DOI:
10.1039/D4RA02014F
(Review Article)
RSC Adv., 2024,
14, 16300-16317
Recent developments in synthesis of attapulgite composite materials for refractory organic wastewater treatment: a review
Received
16th March 2024
, Accepted 14th May 2024
First published on 20th May 2024
Abstract
Attapulgite clay, due to its unique crystalline hydrated magnesium–aluminium silicate composition and layer-chain structure, possesses exceptional adsorption and catalytic properties, which enable it or its composites to be utilized as adsorbents and catalysts for wastewater treatment. But the drawbacks of attapulgite are also very obvious, such as relatively low specific surface area (compared to traditional adsorbents such as activated carbon and activated alumina), easy aggregation, and difficulty in dispersion. In order to fully utilize and improve the performance of attapulgite, researchers have conducted extensive research on its modification, but few specialized works have comprehensively evaluated the synthesis, applications and challenges for attapulgite-based composite materials in refractory organic wastewater treatments. This paper provides a comprehensive review of controllable preparation strategies, characterization methods and mechanisms of attapulgite-based composite materials, as well as the research progress of these materials in refractory organic wastewater treatment. Based on this review, constructive recommendations, such as deep mechanism analysis from molecular level multi-functional attapulgite-based material developments, and using biodegradable materials in attapulgite-based composites, were proposed.
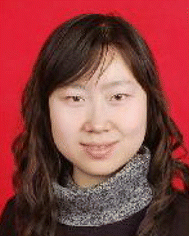 Ting Zhang | Ting Zhang, graduated from Lanzhou University and got her PhD degree in Environmental Science. Her main research field is environmental catalysis based on natural clay materials. She took charge of over 10 research projects successively, including National Natural Science Foundation of China and Major Science and Technology Special Project of Gansu Province. She has received 6 awards of science and technology at the provincial level or above, and published 5 books and more than 40 papers. |
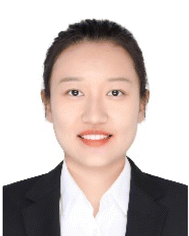 Xiaoyi Huang | Xiaoyi Huang, graduated from Hebei University of Science and Technology in June 2021 with a double bachelor's degrees in environmental science and business english. Now studying at Lanzhou University of Technology for a master's degree, under Professor Zhang Ting, majoring in environmental engineering. At present, her main research direction is environmental catalysis. |
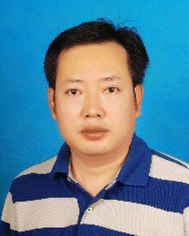 Yi Wang | Yi Wang, received his PhD degree in advanced materials and their preparation techniques from Lanzhou University of Technology. He is the responsible professor of National First-Class Undergraduate Major Construction Points and the leader of Applied Chemistry Innovation & Entrepreneurship Teaching Team at Gansu Province, China. His research interests are thermal energy storage materials and utilization technology of solid waste resources. He took charge of more than ten research projects successively. He has received 6 awards of science and technology at the provincial level or above, and published more than 60 papers. |
1 Introduction
Organic pollution, particularly refractory organic pollution, poses a significant global environmental challenge due to its profound toxicity to living organisms and humans. Refractory organic pollutants (ROPs) comprise a diverse range of highly toxic compounds, including halogenated organics, surfactants, nitro compounds, heterocyclic compounds, phenolic compounds, and polycyclic aromatic hydrocarbons. These compounds are notoriously resistant to microbial degradation, often undergoing slow and incomplete decomposition. Many ROPs exert harmful effects on human health and the environment, with some even possessing carcinogenic properties.
The primary sources of wastewater contaminated with refractory organics stem from various chemical industries such as coking, pharmaceuticals (encompassing traditional Chinese medicine), petrochemicals/oil, textiles/printing and dyeing, and paint manufacturing. Table 1 provides a comprehensive overview of common refractory organics, detailing their sources and associated hazards. This type of wastewater typically exhibits a complex mixture of refractory organics and salts, characterized by high concentrations and poor biodegradability.
Table 1 Types and hazards of refractory organic substances
Refractory organics |
Sources |
Harmful effects |
References |
Polycyclic aromatic hydrocarbons compounds (PAHs), such as naphthalene, anthracene, phenanthrene, pyrene, etc. |
Wood and coal burning, petrol and diesel combustion, industrial discharge, vehicular emission, etc. |
Carcinogenic, teratogenic, mutagenic and toxic |
1–5 |
Heterocyclic compounds, such as furan, pyrrole, thiophene, etc. |
Domestic sewage treatment plants and some chemical engineering industries such as textile, dyestuff, pharmaceutical and chemical productions |
Stable in nature, easy to bioaccumulate, mutagenic and carcinogenic |
6–8 |
Organic cyanides, such as acetonitrile, propionitrile, acrylonitrile, etc. |
Coking, electroplating and pharmaceutical industries |
Highly toxic. Short-term exposure to cyanide could cause shortness of breath and neurological diseases, and prolonged exposure would lead to nerve damage and even death |
9–11 |
Synthetic detergents, such as sodium alkylbenzene sulfonate, sodium fatty alcohol sulfate, etc. |
Laundry wastewater originated from households, industries and hospitals |
Foaming thus affects biological treatment effect and has solubilization effect on polycyclic aromatic hydrocarbons |
12 and 13 |
Polychlorinated biphenyls (PCBs), from PCB3 to PCB10 |
Preparation of capacitors and transformers, oil for chemical engineering |
Entering the human body through the food chain, causing acute poisoning and carcinogenesis to the human body |
14 and 15 |
Plasticizers, such as phthalates, aliphatic dicarboxylic esters, fatty acid esters, etc. |
Plastic products |
It is stable and has inhibitory effect on human central nervous system |
16 |
Synthetic pesticides, such as organochlorine pesticides, organophosphorus pesticides, etc. |
Agricultural fields and non-agricultural settings such as households, industries, sport fields and other urban green areas |
Toxic and carcinogenic to humans |
17 and 18 |
Synthetic dyes, such as methylene blue, orange II, methyl violet, etc. |
Textile industry |
High chroma, toxic and carcinogenic |
19 and 20 |
The pervasive threat posed by refractory organic compounds to human health and ecological systems underscores the critical importance of addressing their pollution control. As such, the exploration of effective strategies for alleviating refractory organic pollution has emerged as a vital research focus within the environmental protection domain.
In recent decades, significant research efforts have been devoted to developing treatments for refractory organic pollutants.21,22 A range of techniques has been explored for their removal, including adsorption,23,24 biological treatment processes,25–27 membrane separation,28,29 catalysis,30,31 and advanced oxidation processes.32,33
Notably, catalytic oxidation processes have made considerable progress in treating refractory organics. These processes encompass photocatalytic oxidation,23 catalytic wet air oxidation,31 electro-catalysis,21,34,35 Fenton catalysis,36–40 semiconductor catalysis, and heterogeneous catalysis.35,41–43 Among these, heterogeneous catalysis has emerged as a particularly promising approach due to its ability to generate hydroxyl radicals (˙OH) and holes with strong oxidizing power. These radicals can effectively decompose toxic, harmful, and non-biodegradable macromolecular organic compounds into non-toxic, biodegradable small molecular organic substances. Heterogeneous catalysis offers advantages such as a wide pH range, no secondary pollution, and good reusability.44–46
Heterogeneous catalysts can be broadly categorized into bulk catalysts and supported catalysts. Catalytic reactions primarily occur on the surface of these catalysts. Supported catalysts are particularly advantageous due to their high surface area and abundance of active sites, which enhance their catalytic efficiency compared to bulk catalysts.47 Supported catalysts typically utilize porous materials as supports, including carbon,48,49 graphene and its derivatives,50,51 oxides such as ZnO, SiO2, Bi2O3,52–54 and clays.55,56 The active components, which can be metals or metal oxides, are deposited onto the surface of these supports to form the catalysts.
2 Attapulgite
Clays, including montmorillonite, kaolin, bentonite, and attapulgite, have found application as natural, economical supports for heterogeneous catalysis in wastewater treatment.57–60 Among these, attapulgite clay distinguishes itself with its distinctive crystalline hydrated magnesium–aluminium silicate composition and its unique layer-chain structural arrangement. It exhibits exceptional dispersibility, high-temperature resistance, salt resistance, and strong adsorption capabilities, alongside good plasticity and cohesion.61,62 These attributes make it a versatile material for use as an adsorbent or catalyst support in the removal of hazardous substances.57–59
Structurally, attapulgite belongs to the monoclinic system and features an acicular, slender hollow tubular structure reminiscent of amphibole asbestos. Its ideal molecular formula is Mg5Si8O20(OH)2(OH2)4·4H2O, and a diagram of its crystal structure is presented in Fig. 1. This structure consists of eight Si–O tetrahedrons arranged in 2
:
1 layers that extend along the C-axis, and cations like Mg2+ and Al3+ are filled in coordination octahedra formed by –O– and –OH, that is, two layers of silicon-oxygen tetrahedrons sandwich a layer of magnesium (or aluminium)- oxygen octahedron, creating pores parallel to the C-axis between the [Si4O10] zones. These pores, with cross-sectional radii ranging from 0.37 to 0.64 nm, are occupied by water molecules. Some of this water is zeolite water aligned with the fiber axis, while the rest is crystalline water coordinated with magnesium ions.63,64
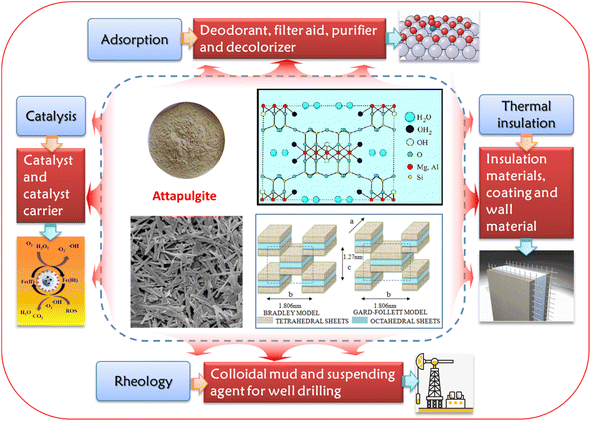 |
| Fig. 1 The schematic diagrams of attapulgite crystal structure and its properties and usages. | |
Attapulgite's distinctive structure, characterized by a high specific surface area and an unbalanced charge surface due to its crystalline morphology and abundant internal channels, endows it with remarkable properties such as excellent adsorption, catalytic activity, heat resistance, and rheological behavior. These qualities have led to its widespread application across various domains,65–70 as illustrated in Fig. 1.
In addition, the crystalline water in the structure of attapulgite is located on the surface of its channel, facilitating the formation of hydrogen bonds with the adsorbate entering the channel.61,62 Consequently, the surface of attapulgite is rich in adsorption active sites, exhibiting a preference for the adsorption of polar molecules like water. Additionally, attapulgite clay boasts impressive thermal stability and mechanical properties, rendering it a versatile material commonly employed as a purifier, decolorizer, filter aid, and deodorant.65–68,70
Although the unique structure and properties of attapulgite give it potential application advantages, its drawbacks are also obvious: the specific surface area of attapulgite is still relatively low compared to other commonly used adsorbents (such as activated carbon, activated alumina, etc.), it is easy to agglomerate, not easy to disperse, has poor compatibility with other substances, and the active substances are not easily uniformly loaded during the preparation of catalysts. To address the aforementioned issues, researchers have undertaken various modifications to attapulgite or have compounded it with other substances. These modifications aim to boost its specific surface area, enhance conductivity, and mitigate its aggregation tendencies. Additionally, the modifications enable uniform loading of active substances, increase the number of effective catalytic active sites, and ultimately improve the catalytic performance of the materials. Until now, there is a lack of comprehensive and multi-dimensional literature summarizing the latest progress in the application of modified attapulgite for the removal of refractory organics from water, particularly addressing the technical challenges and future research avenues. A systematic review of the latest achievements in attapulgite-based material development and mechanism exploration is crucial for guiding future research efforts and promoting the large-scale application of these materials for the removal of refractory organics from water. This review illuminates diverse types of attapulgite-based materials, detailing their preparation methodologies, characterizations, applications, and mechanisms, and it also highlights the associated challenges and recommendations of improvement in this field of research.
3 Modifications of attapulgite and their utilizations in treating refractory organics
To enhance the specific surface area, porosity, adsorption capacity, and catalytic performance of attapulgite, it is commonly modified or combined with various functional materials. The modification of attapulgite can be achieved through numerous techniques, which can be broadly categorized based on the type of modifier used: heat modification, acid/alkaline/salt modification, organic modification, carbon-based materials modification, and metals/metal oxides modification.
Heat and acid/alkaline/salt modification are straightforward methods, primarily aimed at increasing the specific surface area of attapulgite by removing water, melting impurities and facilitating ion exchange. Liu et al.71 have conducted a relatively in-depth discussion on these modifications, and we will not elaborate those further. Instead, this paper will primarily explore the organic modification, carbon-based materials modification, and metals/metal oxides modification of attapulgite.
3.1 Organic modification of attapulgite
Organic modification involves the introduction of organic functional groups onto the surface of attapulgite, which can improve its compatibility with organic systems and enhance its adsorption properties. Organic modifiers commonly used for this purpose include surfactants, silane coupling agents, and polymers (Fig. 2). The are three typical ATP organic modification methods:
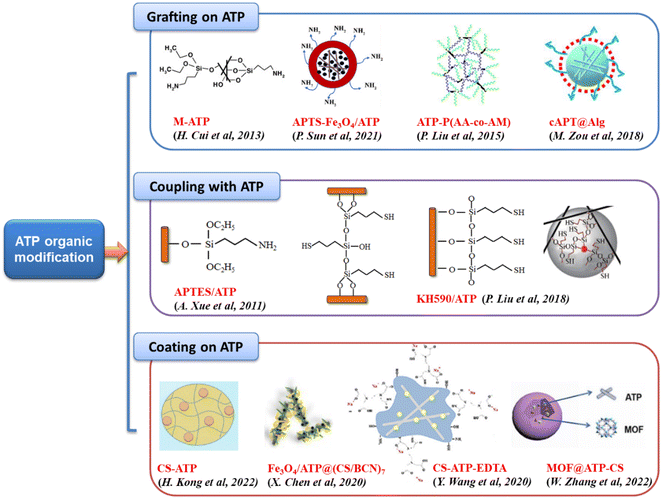 |
| Fig. 2 ATP organic modification methods.75,86–94 Copyright 2024, Elsevier. | |
(1) Grafting: this method often uses surfactants as modifier to change the ATP's surface functional groups, improving its wettability, dispersibility, and compatibility with organic matrices. Grafting can be achieved through chemical reactions that create covalent bonds between the organic molecules and the ATP.
(2) Silane-coupling: silane coupling agents are used to modify the surface of ATP and change its hydrophobicity. These agents react with the hydroxyl groups on the ATP surface, forming siloxane bonds that introduce organic functional groups. This makes the ATP surface more hydrophobic, which can be beneficial for certain applications, such as the adsorption of organic compounds from aqueous solutions.
(3) Coating: coating involves the deposition of a thin layer of organic material onto the surface of ATP. This layer can be composed of polymers or other organic compounds and serves to change the surface structure of ATP. Coating can improve the mechanical properties, stability, and compatibility of ATP with organic media. It can also provide additional functional groups that can enhance the adsorption or catalytic properties of ATP.
3.1.1 Grafting on attapulgite. Grafting is considered as a promising approach for ATP modification, because grafting process can provide functional groups which show helpful properties for up-taking cationic, anionic and neutral pollutants from aqueous media,72 which can help attapulgite to have a better adsorption ability. Typically, functional groups grafting on attapulgite was prepared by graft copolymerization reactions and attapulgite was often modified with surfactants. Take NH2-grafted attapulgite as an example, the common modifiers are amino-terminated surfactants, such as polyacrylamide (PAM),73 octadecyl trimethyl ammonium chloride (OTAC),70,73 octadecyl trimethylammonium bromide (OTAB) and dioctadecyl dimethylammonium bromide (DDAB).74 NH2-grafted attapulgites had higher adsorption capacity than before modification, and they were used to adsorb dyes,75,76 phenol,65,77 tannin68 and obtain high removal percentages. Attapulgite was also functionalized by chloroacetic acid (CA) with –COOH functional groups, improving its adsorption properties for MB and got 99.8% removal ratio after modified.78
3.1.2 Modification of attapulgite with coupling agents. Attapulgite is rich in Si–OH polar groups and can be modified by coupling agents to change its surface property from hydrophilic to hydrophobic. Silane coupling agents and titanate coupling agents are usually used to modified ATP, and silane coupling agents are the earliest developed and most widely used type of coupling agents.79 Silane coupling agents can improve the surface properties of attapulgite at a very small dosage, take 3-aminopropyltriethoxysilane (APTES)75 as an example, its modification mechanisms are as follow: APTES first hydrolyzed then combined with ATP by hydrogen bond, the hydroxyl on ATP surface was replaced by silyl group and its surface changed from hydrophilic to lipophilic, which makes ATP adsorb some oil pollutants.More researches on the modification of attapulgite by coupling agents are focused on the adsorption of heavy metals after modification, which will not be mentioned here.
3.1.3 Modification of attapulgite with polymers. Polymer compounds, such as chitosan, polyaniline and polyurethane, can be used to prepare various of adsorbents by coating on the surface of ATP with non-covalent bond. Chitosan itself can be used as adsorbent for dyes, organic pesticides, fats, heavy metals and other toxic substances in wastewater due to its active sites –NH2 and –OH. Based on this, chitosan can be used to wrap ATP and promote adsorption capacity. Chitosan-coated attapulgite (CCATP) was prepared for removing the organic pollutant tannic acid (TA) from aqueous solution with maximum sorption capacity of 68 mg g−1.80 Chitosan functionalized attapulgite clay nanoparticle adsorbent (ATP@CCS) was fabricated by hydrothermal carbonization of chitosan at a mild temperature and applied for methylene blue (MB) removal from wastewater, and the calculated maximum adsorption capacity could be up to 215.73 mg g−1 at 318.15 K.81 Sun et al.82 fabricated a composite CPA composed of uniform purified attapulgite (PA) nanorods modified by chitosan under acetic acid conditions. Benefiting from the mesoporous structure of the PA nanorods and the carboxyl groups of chitosan, the prepared hybrid CPA exhibited a quick response (2 min for 80% removal ratio) and excellent adsorption capacity (the maximum adsorption capacity could reach 112.07 mg g−1) towards HA.82After coating attapulgite with polyaniline or polyurethane, nitrogen containing groups are introduced to the surface of attapulgite, which makes it have good adsorption effect on heavy metals and organic pollutants. Wang et al.83 used polyaniline coated attapulgite to remove humic acid in water, and the adsorption effect of coated attapulgite was significantly higher than that of unmodified attapulgite, the maximum adsorption capacity can reach 61.35 mg g−1. Dong et al.84 prepared a series of polyurethane–attapulgite porous (HATT/PU) materials for the removal of MG dye from aqueous solutions, and at the optimum conditions, the porous material had the highest adsorption ratio of 99.51%.
3.1.4 Combination attapulgite with COFs. Jia et al.85 reported a facile synthetic method of attapulgite@covalent organic frameworks (ATP/COFs). Covalent organic frameworks were synthesized from 1,3,5-triformylphloroglucinol and p-phenylenediamine, which were grafted onto attapulgite at room temperature, and the composite was synthesized. The extracting ratios of pyrethroids by ATP/COFs ranged from 71.2% to 88.7%. The removal ratios of methyl violet and tetracycline were still remained at 77.6% and 60.2% of the initial adsorption capacity after ten adsorption–regeneration cycles via a facile thermal regeneration strategy.
3.2 Modification attapulgite by carbon-based materials
Carbon-based materials modification, typically involves the incorporation of carbonaceous materials like activated carbon or carbon nanotubes, which can significantly improve the porosity and adsorption capacity of attapulgite.
3.2.1 Combination attapulgite with carbon. Carbon is often used as an additive for attapulgite to enhance its specific surface area (Fig. 3 left). Tang et al.95 developed a cost-effective attapulgite/carbon (APT/C) composite for wastewater treatment using waste hot-pot oil as a carbon precursor through a facile one-step calcination process. The optimal adsorption capacities reached 215.83 mg g−1, and 256.48 mg g−1 for methyl violet and tetracycline, respectively. Furthermore, they96 fabricated magnetic attapulgite/carbon supported NiFe layered double hydroxides (APT/C@NiFe-LDHs) based on the spent bleaching earth via a facile and green hydrothermal approach. The composites exhibited excellent adsorption capacities for methylene blue and chlortetracycline hydrochloride of 271.28 and 308.21 mg g−1, respectively. Li et al.97 used a novel low-cost clay–biochar (APB) composite with potato stem and natural attapulgite to enhance the removal efficiency of Norfloxacin (NOR) from aqueous solution. Batch sorption experiments indicated that NOR was adsorbed to the clay–biochar strongly with a maximum sorption capacity of 5.24 mg g−1, which was about 1.68 times higher than the pristine biochar (PB).
3.2.2 Combination attapulgite with graphene oxide (GO). Graphene oxide (GO), as a graphene derivative, is a two-dimensional (2D) structure material. GO (or rGO) is often combined with metals, metal oxides, polymer materials as precursor or support carrier due to its special characters of easy to functionalize and high controllability, it can also provide large specific surface area to make the attached materials disperse uniformly and prevent agglomeration.98,99 Recent researches on combination ATP with GO are about making composite membranes or catalysts. Cui et al.100 successfully constructed a three-dimensional attapulgite (APT) layer with a sandwich-like structure through a gentle and widely applicable method, no need for any additional modification of the original membranes. This method employs ordinary material with underwater superoleophobicity towards the effective and fast separation of different oil-in-water emulsions and adsorption of organic dyes and heavy metal ions. Luo et al.101 assembled an attapulgite (ATP) nanofibers/GO composite (ATP/GO) membrane by filtration of mixed aqueous colloidal suspensions of ATP and GO, which had a high water flux of 221.16 L m−2 h−1 bar−1, 7.7 times higher than pure GO membrane. Wang et al.102 successfully fabricated graphene oxide/attapulgite (GO/APT) composite membranes by the vacuum-assisted filtration for efficient dyes wastewater treatment. The water permeated flux was as high as 13.3 L m−2 h−1 of GO/APT membrane with preserving high rejection nearly to 100% for 7.5 mg per L Rh B wastewater under optimized conditions. Zhang et al.103 prepared a novel rGO–ATP supported Fe2O3 catalyst for oxidation of ciprofloxacin and got over 80% removal ratio at optimal conditions.
3.2.3 Combination attapulgite with g-C3N4. g-C3N4 is a two-dimensional layered structure material similar to graphene. As a new non-metallic photocatalytic material, g-C3N4 has a wider absorption spectrum range and does not require ultraviolet light, it can play a photocatalytic role under ordinary visible light.104,105 Liu et al.106 synthesized attapulgite (APT) and C3N4 hybridized metal–organic frameworks (MOFs) via different strategies, and systematically studied its adsorption properties for alizarin yellow GG (AYGG). The result showed that APT/C3N4 had excellent adsorption capacity synthesized both by refluxing method (366.0 mg g−1) and by hydrothermal method (271.8 mg g−1).
3.3 Loading metal or metal oxides on attapulgite
Metals/metal oxides (zero valent iron, Cu, Co, Fe2O3, Fe3O4, CuO, CeO2 et al.) modification involves the deposition or incorporation of metal particles or metal oxides onto the surface of attapulgite. This modification can introduce new catalytic active sites, thereby enhancing the catalytic performance of attapulgite for various reactions. The specific metals or metal oxides used for modification depend on the desired catalytic application (Fig. 3 right).
3.3.1 Loading zero-valent iron (nZVI) on attapulgite. Nanoscale zero-valent iron (nZVI) technology is widely applied to treating numerous organic and inorganic pollutants because of its strong reducibility and high reaction activity.107 However it is easy to be oxidized and agglomerate in water, in order to overcome these shortcomings, attapulgite is used as a support on which nZVI can be loaded and uniformly dispersed. For examples, Frost et al.108 used attapulgite loaded nZVI to remove methylene blue from water, and the loaded adsorbent has higher adsorption capacity than before. A nanocomposite of nZVI/ATP109 with nanoscale zero-valent iron (nZVI) directly supported on attapulgite (ATP) was synthesized for activating peroxymonosulfate (PMS) to generate reactive free radicals for oxidation of quinclorac. The removal efficiency of quinclorac was 97.36% in an hour using 0.5 g per L nZVI/ATP and 10 mM PMS.
3.3.2 Loading Fe oxides on attapulgite. As active components, Fe oxides are better than nZVI due to the fact that they are not easy to lose and have broad environmental adaptability. In these cases, metal salts were usually used as precursors, such as nitrates, carbonates, sulfates and chlorides. Fe2O3/ATP was often used due to easy preparation and high catalytic efficiency.110 Fe3O4 normally worked as the carrier of magnetic material111 and it can also be applied with ATP to facilitate the removal of cyanobacteria and heavy metals.112Zhang et al.113 prepared the Fe3O4/ATP catalyst by impregnation method, and nano-Fe3O4 particles were coated evenly onto the surface of ATP. Fe3O4/ATP was used as the catalyst to decolorize methylene blue (MB), and more than 99% MB decolorization were achieved under the optimum conditions.
3.3.3 Loading multi-metal oxides on attapulgite. Multiple metal oxide combinations can take an effect of synergistic oxidation. Zhang et al.114 prepared multi-metal oxides loaded catalysts (CuO–Fe2O3/ATP, CeO2–Fe2O3/ATP and CuO–CeO2–Fe2O3/ATP) by impregnation method with CuO, CeO2 or Fe2O3 directly coated on ATP surface for the degradation of MB. Under the optimum conditions, the as-synthesized three catalysts all showed more than 99% degradation efficiency of MB. Various metal oxides can also be loaded onto ATP in the form of alloys. Tan et al.115 designed and prepared Bi2MoO6/attapulgite photocatalyst for the removal of tetracycline and formaldehyde, and got 1.7 times of removal ratio higher than pure Bi2MoO6. For endowing attapulgite-based catalysts with photocatalytic ability, TiO2 is often used as one of the active materials. An environmentally friendly photocatalyst was synthesized by introducing BiOCl–TiO2 hybrid oxide onto the surface of attapulgite (ATT) (denoted as ATT–BiOCl–TiO2)116 and used for the decomposition of methyl orange (MO). It was found that 100 mg per L MO was totally decomposed under the UV light within 70 min and 92.57% of MO was decomposed under the visible light within 120 min by using ATT–BiOCl–TiO2 as photocatalyst. Magnetically separable attapulgite–TiO2–FexOy composites also prepared for photodegradation of methyl orange under visible light radiation and got the highest methyl orange degradation ratio of 94.13% and COD removal of 90.91%.117
3.3.4 Compound modification of attapulgite. In some cases, researchers hope to achieve multi functions of attapulgite simultaneously, especially the excellent adsorption and catalytic performance, therefore attapulgite is often modified by compound methods. Attapulgite is often firstly modified by organics then coated with metals or metal oxides, as shown in Fig. 4. The surface modification of attapulgite by organics can change the surface functional groups, hydrophobicity and pore structure of attapulgite, and loading active components on ATP can make it have high catalytic performance. Zhang et al.122 prepared catalyst Fe/OATP for HA–Na degradation. Fe/OATP is a heterogeneous catalyst synthesized by loading active substances—nanoscale zero valent iron—on the surface of organo-attapulgite in which ATP was modified by octadecyl trimethyl ammonium chloride (OATC), as seen in Fig. 4 (example 1). The removal ratio of HA–Na by Fe/OATP can reach more than 97% under the optimum conditions. Chen et al.123 successfully fabricated polypyrrole/attapulgite-supported nanoscale zero-valent iron (nZVI–Ppy/ATP) composites by chemical oxidative polymerization and liquid-phase reduction method (Fig. 4 example 2), and employed it to extract Naphthol Green B (NGB) from aqueous solution. The results showed that 99.59% of NGB was removed using nZVI–Ppy/ATP after 25 min. Fe3O4/attapulgite/polyvinylalcohol composites124 were successfully prepared by the method of coprecipitation. The composites had the ability of treating methyl orange effectively in neutral condition. When the dosage of H2O2 was 15 mL and the laying time was 24 h, the efficiency of treatment was 99.99%. Other porous supports are often combined with ATP to improve specific surface area, porosity and catalytic performance of ATP. Li et al.125 prepared a novel ATP/Ce1−xZrxO2 nanocomposite by a facile homogeneous deposition method. The catalytic activity of ATP/Ce1−xZrxO2 was investigated by oxidizing methylene blue with O3, and a maximum degradation ratio (99%) of methylene blue is achieved. Single-atom Cu-supported attapulgite/polymer carbon nitride (PCN/ATP) photocatalyst was successfully synthesized and its degradation rate toward methylene blue dye was 7.7 times higher than that of PCN (Fig. 4 example 3) (Table 2).126
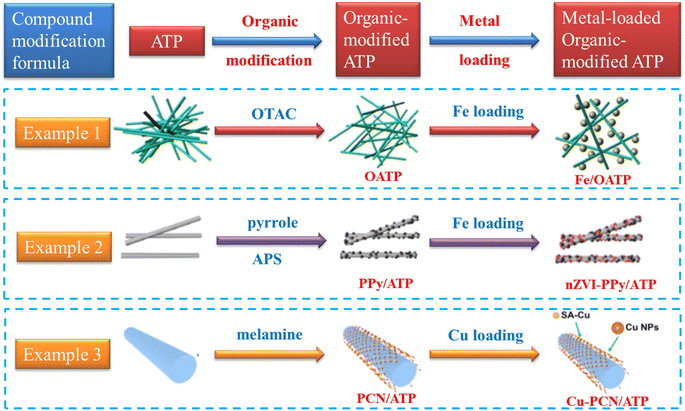 |
| Fig. 4 Compound modification processes of ATP.122,124,126 Copyright 2024, Elsevier. | |
Table 2 The summary of different modified attapulgite
Modification methods |
Modifiers |
Targets |
Applications |
Ref. |
Organic modification |
Grafting |
PAM, OTAC, OTAB, DDAB, CA |
To change the surface functional groups of ATP |
Adsorption of dyes, phenol and tannin |
65, 68, 73, 74 and 78 |
Silane-coupling |
APTES |
To change the hydrophobicity of ATP surface |
Adsorption of oil pollutants |
75 and 79 |
Coating |
Chitosan, polyaniline, polyurethane |
To change surface structure of ATP |
Adsorption of dyes, organic pesticides, fats, and other toxic substances in wastewater |
80–84 |
Modification ATP by carbon-based materials |
3D-C |
Carbon, biochar |
To enhance specific surface area of ATP |
Adsorption of dyes and antibiotics |
95–97 |
2D-C |
GO |
To enhance specific surface area and conductivity of ATP |
Removal of dyes |
98–103 |
g-C3N4 |
To enhance specific surface area and photocatalysis ability of ATP |
Removal of dyes |
104–106 |
Loading metal or metal oxides on ATP |
Fe |
nZVI |
To improve reducibility and high reaction activity of ATP |
Adsorption and catalytic reduction of dyes and organic pesticides |
107–109 |
Fe oxides |
Fe2O3, Fe3O4 |
To improve catalytic ability of ATP |
Catalytic reduction of dyes and other organics |
110–113 |
Multi-metal oxides |
CuO–Fe2O3, CeO2–Fe2O3, BiOCl–TiO2 |
To achieve an effect of synergistic oxidation |
Catalytic reduction of dyes and antibiotics |
114–117 |
Compound modification of attapulgite |
|
Organics and metals or metal oxides |
To achieve multi functions of ATP simultaneously |
Catalytic reduction of dyes, antibiotics and HA–Na |
122–126 |
4 Characterizations of attapulgite composite materials
The morphology and structure properties of attapulgite-based composite materials play a crucial role in their adsorption and catalytic efficiency, making them directly relevant to their performance. Characterization techniques such as BET, SEM, TEM, FT-IR, XRD, and XPS are commonly used to analyze attapulgite and attapulgite-based catalysts. These techniques provide valuable insights into the surface area, pore structure, elemental composition, phase purity, surface functional groups, and other properties that influence their performance.
SEM (Scanning Electron Microscope) tests are essential for observing the morphology of attapulgite and its composites. These tests allow for a close examination of the rod-shaped crystals of attapulgite as well as any new morphologies introduced by other materials. The SEM technique also provides valuable insights into the loading situation of activated materials, such as metals and metal oxides, within the composite, as shown in Fig. 5a.
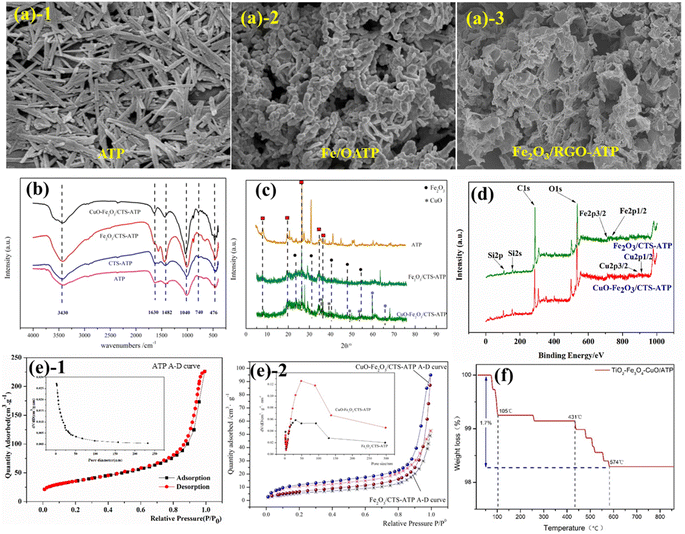 |
| Fig. 5 Characterizations of attapulgite composite materials (a) SEM graphs (b) FT-IR curves, (c) XRD curves, (d) XPS, (e) BET A–D curves and (f) TG curve.103,114,122,128 Copyright 2024, MDPI. | |
FTIR (Fourier Transform Infrared) tests are applied to characterize the molecular structure and chemical bonds of attapulgite composites. As a silico-aluminate mineral, attapulgite exhibits infrared spectral characteristic peaks that provide insights into its structure. The prominent peaks observed around 3500 cm−1 primarily correspond to the –O–H stretching vibration. Specifically, the absorption peak located at 3550 cm−1 represents the stretching vibration of crystalline water within attapulgite, while the peak at 3480 cm−1 signifies the stretching vibration of surface water and zeolite water present in attapulgite. Furthermore, the peak situated around 1655 cm−1 is primarily attributed to the H–O–H bending vibration of water molecules. Lastly, the peak near 1250 cm−1 is primarily associated with the bonding region between Si and Al. On this basis, researchers should be able to observe whether there are new peaks appearing after combination with or loading on attapulgite, as shown in Fig. 5b.
XRD (X-ray Diffraction) tests are employed to identify the crystal structure of attapulgite composite materials. The characteristic diffraction peaks of attapulgite are located at 2θ = 8.35°, 13.77°, 19.82°, and 27.58°, respectively corresponding to (110), (200), (040), and (102) faces of attapulgite.127 When attapulgite is combined with other substances, new peak positions and crystalline phases will appear, proving that there are indeed new substances combining with it, as shown in Fig. 5c.
XPS tests are used to analyze the elemental and chemical valence states as well as valence electron states on the surface of attapulgite composite materials. The main elements of attapulgite are O, Si, C, Al. After loading with active substances, some metal elements such as Fe, Cu, Ti, etc. (based on the loading substances) may be present. The valence states of these elements can be analyzed based on the binding energy (Fig. 5d).
BET tests are to determine the specific surface area of micropores of attapulgite and its composite materials. The specific surface area of attapulgite clay is about 110–130 m2 g−1, after being combined with other substances or loaded with active substances, its specific surface area may increase or decrease. However, researches have shown that the catalytic activity of attapulgite material is not directly proportional to its size of specific surface area. Sometimes, when the specific surface area decreases, the catalytic activity actually increases (Fig. 5e).
In addition to above characterizations, there are other testing methods, such as Raman spectroscopy and thermogravimetry (Fig. 5f), that can be employed to characterize attapulgite-based composite materials. It's worth noting that these tests are not discrete, but rather, they complement one another, offering a more comprehensive and accurate representation of the material's properties. By integrating these various testing methods, a more comprehensive understanding of the nature and performance of attapulgite-based composite materials can be achieved, ultimately aiding in their optimal application.
5 The mechanism of attapulgite composite materials removing contaminants from water
5.1 Adsorption mechanisms
The possible adsorption mechanisms of the refractory organics by attapulgite-based absorbents are based on the following properties: (i) the micropores, mesopores, and macropores structure of ATP or ATP-based composites, which facilitates the free diffusion of pollutants and guarantees mass transport to the internal structure; (ii) after introduction of organics to ATP,74–79 the surface functional groups, surface structure and the hydrophobicity of ATP had been changed, fully increased the exposed active sites (–NH3, oxygen-containing groups etc.) and negative charges, which made it favorable for encountering and capturing cationic molecules via hydrogen bonds and electrostatic interaction;81 (iii) combined ATP with carbon series materials (GO, g-C3N4)95–106 or Fe series materials (nZVI)107–109 to enhance specific surface area and conductivity, and these substances on ATP maybe have chemical reactions with the adsorbates,107,109 reach the goal of refractory organics removal; (iv) the crystalline water in the structure of attapulgite is located on the surface of its channel, which is easy to form hydrogen bonds with the adsorbate entering the channel;95–97 (v) some ATP based catalysts (such as APT/C@NiFe-LDHs96) with primarily p–p stacking could act as electron acceptors and be conducive to adsorbing the cationic pollutants with unsaturated double bond or conjugate structure, and cation–π bonding95 can be formed between cation and π – rich electronic structure by electrostatic attraction and polarization. All these above examples reveal that π–π interaction, hydrophobic effect, cation–π bonding, chemical complexation, hydrogen bond and electrostatic attraction are mainly involved in the adsorption process. The diagram of adsorption mechanisms by attapulgite-based absorbents can be seen in Fig. 6.
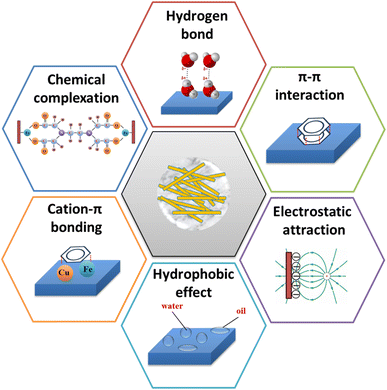 |
| Fig. 6 The diagram of adsorption mechanisms by attapulgite-based absorbents. | |
5.2 Catalysis mechanisms
According to the different active components, the catalytic mechanisms of attapulgite-based catalysts for refractory organics can be divided into three types: heterogeneous Fenton process, photocatalysis and electric catalysis.
5.2.1 ATP-based heterogeneous Fenton catalysts. In general, iron or iron oxides (active components) were loaded on ATP or ATP composites, and the active components can catalyze and decompose the adsorbed hydrogen peroxide (or peroxysulfate) into ˙OH (or ˙SO4−) which have strong oxidation and can oxidize and mineralize most of the organic compounds129,130 (Fig. 7). Attapulgite-based heterogeneous catalysts can catalyze and oxide organic pollutants effectively within a wide pH range, which is beneficial for in situ remediations of polluted groundwater and soil and can be reused for further runs. Heterogeneous Fenton-like reaction is a surface-controlled reaction that depends on the catalyst surface area, H2O2 (or peroxysulfate) concentration, the reaction temperature, and solution pH and ionic strength.41,131
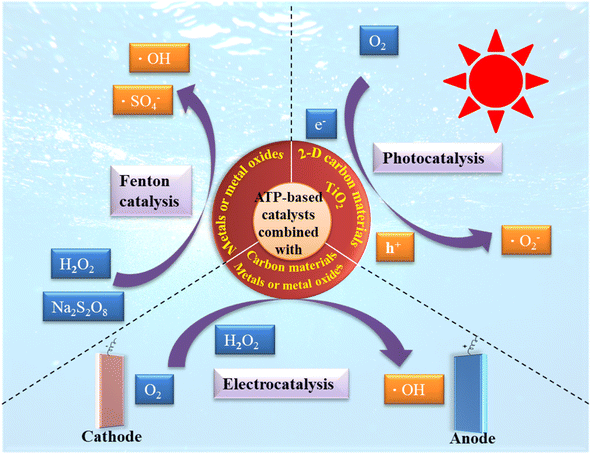 |
| Fig. 7 Schematics of heterogeneous catalysis processes by ATP-based catalysts. | |
5.2.2 ATP-based photocatalysts. An ideal photocatalyst is envisioned to exhibit exceptional performance characteristics, encompassing a suitable band edge potential, narrow band gap energy, enhanced charge separation, improved charge transportation, and minimized recombination.132,133 To achieve this, attapulgite-based photocatalytic materials undergo modifications through alterations in their chemical compositions, achieved by methods such as doping,134 composite formation,135 metal sensitization, and molecule functionalizations.136 Additionally, modifications are made to their physical structures, encompassing adjustments in size, shape, and surface morphology.The fundamental principle underlying attapulgite-based photocatalysis is rooted in the theory of solid energy bands. When the light irradiation absorbed by the semiconductor components of catalysts (TiO2, CdS, etc.) surpasses the width of the photon band gap, electron–hole pairs are generated as a result of the transition of electrons. This light irradiation prompts the excitation of electrons from the valence band (VB) to the conduction band (CB), simultaneously creating holes in the VB.137,138 Subsequently, these electrons and holes, stimulated by the light radiation, migrate to the surface of the semiconductor particles through various interactions. Once there, they react with water or organic substances adsorbed on the surface of the semiconductor catalyst particles, thereby producing a photocatalytic effect.,139,140 as shown in Fig. 7.
5.2.3 ATP-based electrocatalyst. ATP-based electrocatalysis process covers two steps: electrode reaction and catalysis. The electrode can be combined with or separated from the ATP-based catalyst. Firstly, H2O2 is formed from O2 by anodic reaction at the anode; then H2O2 is decomposed into ˙OH under the catalysis of iron containing ATP-based catalyst, which can degrade refractory organic compounds into CO2.35,141 Most of the electrocatalysis processes have the same mechanism (Fig. 7), they just used different electrodes and catalysts.
6 Future perspectives and attractions
Attapulgite indeed offer promising applications in the treatment of refractory organic wastewater due to its abundant reserves and low prices, easy modification and good compatibility, environmental friendliness, unique nanostructure and excellent performance. The attributes of attapulgite make it an attractive candidate for the development of novel composite materials for refractory organic wastewater treatment. With further research and optimization, attapulgite-based composites have the potential to become cost-effective and sustainable solutions for water pollution control.
It is necessary to further strengthen fundamental research, promote removal efficiency and solve the key problems restricting attapulgite applications. The researches of attapulgite composite materials will be carried out in the following aspects.
(1) Deeply explore the chemical and physical properties of attapulgite and attapulgite-based composite materials, as well as their reaction mechanisms in removing organic pollutants from the molecular level. Optimize the design and preparation of attapulgite composite materials through experimental and simulation methods to achieve higher removal efficiency and stability.
(2) The integration of attapulgite with other novel advanced materials could lead to the development of hybrid materials (such as aerogel materials, membrane materials, etc.) with multi-functional properties including filtration, adsorption and catalysis.
(3) Emphasizing the use of renewable and biodegradable modifiers in the synthesis of attapulgite composites could promote more sustainable wastewater treatment practices. Scaling up of attapulgite composites production and integrating them into commercial wastewater treatment systems could significantly contribute to environmental protection and sustainability.
7 Conclusion
Attapulgite exhibits a certain adsorption capacity and catalysis ability for organic pollutants in water due to its unique rod-shaped morphology and pore structure. However, the specific surface area and accumulation nature of natural attapulgite limit its widespread application in increasingly complex wastewater treatments. To overcome these limitations, attapulgite can be modified with organics, carbon series materials, and Fe series materials, significantly enhancing its adsorption and catalysis performance. These modified attapulgite-based materials effectively improve wastewater treatment efficiency, material recycling performance, and significantly reduce treatment costs. While surface modification maybe “sacrifice” the negative charge and pore of attapulgite composites, organic or inorganic modification of attapulgite exhibit good adsorption and catalysis performance due to the surface functional groups carried by the organics or inorganic materials.
At present, the main application problem of attapulgite composite materials is that adsorption and catalysis can only occur on the surface of them, and the internal of particle adsorbent/catalyst cannot be fully utilized. Therefore, attapulgite composite materials are usually made into powder or nano particles, which are not easy to recycle. Future research on attapulgite should not only focus on harnessing the unique properties of attapulgite and the synergistic effect of surface modification, but also focus on fully utilizing attapulgite composite materials both surface and inside, and at the same time they are easy to recycle. Promising attapulgite-based materials are those with multi-functional and stable performance that can be achieved by adjusting their morphology and structure.
Author contributions
Conceptualization, T. Z. and Y. W.; methodology, T. Z. and X. H.; validation, X. H. and J. Q.; formal analysis, T. Z.; investigation, J. Q. and Y. L.; resources, T. Z.; data curation, J. Z.; writing—original draft preparation, T. Z.; writing—review and editing, T. Z. and X. H.; visualization, T. Z.; supervision, Y. W.; project administration, T. Z.; funding acquisition, T. Z. All authors have read and agreed to the published version of the manuscript.
Conflicts of interest
There are no conflicts to declare.
Acknowledgements
This work was financially supported by the National Natural Science Foundation of China (Grant No. 51302123).
References
- S. Pathak, A. K. Sakhiya, A. Anand, K. K. Pant and P. Kaushal, A state-of-the-art review of various adsorption media employed for the removal of toxic Polycyclic aromatic hydrocarbons (PAHs): an approach towards a cleaner environment, J. Water Process Eng., 2022, 47, 102674, DOI:10.1016/j.jwpe.2022.102674.
- S. Barreca, S. Bastone, E. Caponetti, D. F. C. Martino and S. Orecchio, Determination of selected polyaromatic hydrocarbons by gas chromatography-mass spectrometry for the analysis of wood to establish the cause of sinking of an old vessel (Scauri wreck) by fire, Microchem. J., 2014, 117, 116–121, DOI:10.1016/j.microc.2014.06.020.
- C. C. Martins, M. C. Bícego, M. M. Mahiques, R. C. L. Figueira, M. G. Tessler and R. C. Montone, Polycyclic aromatic hydrocarbons (PAHs) in a large south american industrial coastal area (Santos estuary, southeastern Brazil): sources and depositional history, Mar. Pollut. Bull., 2011, 63, 452–458, DOI:10.1016/j.marpolbul.2011.03.017.
- H. I. Abdel-Shafy and M. S. M. Mansour, A review on polycyclic aromatic hydrocarbons: source, environmental impact, effect on human health and remediation Egypt, J. Pet., 2016, 25, 107–123, DOI:10.1016/j.ejpe.2015.03.011.
- S. D. Choi, Time trends in the levels and patterns of polycyclic aromatic hydrocarbons (PAHs) in pine bark, litter, and soil after a forest fire, Sci. Total Environ., 2014, 470–471, 1441–1449, DOI:10.1016/j.scitotenv.2013.07.100.
- B. W. Yang, Z. W. Cheng, T. Yuan, Y. J. Tan, X. P. Gao and Z. M. Shen, Synergetic denitrification through co-removal of nitro and amino/N-heterocyclic compounds in supercritical water, J. Supercrit. Fluids, 2020, 158, 104417, DOI:10.1016/j.supflu.2018.11.023.
- M. X. Li, Z. X. An, Y. R. Huo, J. C. Jiang, Y. X. Zhou, H. J. Cao, Z. H. Jin, J. Xie, J. H. Zhan and M. X. He, Individual and combined degradation of N-heterocyclic compounds under sulfate radical-based advanced oxidation processes, Chem. Eng. J., 2022, 442, 136316, DOI:10.1016/j.cej.2022.136316.
- J. X. Shi, H. J. Han and C. Y. Xu, A novel enhanced anaerobic biodegradation method using biochar and Fe(OH)3@biochar for the removal of nitrogen heterocyclic compounds from coal gasification wastewater, Sci. Total Environ., 2019, 697, 134052, DOI:10.1016/j.scitotenv.2019.134052.
- W. L. Yang, G. S. Liu, Y. H. Chen, D. T. Miao, Q. P. Wei, H. C. Li, L. Ma, K. C. Zhou, L. B. Liu and Z. M. Yu, Persulfate enhanced electrochemical oxidation of highly toxic cyanide-containing organic wastewater using boron-doped diamond anode, Chemosphere, 2020, 252, 126499, DOI:10.1016/j.chemosphere.2020.126499.
- J. T. Sun, L. L. Pan, D. C. W. Tsang, Y. Zhan, L. Z. Zhu and X. D. L, Organic contamination and remediation in the agricultural soils of China: a critical review, Sci. Total Environ., 2018, 615, 724–740, DOI:10.1016/j.scitotenv.2017.09.271.
- Y. P. He, H. B. Lin, Z. C. Guo, W. L. Zhang, H. D. Li and W. M. Huang, Recent developments and advances in boron-doped diamond electrodes for electrochemical oxidation of organic pollutants, Sep. Purif. Technol., 2019, 212, 802–821, DOI:10.1016/j.seppur.2018.11.056.
- C. G. Joseph, Y. Y. Farm, Y. H. Taufiq-Yap, C. K. Pang, J. L. H. Nga and G. L. Puma, Ozonation treatment processes for the remediation of detergent wastewater: a comprehensive review, J. Environ. Chem. Eng., 2021, 9, 106099, DOI:10.1016/j.jece.2021.106099.
- A. C. Kogawa, B. G. Cernic, L. G. D. D. Couto and H. R. N. Salgado, Synthetic detergents: 100 years of history, Saudi Pharm. J., 2017, 25, 934–938, DOI:10.1016/j.jsps.2017.02.006.
- C. Antonetti, D. Licursi, A. M. R. Galletti, M. Martinelli, F. Tellini, G. Valentini and F. Gambineri, Application of microwave irradiation for the removal of polychlorinated biphenyls from siloxane transformer and hydrocarbon engine oils, Chemosphere, 2016, 159, 72–79, DOI:10.1016/j.chemosphere.2016.05.066.
- A. M. Gutierrez, R. Bhandari, J. Y. Weng, A. Stromberg, T. D. Dziubla and J. Z. Hilt, Novel magnetic core-shell nanoparticles for the removal of polychlorinated biphenyls from contaminated water sources, Mater. Chem. Phys., 2019, 223, 68–74, DOI:10.1016/j.matchemphys.2018.10.045.
- W. L. Yang, M. S. Zhu, W. B. Li, G. Q. Liu and E. Y. Zeng, Surface-catalyzed electro-Fenton with flexible nanocatalyst for removal of plasticizers from secondary wastewater effluent, J. Hazard. Mater., 2022, 435, 129023, DOI:10.1016/j.jhazmat.2022.129023.
- L. Ghimici and M. Nichifor, Efficacy of quaternary ammonium groups based polyelectrolytes for the reduction of various pesticide formulations content from synthetic wastewater, Sep. Purif. Technol., 2021, 276, 119325, DOI:10.1016/j.seppur.2021.119325.
- P. S. Goh, N. A. Ahmad, T. W. Wong, L. T. Yogarathinam and A. F. Ismail, Membrane technology for pesticide removal from aquatic environment: status quo and way forward, Chemosphere, 2022, 307, 136018, DOI:10.1016/j.chemosphere.2022.136018.
- J. E. Gaayda, F. E. Titchou, I. Barra, I. Karmal, H. Afanga, H. Zazou, P.-S. Yap, Z. Z. Abidin, M. Hamdani and R. Ait Akbour, Optimization of turbidity and dye removal from synthetic wastewater using response surface methodology: effectiveness of Moringa oleifera seed powder as a green coagulant, J. Environ. Chem. Eng., 2022, 10, 106988, DOI:10.1016/j.jece.2021.106988.
- C. E. Lach, C. S. Pauli, A. S. Coan, E. L Simionatto and L. A. D. Koslowski, Investigating the process of electrocoagulation in the removal of azo dye from synthetic textile effluents and the effects of acute toxicity on Daphnia magna test organisms, J. Water Process Eng., 2022, 45, 102485, DOI:10.1016/j.jwpe.2021.102485.
- G. S. Soomro, C. Qu, N. Ren, S. J. Meng, X. H Li, D. W. Liang, S. J. Zhang and Y. G. Li, Efficient removal of refractory organics in landfill leachate concentrates by electrocoagulation in tandem with simultaneous electro-oxidation and insitu peroxone, Environ. Res., 2020, 183, 109249, DOI:10.1016/j.envres.2020.109249.
- T. Zhang, L. Cheng, L. Ma, F. Meng, R. G. Arnold and A. E. Sáez, Modeling the oxidation of phenolic compounds by hydrogen peroxide photolysis, Chemosphere, 2016, 161, 349–357, DOI:10.1016/j.chemosphere.2016.06.110.
- C. T. Chekem, V. Goetz, Y. Richardson, G. Plantard and J. Blin, Modelling of adsorption/photodegradation phenomena on AC-TiO2 composite catalysts for water treatment detoxification, Catal. Today, 2019, 328, 183–188, DOI:10.1016/j.cattod.2018.12.038.
- J. Huang, Treatment of phenol and p-cresol in aqueous solution by adsorption using a carbonylated hyper crosslinked polymeric adsorbent, J. Hazard. Mater., 2009, 168, 1028–1034, DOI:10.1016/j.jhazmat.2009.02.141.
- E. Xenofontos, A. M. Tanase, I. Stoica and I. Vyrides, Newly isolated alkalophilic Advenella species bioaugmented in activated sludge for high p-cresol removal, New Biotechnol., 2016, 33, 305–310, DOI:10.1016/j.nbt.2015.11.003.
- W. M. Chen, Y. F. Luo, G. Ran and Q. B. Li, An investigation of refractory organics in membrane bioreactor effluent following the treatment of landfill leachate by the O3/H2O2 and MW/PS processes, Waste Manage., 2019, 97, 1–9, DOI:10.1016/j.wasman.2019.07.016.
- J. B. Liu, P. Y. Zhang, Z. Y. Tian, R. Xu, Y. Wu and Y. H. Song, Pollutant removal from landfill leachate via two-stage anoxic/oxic combined membrane bioreactor: Insight in organic characteristics and predictive function analysis of nitrogen-removal bacteria, Bioresour. Technol., 2020, 317, 124037, DOI:10.1016/j.biortech.2020.124037.
- L. P. Gu, X. Tang, Y. Sun and H. J. Kou, Bioavailability of dissolved organic matter in biogas slurry enhanced by catalytic ozonation combined with membrane separation, Ecotoxicol. Environ. Saf., 2020, 196, 110547, DOI:10.1016/j.ecoenv.2020.110547.
- Y. B. Liu, F. Q. Liu, N. Ding, X. M. Hu, C. S. Shen, F. Lia, M. H. Huang, Z. W. Wang, W. Sand and C. C. Wang, Recent advances on electroactive CNT-based membranes for environmental applications. The perfect match of electrochemistry and membrane separation, Chin. Chem. Lett., 2020, 31, 2539–2548, DOI:10.1016/j.cclet.2020.03.011.
- C. Xue, Y. P. Mao, W. L. Wang, Z. L. Song, X. Q. Zhao, J. Sun and Y. X. Wang, Current status of applying microwave-associated catalysis for the degradation of organics in aqueous phase – a review, J. Environ. Sci., 2019, 81, 119–135, DOI:10.1016/j.jes.2019.01.019.
- M. Sun, Y. Zhang, S. Y. Kong, L. F. Zhai and S. B. Wang, Excellent performance of electro-assisted catalytic wet air oxidation of refractory organic pollutants, Water Res., 2019, 158, 313–321, DOI:10.1016/j.watres.2019.04.040.
- Z. F. Luo, D. H. Wang, W. S. Zeng and J. Yang, Removal of refractory organics from piggery bio-treatment effluent by the catalytic ozonation process with piggery biogas residue biochar as the catalyst, Sci. Total Environ., 2020, 734, 139448, DOI:10.1016/j.scitotenv.2020.139448.
- Y. Ji, Z. Pan, D. Yuan and B. Lai, Advanced treatment of the antibiotic production wastewater by ozone/zero-valent iron process, Clean: Soil, Air, Water, 2018, 46, 1700666, DOI:10.1002/clen.201700666.
- G. R. Agladze, G. S. Tsurtsumia, B. I. Jung, J. S. Kim and G. Gorelishvili, Comparative study of chemical and electrochemical Fenton treatment of organic pollutants in wastewater, J. Appl. Electrochem., 2007, 37, 985–990, DOI:10.1007/s10800-007-9325-1.
- M. Ghasemi, A. Khataee and P. Gholami, Template-free microspheres decorated with Cu-Fe-NLDH for catalytic removal of gentamicin in heterogeneous electro-Fenton process, J. Environ. Manage., 2019, 248, 109236, DOI:10.1016/j.jenvman.2019.07.007.
- J. Xu, Y. Long and D. Shen, Optimization of Fenton treatment process for degradation of refractory organics in pre-coagulated leachate membrane concentrates, J. Hazard. Mater., 2017, 323, 674–680, DOI:10.1016/j.jhazmat.2016.10.031.
- Z. Wu, F. J. Yuste-Córdoba, P. Cintas, Z. Wu, S. Mantegna and G. Cravotto, Effects of ultrasonic and hydrodynamic cavitation on the treatment of cork wastewater by flocculation and Fenton processes, Ultrason. Sonochem., 2018, 40, 3–8, DOI:10.1016/j.ultsonch.2017.04.016.
- J. Liang, S. Komarov, N. Hayashi and E. Kasai, Recent trends in the decomposition of chlorinated aromatic hydrocarbons by ultrasound irradiation and Fenton's reagent, J. Mater. Cycles Waste Manage., 2007, 9, 47–55, DOI:10.1007/s10163-006-0158-5.
- M. Derakhshan and M. Fazeli, Improved biodegradability of hardly-decomposable wastewaters from petrochemical industry through photo-Fenton method and determination of optimum operational conditions by response surface methodology, J. Biol. Eng., 2018, 12, 10, DOI:10.1186/s13036-018-0104-9.
- F. F. Dias, A. A. S. Oliveira and A. P. Arcanjo, Residue-based iron catalyst for the degradation of textile dye via heterogeneous photo-Fenton, Appl. Catal., B, 2016, 186, 136–142, DOI:10.1016/j.apcatb.2015.12.049.
- Y. Zhang, P. Wu, Z. Chen, L. Zhou, Y. Zhao, Y. Lai, Y. Duan, F. Wang and S. Li, Synergistic effect in heterogeneous Fenton degradation of tetrabromobisphenol A by MWCNT and β-CD co-modified Fe3O4, Mater. Res. Bull., 2019, 113, 14–24, DOI:10.1016/j.materresbull.2019.01.008.
- G. M. S. ElShafei, F. Z. Yehia, O. I. H. Dimitry and A. M. Badawi, Ultrasonic assisted-Fenton-like degradation of nitrobenzene at neutral pH using nanosized oxides of Fe and Cu, Ultrason. Sonochem., 2014, 21, 1358–1365, DOI:10.1016/j.ultsonch.2013.12.019.
- L. Sun, Y. Yao and L. Wang, Efficient removal of dyes using activated carbon fibers coupled with 8-hydroxyquinoline ferric as a reusable Fenton-like catalyst, Chem. Eng. J., 2014, 240, 413–419, DOI:10.1016/j.cej.2013.12.009.
- M. L. Rache, A. R. García and H. R. Zea, Azo-dye orange II degradation by the heterogeneous Fenton-like process using a zeolite Y-Fe catalyst-kinetics with a model based on the Fermi's equation, Appl. Catal., B, 2014, 146, 192–200, DOI:10.1016/j.apcatb.2013.04.028.
- S. Fukuchi, R. Nishimoto, M. Fukushima and Q. Zhu, Effects of reducing agents on the degradation of 2,4,6-tribromophenolin a heterogeneous Fenton-like system with an iron-loaded naturalzeolite, Appl. Catal., B, 2014, 147, 411–419, DOI:10.1016/j.apcatb.2013.09.032.
- N. Zhang, C. Xue, K. Wang and Z. Fang, Efficient oxidative degradation of fluconazole by a heterogeneous Fenton process with Cu-V bimetallic catalysts, Chem. Eng. J., 2020, 380, 122516, DOI:10.1016/j.cej.2019.122516.
- C. F. Bustillo-Lecompte, Advanced Oxidation Processes – Applications, Trends, and Prospects, IntechOpen, 2020, https://10.5772/intechopen.85681 Search PubMed.
- S. T. Shen, W. Jiang, Q. D. Zhao, L. Y. He, Y. F. Ma, X. Q. Zhou, J. Wang, L. Yang and Z. Q. Chen, Molten-salts assisted preparation of iron-nitrogen-carbon catalyst for efficient degradation of acetaminophen by periodate activation, Sci. Total Environ., 2023, 859, 160001, DOI:10.1016/j.scitotenv.2022.160001.
- A. B. Fortunato, F. E. B. Júnior, J. M. Moreira, T. S. B. D. Barros, A. L. D. J. Pereira, M. Aparecido Gonçalves Trindade, T. A. D. Colman, P. H. Suegama, W. R. P. Barros, F. Gozzi and C. Teodoro de Carvalho, Buriti biomass as catalysts based on activated carbon/Fe3O4 for ciprofloxacin removal by heterogeneous photo-Fenton process, J. Water Process Eng., 2022, 50, 103263, DOI:10.1016/j.jwpe.2022.103263.
- M. Hammad, S. Angel, A. K. Al-kamal, A. Asghar, A. S. Amin, M. A. Kräenbring, H. T. A. Wiedemann, V. Vinayakumar, M. Y. Ali, P. Fortugno, C. Y. Kim, T. C. Schmidt, C. W. M. Kay, C. Schulz, D. Segets and H. Wiggers, Synthesis of novel LaCoO3/graphene catalysts as highly efficient peroxymonosulfate activator for the degradation of organic pollutants, Chem. Eng. J., 2023, 454, 139900, DOI:10.1016/j.cej.2022.139900.
- J. J. An, S. S. Wang, M. X. Huang, J. Zhang and P. Wang, Removal of water-soluble lignin model pollutants with graphene oxide loaded ironic sulfide as an efficient adsorbent and heterogeneous Fenton catalyst, Arabian J. Chem., 2022, 15, 104338, DOI:10.1016/j.arabjc.2022.104338.
- K. Vignesh, M. Rajarajan and A. Suganthi, Visible light assisted photocatalystic performance of Ni and Th co-doped ZnO nanoparticles for the degradation of methylene blue dye, J. Ind. Eng. Chem., 2014, 20, 3826–3833, DOI:10.1016/j.jiec.2013.12.086.
- A. T. Vu, T. N. Xuan and C. H. Lee, Preparation of mesoporous Fe2O3·SiO2 composite from rice husk as an efficient heterogeneous Fenton-like catalyst for degradation of organic dyes, J. Water Process Eng., 2019, 28, 169–180, DOI:10.1016/j.jwpe.2019.01.019.
- K. D. Rajan, D. Srinivasan, P. P. Gotipamul, S. Khanna, S. Chidambaram and M. Rathinam, Design of a novel ZnBi2O4/Bi2O3 type-II photo-catalyst via short term hydrothermal for enhanced degradation of organic pollutants, Mater. Sci. Eng., B, 2022, 285, 115929, DOI:10.1016/j.mseb.2022.115929.
- J. X. Chen and L. Z. Zhu, Oxalate enhanced mechanism of hydroxyl-Fe-pillared bentonite during the degradation of Orange II by UV-Fenton process, J. Hazard. Mater., 2011, 185, 1477–1481, DOI:10.1016/j.jhazmat.2010.10.071.
- J. Y. Feng, X. J. Hu and P. L. Yue, Discoloration and mineralization of Orange II by using a bentonite clay-based Fe nanocomposite film as a heterogeneous photo-Fenton catalyst, Water Res., 2005, 39, 89–96, DOI:10.1016/j.watres.2004.08.037.
- M. Perderiset, P. Baillif and M. C. Jaurand, Chemical analysis and photoelectron spectroscopy of the adsorption of macromolecules on the surface of attapulgite, J. Colloid Interface Sci., 1988, 121, 381–391, DOI:10.1016/0021-9797(88)90441-9.
- J. Zhang, H. Chen and A. Wang, Study on superabsorbent composite. III. Swelling behaviors of polyacrylamide/attapulgite composite based on acidified attapulgite and organo-attapulgite, Eur. Polym. J., 2005, 41(10), 2434–2442, DOI:10.1016/j.eurpolymj.2005.03.022.
- A. Li, J. P. Zhang and A. Q. Wang, Utilization of starch and clay for the preparation of superabsorbent composite, Bioresour. Technol., 2007, 98, 327–332, DOI:10.1016/j.biortech.2005.12.026.
- J. X. Chen and L. Z. Zhu, Catalytic degradation of Orange II by UV-Fenton with hydroxyl-Fe-pillared bentonite in water, Chemosphere, 2006, 65, 1249–1255, DOI:10.1016/j.chemosphere.2006.04.016.
- Q. H. Fan, D. D. Shao, Y. Lu, W. S. Wu and X. K. Wang, Effect of pH, ionic strength, temperature and humic substances on the sorption of Ni(II) to Na–attapulgite, Chem. Eng. J., 2009, 150, 188–195, DOI:10.1016/j.cej.2008.12.024.
- Z. W. Niu, Q. H. Fan, W. H. Wang, J. Z. Xu, L. Chen and W. S. Wu, Effect of pH, ionic strength and humic acid on the sorption of uranium(VI) to attapulgite, Appl. Radiat. Isot., 2009, 67, 1582–1590, DOI:10.1016/j.apradiso.2009.03.113.
- X. P. Wu, W. Y. Zhu, X. L. Zhang, T. H. Chen and R. L. Frost, Catalytic deposition of nanocarbon onto palygorskite and its adsorption of phenol, Appl. Clay Sci., 2011, 52, 400–406, DOI:10.1016/j.clay.2011.04.011.
- E. H. Cao, R. Bryant and D. J. A. Williams, Electrochemical Properties of Na–Attapulgite, J. Colloid Interface Sci., 1996, 179, 143–150, DOI:10.1006/jcis.1996.0196.
- J. H. Huang, X. G. Wang, Q. Z. Jin, Y. F. Liu and Y. Wang, Removal of phenol from aqueous solution by adsorption onto OTMAC-modified attapulgite, J. Environ. Manag., 2007, 84, 229–236, DOI:10.1016/j.jenvman.2006.05.007.
- J. Zhang, S. D. Xie and Y. S. Ho, Removal of fluoride ions from aqueous solution using modified attapulgite as adsorbent, J. Hazard. Mater., 2009, 165, 218–222, DOI:10.1016/j.jhazmat.2008.09.098.
- Y. Tang, H. Zhang, X. N. Liu, D. Q. Cai, H. Y. Feng, C. G. Miao, X. Q. Wang, Z. Y. Wu and Z. L. Yu, Flocculation of harmful algal blooms by modified attapulgite and its safety evaluation, Water Res., 2011, 45, 2855–2862, DOI:10.1016/j.watres.2011.03.003.
- J. H. Huang, Y. F. Liu and X. G. Wang, Selective adsorption of tannin from flavonoids by organically modified attapulgite clay, J. Hazard. Mater., 2008, 160, 382–387, DOI:10.1016/j.jhazmat.2008.03.008.
- M. Li, Z. S. Wu and H. T. Kao, Study on preparation, structure and thermal energy storage property of capric–palmitic acid/attapulgite composite phase change materials, Appl. Energy, 2011, 88, 3125–3132, DOI:10.1016/j.apenergy.2011.02.030.
- J. P. Zhang, A. Li and A. Q. Wang, Study on superabsorbent composite. VI. Preparation, characterization and swelling behaviors of starch phosphate-graft-acrylamide/attapulgite superabsorbent composite, Carbohydr. Polym., 2006, 65, 150–158, DOI:10.1016/j.carbpol.2005.12.035.
- S. Liu, F. Fan, X. Liu, Y. Guo, Z. Ni and S. Wang, Old wine and new bottles: insights into traditional attapulgite adsorbents with functionalized modification strategies applied in efficient phosphate immobilization, J. Cleaner Prod., 2023, 395, 136451, DOI:10.1016/j.jclepro.2023.136451.
- A. G. N. Wamba, G. P. Kofa, S. N. Koungou, P. S. Thue, E. C. Lima, G. S. D. Reis and J. G. Kayem, Grafting of amine functional group on silicate based material as adsorbent for water purification: a short review, J. Environ. Chem. Eng., 2018, 6, 3192–3203, DOI:10.1016/j.jece.2018.04.062.
- J. P. Zhang, H. Chen and A. Q. Wang, Study on superabsorbent composite. XV. Effects of ion-exchanged attapulgite on water absorbency of superabsorbent composites, Polym. Compos., 2007, 28(2), 208–213, DOI:10.1002/pc.20384.
- B. Sarkar, Y. F. Xi, M. Megharaj and R. Naidu, Orange II adsorption on palygorskites modified with alkyl trimethylammonium and dialkyl dimethylammonium bromide—an isothermal and kinetic study, Appl. Clay Sci., 2011, 51, 370–374, DOI:10.1016/j.clay.2010.11.032.
- A. L. Xue, S. Y. Zhou, Y. J. Zhao, X. P. Lu and P. F. Han, Effective NH2-grafting on attapulgite surfaces for adsorption of reactive dyes, J. Hazard. Mater., 2011, 194, 7–14, DOI:10.1016/j.jhazmat.2011.06.018.
- H. Chen and J. Zhao, Adsorption study for removal of Congo red anionic dye using organo-attapulgite, Adsorption, 2009, 15(4), 381–389, DOI:10.1007/s10450-009-9155-z.
- X. Guo, C. He, X. Sun, X. Liang, X. Chen and X. Y. Liu, Adsorption of phenol from aqueous solution by four types of modified attapulgites, Int. J. Environ. Sci. Technol., 2019, 16, 793–800, DOI:10.1007/s13762-018-1699-6.
- Z. F. Zhang, W. B. Wang and A. Q. Wang, Highly effective removal of Methylene Blue using functionalized attapulgite via hydrothermal process, J. Environ. Sci., 2015, 33, 106–115, DOI:10.1016/j.jes.2014.12.014.
- Y. H. Deng, F. Wu, B. Z. Liu, X. B. Hu and C. Sun, Sorptive removal of β-blocker propranolol from aqueous solution by modified attapulgite: effect factors and sorption mechanisms, Chem. Eng. J., 2011, 174, 571–578, DOI:10.1016/j.cej.2011.09.057.
- Y. H. Deng, L. Wang, X. B. Hu, B. Z. Liu, Z. B. Wei, S. G. Yang and C. Sun, Highly efficient removal of tannic acid from aqueous solution by chitosan-coated attapulgite, Chem. Eng. J., 2012, 181–182, 300–306, DOI:10.1016/j.cej.2011.11.082.
- Q. Zhou, Q. Gao, W. J. Luo, C. J. Yan, Z. N. Ji and P. Duan, One-step synthesis of amino-functionalized attapulgite clay nanoparticles adsorbent by hydrothermal carbonization of chitosan for removal of methylene blue from wastewater, Colloids Surf., A, 2015, 470, 248–257, DOI:10.1016/j.colsurfa.2015.01.092.
- N. Sun, Y. Zhang, L. X. Ma, S. L. Yu and J. X. Li, Preparation and characterization of chitosan/purified attapulgite composite for sharp adsorption of humic acid from aqueous solution at low temperature, J. Taiwan Inst. Chem. Eng., 2017, 78, 96–103, DOI:10.1016/j.jtice.2017.03.017.
- J. H. Wang, X. J. Han, H. R. Ma, Y. F. Ji and L. J. Bi, Adsorptive removal of humic acid from aqueous solution on polyaniline/attapulgite composite, Chem. Eng. J., 2011, 173, 171–177, DOI:10.1016/j.cej.2011.07.065.
- K. Dong, F. X. Qiu, X. R. Guo, J. C. Xu, D. Y. Yang and K. C. He, Polyurethane–Attapulgite Porous Material: Preparation, Characterization, and Application for Dye Adsorption, J. Appl. Polym. Sci., 2013, 129, 1697–1706, DOI:10.1002/APP.38874.
- C. D. Jia, Y. D. Mi, Z. K. Liu, W. F. Zhou, H. X. Gao, S. B. Zhang and R. H. Lu, Attapulgite modified with covalent organic frameworks as the sorbent indispersive solid phase extraction for the determination of pyrethroids inenvironmental water samples, Microchem. J., 2020, 153, 104522, DOI:10.1016/j.microc.2019.104522.
- H. Cui, Y. Qian, Q. Li, Z. B. Wei and J. P. Zhai, Fast removal of Hg(II) ions from aqueous solution by amine-modified attapulgite, Appl. Clay Sci., 2013, 72, 84–90, DOI:10.1016/j.clay.2013.01.003.
- P. Sun, W. Zhang, B. Z. Zou, X. Y. Wang, L. C. Zhou, Z. F. Ye and Q. L. Zhao, Efficient adsorption of Cu(II), Pb(II) and Ni(II) from waste water by PANI@APTS-magnetic attapulgite composites, Appl. Clay Sci., 2021, 209, 106151, DOI:10.1016/j.clay.2021.106151.
- P. Liu, L. P. Jiang, L. X. Zhu, J. S. Guo and A. Q. Wang, Synthesis of covalently crosslinked attapulgite/poly(acrylic acid-co-acrylamide) nanocomposite hydrogels and their evaluation as adsorbent for heavy metal ions, J. Ind. Eng. Chem., 2015, 23, 188–193, DOI:10.1016/j.jiec.2014.08.014.
- M. F. Zou, X. Y. Chen, X. J. Lin, M. Y. Chen, N. N. Ding, L. Y. Yang and X. K. Ouyang, Fabrication of magnetic carboxyl-functionalized attapulgite/calcium alginate beads for lead ion removal from aqueous solutions, Int. J. Biol. Macromol., 2018, 120(part A), 789–800, DOI:10.1016/j.ijbiomac.2018.08.144.
- P. Liu, H. Wang and C. Pan, Surface organo-functionalization of palygorskite nanorods with γ-mercaptopropyltrimethoxysilane, Appl. Clay Sci., 2018, 159, 37–41, DOI:10.1016/j.clay.2017.08.010.
- H. Kong, Q. Li, X. Q. Zheng, P. Z. Chen, G. T. Zhang and Z. P. Huang, Lanthanum modified chitosan-attapulgite composite for phosphate removal from water: performance, mechanisms and applicability, Int. J. Biol. Macromol., 2023, 224, 984–997, DOI:10.1016/j.ijbiomac.2022.10.183.
- X. Chen, J. Cui, X. R. Xu, B. J. Sun, L. Zhang, W. Dong, C. T. Chen and D. P. Sun, Bacterial cellulose/attapulgite magnetic composites as an efficient adsorbent for heavy metal ions and dye treatment, Carbohydr. Polym., 2020, 229, 115512, DOI:10.1016/j.carbpol.2019.115512.
- Y. Wang, R. S. Zhou, C. Z. Wang, G. Z. Zhou, C. Y. Hua, Y. Y. Cao and Z. Z. Song, Novel environmental-friendly nano-composite magnetic attapulgite functionalized by chitosan and EDTA for cadmium (II) removal, J. Alloys Compd., 2020, 817, 153286, DOI:10.1016/j.jallcom.2019.153286.
- W. Zhang, P. Sun, X. Y. Wang, X. Zhang, L. Ran, Q. L. Zhao, B. Z. Zou, L. C. Zhou and Z. F. Ye, Elevating the stability and adsorption performance of metal-organic frameworks by chitosan and attapulgite for capturing methylene blue in the water, Mater. Today Commun., 2022, 31, 103601, DOI:10.1016/j.mtcomm.2022.103601.
- J. Tang, B. Mu, L. Zong and A. Q. Wang, From waste hot-pot oil as carbon precursor to development of recyclable attapulgite/carbon composites for wastewater treatment, J. Environ. Sci., 2019, 75, 346–358, DOI:10.1016/j.jes.2018.05.014.
- J. Tang, B. Mu, L. Zong and A. Q. Wang, One-step synthesis of magnetic attapulgite/carbon supported NiFe-LDHs by hydrothermal process of spent bleaching earth for pollutants removal, J. Cleaner Prod., 2018, 172, 673–685, DOI:10.1016/j.jclepro.2017.10.181.
- Y. Li, Z. W. Wang, X. Y. Xie, J. M. Zhu, R. N. Li and T. Y. Qin, Removal of Norfloxacin from aqueous solution by clay-biochar composite prepared from potato stem and natural attapulgite, Colloids Surf., A, 2017, 514, 126–136, DOI:10.1016/j.colsurfa.2016.11.064.
- X. Wang, B. Guo, W. Fu and H. Yang, Triethylenetetramine promoted rGO-Fe3O4 nanocomposites for highly efficient Fenton-like reaction, J. Water Process Eng., 2019, 31, 100814, DOI:10.1016/j.jwpe.2019.100814.
- M. B. Gu, U. Farooq, S. G. Lu, X. Zhang, Z. F. Qiu and Q. Sui, Degradation of trichloroethylene in aqueous solution by rGO supported nZVI catalyst under several oxic environments, J. Hazard. Mater., 2018, 349, 35–44, DOI:10.1016/j.jhazmat.2018.01.037.
- M. K. Cui, P. Mu, Y. Q. Shen, G. R. Zhu, L. Luo and J. Li, Three-dimensional attapulgite with sandwich-like architecture used for multifunctional water remediation, Sep. Purif. Technol., 2020, 235, 116210, DOI:10.1016/j.seppur.2019.116210.
- Z. Q. Luo, Q. L. Fang, X. Y. Xu, D. V. Raj, X. F. Zhou and Z. P. Liu, Attapulgite nanofibers and graphene oxide composite membrane for high-performance molecular separation, J. Colloid Interface Sci., 2019, 545, 276–281, DOI:10.1016/j.jcis.2019.03.027.
- C. Y. Wang, W. J. Zeng, T. T. Jiang, X. Chen and X. L. Zhang, Incorporating attapulgite nanorods into graphene oxide nanofiltration membranes
for efficient dyes wastewater treatment, Sep. Purif. Technol., 2019, 214, 21–30, DOI:10.1016/j.seppur.2018.04.079.
- T. Zhang, C. Y. Qian, P. R. Guo, S. C. Gan, L. Y. Dong, G. Bai and Q. Y. Guo, A Novel Reduced Graphene Oxide-Attapulgite (RGO-ATP) Supported Fe2O3 Catalyst for Heterogeneous Fenton-like Oxidation of Ciprofloxacin: Degradation Mechanism and Pathway, Catalysts, 2020, 10, 189, DOI:10.3390/catal10020189.
- L. Wang, S. Liu, J. Lu, Z. Yuan, D. Gao and Y. Li, Construction of ZnFe2O4/g-C3N4 nanocomposite catalyst for degradation of organic compound through photodegradation and heterogeneous Fenton oxidation, Mater. Today Sustain., 2023, 24, 100514, DOI:10.1016/j.mtsust.2023.100514.
- M. Antonopoulou, P. Bika, I. Papailias, S. K. Zervou, A. Vrettou, I. Efthimiou, G. Mitrikas, N. Ioannidis, C. Trapalis, P. Dallas, D. Vlastos and A. Hiskia, Photocatalytic degradation of organic micropollutants under UV-A and visible light irradiation by exfoliated g-C3N4 catalysts, Sci. Total Environ., 2023, 892, 164218, DOI:10.1016/j.scitotenv.2023.164218.
- Y. F. Liu, Y. Liu, C. N. Ji, Y. Zhang, Y. Wang, R. J. Qu and Y. Z. Niu, Fabrication of attapulgite/C3N4 hybridized metal organic framework nanocomposites by different strategies and study on adsorption properties for alizarin yellow GG, Powder Technol., 2022, 397, 117113, DOI:10.1016/j.powtec.2022.117113.
- Y. R. Li, H. P. Zhao and L. Z. Zhu, Remediation of soil contaminated with organic compounds by nanoscale zero-valent iron: a review, Sci. Total Environ., 2021, 760, 143413, DOI:10.1016/j.scitotenv.2020.143413.
- L. R. Frost, Y. Xi and H. He, Synthesis, characterization of palygorskite supported zero-valent iron and its application for methylene blue adsorption, J. Colloid Interface Sci., 2010, 341, 153–161, DOI:10.1016/j.jcis.2009.09.027.
- C. X. Ding, S. J. Xiao, Y. J. Lin, P. Yu, M. Zhong, L. H. Yang, H. Wang, L. Su, C. J. Liao, Y. Y. Zhou, Y. C. Deng and D. X. Gong, Attapulgite-supported nano-Fe0/peroxymonsulfate for quinclorac removal: performance, mechanism and degradation pathway, Chem. Eng. J., 2019, 360, 104–114, DOI:10.1016/j.cej.2018.11.189.
- T. Zhang and Z. R. Nan, Decolorization of Methylene Blue and Congo Red by Attapulgite-based Heterogeneous Fenton Catalyst, Desalin. Water Treat., 2016, 57, 4633–4640, DOI:10.1080/19443994.2014.992969.
- J. Qi, H. Lan and R. Liu, Efficient Microcystis aeruginosa removal by moderate photocatalysis-enhanced coagulation with magnetic Zn-doped Fe3O4 particles, Water Res., 2020, 171, 115448, DOI:10.1016/j.watres.2019.115448.
- L. F. Chen, X. R. Wu, C. Liu, Y. M. Zhao and Q. Huang, Study on removal of Microcystis aeruginosa and Cr(VI) using attapulgite-Fe3O4 magnetic composite material (MCM), Algal Res., 2021, 60, 102501, DOI:10.1016/j.algal.2021.102501.
- T. Zhang, C. Qian and L. Dong, Preparation of Particle Reusable Heterogeneous Catalyst Fe3O4/ATP for Methylene Blue Decolorization, Int. J. Environ. Sci. Dev., 2019, 10, 343–349, DOI:10.18178/ijesd.2019.10.10.1197.
- T. Zhang, L. Y. Dong, J. H. Du, C. Y. Qian and Y. Wang, CuO and CeO2 assisted Fe2O3/attapulgite catalyst for heterogeneous Fenton-like oxidation of methylene blue, RSC Adv., 2020, 10, 23431–23439, 10.1039/D0RA03754K.
- Y. Tan, C. J. Yin, S. L. Zheng, Y. H. Di, Z. M. Sun and C. Q. Li, Design and controllable preparation of Bi2MoO6/attapulgite photocatalyst for the removal of tetracycline and formaldehyde, Appl. Clay Sci., 2021, 215, 106319, DOI:10.1016/j.clay.2021.106319.
- L. L. Zhang, J. H. Zhang, W. G. Zhang, J. Q. Liu, H. Zhong and Y. J. Zhao, Photocatalytic activity of attapulgite–BiOCl–TiO2 toward degradation of methyl orange under UV and visible light irradiation, Mater. Res. Bull., 2015, 66, 109–114, DOI:10.1016/j.materresbull.2015.02.029.
- J. H. Zhang, L. L. Zhang, S. Y. Zhou, H. Q. Chen, H. Zhong, Y. J. Zhao and X. Wang, Magnetically separable attapulgite–TiO2–FexOy composites with superior activity towards photodegradation of methyl orange under visible light radiation, J. Ind. Eng. Chem., 2014, 20, 3884–3889, DOI:10.1016/j.jiec.2013.12.094.
- Z. H. Yin, Y. G. Liu, X. F. Tan, L. H. Jiang, G. M. Zeng, S. B. Liu, S. R. Tian, S. J. Liu, N. Liu and M. F. Li, Adsorption of 17β-estradiol by a novel attapulgite/biochar nanocomposite: characteristics and influencing factors, Process Saf. Environ. Prot., 2019, 121, 155–164, DOI:10.1016/j.psep.2018.10.022.
- H. T. Xie, J. N. Zhang, D. Wang, J. Liu, L. D. Wang and H. N. Xiao, Construction of three-dimensional g-C3N4/attapulgite hybrids for Cd(II) adsorption and the reutilization of waste adsorbent, Appl. Surf. Sci., 2020, 504, 144456, DOI:10.1016/j.apsusc.2019.144456.
- L. Dai, K. Meng, W. F. Zhao, T. Han, Z. L. Lei, G. Ma, C. F. Wu and H. H. Jia, Enhanced removal of Cd2+ by nano zero-valent iron modified attapulgite from aqueous solution: optimal design, characterization and adsorption mechanism, J. Environ. Chem. Eng., 2022, 10, 107719, DOI:10.1016/j.jece.2022.107719.
- J. M. Ji and W. L. Xie, Removal of aflatoxin B1 from contaminated peanut oils using magnetic attapulgite, Food Chem., 2021, 339, 128072, DOI:10.1016/j.foodchem.2020.128072.
- T. Zhang, J. Liu, M. Chen and Y. Wang, Preparation of heterogeneous Fenton catalyst Fe/organo-attapulgite and its performance in sodium humate degradation, Desalin. Water Treat., 2018, 107, 91–99, DOI:10.5004/dwt.2018.22111.
- Y. Chen, Z. H. Lin, R. G. Hao, H. Xu and C. Y. Huang, Rapid adsorption and reductive degradation of Naphthol Green B from aqueous solution by polypyrrole/attapulgite composites supported nanoscale zero-valent iron, J. Hazard. Mater., 2019, 371, 8–17, DOI:10.1016/j.jhazmat.2019.02.096.
- Y. B. Tong, H. S. Liu, Z. L. He, J. J. Deng, F. K. Meng, L. Q. Sun and L. Zhao, Preparation of Fe3O4/Attapulgite/Polyvinylalcohol Composites and Its Treatment for Methyl Orange in Neutral Condition, Environ. Prog. Sustainable Energy, 2016, 35(3), 715–718, DOI:10.1002/ep.12281.
- X. Z. Li, C. Y. Ni, C. Yao and Z. G. Chen, Development of attapulgite/Ce1−xZrxO2 nanocomposite as catalyst for the degradation of methylene blue, Appl. Catal., B, 2012, 117, 118–124, DOI:10.1016/j.apcatb.2012.01.008.
- Y. Liu, L. Xu, N. Zhang, J. Wang, X. Mu and Y. Wang, A promoted charge separation/transfer and surface plasmon resonance effect synergistically enhanced photocatalytic performance in Cu nanoparticles and single-atom Cu supported attapulgite/polymer carbon nitride photocatalyst, Mater. Today Chem., 2022, 26, 101250, DOI:10.1016/j.mtchem.2022.101250.
- H. Ma, X. F. Zhang, Z. G. Wang, L. Song and J. F. Yao, Flexible cellulose foams with a high loading of attapulgite nanorods for Cu2+ ions removal, Colloids Surf., A, 2021, 612, 126038, DOI:10.1016/j.colsurfa.2020.126038.
- T. Zhang, W. H. Li, Q. Y. Guo, Y. Wang and C. L. Li, Preparation of a Heterogeneous Catalyst CuO-Fe2O3/CTS-ATP and Degradation of Methylene Blue and Ciprofloxacin, Coatings, 2022, 12, 559, DOI:10.3390/coatings12050559.
- M. Xia, C. Chen, M. Long, C. Chen, W. Cai and B. Zhou, Magnetically separable mesoporous silica nanocomposite and its application in Fenton catalysis, Microporous Mesoporous Mater., 2011, 145, 217–223, DOI:10.1016/j.micromeso.2011.05.017.
- R. Matta, K. Hanna and S. Chiron, Fenton-like oxidation of 2,4,6-trinitrotoluene using different iron minerals, Sci. Total Environ., 2007, 385, 242–251, DOI:10.1016/j.scitotenv.2007.06.030.
- W. P. Kwan and B. M. Voelker, Rates of hydroxyl radical generation and organic compound oxidation in mineral-catalyzed Fenton-like systems, Environ. Sci. Technol., 2005, 39, 9303–9308, DOI:10.1021/es020874g.
- F. Opoku, K. K. Govender, C. G. C. E. V. Sittert and P. P. Govender, Recent progress in the development of semiconductor-based photocatalyst materials for applications in photocatalytic water splitting and degradation of pollutants, Adv. Sustainable Syst., 2017, 1, 1700006, DOI:10.1002/adsu.201700006.
- K. R. Reddy, C. V. Reddy, M. N. Nadagouda, N. P. Shetti, S. Jaesool and T. M. Aminabhavi, Polymeric graphitic carbon nitride (g-C3N4)-based semiconducting nanostructured materials: synthesis methods, properties and photocatalytic applications, J. Environ. Manage., 2019, 238, 25–40, DOI:10.1016/j.jenvman.2019.02.075.
- C. H. Ravikumar, M. S. A. Mahto, R. T. Nanjundaiah, R. Thippeswamy, S. R. Teixeira and R. G. Balakrishna, Observation of oxo-bridged yttrium in TiO2 nanostructures and their enhanced photocatalytic hydrogen generation under UV/visible light irradiations, Mater. Res. Bull., 2018, 104, 212–219, DOI:10.1016/j.materresbull.2018.04.014.
- L. P. D'Souza, S. Muralikrishna, H. R. Chandan, T. Ramakrishnappa and R. G. Balakrishna, Neodymium doped titania as photoanode and graphene oxide-CuS composite as a counter electrode material in quantum dot solar cell, J. Mater. Res., 2015, 30, 3241–3251, DOI:10.1557/jmr.2015.314.
- H. R. Chandan, J. D. Schiffman and R. G. Balakrishna, Quantum dots as fluorescent probes: synthesis, surface chemistry, energy transfer mechanisms, and applications, Sens. Actuators, B, 2018, 258, 1191–1214, DOI:10.1016/j.snb.2017.11.189.
- S. N. Ahmed and W. Haide, Heterogeneous photocatalysis and its potential applications in water and wastewater treatment: a review, Nanotechnology, 2018, 29, 342001, DOI:10.1088/1361-6528/aac6ea.
- A. Ibhadon and P. Fitzpatrick, Heterogeneous photocatalysis: recent advances and applications, Catalysts, 2013, 3, 189, DOI:10.3390/catal3010189.
- L. Lu, R. Shan, Y. Shi, S. Wang and H. Yuan, A novel TiO2/biochar composite catalysts for photocatalytic degradation of methyl orange, Chemosphere, 2019, 222, 391–398, DOI:10.1016/j.chemosphere.2019.01.132.
- S. Sun, R. Zhao, Y. Xie and Y. Liu, Photocatalytic degradation of aflatoxin B1 by activated carbon supported TiO2 catalyst, Food Control, 2019, 100, 183–188, DOI:10.1016/j.foodcont.2019.01.014.
- C. Zhang, M. Zhou, G. Ren, X. Yu, L. Ma, J. Yang and F. Yu, Heterogeneous electro-Fenton using modified iron-carbon as catalyst for 2,4-dichlorophenol degradation: influence factors, mechanism and degradation pathway, Water Res., 2015, 70, 414–424, DOI:10.1016/j.watres.2014.12.022.
|
This journal is © The Royal Society of Chemistry 2024 |