derivatives
Entry |
Catalyst (mol%) |
Reaction conditions |
Time (min) |
Yield (%) |
References |
1 |
SDS |
Water/60 °C |
150 |
88 |
48 |
2 |
Nano ZnO |
Ethanol reflux |
90 |
49 |
49 |
3 |
Nano Al(OH)3 |
Ethanol reflux |
120 |
48 |
49 |
4 |
DAHP |
Ethanol–H2O/25 °C |
240 |
85 |
50 |
5 |
(S)-proline |
Ethanol–H2O/100 °C |
180 |
78 |
50 |
6 |
Nano Al2O3 |
Ethanol reflux |
120 |
71 |
51 |
7 |
P4VPy |
Ethanol–H2O/70 °C |
5 |
95 |
47 |
Table 14 Comparison of different catalysts used for the synthesis of biscoumarins
Entry |
Catalyst (mol%) |
Reaction conditions |
Time (min) |
Yield (%) |
References |
1 |
SDS |
Water/60 °C |
150 |
93 |
48 |
2 |
[bmim]BF4 |
Solvent-free/60–70 °C |
150 |
91 |
52 |
3 |
I2 |
H2O/100 °C |
27 |
93 |
53 |
4 |
CHOH |
Solvent-free/50 °C |
120 |
99 |
54 |
5 |
[P4VPy-BuSO3H]Cl–X(AlCl3) |
Toluene/90 °C |
36 |
93 |
55 |
6 |
PSA |
Solvent-free/100 °C |
240 |
96 |
56 |
7 |
Piperidine |
EtOH/r.t |
240 |
96 |
57 |
8 |
P4VPy |
H2O/90 °C |
5 |
96 |
47 |
An efficient, green and inexpensive synthesis of benzylpyrazolyl coumarin (16) by one-pot multicomponent condensation of hydrazine hydrate or phenyl hydrazine (15), β-ketoester (6), substituted aldehydes (2) and 4-hydroxycoumarin (8) in the presence of Amberlite IR-120 as a catalyst in an aqueous medium has been reported by Katariya and his coworkers (Scheme 10).58
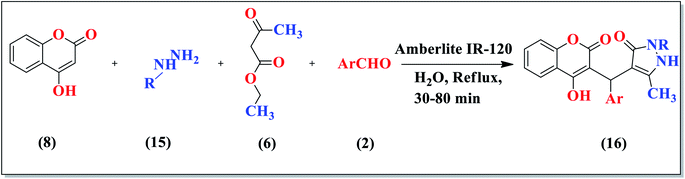 |
| Scheme 10 General scheme for the synthesis of benzlypyrazolyl coumarin. | |
Kaur et al. reported the synthesis of 3,3′-(arylmethylene)bis(4-hydroxy-2H-chromen-2-ones) via one-pot reaction between substituted aldehydes (2) and 4-hydroxy coumarin (8) catalyzed by camphor sulfonic acid (Scheme 11).59 Mild reaction conditions, use of metal-free organocatalyst, excellent yields of desired products, high atom economy, eco-friendly, easy isolation of products and no need of column chromatography are some merits of present methodology. To standardize the reaction conditions they conducted a model reaction between 4-methylbenzaldehyde and 4-hydroxycoumarin. Firstly, they explored the reaction in the absence of catalyst as well as solvent at room temperature and they observed that trace amount of yield was obtained after 24 h. Then under catalyst-free conditions, the same reaction was give 22% yield of desired product in ethanol. After getting the poor yields of desired product, they were interested to check the catalytic activity of camphor sulfonic acid as catalyst for this reaction. They observed that 20 mol% of camphor sulfonic acid in aqueous ethanol (1
:
1 v/v) at room temperature came out as the best suitable conditions for the synthesis of desired product in terms of reaction time as well as product yield as summarized in Table 15.
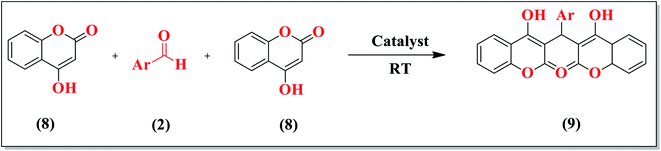 |
| Scheme 11 Reported protocols for the synthesis of biscoumarin. | |
Table 15 Standardization of reaction conditions for the synthesis of 3,3′-(arylmethylene)bis(4-hydroxy-2H-chromen-2-ones)
Entry |
Catalyst (mol%) |
Solvent |
Time (h) |
Yield (%) |
1 |
No catalyst |
Solvent-free |
24 |
Nil |
2 |
No catalyst |
EtOH |
6 |
22 |
3 |
Camphor sulfonic acid (20 mol%) |
EtOH |
6 |
78 |
4 |
Camphor sulfonic acid (20 mol%) |
MeOH |
6 |
72 |
5 |
Camphor sulfonic acid (20 mol%) |
H2O |
6 |
61 |
6 |
Camphor sulfonic acid (20 mol%) |
EtOH : H2O (1 : 1 v/v) |
2 |
94 |
7 |
Camphor sulfonic acid (15 mol%) |
EtOH : H2O (1 : 1 v/v) |
2 |
86 |
8 |
Camphor sulfonic acid (20 mol%) |
EtOH : H2O (1 : 1 v/v) |
2 |
94 |
The plausible mechanism for the synthesis of 3,3-(arylmethylene)-bis(4-hydroxy-2H-chromen-2-ones) is shown in Fig. 7. According to the mechanism, firstly camphor sulfonic acid activate the carbonyl group of aldehydes which enhance the attack from C-3 position of 4-hydroxycoumarin and generate the Knoevenagel intermediate. Then second molecule of 4-hydroxycoumarin attack on Knoevenagel intermediate followed by enolisation gives the desired product in excellent yield.
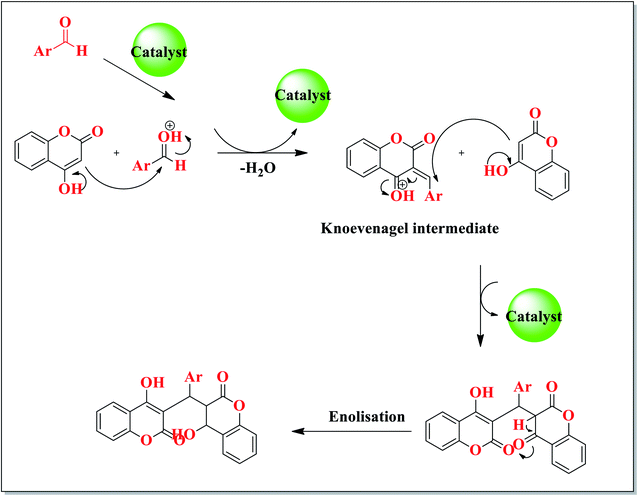 |
| Fig. 7 Proposed mechanism for the synthesis of biscoumarin catalyzed by camphor sulfonic acid. | |
A novel heterogeneous catalytic method was developed for the synthesis of coumarin (7) via reaction between β-ketoesters (6) and substituted phenols (5) in presence of Zn0.925Ti0.075O as catalyst by Jadhav and his coworkers (Scheme 12).60 They also observed that this shows recycle activity up to seven cycles with very good stability. Firstly, they standardized the reaction conditions in order to verify the role of catalyst by conducting a model reaction between phloroglucinol and ethylacetoacetate under solvent-free conditions and the results are summarized in Table 16. They observed that Zn0.925Ti0.075O is best catalyst for optimization studies in the synthesis of coumarin by Pechmann condensation. The various solvents effect viz. DCM, ethylacetate, acetonitrile, water, ethanol, toluene and DMF also studied for optimizing the reaction conditions during the synthesis of coumarin and the results are summarized in Table 17. They conclude that solvent-free conditions and temperature 110 °C was suitable for the synthesis of desired products under the optimized reaction conditions. The effect of catalyst concentration was studied on model reaction and the results are presented in Table 18. They found that 10 mol% Zn0.925Ti0.075O catalyst was the most optimal for Pechmann condensation of ethylacetoacetate and phloroglucinol.
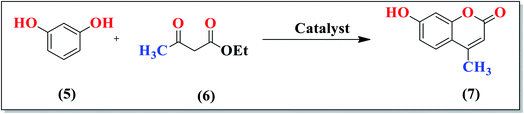 |
| Scheme 12 Coumarin synthesis by Pechmann condensation. | |
Table 16 Catalytic screening for synthesis of substituted coumarin by Pechmann condensation reaction
Entry |
Catalyst |
Time (h) |
Yield (%) |
1 |
No catalyst |
24 |
Nil |
2 |
ZnO |
5 |
Nil |
3 |
Zn0.975Ti0.025O |
3 |
37 |
4 |
Zn0.950Ti0.050O |
4 |
60 |
5 |
Zn0.925Ti0.075O |
3 |
88 |
6 |
Zn0.900Ti0.100O |
3 |
88 |
Table 17 Solvent screening for synthesis of substituted coumarin by Pechmann condensation reaction
Entry |
Solvent |
Temperature (°C) |
Time (h) |
Yield (%) |
1 |
DCM |
40 |
8 |
24 |
2 |
Ethyl acetate |
78 |
8 |
16 |
3 |
Acetonitrile |
80 |
8 |
37 |
4 |
Water |
100 |
5 |
41 |
5 |
Ethanol |
78 |
5 |
63 |
6 |
Toluene |
110 |
10 |
Nil |
7 |
DMF |
150 |
10 |
Nil |
8 |
Solvent-free |
110 |
3 |
88 |
9 |
Solvent-free |
90 |
5 |
61 |
10 |
Solvent-free |
130 |
3 |
80 |
Table 18 Effect of catalyst concentration for synthesis of substituted coumarin by Pechmann condensation reaction
Entry |
Catalyst amount (mol%) |
Time (h) |
Yield (%) |
1 |
5 |
5 |
67 |
2 |
10 |
3 |
88 |
3 |
15 |
3 |
88 |
The reaction pathway for the synthesis of coumarin through Pechmann condensation is represented in Fig. 8. Initially, reaction proceeds with the nucleophilic attack of the hydroxyl group of phloroglucinol on the activated ethylacetoacetate, resulting in the formation of intermediate. The formed intermediate rapidly undergoes cyclization through Lewis acid-catalyzed intramolecular condensation and followed by removal of water molecule give desired products.
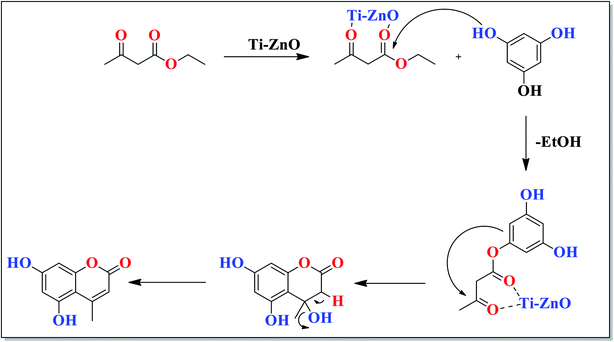 |
| Fig. 8 Plausible mechanism for Pechmann condensation using EAA and phloroglucinol promoted by Zn0.925Ti0.075O NPs. | |
A magnetic nanocatalyst of Fe3O4@SiO2–ZnCl2 has been used for the synthesis of coumarin derivatives (7) via Pechmann condensation reaction of substituted phenols (5) and β-ketoesters (6) in excellent yield under solvent-free conditions by Rahimi and Soleimani (Scheme 13).61 The advantages of this method are straightforward, easy work-up, catalyst reuseability and leading to excellent yields.
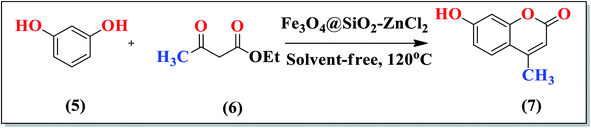 |
| Scheme 13 Direct synthesis of coumarin derivatives. | |
Carrillo and his coworkers reported the synthesis of substituted coumarins (7) via one-pot reaction between substituted phenols (5) and β-ketoesters (6) in presence of propylsulfonic acid supported in FDU-5 (FDU-5-Pr–SO3H) as a catalyst (Scheme 14).62 The catalytic activity of FDU-5-Pr–SO3H for the synthesis of substituted coumarins under optimized conditions was compared with other organic and inorganic catalysts summarized in Table 19.
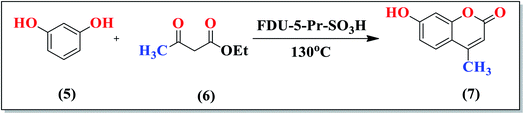 |
| Scheme 14 Synthesis of coumarin derivatives via Pechmann condensation of phenols with β-keto-ester catalyzed by FDU-5-Pr–SO3H. | |
Table 19 Comparison of different catalysts used for the synthesis of substituted coumarins
Catalyst |
Catalyst amount (mol%) |
Reaction time (min) |
Temperature (°C) |
Yield (%) |
References |
FDU-5 |
1.65 |
120 |
130 |
NR |
62 |
FDU-5-Pr–SO3H |
1.65 |
60 |
130 |
97 |
62 |
MCM-41–10SO3H |
3.6 |
120 |
120 |
99 |
63 |
SBA-15–10SO3H |
2.0 |
120 |
120 |
88 |
63 |
C@TiO2–SO3–SbCl2 |
100.0 |
35 |
120 |
94 |
64 |
m-ZrP |
2.0 |
240 |
160 |
76 |
65 |
SiO2–SnCl3 |
5.0 |
35 |
120 |
64 |
66 |
FeCl3 (ultrasound) |
10.0 |
20 |
100 |
97 |
67 |
Fe3O4@SiO2@Et-PhSO3H |
0.3 |
120 |
120 |
93 |
68 |
CMK-5-SO3H |
3.0 |
130 |
130 |
95 |
69 |
SBA-15-Ph-Pr–SO3H |
7.0 |
130 |
130 |
90 |
70 |
p-TsOH |
7.0 |
130 |
130 |
65 |
70 |
Zr-TMS-BSA-10 |
10 wt% |
150 |
150 |
81.4 |
71 |
Saffarian et al. reported the synthesis of coumarin containing 1,4-dihydropyridines (18) via condensation reaction between substituted aldehydes (2), 4-hydroxycoumarin (8) and ammonium acetate (17) under solvent-free conditions (Scheme 15).72 Simple protocol, simplicity of product isolation using water, decrease the temperature of reaction, reduce the use of hazardous solvents, excellent yield of products, eco-friendly conditions and less reaction times are some beauties of present methodology. Firstly, to optimize the reaction conditions they conducted a model reaction between 4-methyl benzaldehyde, 4-hydroxycoumarin and ammonium acetate. They observed that 10 mg of the Fe3O4@SiO2@(CH2)3–urea–quinoline sulfonic acid chloride at 80 °C under solvent free conditions supplied the best results as presented in Table 20. They performed the model reaction also in the presence of related intermediates of the Fe3O4@SiO2@(CH2)3–urea–quinoline sulfonic acid chloride at 80 °C under solvent free conditions for 20 min and results are summarized in Table 21.
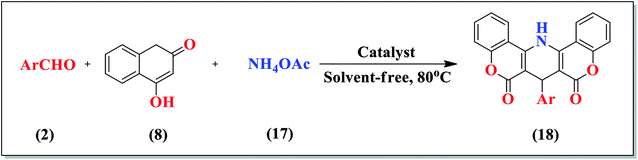 |
| Scheme 15 Catalytic synthesis of coumarin containing 1,4-DHPs. | |
Table 20 Optimization of reaction conditions
Entry |
Solvent |
Temperature (°C) |
Catalyst (mg) |
Time (min) |
Yield (%) |
1 |
— |
90 |
— |
90 |
30 |
2 |
— |
90 |
5 |
30 |
80 |
3 |
— |
90 |
10 |
20 |
86 |
4 |
— |
90 |
15 |
20 |
85 |
5 |
— |
100 |
10 |
20 |
81 |
6 |
— |
80 |
10 |
20 |
85 |
7 |
— |
60 |
10 |
30 |
70 |
8 |
H2O |
Reflux |
10 |
30 |
85 |
9 |
EtOH |
Reflux |
10 |
45 |
70 |
10 |
EtOAc |
Reflux |
10 |
90 |
20 |
11 |
CH2Cl2 |
Reflux |
10 |
90 |
Nil |
12 |
n-Hexane |
Reflux |
10 |
90 |
Nil |
Table 21 Screening the model reaction in the presence of desired catalyst
Entry |
Catalyst |
Yield (%) |
1 |
Fe3O4 |
40 |
2 |
Fe3O4@SiO2 |
40 |
3 |
Fe3O4@SiO2@(CH2)3–urea–quinoline |
65 |
4 |
Fe3O4@SiO2@(CH2)3–urea–quinoline sulfonic acid chloride |
85 |
A suitable protocol for synthesis of coumarins derivatives (7) was reported by Bouasla and his coworkers via one-pot reaction between substituted phenols (5) and β-ketoesters (6) in presence of heterogeneous solid acid catalyst viz. Amberlyst-15 in solvent-free medium under microwave irradiation (Scheme 16).73 Initially, they conducted a model reaction between resorcinol and ethylacetoacetate as model substrate. They observed that by changing the reaction time from 5 min to 20 min, a maximum yield of 97% was obtained and no reaction was observed in absence of catalyst as summarized in Table 22. The plausible mechanism for the reaction is shown in Fig. 9.
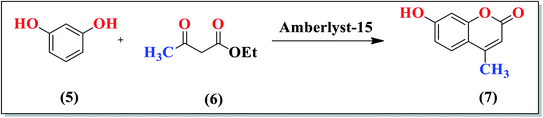 |
| Scheme 16 Pechmann reaction of resorcinol with ethylacetoacetate to produce 7-hydroxy-4-methylcoumarin. | |
Table 22 Condensation reaction of resorcinol with ethyl acetoacetate using various heterogeneous solid acids catalysts
Catalyst |
Acidity |
Yield (%) |
Amberlyst-15 |
4.30 |
97 |
H-β |
1.01 |
21 |
TS–OS–SO3H |
1.24 |
44 |
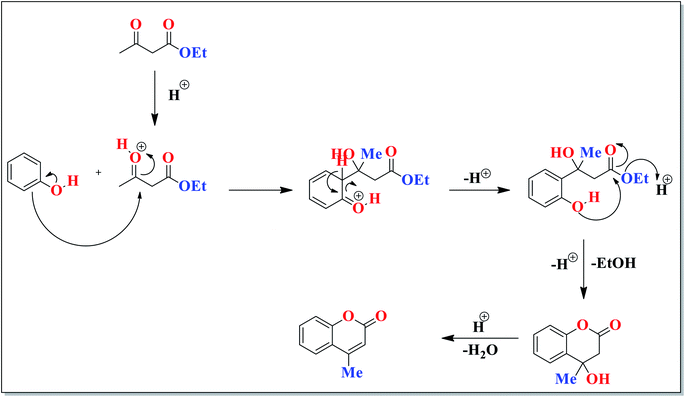 |
| Fig. 9 A plausible mechanism for the Pechmann condensation of phenol and ethylacetoacetate in presence of Amberlyst-15. | |
An efficient method for the synthesis of 3-carboxycoumarins (20) was reported via Knoevenagel condensation reaction between substituted aldehydes (2) and Meldrum's acid (19) in presence of polymeric magnetic nanocatalyst by Maleki et al. (Scheme 17).74 This method has many advantages such as less reaction time, high yield and easy isolation of catalyst. The plausible mechanism for the reaction is shown in Fig. 10.
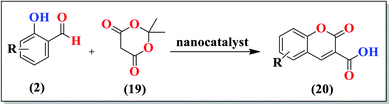 |
| Scheme 17 Synthesis of substituted coumarins. | |
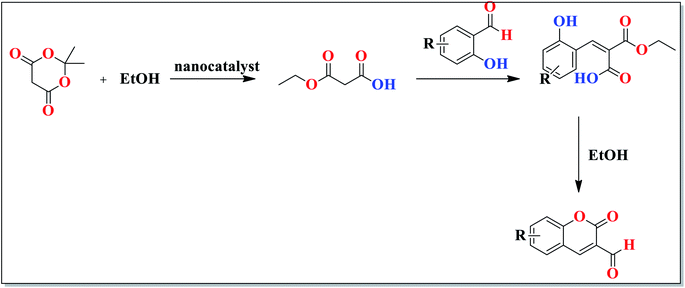 |
| Fig. 10 Proposed mechanism for the synthesis of 3-carboxy coumarins in presence of polymeric magnetic nanocatalyst. | |
Suryawanshi and his coworkers reported the synthesis of coumarins (7) via Pechmann condensation reaction between substituted phenols (5) and β-ketoesters (6) in presence of reuseable polymeric SO3H-functionalized cation exchange resins viz. Amberlite IR-120, Dowex 50, X-8100 and Tulsion T-42 (Scheme 18).75 Excellent yield of products, short reaction time, easy work-up and use of safe catalyst are some advantages of present methodology.
 |
| Scheme 18 The Pechmann condensation between resorcinol and ethyl acetoacetate catalyzed by different cation exchange resins. | |
Rostami and Zare reported the synthesis of substituted coumarins (7) via one-pot reaction between substituted phenols (5) and β-ketoesters (6) in presence of carbonized sugarcane bagasse (CSCB) as a new and efficient solid acid catalyst (Scheme 19).76 Simple preparation of catalyst, safe handling, inexpensive, excellent yield of products, catalyst reuseability, solvent-free and easy work-up are some benefits of present methodology. Initially, model reaction was considered between 3-hydroxyphenol and ethylacetoacetate and the effect of different solvents, temperature and amount of catalyst was investigated and results were summarized in Table 23. The plausible mechanism for the reaction is shown in Fig. 11.
 |
| Scheme 19 Synthesis of substituted coumarins. | |
Table 23 Optimization of reaction conditions for AHS@CSCB catalyzed Pechmann condensation between 1,3 dihydroxy phenol and ethyl acetoacetate
Entry |
Concentration of catalyst (mg) |
Solvent |
Temperature (°C) |
Time (min) |
Yield (%) |
1 |
None |
H2O |
Reflux |
120 |
0 |
2 |
30 |
H2O |
Reflux |
60 |
50 |
3 |
30 |
EtOH |
Reflux |
40 |
80 |
4 |
30 |
Solvent-free |
80 |
15 |
91 |
5 |
30 |
Solvent-free |
70 |
30 |
89 |
6 |
30 |
Solvent-free |
120 |
120 |
26 |
7 |
20 |
Solvent-free |
15 |
15 |
92 |
8 |
10 |
Solvent-free |
5 |
5 |
92 |
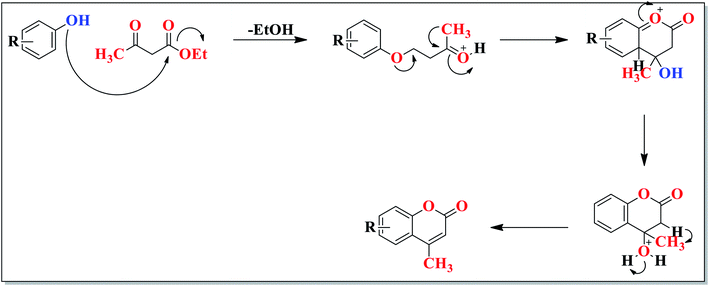 |
| Fig. 11 Plausible mechanism for the synthesis of coumarins, biscoumarins and benzoxanthenes in the presence of AHS@CSCB. | |
Sun and his coworkers reported the synthesis of substituted coumarins (7) via Pechmann condensation reaction between substituted phenols (5) and β-ketoesters (6) catalyzed from Wells–Dawson heteropolyacid (H6P2W18O62). This work provides a novel, cheaper and safer way to synthesize coumarins unsubstituted on the pyranic nucleus (Scheme 20).77 Initially, they optimized the reaction conditions by exploring a model reaction between 2-methyl-3-hydroxy-phenol and ethyl 3, 3-diethoxypropionate. The effect of the temperature and reaction time were investigated and results were summarized in Table 24. The comparison of efficiencies of various catalysts used in the synthesis of 7-hydroxy-8-methylcoumarin was summarized in Table 25. The plausible mechanism for the reaction is shown in Fig. 12.
 |
| Scheme 20 Synthesis of substituted coumarins. | |
Table 24 Optimization of Pechmann condensation reaction for the synthesis of 7-hydroxy-8-methylcoumarin
Entry |
Catalyst concentration |
Temperature (°C) |
Time (h) |
Yield (%) |
1 |
0.10 |
100 |
3 |
75 |
2 |
0.25 |
100 |
3 |
87 |
3 |
0.50 |
100 |
3 |
86 |
4 |
1.00 |
100 |
3 |
84 |
5 |
0.25 |
80 |
3 |
74 |
6 |
0.25 |
90 |
3 |
90 |
7 |
0.25 |
90 |
2 |
72 |
8 |
0.25 |
90 |
4 |
89 |
9 |
0.25 |
90 |
3 |
84 |
10 |
0.25 |
90 |
3 |
90 |
11 |
0.25 |
90 |
3 |
95 |
12 |
0.25 |
90 |
3 |
95 |
Table 25 Synthesis of 7-hydroxy-8-methylcoumarin mediated by different catalysts
Entry |
Catalyst |
Time (h) |
Yield (%) |
1 |
MeSO3H |
3 |
20 |
2 |
MeSO3H/basic Al2O3 |
3 |
30 |
3 |
MeSO3H/neutral Al2O3 |
3 |
34 |
4 |
MeSO3H/acidic Al2O3 |
3 |
80 |
5 |
Acidic Al2O3 |
3 |
30 |
6 |
Al2O3 |
2 |
10 |
7 |
AlCl3/MeSO3H |
2 |
12 |
8 |
ZnCl3/MeSO3H |
2 |
5 |
9 |
Cu(CH3CN)4PF6 |
2 |
10 |
10 |
H6P2W18O62 |
2 |
82 |
11 |
FeCl3 |
3 |
8 |
12 |
TiCl4 |
3 |
5 |
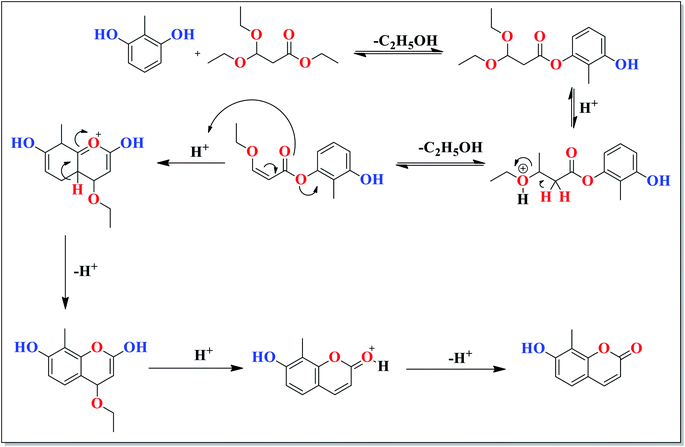 |
| Fig. 12 Possible mechanism for the synthesis of coumarins catalyzed from Wells–Dawson heteropolyacid (H6P2W18O62). | |
An efficient and facile synthesis of coumarins (7) was reported in excellent yields via Pechmann condensation reaction between substituted phenols (5) and β-ketoesters (6) under solvent-free medium using both conventional method and microwave irradiation in less reaction times in presence of cellulose sulfuric acid by Kuram et al. (Scheme 21).78 The efficiency of the cellulose sulfuric acid compared with other catalysts is summarized in Table 26. It was found that cellulose sulfuric acid is a more efficient and superior catalyst over other acidic catalysts with respect to reaction time and yield.
 |
| Scheme 21 Synthesis of coumarins by using cellulose sulfuric acid as a solid acid catalyst. | |
Table 26 Comparison of efficiency of cellulose sulfuric acid with reported catalysts
Entry |
Catalyst |
Yield (%) |
1 |
Cellulose sulfuric acid |
97 |
2 |
Silica sulfuric acid |
92 |
3 |
p-Toluene sulfonic acid |
85 |
4 |
Sulfuric acid in acetic acid |
55 |
5 |
No catalyst |
15 |
Palaniappan and John et al. reported the synthesis of substituted coumarins (7) via one-pot reaction between substituted phenols (5) and β-ketoesters (6) in presence of novel polyaniline–fluoroboric acid–dodecylhydrogensulfate (PANI–HBF4–DHS) as reuseable catalyst (Scheme 22).79
 |
| Scheme 22 Synthesis of substituted coumarins. | |
Kolvari and his coworkers reported the synthesis of substituted coumarins (7) via one-pot reaction between substituted phenols (5) and β-ketoesters (6) in presence of perlite sulfonic acid (perlite-SO3H (PeSA)) as heterogeneous reuseable solid acid catalysts (Scheme 23).80 Inexpensive, ease of preparation, more stability and reusability, low toxicity and easy of handling are some advantages of present catalytic systems. To show the advantages of current protocol in comparison with reported results in literature was summarized in Table 27. They found that PeSA showed greater activity than some other than some other heterogeneous catalysts.
 |
| Scheme 23 Synthesis of substituted coumarins catalyzed by PeSA. | |
Table 27 Comparison of activity of the PeSA catalyst with some other reported catalysts
Entry |
Catalyst |
Condition |
Yield (%) |
Time (min) |
References |
1 |
PeSA |
110 °C/Solvent-free |
97 |
15 |
80 |
2 |
ASA |
110 °C/Solvent-free |
85 |
30 |
81 |
3 |
CMK-5-SO3H |
110 °C/Solvent-free |
95 |
20 |
82 |
Reddy et al. reported the synthesis of substituted coumarins (7) via Pechmann condensation reaction between substituted phenols (5) and β-ketoesters (6) in presence of W/ZrO2 solid acid catalyst (Scheme 24).83
 |
| Scheme 24 W/ZrO2 solid acid catalyzed synthesis of substituted coumarins. | |
Kim et al. reported the synthesis of substituted coumarins (22) via condensation reaction between substituted phenols (5) and allenes (21) in the presence of TfOH as Bronsted acid catalyst in excellent yield (Scheme 25).84 The plausible mechanism for the reaction is shown in Fig. 13.
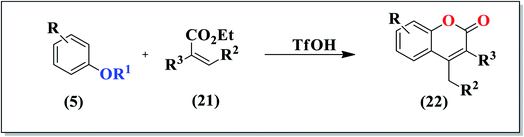 |
| Scheme 25 TfOH-mediated preparation of coumarins. | |
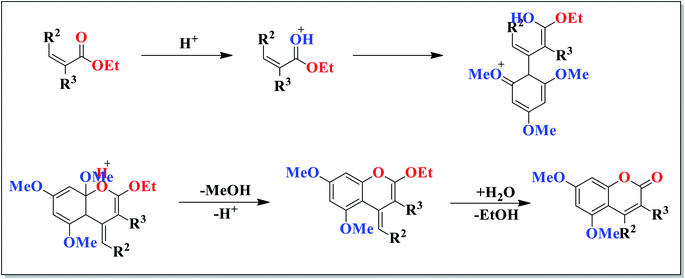 |
| Fig. 13 Plausible mechanism for the synthesis of coumarins in the presence of TfOH as Brønsted acid catalyst. | |
Maheswara and his coworkers synthesized substituted coumarins (7) via Pechmann condensation reaction between substituted phenols (5) and β-ketoesters (6) in presence of heterogeneous recyclable catalyst (HClO4.SiO2) under solvent-free medium (Scheme 26).85 Cost-effective, less reaction time and operational simplicity are some benefits of present methodology.
 |
| Scheme 26 Synthesis of coumarins using HClO4.SiO2 under solvent-free conditions. | |
Kuram et al. reported the synthesis of substituted coumarins (7) via Pechmann condensation reaction between substituted phenols (5) and β-ketoesters (6) in the presence of xanthan sulfuric acid as a solid acid catalyst under solvent-free conditions (Scheme 27).86 They found that this method is very simple, inexpensive, less reaction time and catalyst could be reused. The effect of catalyst on the yield of products was summarized in Table 28. They also investigated the efficiency of the XSA compared to various sulphur analog acidic catalysts and results are summarized in Table 29.
 |
| Scheme 27 Synthesis of coumarin by xanthan sulfuric acid as a solid acid catalyst. | |
Table 28 Effect of catalysts on yield of synthesis of substituted coumarins
Entry |
Catalyst |
Quantity |
Yield (%) |
1 |
Xanthan sulfuric acid |
0.08 g |
96 |
2 |
Silica sulfuric acid |
0.08 g |
92 |
3 |
Methane sulfonic acid |
0.1 mmol |
86 |
4 |
Sulfuric acid in acetic acid |
0.1 mmol |
56 |
5 |
No catalyst |
None |
10 |
Table 29 Influence of the catalytic amounts of xanthan sulfuric acid for synthesis of substituted coumarins
Entry |
Catalyst (g) |
Time (min) |
Yield (%) |
1 |
None |
60 |
Nil |
2 |
0.01 |
20 |
28 |
3 |
0.03 |
20 |
51 |
4 |
0.05 |
20 |
79 |
5 |
0.08 |
40 |
96 |
6 |
0.08 |
20 |
96 |
Singh and his coworkers reported the synthesis of substituted coumarins (7) via Pechmann condensation reaction between substituted phenols (6) and β-ketoesters (5) in presence of sulphamic acid (Scheme 28).87
 |
| Scheme 28 Pechmann condensation using sulphamic acid (SA) as catalyst. | |
Bose et al. reported the synthesis of substituted coumarins (7) via Pechmann condensation reaction between substituted phenols (6) and β-ketoesters (5) in presence of indium(III) chloride as an efficient catalyst (Scheme 29).88
 |
| Scheme 29 Synthesis of coumarins via von Pechmann condensation of phenols with β-ketoesters induced by In(III)Cl3. | |
An efficient and facile synthesis of substituted coumarins (7) was reported by one-pot reaction between substituted phenols (5) and β-ketoesters (6) in presence of new magnetic nanocomposites of ZrO2–Al2O3–Fe3O4 as green solid acid catalysts (Scheme 30).89
 |
| Scheme 30 Synthesis of 7-hydroxyl-4-methyl coumarin. | |
Mesoporous zirconium phosphate (m-ZrP) is used as solid acid catalyst for the synthesis of substituted coumarins (7) via Pechmann condensation reaction between substituted phenols (5) and β-ketoesters (6) in both conventional heating as well as microwave assisted method by Sinhamahapatra and his coworkers (Scheme 31).90 The effect of solvent on reaction was summarized in Table 30.
 |
| Scheme 31 Synthesis of substituted coumarins. | |
Table 30 Effect of different solvents on Pechmann condensation reaction for synthesis of substituted coumarins
Solvent |
Time (h) |
Temperature (°C) |
Yield (%) |
Nitrobenzene |
4 |
120 |
25 |
Toluene |
15 |
120 |
34 |
Solvent-free |
4 |
120 |
51 |
Solvent-free |
4 |
150 |
76 |
Tahanpesar and Sarami reported the synthesis of substituted coumarins (7) via one-pot Pechmann condensation reaction between substituted phenols (5) and β-ketoesters (6) in presence of sulfonated sawdust (SD-SO3H) as solid acid catalyst under solvent-free conditions (Scheme 32).91 Further, they observed the catalytic efficiency of SD-SO3H on the yield of product and results were presented in Table 31. They also observed the effects of different solvents viz. CHCl3, CH3CN, CH2Cl2, THF, MeOH and H2O and temperature on the synthesis of desired products and results were presented in Table 32.
 |
| Scheme 32 Synthesis of coumarins catalyzed by SD-SO3H under solvent-free conditions. | |
Table 31 Effect of SD-SO3H catalyst concentration on the yield of product
Entry |
Catalyst (g) |
Temperature (°C) |
Time (min) |
Yield (%) |
1 |
— |
90 |
120 |
0 |
2 |
0.025 |
90 |
120 |
40 |
3 |
0.05 |
90 |
75 |
72 |
4 |
0.075 |
90 |
120 |
70 |
5 |
0.10 |
90 |
120 |
60 |
6 |
0.15 |
90 |
120 |
60 |
Table 32 Effect of solvents and temperature on the synthesis of substituted coumarins
Entry |
Solvent |
Temperature (°C) |
Time (min) |
Yield (%) |
1 |
— |
90 |
75 |
72 |
2 |
CHCl3 |
Reflux |
200 |
35 |
3 |
CH3CN |
Reflux |
200 |
10 |
4 |
CH2Cl2 |
Reflux |
200 |
20 |
5 |
THF |
Reflux |
200 |
0 |
6 |
MeOH |
Reflux |
200 |
0 |
7 |
H2O |
Reflux |
200 |
0 |
8 |
— |
70 |
120 |
70 |
9 |
— |
110 |
25 |
91 |
10 |
— |
130 |
25 |
92 |
The plausible mechanism for the synthesis of substituted coumarins was presented in Fig. 14. The comparison of catalytic activity of SD-SO3H with other catalyst found in literature was presented in Table 33.
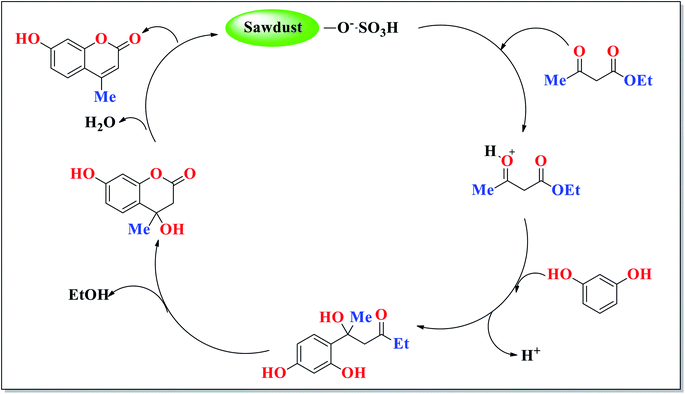 |
| Fig. 14 The plausible mechanism of formation of 7-hydroxy-4-methylcoumarin in presence of sulfonated sawdust (SD-SO3H) as solid acid catalyst. | |
Table 33 Comparison of catalytic activity of SD-SO3H with some other catalysts
Entry |
Catalyst |
Temperature (°C) |
Time (min) |
Yield (%) |
References |
1 |
SD-SO3H |
110 |
9 |
98 |
91 |
2 |
m-ZrP |
160 |
240 |
94 |
92 |
3 |
SCZ |
120 |
143 |
87 |
93 |
4 |
ASA |
100 |
30 |
98 |
94 |
5 |
CMK-5-SO3H |
130 |
20 |
95 |
95 |
6 |
H6P2W18O62·24H2O |
130 |
42 |
87 |
96 |
7 |
Zeolite-E4a |
110 |
180 |
97 |
97 |
8 |
HClO4·SiO2 |
130 |
35 |
95 |
98 |
Conclusion and future prospects
This review article summarized the synthesis of substituted coumarins using solid acid catalysts. Benefits of these methods include clean reaction profiles, minimization of side products, efficient and facile experimental procedures and inexpensive. This review is endeavouring to find potential future directions in the development of more potent and specific analogs of nitrogen and oxygen containing heterocyclic compounds for the biological target by the use of heterogeneous catalysts. The information illustrated in this review also encourage organic chemist for the design of novel molecules to identify many more biologically active heterocycles for the benefit of humanity.
Author contributions
Conceptualization: Susheel Gulati, Rajvir Singh, Suman Sangwan, formal analysis: Susheel Gulati, Suman Sangwan, investigation: Susheel Gulati, Rajvir Singh, Suman Sangwan, supervision: Susheel Gulati, Rajvir Singh, validation: Susheel Gulati, Suman Sangwan, writing-original draft: Susheel Gulati, writing-review & editing: Susheel Gulati, Rajvir Singh, Suman Sangwan.
Conflicts of interest
Authors declared that there is no conflict of interest regarding the publication of this paper.
Abbreviations
TSA | Tungstate sulphuric acid |
MW | Microwave irradiation |
PVPHS | Poly(4-vinylpyridinium) hydrogen sulfate |
SCCMNPs | Sulfonated carbon-coated magnetic nanoparticles |
SSA | Silica sulphuric acid |
XSA | Xanthan sulphuric acid |
Acknowledgements
The authors are thankful to the Department of Chemistry, Chaudhary Charan Singh Haryana Agricultural University, Hisar for providing the necessary facilities. Financial assistance from Department of Science and Technology (DST), New Delhi, India is gratefully acknowledged.
References
- X. He, Y. Shang, Y. Zhou, Z. Yu, G. Han, W. Jin and J. Chen, Tetrahedron, 2015, 71, 863–868 CrossRef CAS.
- N. Zhang, S. Ayral-Kaloustian, T. Nguyen, R. Hernandez, J. Lucas, C. Discafani and C. Beyer, Bioorg. Med. Chem., 2009, 17, 111–118 CrossRef CAS PubMed.
- X. He, Y. Chen, J. Shi, W. Tang, Z. Pan, Z. Dong, B. Song, J. Li and X. Liu, Bioorg. Med. Chem., 2014, 22, 3732–3738 CrossRef CAS PubMed.
- L. Huang, X. Yuan, D. Yu, K. Lee and C. Chen, Virology, 2005, 332, 623–628 CrossRef CAS PubMed.
- F. Borges, F. Roleira, N. Milhazes, L. Santana and E. Uriarte, Curr. Med. Chem., 2005, 12, 887–916 CrossRef CAS PubMed.
- H. Abd El-Wahab, M. Abd El-Fattah, N. Abd El-Khalik, H. Nassar and M. Abdelall, Prog. Org. Coat., 2014, 77, 1506–1511 CrossRef.
- G. Zhao, C. Peng, W. Du and S. Wang, Fitoterapia, 2013, 89, 250–256 CrossRef CAS PubMed.
- T. Smyth, V. Ramachandran and W. Smyth, Int. J. Antimicrob. Agents, 2009, 33, 421–426 CrossRef CAS PubMed.
- M. Razavi, H. Nazemiyeh, A. Delazar, R. Hajiboland, M. Rahman, S. Gibbons, L. Nahar and S. Sarker, Phytochem. Lett., 2008, 1, 159–162 CrossRef.
- N. Ballin and A. Sørensen, Food Control, 2014, 38, 198–203 CrossRef CAS.
- N. Khaligh, Catal. Sci. Technol., 2012, 2, 1633–1636 RSC.
- A. Fischer, C. Cremer and E. H. K. Stelzer, Appl. Opt., 1995, 34, 1989–2003 CrossRef CAS PubMed.
- G. Cravotto, G. M. Nano, G. Palmisano and S. Tagliapietra, Tetrahedron: Asymmetry, 2001, 12, 707–709 CrossRef CAS.
- I. B. Masesane and Z. Y. Desta, Beilstein J. Org. Chem., 2012, 8, 2166–2175 CrossRef CAS PubMed.
- B. M. Reddy, M. Patil and P. Lakshmanan, J. Mol. Catal. A: Chem., 2006, 256, 290–294 CrossRef CAS.
- B. C. Raju, T. H. Babu and J. M. Rao, Indian J. Chem. B, 2009, 48, 120–123 Search PubMed.
- N. Ahmed and Z. Siddiqui, J. Mol. Catal. A: Chem., 2014, 387, 45–56 CrossRef CAS.
- S. Sharma, P. Parikh and R. Jasra, Appl. Catal., A, 2010, 386, 34–42 CrossRef CAS.
- E. Kolvari, N. Koukabi and M. Hosseini, J. Mol. Catal. A: Chem., 2015, 397, 68–75 CrossRef CAS.
- S. Ghodke and U. Chudasama, Appl. Catal., A, 2013, 453, 219–226 CrossRef CAS.
- B. Movassagh, L. Tahershamsi and A. Mobaraki, Tetrahedron Lett., 2015, 56, 1851–1854 CrossRef CAS.
- D. R. Naikwadi, B. D. Bankar, K. Ravi and A. V. Biradar, Res. Chem. Intermed., 2021, 1–13, DOI:10.1007/s11164-021-04499-3.
- B. Karami, M. Farahi, N. Farmani and H. M. Tanuraghaj, New J. Chem., 2015, 1–5 Search PubMed.
- N. G. Khaligh, Ultrason. Sonochem., 2013, 20, 1062–1068 CrossRef CAS PubMed.
- Z. Z. Akbari, S. Dastmalchi, L. Edijlali, L. Dinparast and M. Es'haghi, Appl. Organomet. Chem., 2019, 1–14 Search PubMed.
- A. N. Nadaf and K. Shivashankar, J. Heterocycl. Chem., 2018, 55, 1375–1381 CrossRef CAS.
- A. Zhu, S. Bai, L. Li, M. Wang and J. Wang, Catal. Lett., 2015, 145, 1089–1093 CrossRef CAS.
- A. Zhu, M. Wang, L. Li and J. Wang, RSC Adv., 2015, 5, 73974–73979 RSC.
- Z. Samiei, S. S. Amiri and Z. Azizi, Mol. Diversity, 2019, 1–20 Search PubMed.
- M. A. Nasseri and S. M. Sadeghzadeh, J. Iran. Chem. Soc., 2014, 11, 27–33 CrossRef CAS.
- Z. Abbasi, S. Rezayati, M. Bagheri and R. Hajinasiri, Chin. Chem. Lett., 2017, 28(1), 75–82 CrossRef CAS.
- F. K. Esfahani, D. Zareyee and R. Yousef, ChemCatChem, 2014, 6(12), 3333–3337 CrossRef CAS.
- E. D. Glendening, NBO 6.0. Theoretical Chemistry Institute, University of Wisconsin, Madison, 2013 Search PubMed.
- D. Zareyee and M. Serehneh, J. Mol. Catal. A: Chem., 2014, 1, 88–91 CrossRef.
- M. Samadizadeh, S. Nouri and F. K. Moghadam, Res. Chem. Intermed., 2016, 42(6), 6089–6103 CrossRef CAS.
- R. Sun, Y. Gao, Y. Ma, G. Yang and Y. Li, J. Iran. Chem. Soc., 2017, 14, 737–742 CrossRef CAS.
- H. M. Altass and A. E. R. S. Khder, React. Kinet., Mech. Catal., 2018, 125(1), 227–243 CrossRef CAS.
- A. Mirosanloo, D. Zareyee and M. A. Khalilzadeh, Appl. Organomet. Chem., 2018, 32(12), e4546 CrossRef.
- N. H. Jadhav, S. S. Sakate, N. K. Rasal and R. A. Pawar, ACS Omega, 2019, 4(5), 8522–8527 CrossRef CAS PubMed.
- S. Pakdel, B. Akhlaghinia and A. Mohammadinezhad, Chem. Afr., 2019, 2(3), 10 Search PubMed.
- S. A. Khan, S. B. Khan, A. M. Asiri and I. Ahmad, Nanoscale Res. Lett., 2016, 11, 345 CrossRef PubMed.
- F. K. Esfahani, D. Zareyee and R. Zareyee, ChemCatChem, 2014, 6(12), 3333–3337 CrossRef CAS.
- V. Vahabi and F. Hatamjafari, Molecules, 2014, 19(9), 13093–13103 CrossRef PubMed.
- D. Zareyee and M. Serehneh, J. Mol. Catal. A: Chem., 2014, 391, 88–91 CrossRef CAS.
- S. S. Kumbar, K. M. Hosamani, G. C. Gouripur and S. D. Joshi, R. Soc. Open Sci., 2018, 5, 172416 CrossRef PubMed.
- F. M. Moghaddam, M. Eslami and G. Hoda, Sci. Rep., 2020, 10, 20968 CrossRef PubMed.
- L. N. Nasirmahale, O. G. Jolodar, F. Shirini and H. Tajik, Polycyclic Aromat. Compd., 2021, 41, 199–210 CrossRef.
- H. Mehrabi and H. Abusaidi, J. Iran. Chem. Soc., 2010, 7(4), 890–894 CrossRef CAS.
- J. M. Khurana and K. Vij, J. Chem. Sci., 2012, 124(4), 907–912 CrossRef CAS.
- S. Abdolmohammadi and S. Balalaie, Tetrahedron Lett., 2007, 48(18), 3299–3303 CrossRef CAS.
- A. R. Montaghami and N. Montazeri, Orient. J. Chem., 2014, 30(3), 1361–1364 CrossRef CAS.
- J. M. Khurana and S. Kumar, Monatsh. Chem., 2010, 141(5), 561–564 CrossRef CAS.
- M. Kidwai, V. Bansal, P. Mothsra, S. Saxena, R. K. Somvanshi, S. Dey and T. P. Singh, J. Mol. Catal., 2007, 268(1), 76–81 CrossRef CAS.
- A. Zhu, Sh Bai, L. Li, M. Wang and J. Wang, Catal. Lett., 2015, 145, 1089–1093 CrossRef CAS.
- K. P. Boroujeni and P. Ghasemi, Catal. Commun., 2014, 37, 50–54 CrossRef.
- A. Kiasat and L. Hemat-Alian, Res. Chem. Intermed., 2015, 41(2), 873–880 CrossRef CAS.
- K. M. Khan, S. Iqbal, M. A. Lodhi, G. M. Maharvi, Z. Ullah, M. I. Choudhary, A. ur Rahman and S. Perveen, Bioorg. Med. Chem., 2004, 12(8), 1963–1968 CrossRef CAS PubMed.
- A. P. Katariya, S. U. Deshmukh, S. U. Tekale, M. V. Katariya and R. P. Pawar, Lett. Appl. NanoBioSci., 2021, 10(3), 2525–2534 Search PubMed.
- G. Kaur, D. Singh, A. Singh and B. Banerjee, Synth. Commun., 2020, 1–14, DOI:10.1080/00397911.2020.1856877.
- N. H. Jadhav, S. S. Sakate, N. K. Rasal, D. R. Shinde and R. A. Pawar, ACS Omega, 2019, 4, 8522–8527 CrossRef CAS PubMed.
- S. Rahimi and E. Soleimani, Results Chem., 2020, 2, 1–7 Search PubMed.
- G. G. Carrillo, J. Gonzalez, M. J. E. Legaspi, G. J. L. Lopez, I. A. A. Villarreal, S. G. C. Magana, F. J. M. Martinez and R. M. Valencia, Microporous Mesoporous Mater., 2020, 307, 1–10 Search PubMed.
- A. E. R. S. Khder, S. A. Ahmed, K. S. Khairou and H. M. Altass, J. Porous Mater., 2018, 25, 1–13 CrossRef CAS.
- M. Kour and S. Paul, Monatsh. Chem., 2017, 148, 327–337 CrossRef CAS.
- A. Sinhamahapatra, N. Sutradhar, S. Pahari, H. C. Bajaj and A. B. Panda, Appl. Catal., A, 2011, 394, 93–100 CrossRef CAS.
- R. Sun, Y. Gao, Y. Ma, G. Yang and Y. Li, J. Iran. Chem. Soc., 2017, 14, 737–742 CrossRef CAS.
- K. C. Prousis, N. Avlonitis, G. A. Heropoulos and T. Calogeropoulou, Ultrason. Sonochem., 2014, 21, 937–942 CrossRef CAS PubMed.
- A. Mobaraki, S. Yasham and B. Movassagh, Synlett, 2015, 26, 1263–1268 CrossRef CAS.
- D. Zareyee and M. Serehneh, J. Mol. Catal. A: Chem., 2014, 391, 88–91 CrossRef CAS.
- B. Karimi and D. Zareyee, Org. Lett., 2008, 10, 3989–3992 CrossRef CAS PubMed.
- S. Selvakumar, M. Chidambaram and A. P. Singh, Catal. Commun., 2007, 8, 777–783 CrossRef CAS.
- H. Saffarian, F. Karimi, M. Yarie and M. A. Zolfigol, J. Mol. Struct., 2020, 1224, 1–12 Search PubMed.
- S. Bouasla, J. A. Gahete, D. Esquivel, M. I. Lopez, C. J. Sanchidrian, M. Teguiche and F. J. R. Salguero, Molecules, 2017, 22, 1–8 CrossRef PubMed.
- A. Maleki, P. Ravaghi, M. Aghaie and H. Movahed, http://sciforum.net/conference/ecsoc-20.
- V. D. Suryawanshi, A. H. Gore, P. V. Anbhule, S. R. Patil and G. B. Kolekar, J. Shivaji Univ., 2017, 42(1), 40–45 Search PubMed.
- E. Rostami and S. H. Zare, ChemistrySelect, 2019, 4, 13295–13303 CrossRef CAS.
- Y. F. Sun, J. M. Liu, J. Sun, Y. T. Haung, J. Lu, M. M. Li, N. Jin, X. F. Dai and B. Fan, Molecules, 2018 DOI:10.20944/preprints201809.0349.v1.
- B. S. Kuram, J. V. Madhav, S. Vijaya Laxmi, B. Rajitha, Y. Thirupathi Reddy, P. Narsimha Reddy and P. A. Crooks, Synth. Commun., 2010, 1770–1777 Search PubMed , http://www.tandfonline.com/loi/lsyc20.
- S. Palaniappan and A. John, J. Mol. Catal., 2005, 233, 9–15 CrossRef CAS.
- E. Kolvari, N. Koukabi and M. M. Hosseini, J. Mol. Catal. DOI:10.1016/j.molcata.2014.10.026.
- A. Amoozadeh, M. Ahmadzadeh and E. Kolvari, J. Chem., 2012, 2013, 1–7 Search PubMed.
- D. Zareyee and M. Serehneh, J. Mol. Catal. A: Chem., 2014, 391, 88–91 CrossRef CAS.
- B. M. Reddy, V. R. Reddy and D. Giridhar, Synth. Commun., 2006, 31(23), 3603–3607 CrossRef.
- S. Kim, D. Kang, C. H. Lee and P. H. Lee, J. Org. Chem., 2012, 77, 6530–6537 CrossRef CAS PubMed.
- M. Maheswara, V. Siddaiah, G. Lakishmi, V. Damu, Y. K. Rao and C. V. Rao, J. Mol. Catal. A: Chem., 2006, 255, 49–52 CrossRef CAS.
- B. S. Kuram, J. V. Madhav and B. Rajitha, Synth. Commun., 2011, 42(12), 1770–1777 CrossRef.
- P. R. Singh, D. U. Singh and S. D. Samant, Synlett, 2004, 11, 909–1912 Search PubMed.
- D. S. Bose, A. P. Rudradas and M. H. Babu, Tetrahedron Lett., 2002, 43, 9195–9197 CrossRef.
- A. Wang, X. Lu, Z. Su and H. Jing, Catal. Sci. Technol., 2014, 4, 71–80 RSC.
- A. Sinhamahapatra, N. Sutradhar, S. Pahari, H. C. Bajaj and A. B. Panda, Appl. Catal., A, 2011, 394, 93–100 CrossRef CAS.
- E. Tahanpesar and L. Sarami, Russ. J. Gen. Chem., 2015, 85(9), 2135–2140 CrossRef CAS.
- A. Sinhamahapatra, N. Sutradhar, S. Pahari, H. C. Bajaj and A. B. Panda, Appl. Catal., A, 2011, 394, 93 CrossRef CAS.
- B. M. Reddy, M. K. Patil and P. J. Lakshmanan, J. Mol. Catal. A: Chem., 2006, 256, 290 CrossRef CAS.
- A. Amoozadeh, M. Ahmadzadeh and E. Kolvari, J. Chem., 2013, 2013, 6 Search PubMed.
- D. Zareyee and M. J. Serehneh, J. Mol. Catal. A: Chem., 2014, 391, 88 CrossRef CAS.
- G. P. Romanelli, D. Bennardi, D. M. Ruiz, G. Baronetti, H. J. Thomas and J. C. Autino, Tetrahedron Lett., 2004, 45, 8935 CrossRef CAS.
- A. Hegedüs and Z. Hell, Catal. Lett., 2006, 112, 105 CrossRef.
- M. Maheswara, V. Siddaiah, G. L. V. Damu, Y. K. Rao and C. V. Rao, J. Mol. Catal. A: Chem., 2006, 255, 49 CrossRef CAS.
|
This journal is © The Royal Society of Chemistry 2021 |
Click here to see how this site uses Cookies. View our privacy policy here.