DOI:
10.1039/C2BM00108J
(Paper)
Biomater. Sci., 2013,
1, 379-392
The effect of silicate ions on proliferation, osteogenic differentiation and cell signalling pathways (WNT and SHH) of bone marrow stromal cells†
Received
6th August 2012
, Accepted 15th November 2012
First published on 12th December 2012
Abstract
Silicon (Si) is a trace element, which plays an important role in human bone growth. Si has been incorporated into biomaterials for bone regeneration in order to improve their osteogenic potential, both in vitro and in vivo. Little is known, however, as to how Si ions elicit their biological response on bone-forming cells. The aim of this study was to investigate the effect of Si ions on the proliferation, differentiation, bone-related gene expression and cell signalling pathways of bone marrow stromal cells (BMSCs) by comparing the BMSC responses to different concentrations of NaCl and Na2SiO3, while taking into account and excluding the effect of Na ions. Our study showed that Si ions at a concentration of 0.625 mM significantly enhanced the proliferation, mineralization nodule formation, bone-related gene expression (OCN, OPN and ALP) and bone matrix proteins (ALP and OPN) of BMSCs. Furthermore, Si ions at 0.625 mM could counteract the effect of the WNT inhibitor (W.I.) cardamonin on the osteogenic genes expression, (OPN, OCN and ALP), WNT and SHH signalling pathway-related genes in BMSCs. These results suggest that Si ions by themselves play an important role in regulating the proliferation and osteogenic differentiation of BMSCs, with the involvement of WNT and SHH signalling pathways. Our study provides evidence to explain possible molecular mechanisms whereby Si ions released from Si-containing biomaterials can acquire enhanced bioactivity at desired concentration.
1. Introduction
For the past thirty years, significant attention has been paid to silicon (Si), due to the studies of Carlisle and Schwarz, who showed that Si is an important factor in skeletal development and repair.1,2 Si has been found to be an important trace element in human body. Si is located at active calcification sites in the bones and is directly involved in the mineralization process of bone growth.1 Due to this significant function of Si in human bone, Si has been widely incorporated into Ca–P bioceramics in order to enhance their bioactivity.3–8 Typically, Si-doped hydroxyapatite and tricalcium phosphate ceramics have been prepared. It has been found that Si-doped Ca–P bioceramics have improved biological performance compared to pure Ca–P ceramics.6,9–11 Silicate-based bioactive glasses and ceramics have also been widely studied for bone regeneration applications.12–16 The significant advantages of this family of materials are that Si-containing ionic products from these biomaterials significantly enhance the proliferation and osteogenic differentiation of a series of stem cells, including bone marrow stromal cells, human dental pulp cells, periodontal ligament cells, and adipose-derived stem cells.17–23 Our recent study found that silicate ceramics have significantly enhanced in vivo bone formation in a form of lactide-co-glycolide microspheres.24 Some silicate ceramics, CaSiO3 and Ca2MgSi2O7, have significantly improved in vivo osteogenesis compared to conventional β-tricalcium phosphate ceramics.25,26 These in vitro and in vivo studies seem to indicate that Si may play an important role in stimulating the biological response of bone-forming cells and further enhancing the osteogenic bioactivity of biomaterials. However, as reported by Bohner in his leading opinion paper, until now there has been no obvious and direct evidence linking the improved biological performance of Si-substituted calcium phosphates to Si release.27 Similarly, for silicate bioactive glasses and ceramics, their ionic products contain not only Si, but also other important biological ions, such as Na, Mg, Zn, Ca, etc.22,28,29 It is difficult to claim that silicate-based bioactive glasses and ceramics have improved in vitro and in vivo bioactivity due to the Si ions. It is therefore of great importance to investigate the role of Si ions themselves on the biological response of bone-forming cells. Previously, Zou et al. applied sodium silicate (Na2SiO3) to study the effect of Si ions on human osteoblast adhesion, proliferation and alkaline phosphatase (ALP) activity and found that 30 mM of Si ions could enhance the metabolic activity of osteoblasts.30 However, in their study, Na ions were also incorporated into cell culture medium, which may also influence cell response.30 The study has, therefore, not evaluated independently the effect of Si ions on cell response. Although Reffitt and Duivenvoorden found that orthosilicic acid (H4SiO4) could stimulate collagen type 1 synthesis and osteoblastic differentiation of human osteoblasts, it was still unclear how Si ions themselves influence cell response.31,32 To the best of our knowledge, there are few studies concerning the influence of Si ions on bone-related gene expression and cell signalling pathways of bone-forming cells. The aim of this study was therefore to systematically investigate the effects of Si ions on the proliferation, differentiation, nodule formation, bone-related gene expression and signalling pathways of BMSCs by comparing the response of BMSCs to various concentrations of NaCl and Na2SiO3 in order to exclude the effect of Na ions. Here we provide some evidence of the important role of Si ions in Si-containing biomaterials in influencing the cell response during osteogenic differentiation.
2. Experimental section
2.1 Preparation of cell culture medium containing different concentrations of Na2SiO3 and NaCl
In order to investigate the effect of Si ions on the proliferation, differentiation, bone-related gene expression and signalling pathways of BMSCs, two groups of studies were carried out with the same concentration of Na ions. Cells in group A were cultured with different concentrations of NaCl (A group: NaCl), while cells in group B were treated with a range of concentrations of Na2SiO3 (B group: Na2SiO3), as shown in Table 1. As group A and group B have the same concentration of Na ions in each corresponding concentration pair (Table 1), the effect of Si ions on cell response could be studied through the fold change by comparing group A and group B (fold change > 1: Si ions stimulating cell response; fold change < 1: Si ions down-regulating cell response). To prepare the stock solutions of Na2SiO3 and NaCl, Na2SiO3 and NaCl powders were dissolved into low glucose Dulbecco's Modified Eagle Medium (DMEM; Invitrogen Pty Ltd., Australia) at concentrations of 50 mM and 100 mM, respectively. After sufficient dissolution, serial concentrations of NaCl and Na2SiO3 were prepared using DMEM medium. Subsequently, the original and diluted solutions were sterilized using 0.2 μm filter and supplemented with fetal calf serum (FCS, 10% by volume; InVitro Technology, Australia) and penicillin/streptomycin (P/S, 1% by volume; Invitrogen Pty Ltd., Australia) for further cell culture experiments.
Table 1 Corresponding concentrations of Na and Si ions in two groups (A: NaCl; B: Na2SiO3) (mM)
Group A |
Na |
10 |
5 |
2.5 |
1.25 |
0.125 |
0.0125 |
|
Si |
0 |
0 |
0 |
0 |
0 |
0 |
Group B |
Na |
10 |
5 |
2.5 |
1.25 |
0.125 |
0.0125 |
|
Si |
5 |
2.5 |
1.25 |
0.625 |
0.0625 |
0.00625 |
2.2 Isolation and culture of BMSCs
Isolation and culture of BMSCs was performed as described before.24,33 BMSCs were isolated from bone marrow aspirates from patients (mean age: 65 years) undergoing elective knee and hip replacement surgery, with consent by all patients involved and ethical approval by the Human Ethics Committees of Queensland University of Technology and The Prince Charles Hospital. In brief, to prevent coagulation, 2 mL of heparin was mixed with 10 mL of bone marrow aspirate and then diluted with 12 mL of PBS. Then, using a serological pipette, 12 mL of a density gradient solution (Lymphoprep; Axis-Shield PoC AS, Oslo, Norway) was carefully placed underneath the bone marrow solution and centrifuged at 800 rpm for 10 min. The resulting supernatants were cultured in DMEM supplemented with 10% v/v fetal bovine serum, 1% v/v P/S at 37 °C in a humidified 5% CO2 incubator. The medium was then changed after five days to remove non-adherent cells. The cells were passaged at approximately 80% confluence and expanded through two passages to obtain a sufficient number of cells for the in vitro assays.
2.3 The effect of Si on the cell proliferation of BMSCs
The proliferation of BMSCs in two groups was carried out with a colorimetric 3-(4,5-dimethyl-2-thiazolyl)-2,5-diphenyl-2H-tetrazolium bromide (MTT) assay as described before.24,33 Briefly, BMSCs were cultured in 96-well plates at an initial density of 5000 cells per well. On days 1 and 7, 10 μL of 5 mg mL−1 of MTT solution (Sigma-Aldrich, Australia) were added to each well and incubated at 37 °C to form formazan crystals. After 4 h, the medium was removed and dimethyl sulfoxide (DMSO) was added to dissolve the MTT-formazan product. The absorbance for the MTT-formazan was measured at λ 495 nm with a microplate reader (SpectraMax, Plus 384, Molecular Devices, Inc., USA).
2.4 The effect of Si on alkaline phosphatase (ALP) activity of BMSCs
BMSCs were cultured in 24-well culture plates with a seeding density of 1 × 105 cells per well. ALP activity was assessed at day 7 and 14 in BMSCs cultured in different concentrations of NaCl and Na2SiO3 using the method as published before.24,33 Briefly, the cells were lysed in 200 μL of 0.2% Triton® X-100 and then centrifuged at 14
000 rpm for 15 min at 4 °C. 50 μL supernatants were mixed with 150 μL working solution according to the manufacturer's protocol (QuantiChromTM Alkaline Phosphatase Assay Kit, BioAssay Systems, USA). The total protein content was measured by the bicinchoninic acid protein assay kit (Sigma-Aldrich, Australia). The relative ALP activity was then obtained as the changed optical density (OD) values divided by the reaction time and total protein content.
2.5 The effect of Si on nodule formation of BMSCs
In order to identify mineralization-like nodule formation, Alizarin Red S staining was performed on day 14 after the BMSCs were cultured in different concentrations of NaCl and Na2SiO3. The cells were fixed in cold 100% methanol for 10 min, then stained in a solution of 1% Alizarin Red S at pH 4.1 for 10 min and then washed with H2O. The samples were air dried and photos were taken under a light microscope.
2.6 The effect of Si on bone-related gene expression of BMSCs
To investigate the effect of Si on the gene expression of Alkaline Phosphatase (ALP), osteocalcin (OCN), osteopontin (OPN), the total RNA was extracted from BMSCs cultured in different concentrations of NaCl and Na2SiO3 using Trizol reagent (Invitrogen Pty Ltd., Australia) and complementary DNA was synthesized using Superscript III reverse transcriptase (Invitrogen Pty Ltd., Australia) from 1 μg of the total RNA, following the manufacturer's instructions. RT-qPCR primers (Table 2) were designed based on cDNA sequences from the NCBI Sequence database using the Primer Express® software, and the primer specificity was confirmed by BLASTN searches. RT-qPCR was performed on an ABI Prism 7300 Thermal Cycler (Applied Biosystems, Australia) with SYBR Green detection reagent following the protocol published previously.24,33 The relative mRNA expressions of ALP, OPN and OCN were assayed and normalized against the house keeping gene 18s. Each sample was performed in triplicate. The mean cycle threshold (Ct) value of each target gene was normalized against the Ct value of housekeeping gene 18s ribosomal RNA and the relative expression calculated using the following formula: 2−(normalized average Cts) × 104.
Table 2 Primer pairs used in qRT-PCR analysis
Gene |
Forward primer |
Reverse primer |
OCN |
5′ GCAAAGGTGCAGCCTTTGTG 3′ |
5′ GGCTCCCAGCCATTGATACAG 3′ |
OPN |
5′ TCACCTGTGCCATACCAGTTAA 3′ |
5′ TGAGATGGGTCAGGGTTTAGC 3′ |
ALP |
5′ TCAGAAGCTAACACCAACG 3′ |
5′ TTGTACGTCTTGGAGAGGGC 3′ |
AXIN2 |
5′ CCCCAAAGCAGCGGTGC 3′ |
5′ GCGTGGACACCTGCCAG 3′ |
β-CATENIN |
5′ GCTACTGTTGGATTGATTCGAAATC 3′ |
5′ CCCTGCTCACGCAAAGGT 3′ |
SHH |
5′ CGCCAGCGGAAG GTATGAAG 3′ |
5′ CAACTTGTCCTTACACCTCTGAGTCA 3′ |
PTCH1 |
5′ GAGTCCCTTTCGTCATGCTAGGTC 3′ |
5′ GCC CCTATGCCAAAGGTGACC 3′ |
18s |
5′ TTCGGAACTGAGGCCATGAT 3′ |
5′ CGAACCTCCGACTTCGTTC 3′ |
2.7 The effect of Si on WNT and SHH signalling pathways of BMSCs
To investigate the effect of different concentrations of Si on the expression of WNT and SHH signalling related genes of BMSCs, measurements of the gene expression of axins inhibition protein 2 (AXIN2), beta-catenin (β- CATENIN or CTNNB), sonic hedgehog homolog (SHH) and protein patched homolog (PTCH1) were carried out. Cells were collected at two time points (day 7 and 14) and RT-PCR was carried out using the same method as described in Section 2.6.
To further investigate the effect of Si ions on cell proliferation in relation to the WNT signalling pathway, cardamonin, an effective W.I., was added at a concentration of 10 μM directly to the culture medium containing a specific concentration of Si ions. After 1, 3 and 7 days, cells were collected and MTT assays were performed using the same method described in Section 2.3. When indicated, the effect of Si ions on cell response was displayed using the fold changes by comparing corresponding concentration of group A and group B (fold change > 1: Si ions stimulating cell response; fold change < 1: Si ions down-regulating cell response).
The effect of Si ions on osteogenic differentiation and WNT/SHH cell signalling pathways in the present of a W.I. was also investigated. Cells were cultured in the presence of 10 μM of cardamonin in culture media containing NaCl and Na2SiO3. After 3 and 7 days, cells were collected and the expression of osteogenic genes (ALP, OCN, OPN), AXIN2, CTNNB, SHH and PTCH1 was performed with RT-PCR using the same method described in Section 2.6. When indicated, the effect of Si ions on cell response was measured using the same method described in Section 2.1.
2.8 The effect of Si on bone-related protein of human BMSCs (hBMSCs) by western blotting analysis
Western blotting analysis was performed for the direct detection of the ALP, OPN and OCN protein expression. In brief, whole cell lysates were obtained from cells cultured with 0.625 mM Na2SiO3 and 1.25 mM NaCl for one week. 10 μg proteins from each sample were separated on SDS-PAGE gels and then transferred onto a nitrocellulose membrane (Pall Corporation, East Hills, NY, USA) and blocked in 5% non-fat milk. The membranes were incubated with primary antibodies against ALP (1
:
1000, rabbit anti-human; Abcam, Sapphire Bioscience Pty. Ltd., Australia), OPN (1
:
1000, rabbit anti-human; Thermo Fisher Scientific, USA), OCN (1
:
1000, rabbit anti-human; Thermo Fisher Scientific, USA) and GAPDH (1
:
1000, mouse anti-human; Abcam, Sapphire Bioscience Pty. Ltd., Australia) overnight at 4 °C. The membranes were washed three times in TBS-Tween buffer, and then incubated with anti-mouse/rabbit HRP conjugated secondary antibodies at 1
:
4000 dilutions for 1 h at room temperature. The protein bands were visualized using the SuperSignal West Femto Maximum Sensitivity Substrate (Thermo Fisher Scientific, Australia) and exposed on X-ray films (Fujifilm, Australia). The relative intensity of protein bands was quantified using ImageJ software.
2.9 Statistical analysis
The experiments were carried out in triplicate. Analysis was performed using SPSS software (SPSS Inc., Chicago, IL, USA). All the data were expressed as mean ± standard deviation (SD) and were analysed using one-way ANOVA with a Post Hoc test. A p-value < 0.05 was considered statistically significant.
3. Results
3.1 The effect of Si on cell proliferation
An MTT proliferation assay showed that NaCl at high concentration (2.5–10 mM) decreased the cell number and proliferation of BMSCs at both day 1 and day 7; whereas there was no significant difference in the BMSC proliferation in other NaCl concentrations in comparison with that in normal culture media (blank control) (Fig. 1a). The concentrations of Na2SiO3, on the other hand, had an obvious effect on cell proliferation (Fig. 1b). The cell viability at the low end of the Na2SiO3 concentration ranges (0.00625 to 2.5 mM) was much higher than at high concentrations (5 mM) and there was a trend of stimulation of cell proliferation with increased concentrations of Si (0.00625 to 2.5 mM) (Fig. 1b). By comparing the viability and proliferation of BMSCs between NaCl and Na2SiO3, it is clear that concentrations of Si of 1.25 mM and 2.5 mM had the effect of increasing cell viability at day 1 and proliferation at day 7 (fold change > 1), while Si at 5 mM inhibited cell proliferation of BMSCs (Fig. 1c).
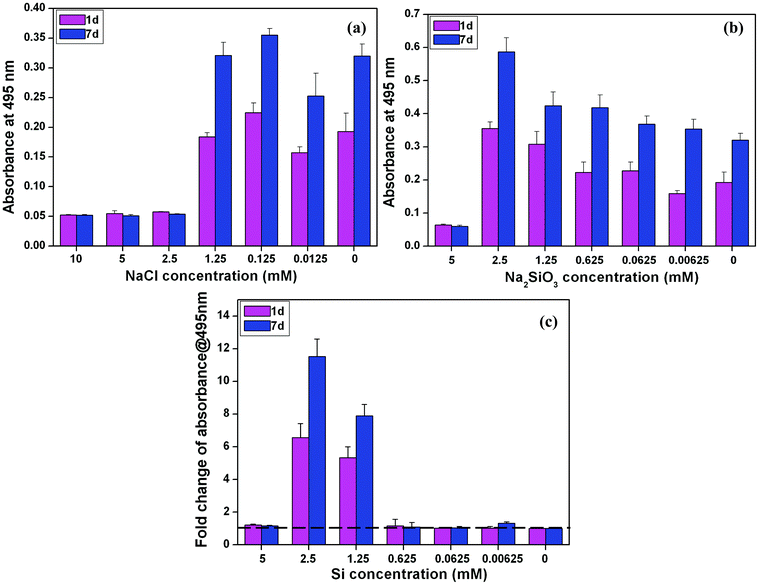 |
| Fig. 1 BMSCs viability at day 1 and proliferation at day 7 in medium containing different concentrations of NaCl (a) and Na2SiO3 (b) and the fold change of cell proliferation with different Si concentrations (c) after comparing the groups of NaCl and Na2SiO3. At both day 1 and 7, Si ions at the concentration of 2.5 and 1.25 mM significantly enhanced cell viability and proliferation (fold change > 1) (c). The dotted line shows a fold change of 1 in cell proliferation between NaCl and Na2SiO3 groups, indicating there is no difference between these two groups. | |
3.2 The effect of Si on ALP activity and mineralization nodule formation of BMSCs
The NaCl concentration had no significant effect on the ALP activity of BMSCs (Fig. 2a). At the lower NaCl concentration of 0.0125 mM, ALP activity showed an increase over time (Fig. 2a). The ALP activity of BMSCs cultured in low concentrations of Na2SiO3 (0.00625–1.25 mM) showed no difference and was decreased at high concentrations (2.5 and 5 mM) at both 7 and 14 days (Fig. 2b). A comparison of ALP activities between cells cultured in NaCl and Na2SiO3 revealed that the relative ALP activity increased significantly at an Si concentration of 0.00625 mM on day 7 (fold change = 1.7) and day 14 (fold change = 1.1) (Fig. 2c). There were no stimulatory effects at other Si concentrations; in contrast, ALP activity was reduced (Fig. 2c).
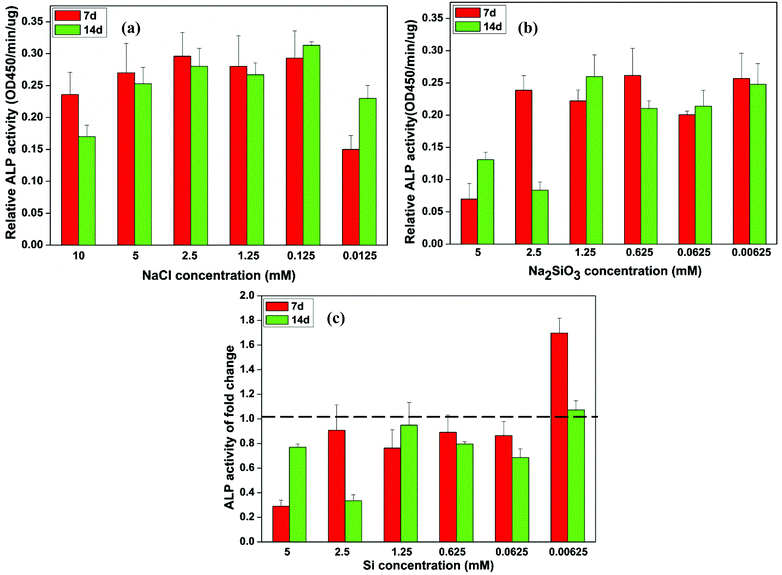 |
| Fig. 2 The relative ALP activity of BMSCs observed in culture with different concentrations of NaCl (a) and Na2SiO3 (b) and the independent Si effect shown by the fold change of ALP activity between the treatment groups of NaCl and Na2SiO3 (c). At days 7 and 14, a concentration of Si ions of 0.00625 mM significantly increased the ALP activity of BMSCs (∼1.7 and 1.1 fold change, respectively); in contrast, ALP activity of BMSCs decreased at other concentrations of Si (fold change < 1) (c). The dotted line indicates a fold change of ALP activity of 1 between NaCl and Na2SiO3 groups. | |
Alizarin Red S staining showed evidence of nodule formation and calcium deposition. It was noted that mineralized nodules were seen at all concentrations of Na2SiO3 (Fig. 3) and the nodules became more distinct with increased concentration of Na2SiO3 (Fig. 3 right column). There was no obvious effect on Ca mineralization seen in the NaCl groups (Fig. 3 left column).
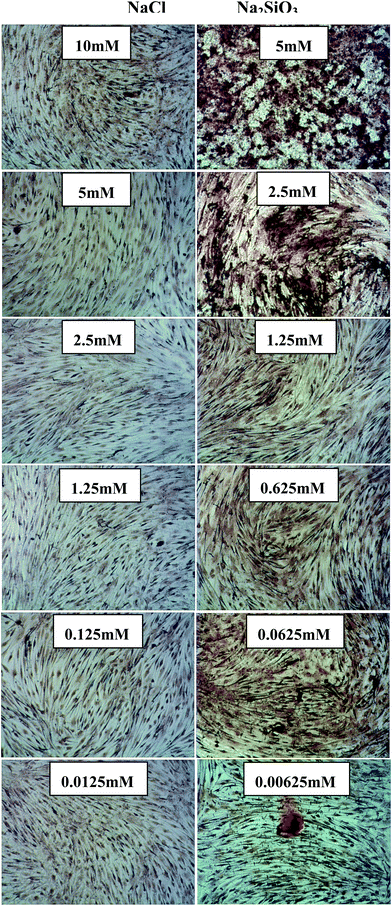 |
| Fig. 3 Alizarin Red S staining of BMSCs treated with different concentrations of NaCl (left column) and Na2SiO3 (right column). There is no obvious nodule formation and calcium deposition in NaCl group, however, Na2SiO3 increased nodule formation and slightly enhanced the calcium deposition in BMSC cultures. | |
3.3 The effect of Si on bone-related gene expression of BMSCs
At day 7, the mRNA expression of OPN in BMSCs increased as NaCl concentration decreased. At day 14, OPN expression was increased in NaCl concentrations of 0.0125 and 5 mM compared to the other concentrations and also had increased over time (Fig. 4a). OPN expression increased over time with most Na2SiO3 concentrations. A comparison of OPN expression between the NaCl and Na2SiO3 groups showed that, at day 7, Si significantly enhanced OPN expression across the concentration range (fold change > 1) and 0.625 mM Si in particular stood out with a 25 fold increase of OPN expression (Fig. 4c). At day 14, OPN expression was reduced at high Si concentrations (2.5 and 5 mM) and expression was highest at 0.625 mM Si (∼5 fold change) compared to the other concentrations (Fig. 4c).
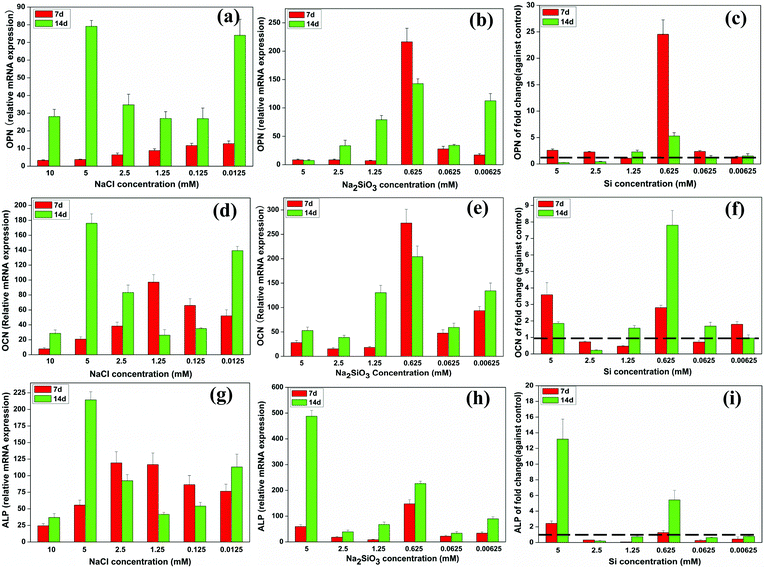 |
| Fig. 4 The expression of osteogenic genes of BMSCs cultured with a range of concentrations of NaCl (a, d, g) and Na2SiO3 (b, e, h). The effect of Si icon on osteogenic genes expression by comparing treatments with NaCl and Na2SiO3 (c, f, i). At both days 7 and 14, Si ions significantly enhanced OPN (c), OCN (f) and ALP (i) gene expression at a concentration of 0.625 mM (fold change > 1). The dotted line indicates a fold change of gene expression of 1 between NaCl and Na2SiO3 groups. | |
The relative mRNA expression of OCN in BMSCs at day 7 was higher at 1.25 mM NaCl than at the other concentrations and, at day 14, OCN expression was significantly increased at 0.0125 and 5 mM of NaCl (Fig. 4d). The OCN expression level of cells treated with 0.625 mM of Na2SiO3 was significantly higher than in other concentrations at both days 7 and 14 (Fig. 4e). Comparing the OCN expression of the NaCl and Na2SiO3 groups revealed a reduced expression of OCN at day 7 in 0.0625, 1.25 and 2.5 mM of Si (fold change < 1); at the other Si concentrations OCN expression increased (fold change > 1). At day 14, OCN expression increased strongly at 0.625 mM of Si by an 8 fold change compared to the other concentrations (Fig. 4f).
The relative mRNA expression of ALP rose gradually and peaked at 2.5 mM of NaCl at day 7. 5 mM of NaCl caused a significant increase in ALP expression at day 14 (Fig. 4g). ALP expression increased at 0.625 mM of Na2SiO3 at both days 7 and 14 (Fig. 4h). The effect of Si ions alone showed that, at day 7, 5 mM of Si had the greatest effect of increasing ALP expression in BMSCs (fold change > 1). Other Si concentrations had the effect of decreasing ALP expression (fold change < 1). At day 14, Si concentrations at 0.625 and 5 mM significantly enhanced ALP expression (fold change > 1), but other concentrations led to decreased expression (fold change < 1) (Fig. 4i).
3.4 The effect of Si on WNT and SHH signalling pathway-related genes expression of BMSCs
Quantitative RT-PCR analysis indicated that relative AXIN2 mRNA expression peaked at 2.5 and 5 mM of NaCl at days 7 and 14, respectively (Fig. 5a). Na2SiO3 dramatically enhanced AXIN2 expression across the range of concentrations over time (Fig. 5b). The effect on AXIN2 expression of Si ions alone was deduced by comparing the NaCl and Na2SiO3 groups. At day 7, Si concentration of 1.25 and 2.5 mM slightly decreased AXIN2 expression (fold change < 1), whereas the other Si concentrations enhanced AXIN2 expression (fold change > 1). At day 14, AXIN2 expression rose across the Si concentration range (fold change > 1), especially so at 0.625 mM, which had a 32 fold expression change (Fig. 5c).
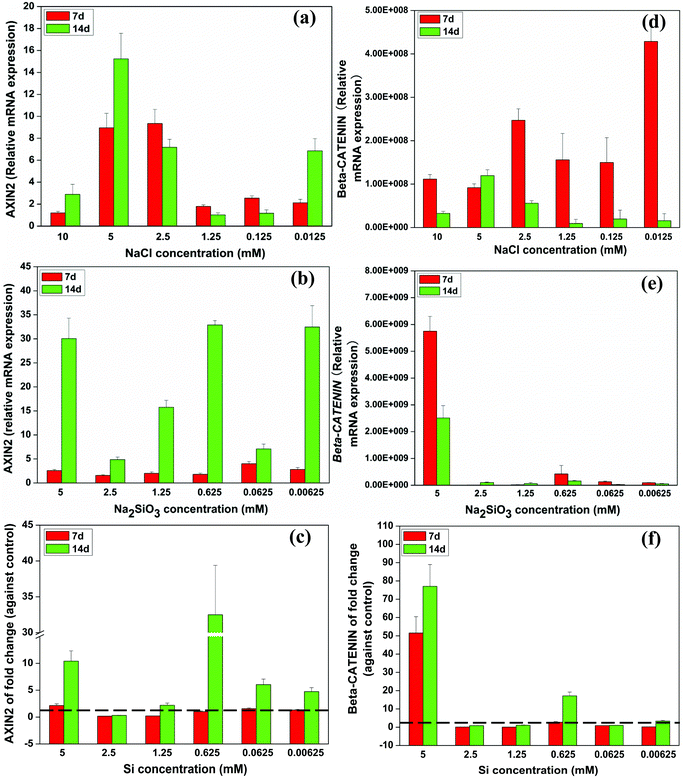 |
| Fig. 5 Relative expression of WNT-related genes of BMSCs cultured with a range of concentrations of NaCl (a, d) and Na2SiO3 (b, e). Comparing groups with NaCl and Na2SiO3, effect of different concentrations of Si ions on the fold change of AXIN2 and CTNNB gene expression of BMSCs (c, f). At day 14, Si ions at a concentration of 0.625 mM increased AXIN2 (c), and CTNNB (f) expression (fold change > 1). The dotted line indicates a fold change of gene expression of 1 between NaCl and Na2SiO3 groups. | |
The expression of β-CATENIN (CTNNB) rose significantly at day 7 in 0.0125 and 2.5 mM of NaCl compared to the other concentrations (Fig. 5d). Na2SiO3 at 5 mM increased CTNNB gene expression of BMSCs (Fig. 5e) and the fold change of CTNNB expression as the result of Si alone confirmed that Si at 5 mM had the greatest influence on CTNNB expression of BMSCs over time. At days 7 and 14, the Si concentration of 0.625 mM also induced a distinct increase in CTNNB expression (Fig. 5f).
The relative mRNA expression of sonic hedgehog (SHH) in BMSCs gradually decreased with decreasing concentrations of NaCl from 10 mM to 1.25 mM at day 7, but expression of SHH was increased by NaCl at the concentrations of 0.125 and 0.0125 mM (Fig. 6a). The relative SHH expression increased across the whole Na2SiO3 concentration range over time (Fig. 6b). The effect of Si alone when comparing the NaCl and Na2SiO3 groups showed that Si at 0.625 mM dramatically enhanced SHH gene expression 38 and 93 fold at days 7 and 14, respectively (Fig. 6c).
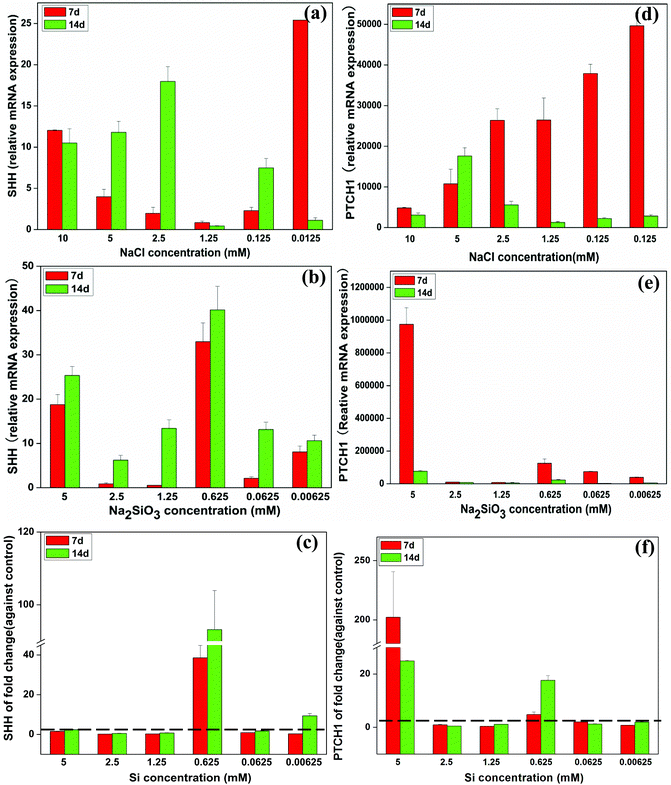 |
| Fig. 6 The expression of SHH-related genes of BMSCs in the presence of a range of concentrations of NaCl (a, d) and Na2SiO3 (b, e). After comparing groups containing NaCl and Na2SiO3, effect of different concentrations of Si ions on the fold change of SHH and PTCH1 gene expression of BMSCs (c, f). At day 14, Si ions at a concentration of 0.625 mM enhanced SHH (c) and PTCH1 (f) expression (fold change > 1). The dotted line indicates a fold change of gene expression of 1 between NaCl and Na2SiO3 groups. | |
At day 7, the mRNA expression of patched-1 (PTCH1) increased as NaCl concentrations decreased. PTCH1 expression reached a maximum at day 14 at a concentration of 5 mM NaCl (Fig. 6d). Na2SiO3 at 5 mM induced the highest PTCH1 expression at both day 7 and 14 (Fig. 6e). Allowing for the effect of Na+ ions, Si ions at 5 mM increased PTCH1 gene expression 200 and 20 fold at days 7 and 14, respectively. Si at 0.625 mM also enhanced PTCH1 expression at both day 7 and 14 by a ∼4 and 17 fold change, respectively (Fig. 6f).
3.5 The effect of Si on BMSCs proliferation, osteogenic gene expression, and signalling pathway-related genes in the presence of W.I.
The effect of cardamonin (W.I.) at a concentration of 10 μM on the BMSC proliferation (A) and WNT-related gene expression (B) was evaluated (ESI, Fig. S1†). The cell proliferation of BMSCs was significantly decreased in the presence of cardamonin (A). The fold change of WNT related genes of AXIN2 and CTNNB was also significantly inhibited by the W.I. cardamonin (B) (fold change < 1).
Interestingly, it was noted that when the culture media contained Si ions at a concentration of 0.625 mM, the number of BMSCs cultured with the W.I. was higher than that without W.I. (Fig. 7a). However, at both day 3 and 7, the fold change of mRNA expression of OPN, OCN and ALP was significantly enhanced by 0.625 mM of Si ions in the presence of cardamonin at 10 μM (fold change > 1) (Fig. 7b–d). Furthermore, when the culture media contained both W.I. and Si ions, the mRNA expression of AXIN2, CTNNB, SHH, and PTCH1 was significantly enhanced at day 3 (fold > 1), then decreased at day 7 (Fig. 7e–h).
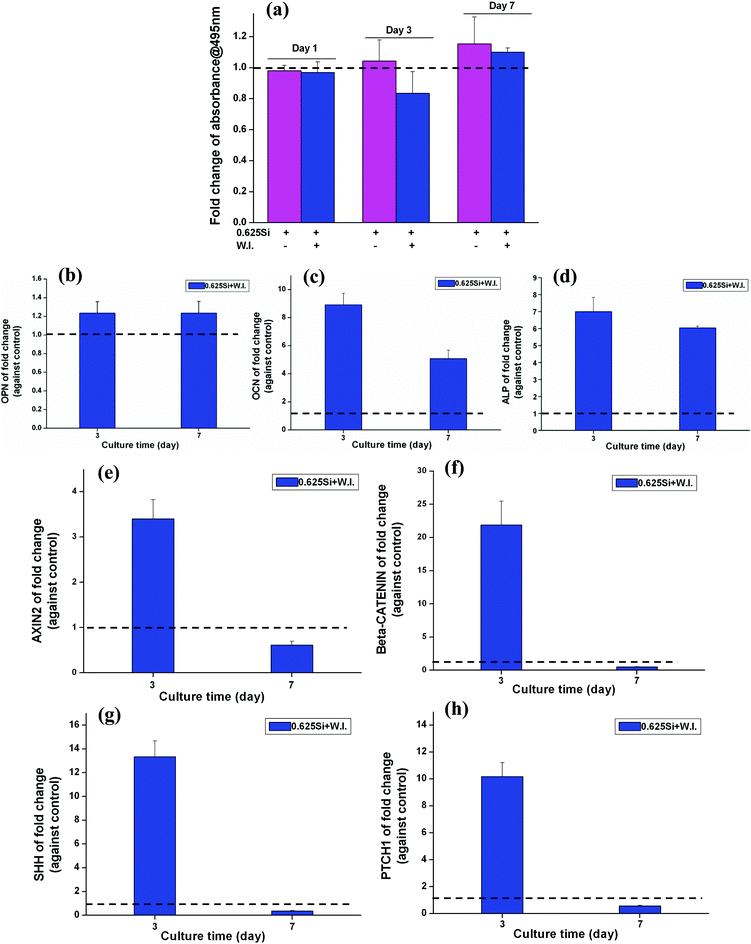 |
| Fig. 7 The effect of Si ions on cell proliferation (a), osteogenic genes (b–d) and WNT, SHH-related genes expression (e–h) of BMSCs in the presence of cardamonin (W.I.) at a concentration of 10 μM. At day 7, Si ions at a concentration of 0.625 mM stimulated the proliferation of BMSCs cultured with the W.I. at a concentration of 10 μM (a). At both day 3 and 7, Si ions at a concentration of 0.625 mM enhanced the gene expression of OPN, OCN and ALP (b–d), AXIN2, CTNNB (e, f), SHH and PTCH1 (g, h) with W.I. at a concentration of 10 μM (fold change > 1). The dotted line shows a fold change of 1 between the NaCl and Na2SiO3 groups. | |
3.6 The effect of Si on bone-related protein expression of hBMSCs by western blotting analysis
Western blotting analysis showed that ALP and OPN were elevated when hBMSCs were cultured with 0.625 mM Na2SiO3 compared to those cultured with 1.25 mM NaCl (Fig. 8a). The relative intensity analysis demonstrated that the expression of ALP and OPN for hBMSCs cultured with 0.625 mM Na2SiO3 was higher than that in the 1.25 mM NaCl group (Fig. 8b and c). OCN protein expression for hBMSCs cultured with 0.625 mM Na2SiO3 is comparable to that of 1.25 mM NaCl (Fig. 8d).
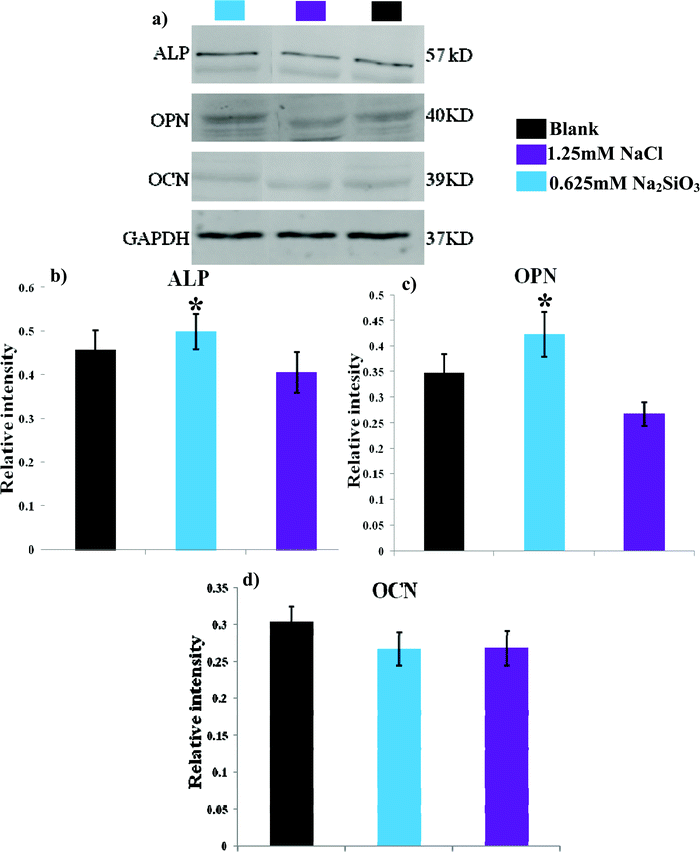 |
| Fig. 8 The western blotting analysis of bone-related protein of ALP, OPN and OCN expression of hBMSCs after 7 days cultured at the concentration of 0.625 mM Na2SiO3 and 1.25 mM NaCl. * indicates a significant difference (p < 0.05) between 0.625 mM Na2SiO3 and 1.25 mM NaCl group. | |
4. Discussion
In this study we have explored the effect of Si ions on the proliferation, differentiation and mineralization, as well as the gene expression of bone, WNT and SHH-related pathways, of BMSCs cultured in two groups with defined concentrations of either NaCl or Na2SiO3. Given that both salts contain Na+, it was therefore possible to account for the effect of Si ions alone by comparing the results from both groups. It was found that Si ions showed a mixed effect on BMSC osteogenic gene expression and that, at certain concentrations, they significantly enhanced the proliferation, mineralization nodule formation, bone-related gene expression, and WNT and SHH signalling pathways in BMSCs. These results demonstrate that Si ions play an important role in regulating cellular responses, including WNT and SHH signalling pathways, in BMSCs. Our study may therefore provide direct evidence to explain the stimulatory effect on osteogenesis of Si-containing biomaterials and may potentially provide a guide for the design of Si-containing biomaterials with desirable Si ion release in order to achieve osteogenesis.
There has been longstanding interest in the osteogenically stimulatory effects of Si-containing biomaterials on bone-derived cell types,4,34 but until now the mechanism of the biological role of Si ions on bone-forming cells has been unclear. Recent studies have sought to investigate the effect of Si ions on cell functions by two separate means. In one method, human osteoblastic cell lines were treated with different concentrations of orthosilicic acid (H4SiO4) and it was found that H4SiO4 could enhance osteogenic differentiation.31,32 The other method investigated the effect of silicate ions on the proliferation, adhesion and differentiation of osteoblast or osteoblast-like cells by culturing these in a range of concentrations of Na2SiO3.30,35 Both approaches have disadvantages for exploring the effect of Si ions themselves on cell proliferation and differentiation. For example, the effect on the cell response of the low pH caused by the release of H+ ions from H4SiO4 was not taken into account in that approach31 and, in the approach using Na2SiO3, the effect of Na ions has not been factored in.35 As a result, the actual cellular effect of Si ions on their own remains unclear from either of these two methods. In addition, the cell molecular effect of Si ions on the bone-related gene expression and cell signaling pathways is largely unknown. In the present study, we have investigated the effect of Si ions on cell proliferation, differentiation and the bone-related gene expression and signalling pathways of BMSCs by implementing two treatment groups which were both exposed to the same relative concentrations of Na ions, therefore accounting for the effect of Na ions. The fold change attributable to Si ions alone could thus be estimated. This approach gave a more accurate picture of the real impact of Si ions on cell responses than those methods used previously.
Previous studies have shown that cellular responses are influenced by several factors (e.g. ionic compositions, ionic concentrations and pH values).36–39 We have previously found that ionic products with graded concentrations from silicate bioceramics, such as akermanite (Ca2MgSi2O7),36 bredigite (Ca7MgSi4O16)40 and nagelschmidtite (Ca7P2Si2O16)41 play an important role in influencing the proliferation and differentiation. Generally, at a certain concentration of ionic products, the released silicate-based ionic products stimulate cell proliferation and differentiation. However, if the ionic concentrations are too high, this will result in a cytotoxic effect on cell growth. For this reason, in this study, we chose a wide ionic concentration range (from 0.00625 to 5 mM) to investigate the effect of Si ions on BMSC response. Our results showed that high concentrations of Si ions at 5 mM suppressed cell proliferation. One potential reason may be that high concentrations of Si ions themselves caused cytotoxicity. Another reason is that high concentrations of Si ions may influence pH and extracellular ionic environment and further inhibit cellular response. Our results demonstrate that Na2SiO3 affects cell proliferation, differentiation and mineralization nodule formation in hBMSCs. Treatment with Na2SiO3 enhanced osteogenic differentiation of BMSCs via up-regulation of the bone-related genes OPN, OCN and ALP, as well as the expression of WNT and SHH signaling-related genes. Besides the effect of Si ions, we were also able to demonstrate that Na ions play an important role in regulating cell proliferation and differentiation. By allowing for the effect of Na ions in our study, the effect of Si ions themselves could be effectively revealed in terms of the fold change after comparing the two groups. Interestingly, comparing Si ions at different concentrations, Si ions alone at certain concentrations (particularly 0.625 mM) significantly stimulated the proliferation, differentiation, Ca-mineralization, bone-related gene/protein expression and signaling pathways of the BMSCs. These results provide an insight into the importance of Si ions for differentiation and bone-related gene expression, as well as WNT and SHH signaling pathways, in BMSCs. This knowledge will make it easier to understand the biological role of Si, both in vitro and in vivo. Many earlier studies have shown that Si-containing biomaterials improve in vitro and in vivo osteogenesis,17–23,25 but the underlying mechanisms and the role of Si in biomaterials have remained unclear. The present study confirms the osteogenic effects of Si and offers some evidence to explain the molecular mechanisms responsible for the bioactivity of Si-containing biomaterials. It has been reported that Si ions at 4 mM can activate the MAPK-ERK pathway in osteoblast-like cells35 and Ca and Si ions in Ca2SiO4 coatings enhanced cellular differentiation and collagen production via the TGF pathway by TGF-1 binding to its cognate receptors.42 To date there are no reports as to whether WNT and SHH signalling pathways are involved in the effect of Si ions on BMSC differentiation. The WNT canonical signalling pathway is known to play an important role in regulating cellular events, such as cell proliferation, differentiation and morphogenesis.26,43 AXIN2, a member of the WNT pathway, is recognized as a reporter gene of canonical WNT signalling pathway activity, since it is a direct target gene of the canonical WNT signalling pathway.44,45 β-Catenin is also a critical member of THE WNT/β-catenin signalling pathway involved in cell proliferation and differentiation.46,47 The Hedgehog-Gli signalling pathway also plays an important role in cell proliferation and differentiation.48–50 SHH is one of the ligands of this pathway and its cognate receptor, Patched-1 (Ptch1), is one of the target genes of the SHH-Gli signalling pathway.51 In this study, Si ions at 0.625 mM significantly stimulated these signalling pathway of BMSCs, as shown by upregulation of AXIN2, β-catenin, SHH and PTCH1 gene expression. Furthermore, at a concentration of 0.625 mM, Si ions also stimulated bone-related gene expression (OCN, OPN and ALP) of BMSCs. Cardamonin has been identified as an inhibitor of the WNT signalling pathway by inactivating β-catenin, thereby blocking WNT/β-catenin signalling.52 Further experiments confirmed that the corresponding protein levels of ALP and OPN were increased by 0.625 mM Na2SiO3 compared to those with 1.25 mM NaCl. Our results demonstrated that Si ions at a concentration of 0.625 mM enhanced OPN, OCN, ALP and WNT and SHH-related gene expression, even when W.I. was added in the culture media, indicating that Si ions can rescue to a certain degree the effect induced by W.I. However, this counteracting effect of Si ions and cardamonin on BMSCs needs further investigation. Our results showed that soluble SiO32− ions may stimulate cell proliferation and osteogenic differentiation of BMSCs through activation of WNT and SHH-related gene expression. The possible mechanisms have been summarized in Fig. 9. In brief, after travelling across the plasma membrane via ion channels, SiO32− ions may play a role in stabilization of β-catenin and in leading its translocation into the nucleus. SiO32− ions may also be associated with the LEF/TCF family to induce expression of the target gene AXIN2. On the other hand, SiO32− ions could also induce SHH ligands binding with the receptors and subsequently promote the SHH target gene (PTCHED1) expression. Activation of WNT and SHH-related genes leads to the upregulation of cell proliferation and osteogenic differentiation of BMSCs.
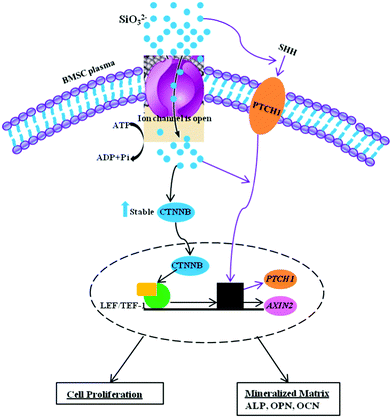 |
| Fig. 9 Schematic model depicting the effect of SiO32− on BMSCs differentiation. | |
5. Conclusions
In the present study, the effect of Si ions on the proliferation, differentiation, mineralization and WNT and SHH-related gene expression of BMSCs has been systematically investigated by comparing the biological effects of NaCl and Na2SiO3. Si ions at a given concentration significantly enhanced the proliferation, mineralization nodule formation, bone-related gene expression and WNT and SHH signalling pathway of BMSCs. These results suggest that Si ions alone play an important role in regulating the cellular response of BMSCs and that the WNT and SHH signalling pathways are both involved in these cellular responses. Therefore, our results provide evidence for possible mechanisms whereby Si ions released from Si-containing biomaterials can exert their bioactivity.
Acknowledgements
Funding for this study was provided by the Recruitment Program of Global Young Talent, China (Dr Wu), Natural Science Foundation of China (Grant 81201202), Shanghai Pujiang Talent Program (12PJ1409500) and ARC Discovery DP120103697.
References
- E. M. Carlisle, Silicon: a possible factor in bone calcification, Science, 1970, 167, 279–280 CAS.
- K. Schwarz and D. B. Milne, Growth-promoting effects of silicon in rats, Nature, 1972, 239, 333–334 CrossRef CAS.
- M. Vallet-Regi and D. Arcos, Silicon substituted hydroxyapatites. A method to upgrade calcium phosphate based implants, J. Mater. Chem., 2005, 15, 1509–1516 RSC.
- I. R. Gibson, S. M. Best and W. Bonfield, Chemical characterization of silicon-substituted hydroxyapatite, J. Biomed. Mater. Res., 1999, 44, 422–428 CrossRef CAS.
- S. Langstaff, M. Sayer, T. J. Smith, S. M. Pugh, S. A. Hesp and W. T. Thompson, Resorbable bioceramics based on stabilized calcium phosphates. Part I: rational design, sample preparation and material characterization, Biomaterials, 1999, 20, 1727–1741 CrossRef CAS.
- F. Balas, J. Perez-Pariente and M. Vallet-Regi,
In vitro bioactivity of silicon-substituted hydroxyapatites, J. Biomed. Mater. Res., 2003, 66, 364–375 CrossRef CAS.
- S. Gomes, G. Renaudin, A. Mesbah, E. Jallot, C. Bonhomme and F. Babonneau,
et al. Thorough analysis of silicon substitution in biphasic calcium phosphate bioceramics: a multi-technique study, Acta Biomater., 2010, 6, 3264–3274 CrossRef CAS.
- J. W. Reid, L. Tuck, M. Sayer, K. Fargo and J. A. Hendry, Synthesis and characterization of single-phase silicon-substituted alpha-tricalcium phosphate, Biomaterials, 2006, 27, 2916–2925 CrossRef CAS.
- M. Mastrogiacomo, A. Muraglia, V. Komlev, F. Peyrin, F. Rustichelli and A. Crovace,
et al. Tissue engineering of bone: search for a better scaffold, Orthodont. Craniofacial Res., 2005, 8, 277–284 CrossRef CAS.
- K. A. Hing, P. A. Revell, N. Smith and T. Buckland, Effect of silicon level on rate, quality and progression of bone healing within silicate-substituted porous hydroxyapatite scaffolds, Biomaterials, 2006, 27, 5014–5026 CrossRef CAS.
- C. M. Botelho, R. A. Brooks, S. M. Best, M. A. Lopes, J. D. Santos and N. Rushton,
et al. Human osteoblast response to silicon-substituted hydroxyapatite, J. Biomed. Mater. Res., Part A, 2006, 79, 723–730 CrossRef CAS.
- C. Wu, W. Fan, J. Chang and Y. Xiao, Mussel-inspired porous SiO2 scaffolds with improved mineralization and cytocompatibility for drug delivery and bone tissue engineering, J. Mater. Chem., 2011, 21, 18300–18307 RSC.
- Y. Zhu, X. Li, J. Yang, S. Wang, H. Gao and N. Hanagata, Composition–structure–property relationship of the CaO–MxOy–SiO2–P2O5 (M = Zr, Mg, Sr) mesoporous bioactive glass (MBG) scaffolds, J. Mater. Chem., 2011, 21, 9208–9218 RSC.
- X. Li, X. Wang, D. He and J. Shi, Synthesis and characterization of mesoporous CaO–MO–SiO2–P2O5 (M = Mg, Zn, Cu) bioactive glasses/composites, J. Mater. Chem., 2008, 18, 4103–4109 RSC.
- Y. Ramaswamy, C. Wu, A. Van Hummel, V. Combes, G. Grau and H. Zreiqat, The responses of osteoblasts, osteoclasts and endothelial cells to zirconium modified calcium-silicate-based ceramic, Biomaterials, 2008, 29, 4392–4402 CrossRef CAS.
- C. Wu, Y. Luo, G. Cuniberti, Y. Xiao and M. Gelinsky, Three-dimensional printing of hierarchical and tough mesoporous bioactive glass scaffolds with a controllable pore architecture, excellent mechanical strength and mineralization ability, Acta Biomater., 2011, 7, 2644–2650 CrossRef CAS.
- P. Valerio, M. M. Pereira, A. M. Goes and M. F. Leite, The effect of ionic products from bioactive glass dissolution on osteoblast proliferation and collagen production, Biomaterials, 2004, 25, 2941–2948 CrossRef CAS.
- I. D. Xynos, A. J. Edgar, L. D. Buttery, L. L. Hench and J. M. Polak, Ionic products of bioactive glass dissolution increase proliferation of human osteoblasts and induce insulin-like growth factor II mRNA expression and protein synthesis, Biochem. Biophys. Res. Commun., 2000, 276, 461–465 CrossRef CAS.
- H. Sun, C. Wu, K. Dai, J. Chang and T. Tang, Proliferation and osteoblastic differentiation of human bone marrow-derived stromal cells on akermanite-bioactive ceramics, Biomaterials, 2006, 27, 5651–5657 CrossRef CAS.
- W. Peng, W. Liu, W. Zhai, L. Jiang, L. Li and J. Chang,
et al. Effect of tricalcium silicate on the proliferation and odontogenic differentiation of human dental pulp cells, J. Endod., 2011, 37, 1240–1246 CrossRef.
- L. Xia, Z. Zhang, L. Chen, W. Zhang, D. Zeng and X. Zhang,
et al. Proliferation and osteogenic differentiation of human periodontal ligament cells on akermanite and beta-TCP bioceramics, Eur. Cell. Mater., 2011, 22, 68–82 CAS.
- H. Gu, F. Guo, X. Zhou, L. Gong, Y. Zhang and W. Zhai,
et al. The stimulation of osteogenic differentiation of human adipose-derived stem cells by ionic products from akermanite dissolution via activation of the ERK pathway, Biomaterials, 2011, 32, 7023–7033 CrossRef CAS.
- C. Wu, J. Chang, S. Ni and J. Wang,
In vitro bioactivity of akermanite ceramics, J. Biomed. Mater. Res., A, 2006, 76, 73–80 CrossRef.
- C. Wu, Y. Zhang, W. Fan, X. Ke, X. Hu and Y. Zhou,
et al. CaSiO3 microstructure modulating the in vitro and in vivo bioactivity of poly(lactide-co-glycolide) microspheres, J. Biomed. Mater. Res., A, 2011, 98, 122–131 CrossRef.
- S. Xu, K. Lin, Z. Wang, J. Chang, L. Wang and J. Lu,
et al. Reconstruction of calvarial defect of rabbits using porous calcium silicate bioactive ceramics, Biomaterials, 2008, 29, 2588–2596 CrossRef CAS.
- Y. Huang, X. Jin, X. Zhang, H. Sun, J. Tu and T. Tang,
et al. In vitro and in vivo evaluation of akermanite bioceramics for bone regeneration, Biomaterials, 2009, 30, 5041–5048 CrossRef CAS.
- M. Bohner, Silicon-substituted calcium phosphates – a critical view, Biomaterials, 2009, 30, 6403–6406 CrossRef CAS.
- L. L. Hench and J. M. Polak, Third-generation biomedical materials, Science, 2002, 295, 1014–1017 CrossRef CAS.
- L. L. Hench and I. Thompson, Twenty-first century challenges for biomaterials, J. R. Soc. Interface, 2010, 7(Suppl 4), S379–S391 CrossRef CAS.
- S. Zou, D. Ireland, R. A. Brooks, N. Rushton and S. Best, The effects of silicate ions on human osteoblast adhesion, proliferation, and differentiation, J. Biomed. Mater. Res., B: Appl. Biomater., 2009, 90, 123–130 CAS.
- D. M. Reffitt, N. Ogston, R. Jugdaohsingh, H. F. Cheung, B. A. Evans and R. P. Thompson,
et al. Orthosilicic acid stimulates collagen type 1 synthesis and osteoblastic differentiation in human osteoblast-like cells in vitro, Bone, 2003, 32, 127–135 CrossRef CAS.
- W. C. Duivenvoorden, A. Middleton and S. D. Kinrade, Divergent effects of orthosilicic acid and dimethylsilanediol on cell survival and adhesion in human osteoblast-like cells, J. Trace Elem. Med. Biol., 2008, 22, 215–223 CAS.
- J. Li, S. Mareddy, D. M. Tan, R. Crawford, X. Long and X. Miao,
et al. A minimal common osteochondrocytic differentiation medium for the osteogenic and chondrogenic differentiation of bone marrow stromal cells in the construction of osteochondral graft, Tissue Eng. A, 2009, 15, 2481–2490 CrossRef CAS.
- I. R. Gibson, J. Huang, S. M. Best and W. Bonfield, Enhanced in vitro cell activity and surface apatite layer formation on novel silicon-substituted hydroxyapatite, Bioceramics, 1999, 12, 191–194 CAS.
- M. Y. Shie, S. J. Ding and H. C. Chang, The role of silicon in osteoblast-like cell proliferation and apoptosis, Acta Biomater., 2011, 7, 2604–2614 CrossRef CAS.
- C. Wu, J. Chang, S. Ni and J. Wang,
In vitro bioactivity of akermanite ceramics, J. Biomed. Mater. Res., Part A, 2006, 76(1), 73–80 CrossRef.
- C. Wu, Y. Ramaswamy, A. Soeparto and H. Zreiqat, Incorporation of titanium into calcium silicate improved their chemical stability and biological properties, J. Biomed. Mater. Res., Part A, 2008, 86(2), 402–410 CrossRef.
- I. A. Silver, J. Deas and M. Erecińska, Interactions of bioactive glasses with osteoblasts in vitro: effects of 45S5 bioglass, and 58S and 77S bioactive glasses on metabolism, intracellular ion concentrations and cell viability, Biomaterials, 2001, 22, 175–185 CrossRef CAS.
- D. A. Bushinsky, Metabolic alkalosis decreases bone calcium efflux by suppressing osteoclasts and stimulating osteoblasts, Am. J. Physiol., 1996, 271, F216–F222 CAS.
- C. Wu, J. Chang, J. Y. Wang, S. Y. Ni and W. Y. Zhai, Preparation and characteristics of a calcium magnesium silicate (bredigite) bioactive ceramic, Biomaterials, 2005, 26, 2925–2931 CrossRef CAS.
- Y. Zhou, C. Wu and Y. Xiao, The stimulation of proliferation and differentiation of periodontal ligament cells by the ionic products from Ca7Si2P2O16 bioceramics, Acta Biomater., 2012, 8, 2307–2316 CrossRef CAS.
- J. Li, L. Wei, J. Sun and G. Guan, Effect of ionic products of dicalcium silicate coating on osteoblast differentiation and collagen production via TGF-beta1 pathway, J. Biomater. Appl., 2011 DOI:10.1177/0885328211416393.
- B. Zhang and J. X. Ma, Wnt pathway antagonists and angiogenesis, Protein Cell, 2010, 1, 898–906 CrossRef CAS.
- E. H. Jho, T. Zhang, C. Domon, C. K. Joo, J. N. Freund and F. Costantini, Wnt/beta-Catenin/Tcf signalling induces the transcription of Axin2, a negative regulator of the signalling pathway, Mol. Cell. Biol., 2002, 22, 1172–1183 CrossRef CAS.
- H. M. Yu, B. Jerchow, T. J. Sheu, B. Liu, F. Costantini and J. E. Puzas,
et al. The role of Axin2 in calvarial morphogenesis and craniosynostosis, Development, 2005, 132, 1995–2005 CrossRef CAS.
- J. Huelsken and W. Birchmeier, New aspects of Wnt signaling pathways in higher vertebrates, Curr. Opin. Genet. Dev., 2001, 11, 547–553 CrossRef CAS.
- N. Barker, The canonical Wnt/beta-catenin signalling pathway, Methods Mol. Biol., 2008, 468, 5–15 CAS.
- P. N. De Aza, Z. B. Luklinska, A. Martinez, M. R. Anseau, F. Guitian and S. De Aza, Morphological and structural study of pseudowollastonite implants in bone, J. Microsc., 2000, 197, 60–67 CrossRef CAS.
- Y. Takahashi, S. Imazato, A. V. Kaneshiro, S. Ebisu, J. E. Frencken and F. R. Tay, Antibacterial effects and physical properties of glass-ionomer cements containing chlorhexidine for the ART approach, Dent. Mater., 2006, 22, 647–652 CrossRef CAS.
- S. V. Prykhozhij, In the absence of Sonic hedgehog, p53 induces apoptosis and inhibits retinal cell proliferation, cell-cycle exit and differentiation in zebrafish, PLoS One, 2010, 5, e13549 Search PubMed.
- M. Varjosalo and J. Taipale, Hedgehog: functions and mechanisms, Genes Dev., 2008, 22, 2454–2472 CrossRef CAS.
- M. Cho, M. Ryu, Y. Jeong, Y. H. Chung, D. E. Kim and H. S. Cho,
et al. Cardamonin suppresses melanogenesis by inhibition of Wnt/beta-catenin signaling, Biochem. Biophys. Res. Commun., 2009, 390, 500–505 CrossRef CAS.
Footnote |
† Electronic supplementary information (ESI) available. See DOI: 10.1039/c2bm00108j |
|
This journal is © The Royal Society of Chemistry 2013 |
Click here to see how this site uses Cookies. View our privacy policy here.