DOI:
10.1039/D0FO02125C
(Paper)
Food Funct., 2021,
12, 107-118
Blueberry phenolics are associated with cognitive enhancement in supplemented healthy older adults†
Received
11th August 2020
, Accepted 1st December 2020
First published on 15th December 2020
Abstract
Blueberries (BB) contain an array of bioactive phenolic compounds that may play a protective role against various age-related diseases. Here we explored the metabolic fate of BB phenolics and their relationship to cognitive function after chronic (90 days) supplementation of freeze-dried BB (24 g d−1, equivalent to 1 cup of fresh BB) or control in a randomized, double-blind, parallel study with 38 healthy older adults (60–75 years). Blood samples were collected at fasting (t = 0 h) and 2 h after a breakfast meal on days 0 (no treatment), 45, and 90, and a battery of cognitive tests was also conducted on these days. Hippuric acid, phloroglucinaldehyde, syringic acid, ferulic acid-glucuronide, cyanidin-3-O-galactoside, cyanidin-3-O-glucoside, malvidin-3-O-galactoside, malvidin-3-O-glucoside, peonidin-3-O-xyloside, peonidin glucuronide, and petunidin-3-O-glucoside concentrations were significantly altered after 90 days of BB consumption compared to control. Stepwise regression was used to assess the relationship between significantly altered concentrations of plasma phenolics and observed improvements in cognition. Among participants in the BB group, changes in switch errors on the task-switching test (TST) from day 0 to 90 were associated with changes in postprandial levels of plasma ferulic acid-glucuronide, syringic acid, and malvidin-3-galactoside (R2 = 0.521, p < 0.05). Changes in repetition errors on the California Verbal Learning Test (CVLT-II) from day 0 to 90 were associated with changes in postprandial levels of ferulic acid-glucuronide, syringic acid, and hippuric acid (R2 = 0.807, p < 0.001). These findings demonstrate that the addition of easily achievable quantities of BB to the diets of older adults significantly alters levels of circulating phenolic compounds which are related to improvements in cognition.
Introduction
Aging is often accompanied by declines in cognitive function, leading to lower quality of life and increased need for care among older adults.1 Diets rich in fruits and vegetables can reduce the risk of age-related cognitive impairment, in part due to the presence of bioactive phytochemicals in these foods, e.g., polyphenols.2 Studies in aged rats demonstrate that berry polyphenols are bio- and neuro-available, many of which have been linked to improvements in cognitive performance.3
Blueberries (BB), in particular, have received increased attention due to their potential neuroprotective effects.4 Dietary interventions with BB have shown improvements in cognitive function in rodents5,6 and humans.7–10 In a recent 90-day randomized, placebo-controlled clinical trial in healthy older adults (ages 60–75) who consumed 24 g d−1 freeze-dried BB, our research group reported improved executive function and memory relative to the control, when retested on days 45 and 90.8 Specifically, significant effects were observed on the Task-switching test (TST)11–13 with subjects in the BB group showing reduced switch cost compared to those in the control group.8 Significant effects were also observed on the California Verbal Learning Test (CVLT-II),14 with subjects consuming the BB showing lower repetition errors compared to the control group.8 However, the mechanism of action underlying these and other cognitive benefits of BB has not yet been identified.15
BBs have a unique polyphenol profile and are an excellent source of various anthocyanins and other phenolic compounds such as proanthocyanidins, chlorogenic acids, and flavonols.16,17 Only a few clinical trials investigating the health benefits of chronic BB supplementation have studied the polyphenol metabolites present in the plasma and urine following consumption. One such study investigated the profile of plasma polyphenol metabolites after acute and daily consumption of BB for 30 days in 18 healthy men (18–70 years).15 They concluded that BB polyphenols are extensively absorbed and metabolized by the gut microbiota and phase II enzymes, leading to an array of metabolites that may contribute to the health benefits observed following BB supplementation. However, this study measured polyphenol concentrations in the plasma and urine of individuals supplemented with BB for only 30 days. Understanding the metabolic fate of BB polyphenols in an older population for a longer duration of supplementation can help develop dietary recommendations that maximize cognitive improvement.
The present study aimed to explore changes in the concentrations of anthocyanins and phenolic acid metabolites in plasma samples that were collected from a recently published randomized, double-blind, 2-arm, parallel study that reported cognitive effects in healthy older adults supplemented with BB or control.8 Blood samples were collected after an overnight fast (∼12–15 h) and 2 h postprandially on days 0, 45, and 90. Regression analysis was performed on phenolic compounds that were significantly increased in the BB group compared to the control group and measures of cognitive function. We hypothesized that dietary supplementation with BB would increase the concentrations of circulating polyphenols in plasma of healthy older adults, and the changes would be associated with enhanced cognitive functions.
Methods
Design and procedures
Informed consent was obtained from all study participants. This study was approved by the Tufts University Institutional Review Board and registered on clinicaltrials.gov (clincaltrials.gov identifier: NCT01888848). Screened participants were enrolled in a double-blind, placebo-controlled, 2-arm, chronic feeding trial. Participants visited the USDA Jean Mayer Human Nutrition Research Center on Aging (HNRCA) at Tufts University in Boston, MA on four different occasions: a practice visit (visit 1), a baseline visit (day 0, visit 2), a mid-point visit (day 45, visit 3), and a final visit (day 90, visit 4) (Fig. 1).
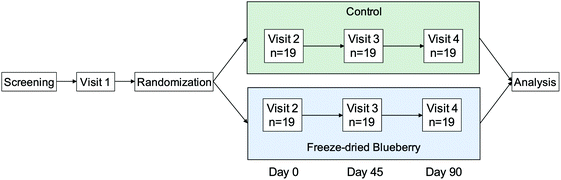 |
| Fig. 1 Study Schema. Blood samples were collected at fasting (t = 0 h) and 2 h after consuming breakfast on visits 2, 3, and 4. Breakfast included the control or blueberry drink on visits 3 and 4. | |
Visit 1 was a practice visit to familiarize study participants with the procedures of the study. Upon arrival at the HNRCA for the baseline visit (day 0, visit 2), fasting (∼12–15 h) blood samples, anthropometrics, and vital signs were collected by registered nurses. Participants then consumed a standard breakfast consisting of a corn muffin, butter, apple juice, a banana, and coffee (∼600 calories, 58 g sugar, and 21 g fat) within 15 minutes and were escorted to a testing room where experimenters administered a battery of cognitive tests and questionnaires. Two hours after consuming the breakfast, a second blood sample was collected by nurses. At the end of the visit, participants were given their first drink (BB or control) and given enough supplements to last until their midpoint visit (visit 3, day 45).
Upon arrival at the HNRCA for visit 3, participants provided a fasting blood sample before and 2 h following the completion of breakfast. This standard breakfast also included the BB or control drink according to their randomly assigned group. Upon finishing the midpoint visit's cognitive tests and questionnaires, participants were sent home with additional supplement packets to last them until their final visit. During visit 4, participants consumed their final supplement packet with breakfast following the same study procedures as visit 3. More details on the design of this clinical trial including recruitment, randomization, power calculations, and process drop out are available in our published study on the cognitive effects of BB consumption.8
Treatments
Participants in the BB study group consumed 24 g day−1 of lyophilized, cultivated BB (Tifblue variety; equivalent to 1 cup per day of BB; 12 g powder in ∼1 cup water taken with each morning and evening meal). This dose of BB contained ∼36 mg g−1 total phenolics, ∼19.2 mg g−1 anthocyanins and contributed ∼90 kcal day−1 to the diet.8 (see Table S1† for phenolic composition of the BB powder). Participants in the control group consumed 24 g of a seemingly identical, isocaloric control powder comprised of maltodextrin, fructose, artificial and natural BB flavor, artificial colors, and citric acid. Control and BB powders were provided by the US Highbush Blueberry Council. Participants were instructed to abstain from consuming berry fruit or berry containing products for the duration of the study but to otherwise maintain their usual diet.
Participants
Inclusion criteria included: men and women (60–75 years; BMI 18.5–29.9 kg m−2), nonsmoker, English fluency, >12 months postmenopausal, adequate vision for computer use, and in otherwise good health (i.e., no history of cardiovascular, metabolic, respiratory, renal, hepatic or gastrointestinal diseases). Exclusion criteria included: medications or dietary supplements that would interfere with the outcomes of the study, mini-mental status exam <24, illicit drug use, at risk for falls, impaired mobility, neurological disorders, presenting with cognitive deficits, consuming >2 alcoholic drinks per day, vegetarians or vegans, and allergies or sensitivity to berries or berry containing products.
Dietary assessment and compliance
Participants completed the National Cancer Institute's Diet History Questionnaire II (DHQ-II) during study visit 1. This questionnaire assessed participants’ diet over the previous 12 months. The DHQ-II is a comprehensive, 124-item inventory that collects information on specific foods commonly consumed in the USA. Participants also completed an additional questionnaire on overall berry consumption. Participants were contacted by telephone once a week by one of the investigators to check supplement compliance and to monitor for adverse events and changes in health history. To further track compliance, participants were instructed to record when they consumed the supplements each day. Participants also returned empty and any unused supplement packets each time they visited the center (visit 3 and 4), which were counted as an additional check on compliance.
Cognitive tests
The task-switching test (TST) was administered on days 0, 45, and 90 to assess executive function. During the test, participants viewed four intersecting lines on a computer monitor. Digits (1–4, 6–9) appeared, one at a time, in clockwise locations around the display. Participants responded by pressing one of two buttons depending on whether the number appeared in the top (odd vs. even) or bottom (>5 vs. <5) half of the display. The TST took approximately 30 minutes to complete. An abbreviated practice session was conducted during the practice visit (visit 1) to familiarize participants with the task.
The standard and alternate versions of the California Verbal Learning Test (CVLT-II) were administered, in counterbalanced order, on intervention days 0 and 90 to assess verbal learning and memory. During the CVLT-II, an experimenter read a 16-word list (list A), containing words from four semantic categories, and participants immediately recalled the list after each of 5 presentations. A 16-word distractor list (list B) was then presented for immediate recall, followed by free recall and category-cued recall of list A. Following a 20-minute delay, free recall, category-cued recall, and recognition of list A were tested. After a further 10-minute delay, forced recognition of List A was tested (see Miller and colleagues8 for a more detailed description of the cognitive tests administered in this clinical trial). The TST and CVLT-II data were previously published in Miller and colleagues.8
Sample preparation and HPLC analysis of anthocyanins and phenolic acid metabolites
Fasting and 2 h postprandial blood were collected on intervention days 0, 45, and 90 in tubes containing EDTA as an anticoagulant. Blood samples were centrifuged at 453 g for 15 minutes at 4 °C. Plasma was separated immediately from buffy coat and red blood cells after centrifugation and aliquots were stored at −80 °C for batch analysis at the end of the study. Solid-phase extraction (SPE) (Bond Elut Plexa, 200 mg, 3 mL, Agilent Technologies) was used for the extraction of anthocyanins and phenolic acid metabolites from the plasma. Briefly, plasma was thawed on ice and 400 μL of sample was diluted with 1.2 mL of acidified water (1% formic acid). Samples were loaded on the pre-conditioned cartridges under gravity. The SPE cartridges were washed with 1.5 mL of acidified water (1% formic acid). Elution of metabolites was done with 1.5 mL of acidified methanol (1% formic acid). The collected elute was dried under nitrogen at room temperature. The dried sample was dissolved in acetonitrile (5% containing 1% formic acid), and centrifuged at 18
514g for 10 min at 4 °C. Samples were transferred to amber HPLC vials and were analyzed using an Agilent 1290 Infinity ultra-high pressure liquid chromatography (UHPLC) system with an Agilent 6460 Triple Quadrupole Mass Spectrometer (Agilent Technologies, Santa Clara, CA). The system was equipped with a binary pump with an integrated vacuum degasser, an autosampler with a thermostat, and a column compartment with a thermostat. Separation of anthocyanins and metabolites was conducted using poroshell 120 stablebond C18 column (2.1 mm × 150 mm, 2.7 μm, Agilent Technologies) at a constant temperature of 35 °C. The mobile phase used for the separation of compounds consisted of acidified water (1% formic acid) and acetonitrile. The injection volume was 5 μL. The flow rate was maintained at 0.3 mL min−1 and the gradient was as follows: 5% B at 1 min, 18.5% B at 45 min, 90% B at 50 min, 5% B at 52 min and 8 min for post run. Agilent Pursuit 3 PFP column (150 × 2.0 mm) with guard column (Pursuit 3 PFP MetaGurad 10 × 2.0 mm) was used for phenolic acids analysis. The column temperature was set at 40 °C and the mobile phase used was acidified water (0.1% formic acid) and acidified acetonitrile (0.1% formic acid). The solvent gradient was 5% B at 1 min, 10% B at 10 min, 15% B at 3 min, 15% B at 7 min, 20% B at 9 min, 20% B at 10 min, 25% B at 11 min, 30% B at 13 min, 30% B at 14 min, 95% B at 15 min, 5% B at 16 min and 4 min for post run. Injection volume was 5 μL. For quantification of compounds, standards were prepared in blank plasma (charcoal stripped human plasma obtained from BioreclamationIVT, NY) for matrix match. Authentic standards were used for quantification when available and metabolites were quantified using parent compounds or compounds sharing similar chemical structure or molecular weight.
Statistical analyses
All statistical analyses were performed using SYSTAT software (SPSS, Inc, Chicago, IL). DHQ-II and demographic data were analyzed by Student's t-test. Phenolic acid and anthocyanin metabolites were analyzed by two-way analysis of variance (ANOVA) followed by post hoc testing with Fisher's LSD to determine differences between the groups. Data from the fasting and postprandial timepoints were analyzed separately. A forward stepwise regression was performed on the change (day 90–day 0) in postprandial levels of polyphenols with significant treatment by visit interactions from ANOVA and the change (day 90–day 0) in task switching errors on the TST and repetition errors on the CVLT-II. Switching errors and repetition errors were chosen as dependent variables in each analysis because they were significantly lower in the BB group compared to the control group.8 Results were considered statistically significant if the observed significance level was p < 0.05.
Results
Subject demographics and compliance
The final analysis consisted of 38 participants of which 19 consumed the control powder and 19 consumed the BB powder. The average age and BMI of the groups are shown in Table 1. Age and BMI were not significantly different between the BB and control groups (p > 0.05; Table 1). There was no difference in the number of missed supplement packets between the two groups (p > 0.05; Table 1).
Table 1 Participant demographics and compliance
|
Control |
Blueberry |
Values presented as mean ± standard deviation. |
Number of participants (N) |
19 |
19 |
Women |
63% |
72% |
Age (years) |
67.3 ± 4.8 |
67.8 ± 4.6 |
Baseline BMI (kg m−2) |
24.0 ± 2.5 |
24.1 ± 3.7 |
Compliance |
99.2% |
99.2% |
Dietary assessment
Analysis of DHQ-II data at baseline revealed no significant differences between the control and BB groups for usual intake of key nutrients and food groups, however participants in the BB group reported consumption of significantly more tomatoes (0.311 vs. 0.187 estimated cups per day, p < 0.05) and eggs (11.25 vs. 4.48 grams day−1, p < 0.05) compared to those in the control group. Participants assigned to the control group reported consumption of significantly more of the sweetener xylitol (0.033 vs. 0.021 grams day−1, p < 0.05). The control and BB groups did not significantly differ in the frequency of berry fruit intake in general (p > 0.05) or intake of BB specifically (p > 0.05).
Phenolic acid metabolites
Fifteen phenolic acids and their conjugated metabolites were quantified in fasting (0 h) and postprandial (2 h) plasma of participants on the control and BB supplements on days 0, 45, and 90 (Table 2). Among all the phenolic acids quantified, hippuric acid was present in the highest concentration in fasting plasma of the BB group on day 45 (80.8 ± 8.4 μmol L−1vs. 12.6 ± 3.0 μmol L−1 in the control group). Significant intervention group (control and BB) by day (0, 45, and 90) interactions were observed for hippuric acid (F (2, 72) = 21.81, p < 0.001; Fig. 2A), syringic acid (F (2, 72) = 16.42, p < 0.001; Fig. 2B), ferulic acid-glucuronide (F (2, 72) = 4.04, p < 0.05; Fig. 2C), and phloroglucinaldehyde (F (2, 72) = 10.05, p < 0.001; Fig. 2D) at the postprandial timepoint. Interestingly, hippuric acid was the only phenolic acid that was significantly altered at the fasting timepoint (F (2, 72) = 25.47, p < 0.001; Fig. 2A). At the postprandial timepoint, a significant intervention group effect was observed for hippuric acid (F (1, 36) = 33.90, p < 0.001; Table 2), isovanillic acid (F (1, 36) = 6.42, p < 0.05; Table 2), phloroglucinaldehyde (F (1, 36) = 40.57, p < 0.001; Table 2), syringic acid (F (1, 36) = 33.81, p < 0.001; Table 2), and trans-cinnamic acid (F (1, 36) = 7.15, p < 0.05; Table 2). At the fasting time-point, a significant intervention group effect was observed for hippuric acid only (F (1, 36) = 26.24, p < 0.001; Table 2).
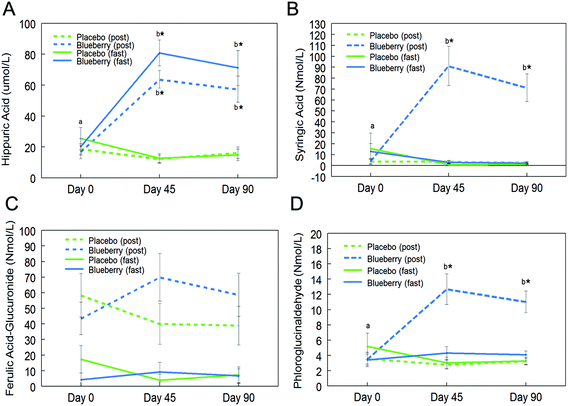 |
| Fig. 2 Circulating phenolic acid concentrations in plasma of subjects consuming blueberry (BB) or control at fasting (fast) and postprandial (post) timepoints. Two-way analysis of variance showed that BB significantly altered plasma concentrations of hippuric acid (A), syringic acid (B), ferulic acid-glucuronide (C), and phloroglucinaldehyde (D) at the postprandial time-point compared to control. Hippuric acid was the only phenolic compound that was significantly altered by BB at the fasting time-point. Data are represented as mean ± SEM. Asterisk (*) denotes significant post hoc differences between treatments at fasting or postprandial timepoints (p < 0.05). Different letters denote significant post hoc differences within groups (p < 0.05). | |
Table 2 Plasma phenolic acid concentrations (nmol L−1) at fasting (t = 0 h) and 2 h after consuming the breakfast at days 0, 45, and 90
|
Plasma concentrations (nmol L−1) |
Fasting (0 h) |
Postprandial (2 h) |
|
Treatment |
Day 0 |
Day 45 |
Day 90 |
Day 0 |
Day 45 |
Day 90 |
Values are presented as mean ± standard error of the mean. Significant intervention group (control and blueberry) by day (0, 45 and 90) interactions were observed at the †fasting and *postprandial timepoints (p ≤ 0.05). Significant intervention group effects were observed at the ^fasting and #postprandial timepoints (p ≤ 0.05). Sample sizes: control N = 19 and BB N = 19. LOQ and LOD for these metabolites are found in Table S2.† |
Phenolic acids
|
3,4-Dihydroxybenzoic acid |
Control |
37.4 ± 11.5 |
134.6 ± 110.3 |
23.9 ± 5.2 |
26.6 ± 5.3 |
24.9 ± 4.3 |
22.7 ± 4 |
Blueberry |
33.1 ± 8 |
31.1 ± 6.5 |
32.7 ± 4.3 |
27.1 ± 5.1 |
36.7 ± 6.3 |
36.6 ± 4.6 |
4-Hydroxybenzaldehyde |
Control |
242.7 ± 29.1 |
256.9 ± 34.3 |
186.5 ± 19 |
242.3 ± 33.4 |
259 ± 27 |
220.6 ± 24.9 |
Blueberry |
216.2 ± 27.5 |
248.4 ± 33.8 |
171.1 ± 22.8 |
246.3 ± 34.6 |
265.4 ± 38 |
209.5 ± 37.8 |
4-Hydroxyphenyacetic acid |
Control |
619.3 ± 144 |
434.5 ± 121.6 |
435.2 ± 105 |
639.5 ± 107.1 |
551.6 ± 105.9 |
528.3 ± 128.3 |
Blueberry |
564.1 ± 108.6 |
574.3 ± 110.7 |
474.3 ± 88.3 |
678.9 ± 109.2 |
1205.9 ± 515.5 |
500.4 ± 76.7 |
Ferulic acid |
Control |
8 ± 5.1 |
1.1 ± 1.1 |
2.4 ± 1.7 |
26 ± 2.9 |
26.6 ± 4.3 |
26.7 ± 4 |
Blueberry |
1.6 ± 1.6 |
3.5 ± 2.6 |
2.4 ± 1.7 |
27.9 ± 4.7 |
36.2 ± 5 |
33.7 ± 5.4 |
Hippuric acid (μmol L−1)†,*,^,# |
Control |
25.4 ± 7.2 |
12.6 ± 3 |
14.9 ± 3.7 |
18.8 ± 3.3 |
12.2 ± 2.5 |
16.3 ± 3.8 |
Blueberry |
20.2 ± 5.4 |
80.8 ± 8.4 |
71.1 ± 11.3 |
16.7 ± 4.3 |
63.8 ± 5.7 |
57.4 ± 8.5 |
Isovanillic acid# |
Control |
31.9 ± 22.5 |
6.2 ± 3 |
11.5 ± 4.1 |
114 ± 8.6 |
113.9 ± 11.7 |
106.9 ± 8.4 |
Blueberry |
13.6 ± 3.9 |
24.4 ± 4.6 |
20.2 ± 3.8 |
136.2 ± 11.9 |
151.7 ± 16.1 |
162.7 ± 17.5 |
p-Coumaric acid |
Control |
3.2 ± 1.3 |
2.3 ± 0.7 |
1.9 ± 0.6 |
4.5 ± 0.8 |
7.6 ± 1.5 |
8 ± 1.3 |
Blueberry |
0.9 ± 0.4 |
1.8 ± 0.8 |
1.7 ± 0.5 |
3.5 ± 0.7 |
7.4 ± 1.6 |
8.3 ± 2.2 |
Phloroglucinaldehyde*,# |
Control |
5.2 ± 1.7 |
3 ± 0.7 |
3.3 ± 0.5 |
3.6 ± 0.7 |
2.8 ± 0.6 |
3.3 ± 0.5 |
Blueberry |
3.4 ± 0.7 |
4.3 ± 0.8 |
4.1 ± 0.5 |
3.5 ± 1 |
12.7 ± 2 |
11.1 ± 1.4 |
Syringic acid*,# |
Control |
15.7 ± 14 |
0.9 ± 0.9 |
1.1 ± 0.6 |
3.6 ± 1.9 |
3.5 ± 1.4 |
2.7 ± 0.9 |
Blueberry |
13.1 ± 7 |
3 ± 1.4 |
2.3 ± 0.9 |
3.9 ± 2.6 |
91.3 ± 18.1 |
71.3 ± 12.6 |
Trans-cinnamic acid# |
Control |
13.4 ± 5.2 |
3.7 ± 1.8 |
2.6 ± 1.4 |
24.9 ± 2.8 |
28.3 ± 3.4 |
24.5 ± 2.9 |
Blueberry |
14.5 ± 2.3 |
15.2 ± 3 |
193.4 ± 175.1 |
33.4 ± 2.6 |
36 ± 6 |
40.6 ± 5.1 |
Vanillic acid |
Control |
52 ± 52 |
0 ± 0 |
0 ± 0 |
324.3 ± 27.3 |
335.7 ± 35.6 |
347.6 ± 34.6 |
Blueberry |
0 ± 0 |
0 ± 0 |
0 ± 0 |
323.5 ± 34 |
444 ± 51.9 |
437 ± 53.6 |
Vanillic acid-glucuronide |
Control |
545.7 ± 346.6 |
183.5 ± 77.4 |
201 ± 49.6 |
2685.2 ± 279.4 |
2692.9 ± 326.7 |
2980.9 ± 334.8 |
Blueberry |
90.7 ± 31.9 |
416.5 ± 107.5 |
256.3 ± 70.1 |
2663.9 ± 258.2 |
3196.4 ± 369.4 |
3129.7 ± 361.8 |
Isovanillic acid-glucuronide |
Control |
126.9 ± 89.5 |
18.3 ± 10.2 |
27.9 ± 9.8 |
591.3 ± 64.8 |
515.8 ± 70.1 |
446.2 ± 44.3 |
Blueberry |
28.6 ± 10.8 |
54.9 ± 19.1 |
24 ± 11.1 |
683.2 ± 66.4 |
646.2 ± 59.9 |
603 ± 49.5 |
Ferulic acid-glucuronide* |
Control |
17.4 ± 8.8 |
3.9 ± 3.9 |
7.3 ± 5.1 |
58.4 ± 13.9 |
40 ± 13.1 |
38.9 ± 12.4 |
Blueberry |
4.3 ± 4.3 |
9.1 ± 6.3 |
6.7 ± 4.6 |
43.6 ± 10.4 |
70 ± 15.3 |
58.7 ± 13.8 |
3-CGA |
Control |
5 ± 2 |
2.3 ± 1.3 |
3.2 ± 2.5 |
34.8 ± 10.9 |
74.7 ± 45.5 |
42.8 ± 16.1 |
Blueberry |
7.4 ± 2.9 |
4.7 ± 3.8 |
4.4 ± 3.2 |
70 ± 19.5 |
64.5 ± 8.5 |
64.5 ± 13.5 |
Anthocyanins
|
Cyanidin-3-O-arabinoside# |
Control |
0 ± 0 |
0 ± 0 |
0 ± 0 |
0.5 ± 0.5 |
0 ± 0 |
0 ± 0 |
Blueberry |
0 ± 0 |
0.7 ± 0.7 |
0 ± 0 |
0.9 ± 0.9 |
3.5 ± 1.9 |
2.5 ± 0.7 |
Cyanidin-3-O-galactoside*,# |
Control |
0 ± 0 |
0 ± 0 |
0 ± 0 |
0.8 ± 0.8 |
0 ± 0 |
0.7 ± 0.7 |
Blueberry |
0 ± 0 |
2.4 ± 1.6 |
0.5 ± 0.4 |
1.8 ± 1.8 |
18.5 ± 6.2 |
11.2 ± 2.3 |
Cyanidin-3-O-glucoside*,# |
Control |
0 ± 0 |
0 ± 0 |
0 ± 0 |
0.4 ± 0.4 |
0 ± 0 |
0 ± 0 |
Blueberry |
0 ± 0 |
1 ± 1 |
0.3 ± 0.3 |
0 ± 0 |
4.2 ± 2.2 |
2.5 ± 0.9 |
Delphinidin-3-O-galactoside |
Control |
0 ± 0 |
0 ± 0 |
0 ± 0 |
1.3 ± 1.3 |
0 ± 0 |
0 ± 0 |
Blueberry |
0 ± 0 |
0 ± 0 |
0 ± 0 |
0 ± 0 |
3 ± 2 |
2.2 ± 0.9 |
Delphinidin-3-O-glucoside |
Control |
0 ± 0 |
0 ± 0 |
0 ± 0 |
0.7 ± 0.7 |
0 ± 0 |
0 ± 0 |
Blueberry |
0 ± 0 |
0 ± 0 |
0 ± 0 |
0 ± 0 |
1.5 ± 0.9 |
1.4 ± 0.8 |
Malvidin-3-O-arabinoside# |
Control |
0 ± 0 |
0 ± 0 |
0 ± 0 |
2 ± 2 |
0 ± 0 |
0 ± 0 |
Blueberry |
0 ± 0 |
1.4 ± 1.4 |
0 ± 0 |
1.9 ± 1.9 |
9.9 ± 7.6 |
7.8 ± 2.7 |
Malvidin-3-O-galactoside*,# |
Control |
0 ± 0 |
0 ± 0 |
0 ± 0 |
2.7 ± 2.7 |
0 ± 0 |
0 ± 0 |
Blueberry |
0 ± 0 |
1.3 ± 1.3 |
0.5 ± 0.4 |
2.8 ± 2.8 |
29.5 ± 8.6 |
18 ± 4.5 |
Malvidin-3-O-glucoside*,# |
Control |
0 ± 0 |
0 ± 0 |
0 ± 0 |
1.7 ± 1.7 |
0 ± 0 |
0 ± 0 |
Blueberry |
0 ± 0 |
2.3 ± 2.3 |
0 ± 0 |
2 ± 2 |
20.8 ± 8.3 |
12.4 ± 3.5 |
Peonidin-3-O-arabinoside |
Control |
0 ± 0 |
0 ± 0 |
0 ± 0 |
0 ± 0 |
0 ± 0 |
0 ± 0 |
Blueberry |
0 ± 0 |
0.7 ± 0.7 |
0 ± 0 |
0 ± 0 |
1.5 ± 1.5 |
0 ± 0 |
Peonidin-3-O-galactoside# |
Control |
0 ± 0 |
0 ± 0 |
0 ± 0 |
0.4 ± 0.4 |
0 ± 0 |
0 ± 0 |
Blueberry |
0 ± 0 |
1.1 ± 1.1 |
0 ± 0 |
1.3 ± 1.3 |
3.6 ± 3.1 |
2.9 ± 2 |
Peonidin-3-O-glucoside# |
Control |
0 ± 0 |
0 ± 0 |
0 ± 0 |
0.5 ± 0.5 |
0 ± 0 |
0 ± 0 |
Blueberry |
0 ± 0 |
0 ± 0 |
0 ± 0 |
1.3 ± 1.3 |
3.7 ± 2.4 |
3.3 ± 1 |
Peonidin-3-O-xyloside*,# |
Control |
0 ± 0 |
0 ± 0 |
0 ± 0 |
0.5 ± 0.5 |
0 ± 0 |
0 ± 0 |
Blueberry |
0 ± 0 |
0 ± 0 |
0 ± 0 |
0 ± 0 |
1.1 ± 0.5 |
1.9 ± 0.5 |
Peonidin glucuronide*,# |
Control |
1.9 ± 1.9 |
0.7 ± 0.7 |
0 ± 0 |
15.4 ± 15.4 |
0 ± 0 |
0 ± 0 |
Blueberry |
0 ± 0 |
1.8 ± 1.8 |
0.2 ± 0.2 |
0 ± 0 |
150.7 ± 67.2 |
41 ± 18.1 |
Petunidin-3-O-galactoside# |
Control |
0 ± 0 |
0 ± 0 |
0 ± 0 |
1 ± 1 |
0 ± 0 |
0 ± 0 |
Blueberry |
0 ± 0 |
0.8 ± 0.8 |
0 ± 0 |
1.3 ± 1.3 |
3.4 ± 1.9 |
4.4 ± 1.2 |
Petunidin-3-O-glucoside*,# |
Control |
0 ± 0 |
0 ± 0 |
0 ± 0 |
0.7 ± 0.7 |
0 ± 0 |
0 ± 0 |
Blueberry |
0 ± 0 |
0 ± 0 |
0 ± 0 |
0 ± 0 |
2.6 ± 1.2 |
1.4 ± 0.8 |
Further post hoc comparisons showed significantly higher concentrations of hippuric acid, syringic acid, and phloroglucinaldehyde on days 45 and 90 in the BB group compared to the control group at the postprandial timepoint (p < 0.05; Fig. 2). Furthermore, the BB group had significantly higher concentrations of these phenolic acids at the postprandial timepoint on days 45 and 90 compared to baseline (day 0) (p < 0.05; Fig. 2). Hippuric acid was also significantly increased at the fasting timepoint on days 45 and 90 compared to baseline (day 0) and the control group (p < 0.05; Fig. 2A). Interestingly, concentrations of these phenolics did not significantly differ between days 45 and 90 (p > 0.05; Fig. 2). No significant differences were observed for ferulic acid-glucuronide at the fasting and postprandial timepoints (p > 0.05; Fig. 2C).
Anthocyanins and their conjugated metabolites
Fifteen anthocyanins and their conjugated metabolites were quantified in fasting (0 h) and postprandial (2 h) plasma of participants on the control and BB supplements on days 0, 45, and 90 (Table 2). Anthocyanins and their metabolites were not significantly detected in plasma samples from the control group or in samples collected at the baseline visit (day 0). Peonidin glucuronide, a conjugated metabolite of peonidin, reached 150.7 ± 67.2 nmol L−1 at 2 h on day 45 in the BB group, which was the highest concentration among all phase II metabolites maintaining the parent structure. Among the parent untransformed anthocyanins, the content of malvidin-3-O-galactoside was highest (29.5 ± 8.6 nmol L−1) in 2 h plasma sample on day 45 in the BB group. Significant intervention group (control and BB) by day (0, 45, and 90) interactions were observed for cyanidin-3-O-galactoside (F (2, 72) = 5.34, p < 0.01; Fig. 3A), cyanidin-3-O-glucoside (F (2, 72) = 3.11, p = 0.051 marginal; Fig. 3B), malvidin-3-O-galactoside (F (2, 72) = 8.40, p < 0.01; Fig. 3C), malvidin-3-O-glucoside (F (2, 72) = 4.23, p < 0.05; Fig. 3D), petunidin-3-O-glucoside (F (2, 72) = 3.18, p < 0.05; Fig. 4A), peonidin glucuronide (F (2, 72) = 5.33, p < 0.01; Fig. 4B), and peonidin-3-O-xyloside (F (2, 72) = 7.65, p < 0.01; Fig. 4C) at the postprandial timepoint only. A significant intervention group effect was observed for all of the anthocyanin/metabolites studied (p ≤ 0.05) except delphinidin-3-O-galactoside, delphinidin-3-O-glucoside and peonidin-3-O-arabonisde (p > 0.05; Table 2). No significant differences were observed at the fasting timepoint (p > 0.05; Table 2).
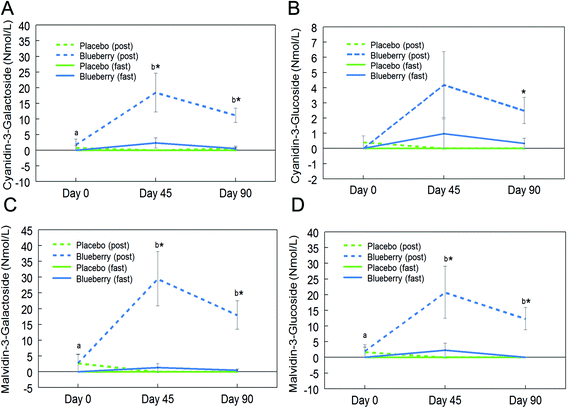 |
| Fig. 3 Circulating anthocyanin concentrations in the plasma of subjects consuming blueberry (BB) or control at fasting (fast) and postprandial (post) timepoints. Two-way analysis of variance showed that BB significantly altered plasma concentrations of cyanidin-3-O-galactoside (A), cyanidin-3-O-glucoside (B), malvidin-3-O-galactoside (C), and malvidin-3-O-glucoside (D) at the postprandial time-point only. Data are represented as mean ± SEM. Asterisk (*) denotes significant post hoc differences between treatments at fasting or postprandial timepoints (p < 0.05). Different letters denote significant post hoc differences within groups (p < 0.05). | |
 |
| Fig. 4 Circulating anthocyanin concentrations in subjects consuming blueberry (BB) or control at fasting (fast) and postprandial (post) timepoints. Two-way analysis of variance showed that BB significantly altered plasma concentrations of petunidin-3-O-glucoside (A), petunidin glucuronide (B), and peonidin-3-O-xyloside (C) at the postprandial timepoint only. Data are represented as mean ± SEM. Asterisk (*) denotes significant post hoc differences between treatments at fasting or postprandial timepoints (p < 0.05). Different letters denote significant post hoc differences within groups (p < 0.05). | |
Further post hoc comparisons of postprandial samples from the BB group showed significantly higher concentrations of cyanidin-3-O-galactoside (day 45 and 90), cyanidin-3-O-glucoside (day 90), malvidin-3-O-galactoside (day 45 and 90), malvidin-3-O-glucoside (day 45 and 90), petunidin-3-O-glucoside (day 45), peonidin glucuronide (day 45 and 90), and peonidin-3-O-xyloside (day 45 and 90) compared to the control group (p < 0.05; Fig. 3 and 4). As observed with the phenolic acids, the concentrations of these anthocyanins did not significantly differ between days 45 and 90 (p > 0.05; Fig. 3 and 4), with the exception of peonidin glucuronide whose concentration was significantly lower at day 90 compared to day 45, however still significantly higher than baseline (p < 0.05; Fig. 4B).
Regression analysis
Overall change (day 90–day 0) in the eleven phenolic acids and anthocyanins which were enhanced in postprandial blood of BB group and change (day 90–day 0) in repetition errors in the CVLT-II or switching errors in the TST were entered into a forward stepwise regression. Change in postprandial plasma levels of ferulic acid-glucuronide, syringic acid, and malvidin-3-O-galactoside was associated with change in task switching errors (R2 = 0.521, p < 0.05; Fig. 5A–C). Change in ferulic acid-glucuronide was negatively associated with change in switching errors (β = −0.150, p < 0.01; Fig. 5A). Change in syringic acid was negatively associated with change in switching errors, however this was not significant (β = −0.098, p > 0.05; Fig. 5B). Interestingly, change in malvidin-3-O-galactoside was positively associated with change in switching errors (β = 0.535, p < 0.05; Fig. 5C).
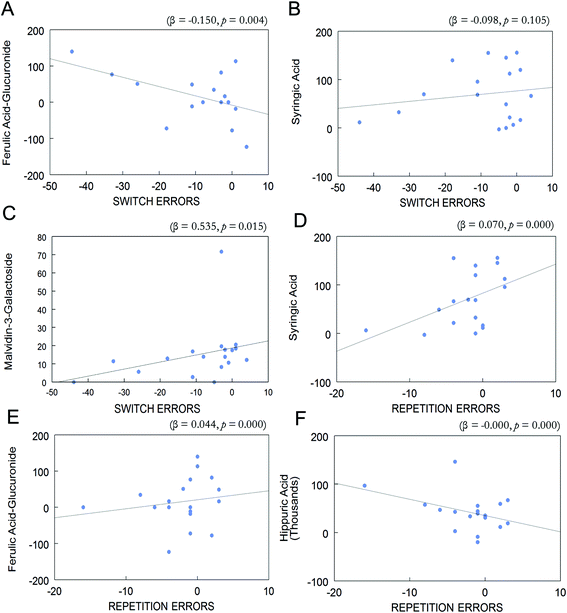 |
| Fig. 5 Overall change (Day 90 – Day 0) in postprandial plasma phenolic levels vs. change (Day 90 – Day 0) in switch errors in the TST and repetition errors in the CVLT-II in subjects consuming BB. Change in (A) ferulic acid-glucuronide (A), syringic acid (B), and malvidin-3-O-galactoside (C) was significantly associated with a change in task switching errors (R2 = 0.521, p < 0.05). Change in syringic acid (D), ferulic acid-glucuronide (E), and hippuric acid (F) was associated with a change in CVLT-II repetition errors (R2 = 0.807, p < 0.001). | |
Change (day 90–day 0) in postprandial plasma levels of ferulic acid glucuronide, syringic acid, and hippuric acid was associated with change (day 90–day 0) in CVLT-II repetition errors (R2 = 0.807, p < 0.001; Fig. 5D–F). Change in concentration of syringic acid was also positively associated with change in repetition errors (β = 0.070, p < 0.001; Fig. 5D). Change in concentration of ferulic acid-glucuronide was positively associated with change in repetition errors (β = 0.044, p < 0.001; Fig. 5E). Lastly, change in hippuric acid was negatively associated with change in repetition errors (β < −0.001, p < 0.001; Fig. 5F).
Discussion
Prior to the current study, characterization of phenolic compound profiles in plasma after BB supplementation had been performed up to 6 h after acute consumption,18,19 up to 24 h after acute consumption,20 and up to 2 h after chronic consumption of BB for 30 days.15 These studies demonstrated that BB metabolites peak at different times during a 24 h period post-consumption and some metabolites exhibit biphasic patterns.20 Furthermore, chronic consumption of BB leads to the retention and persistence of some phenolic acid compounds over a longer period than is observed in acute studies.15 The purpose of this study was to quantify plasma phenolic acids and anthocyanin concentrations in the plasma of healthy older adults supplemented with BB or control over 90 days. To our knowledge, this is the first study to characterize BB polyphenol profiles after 90 days of chronic feeding in older adults. In addition, this is the first study to correlate plasma phenolics with measures of cognitive function to further study the possible mechanisms of action underlying the cognitive benefits observed with chronic BB intake.8,10,21,22 Plasma phenolic acid and anthocyanin concentrations were significantly altered after 90 days of BB consumption compared to the control, and concentrations of these plasma phenolics correlated with improvements in cognition.
Anthocyanins were not significantly detected in fasting or postprandial samples from the control group on days 0, 45, and 90 or on day 0 of samples from the BB group ruling out the possibility that anthocyanins were present in blood circulation before participants started the study. A significant group by day interaction was observed for seven anthocyanins (cyanidin-3-O-galactoside, cyanidin-3-O-glucoside, malvidin-3-O-galactoside, malvidin-3-O-glucoside, peonidin-3-O-xyloside, peonidin glucuronide, and petunidin-3-O-glucoside) in 2 h postprandial samples. All anthocyanins quantified, except for delphinidin-3-O-galactoside, delphinidin-3-O-glucoside, and peonidin-3-O-arabinoside were significantly elevated in 2 h postprandial, but not fasting, samples from the BB group compared to the control (Table 2). These findings are in line with previous research showing that anthocyanin concentrations peak around 2 h following acute consumption of BB and decline back to baseline by 24 h.20 Furthermore, an additional 45 days of supplementation did not further increase the concentrations of anthocyanins in the plasma as was observed in a previous chronic strawberry supplementation study, as there was no difference in anthocyanin levels from day 45 to day 90.23
Anthocyanins were not significantly detected in fasting (∼12–15 h) plasma of subjects on the BB supplement on days 45 and 90. However, this finding does not preclude the possibility that anthocyanins accumulated in tissues, including the brain, following chronic consumption of BB. Anthocyanins, including those found in BB, have a high affinity for animal tissues,24 and it has been demonstrated that anthocyanins can cross the blood–brain barrier.3 In a study with pigs, anthocyanins were absent from fasting plasma and urine after chronic consumption of a BB-enriched diet for 4 weeks, however anthocyanins were found in tissues from the eye, cortex, and cerebellum.25 Andres-Lacueva and colleagues3 fed BB to rats for 70 days and detected anthocyanins in various regions of the brain, particularly the cortex. Zhong et al.20 found that by 24 h post-consumption of a BB beverage, no anthocyanins were detected in subjects’ plasma. However, this was an acute study and plasma anthocyanin levels were not measured during 10–24 h post-consumption. In an acute pharmacokinetic study conducted with strawberries, anthocyanins peaked 1–3 h post-consumption and were back to baseline concentrations 8–10 h post-consumption.26 In a previous study from our lab, subjects consumed 24 g d−1 of freeze-dried strawberry (equivalent to 2 cups of fresh strawberries) and anthocyanins were measured at fasting and postprandial timepoints on days 0, 45 and 90.23 Three anthocyanins/metabolites (pelargonidin glucuronide, pelargonidin-3-rutinoside, and pelargonidin-2-glucoside) were detected in fasting and 2 h plasma on days 45 and 90 suggesting that anthocyanins persisted in the blood of subjects longer than what was found in previous acute studies.26,27 However, previous studies have shown that blueberries do not contain pelargonidin,28 and pelargonidin-based metabolites were not quantified in the current study.
We found that a change (day 90–day 0) in plasma concentrations of one anthocyanin (malvidin-3-O-galactoside) at the postprandial timepoint was positively correlated with the change (day 90–day 0) in switch errors on the TST. In other words, individuals with greater improvements on the TST had smaller increases in plasma concentrations of malvidin-3-O-galactoside. In a previous study performed in rats supplemented with BB, malvidin-3-O-galctoside was the most prevalent anthocyanin in the cortex, and correlational analysis found a positive relationship between Morris water maze performance and the total number of anthocyanins found in the cortex.3 It is possible that less malvidin-3-O-galactoside was found in the plasma as it had been absorbed by the tissue. However, more work must be completed to further understand the associations between anthocyanins and cognitive performance in humans and other model organisms.
Phenolic acid derivatives were present in plasma at much higher concentrations than parent anthocyanins. Research suggests that phenolic acid metabolites, derived from the metabolism of anthocyanins, are the major compounds circulating in urine and blood after consumption of anthocyanin-rich foods.29,30 Anthocyanins can be absorbed from the gastrointestinal lumen and undergo presystemic metabolism in the intestinal wall or they can be metabolized to various phenolic acids by bacteria in the colon.29,30 A significant increase in four phenolic acids (hippuric acid, syringic acid, ferulic acid-glucuronide, and phloroglucinaldehyde) was observed in postprandial samples after BB consumption for 90 days, compared to the control. Peak levels of these compounds occurred on day 45 in the BB group, and an additional 45 days of supplementation did not further increase the concentrations of these compounds in the plasma.
Ferulic acid-glucuronide was elevated at baseline (day 0) in both BB and control subjects at the postprandial timepoint. This result was not surprising considering that the consumption of breakfast cereals and other foods can significantly increase ferulic acid concentrations in the blood.31 Contrary to other BB supplementation studies,15,20post-hoc analysis did not show any significant differences in ferulic acid-glucuronide levels between the BB and control groups at day 45 and 90. On the other hand, syringic acid and phloroglucinaldehyde were significantly elevated in individuals from the BB group compared to the control at the postprandial timepoint only (day 45 and 90). When analyzing the associations between phenolic acid concentrations and measures of cognition, we found that a change in ferulic acid-glucuronide in the BB group at the postprandial timepoint was positively correlated with a change in switch errors on the TST but negatively correlated with a change in repetition errors on the CVLT-II. Syringic acid was positively correlated with a change in repetition errors only. These conflicting results could be partly due to small sample size; however, it is clear that more studies are needed to further understand the relationship between the bio- and neuro-availability of phenolic acids and the mechanisms by which they directly or indirectly affect cognition and overall brain health in humans.
Lastly, hippuric acid was present in the highest concentration in plasma samples of the BB group as has been shown in previous BB supplementation15,18,19,32 and strawberry supplementation studies.23 Furthermore, hippuric acid was the only phenolic acid that increased in concentration in the BB group, compared to the control, at the fasting timepoint. This result is consistent with results from previous berry intervention studies.33,34 Zhong and collegues20 characterized BB polyphenols in plasma of subjects over a 24 h period after acute consumption of BB and found hippuric acid increased significantly after 6 h and peaked 24 h post-consumption. Hippuric acid is a metabolite of many polyphenols, including flavanols and anthocyanidins,33,35 and it is also generated from protein and amino acid metabolism,36 thus explaining the elevated levels at baseline (day 0) in the BB and control group. In addition, hippuric acid was present in the highest concentration compared to the other phenolic acids quantified. Feliciano and colleagues15 found hippuric acid to be the biggest contributor to the polyphenol pool of metabolites in circulation (86%) following BB supplementation for one month. Evidence for higher levels of hippuric acid in postprandial blood samples of subjects supplemented with BB compared to the control could substantiate, in part, improvements in cognitive function observed in BB supplementation studies. In this study, we found that the change (day 90–day 0) in hippuric acid levels in the BB group at the postprandial timepoint was inversely correlated with the change in repetition errors on the CVLT-II, suggesting that hippuric acid may have a beneficial effect on cognitive performance.
This study had both strengths and limitations. First, plasma samples were collected at only two timepoints after chronic supplementation. Collecting blood samples at more timepoints postprandially would have allowed a more complete pharmacokinetic analysis of BB polyphenol metabolism. Second, urine analysis of polyphenol metabolites was not performed in this study and would have allowed for total polyphenol absorption to be more accurately assessed. Third, a larger sample size would make regression analyses more robust. Strengths included the use of a parallel design making it possible to minimize issues with carryover; however, a cross-over design would have made it easier to compare treatment effects within subjects. In addition, this study included analysis of a wide array of phenolic acid and anthocyanin metabolites following blueberry consumption over 90 days.
Future work is needed to further study the possible mechanisms underlying the cognitive benefits observed in humans and animal models following chronic BB consumption. This work should include blood analyses of growth factors such as insulin-like growth factor 1 (IGF-1) and brain-derived neurotrophic factor (BDNF). Lower serum IGF-1 levels have been associated with cognitive impairments in humans.37,38 Lower serum BDNF is associated with lower cognitive test scores and mild cognitive impairment (MCI).39 Overall, our results suggest that BB anthocyanins are absorbed and extensively metabolized/catabolized resulting in the production of various phenolic acid derivatives and their conjugates, all together contributing to the bioavailability and beneficial effects associated with BB consumption. In conclusion, cognitive improvements are related to changing levels of circulating phenolics.
Conflicts of interest
There are no conflicts of interest to declare.
Acknowledgements
This research was funded by USDA intramural funds and agreements between the USDA and US Highbush Blueberry Council. Freeze-dried blueberry and control powders were provided by the US Highbush Blueberry Council. This clinical trial was conducted at the USDA Human Nutrition Research Center on Aging at Tufts University. HPLC analysis of anthocyanins and phenolic acid metabolites was conducted at the Illinois Institute of Technology. Marshall Miller's present affiliation is the Center for the Study of Aging at Duke University Medical Center, Durham, NC. Marshall Miller is currently supported by NIH T32 AG00002941.
References
-
E. L. Glisky, in Brain Aging: Models, Methods, and Mechanisms, ed. D. R. Riddle, CRC Press/Taylor & Francis Group, Boca Raton, 2007 Search PubMed.
- M. G. Miller, N. Thangthaeng, S. M. Poulose and B. Shukitt-Hale, Role of fruits, nuts, and vegetables in maintaining cognitive health, Exp. Gerontol., 2017, 94, 24–28 CrossRef CAS PubMed.
- C. Andres-Lacueva, B. Shukitt-Hale, R. L. Galli, O. Jauregui, R. M. Lamuela-Raventos and J. A. Joseph, Anthocyanins in aged blueberry-fed rats are found centrally and may enhance memory, Nutr. Neurosci., 2005, 8, 111–120 CrossRef CAS PubMed.
-
M. Giacalone, F. Di Sacco, I. Traupe, N. Pagnucci, F. Forfori and F. Giunta, in Bioactive Nutraceuticals and Dietary Supplements in Neurological and Brain Disease, ed. R. R. Watson and V. R. Preedy, Academic Press, San Diego, 2015 Search PubMed.
- B. Shukitt-Hale, D. F. Bielinski, F. C. Lau, L. M. Willis, A. N. Carey and J. A. Joseph, The beneficial effects of berries on cognition, motor behaviour and neuronal function in ageing, Br. J. Nutr., 2015, 114, 1542–1549 CrossRef CAS PubMed.
- A. N. Carey, S. M. Gomes and B. Shukitt-Hale, Blueberry supplementation improves memory in middle-aged mice fed a high-fat diet, J. Agric. Food Chem., 2014, 62, 3972–3978 CrossRef CAS PubMed.
- S. Hein, A. R. Whyte, E. Wood, A. Rodriguez-Mateos and C. M. Williams, Systematic review of the effects of blueberry on cognitive performance as we age, J. Gerontol., 2019, 74, 984–995 CrossRef CAS PubMed.
- M. G. Miller, D. A. Hamilton, J. A. Joseph and B. Shukitt-Hale, Dietary blueberry improves cognition among older adults in a randomized, double-blind, placebo-controlled trial, Eur. J. Nutr., 2018, 57, 1169–1180 CrossRef CAS PubMed.
- A. R. Whyte and C. M. Williams, Effects of a single dose of a flavonoid-rich blueberry drink on memory in 8 to 10 y old children, Nutr. J., 2015, 31, 531–534 CrossRef CAS PubMed.
- R. Krikorian, M. D. Shidler, T. A. Nash, W. Kalt, M. R. Vinqvist-Tymchuk, B. Shukitt-Hale and J. A. Joseph, Blueberry supplementation improves memory in older adults, J. Agric. Food Chem., 2010, 58, 3996–4000 CrossRef CAS PubMed.
- R. D. Rogers and S. Monsell, Costs of a predictible switch between simple cognitive tasks, J. Exp. Psychol. Gen., 1995, 124, 207–231 Search PubMed.
- S. Monsell, N. Yeung and R. Azuma, Reconfiguration of task-set: Is it easier to switch to the weaker task?, Psychol. Res., 2000, 63, 250–264 CrossRef CAS PubMed.
- S. Monsell, P. Sumner and H. Waters, Task-set reconfiguration with predictable and unpredictable task switches, Mem. Cogn., 2003, 31, 327–342 CrossRef PubMed.
- S. P. Woods, D. C. Delis, J. C. Scott, J. H. Kramer and J. A. Holdnack, The California Verbal Learning Test – second edition: Test-retest reliability, practice effects, and reliable change indices for the standard and alternate forms, Arch. Clin. Neuropsychol., 2006, 21, 413–420 CrossRef PubMed.
- R. P. Feliciano, G. Istas, C. Heiss and A. Rodriguez-Mateos, Plasma and urinary phenolic profiles after acute and repetitive intake of wild blueberry, Molecules, 2016, 21, 1120 CrossRef PubMed.
- A. Rodriguez-Mateos, T. Cifuentes-Gomez, S. Tabatabaee, C. Lecras and J. P. E. Spencer, procyanidin, anthocyanin, and chlorogenic acid contents of highbush and lowbush blueberries, J. Agric. Food Chem., 2012, 60, 5772–5778 CrossRef CAS PubMed.
- J. Scalzo, A. Currie, J. Stephens, T. McGhie, P. Alspach and Horticulture and, Food Research Institute Of New Zealand Limited Hortresearch, The anthocyanin composition of different Vaccinium, Ribes and Rubus genotypes, Biofactors, 2008, 34, 13–21 CrossRef PubMed.
- A. Rodriguez-Mateos, C. Rendeiro, T. Bergillos-Meca, S. Tabatabaee, T. W. George, C. Heiss and J. P. Spencer, Intake and time dependence of blueberry flavonoid-induced improvements in vascular function: a randomized, controlled, double-blind, crossover intervention study with mechanistic insights into biological activity, Am. J. Clin. Nutr., 2013, 98, 1179–1191 CrossRef CAS PubMed.
- A. Rodriguez-Mateos, R. P. Feliciano, T. Cifuentes-Gomez and J. P. E. Spencer, Bioavailability of wild blueberry (poly)phenols at different levels of intake, J. Berry Res., 2016, 6, 137–148 CAS.
- S. Zhong, A. Sandhu, I. Edirisinghe and B. Burton-Freeman, Characterization of wild blueberry polyphenols bioavailability and kinetic profile in plasma over 24 h period in human subjects, Mol. Nutr. Food Res., 2017, 61, 1700405 CrossRef PubMed.
- N. Travica, N. M. D'Cunha, N. Naumovski, K. Kent, D. D. Mellor, J. Firth, E. N. Georgousopoulou, O. M. Dean, A. Loughman, F. Jacka and W. Marx, The effect of blueberry interventions on cognitive performance and mood: A systematic review of randomized controlled trials, Brain, Behav., Immun., 2020, 85, 96–105 CrossRef CAS PubMed.
- J. L. Bowtell, Z. Aboo-Bakkar, M. E. Conway, A. R. Adlam and J. Fulford, Enhanced task-related brain activation and resting perfusion in healthy older adults after chronic blueberry supplementation, Appl. Physiol., Nutr., Metab., 2017, 42, 773–779 CrossRef CAS PubMed.
- A. K. Sandhu, M. G. Miller, N. Thangthaeng, T. M. Scott, B. Shukitt-Hale, I. Edirisinghe and B. Burton-Freeman, Metabolic fate of strawberry polyphenols after chronic intake in healthy older adults, Food Funct., 2018, 9, 96–106 RSC.
- B. A. Sandoval-Ramírez, Ú. Catalán, S. Fernández-Castillejo, L. Rubió, A. Macià and R. Solà, Anthocyanin tissue bioavailability in animals: Possible implications for human health. A systematic review, J. Agric. Food Chem., 2018, 66, 11531–11543 CrossRef PubMed.
- W. Kalt, J. B. Blumberg, J. E. McDonald, M. R. Vinqvist-Tymchuk, S. A. Fillmore, B. A. Graf, J. M. O'Leary and P. E. Milbury, Identification of anthocyanins in the liver, eye, and brain of blueberry-fed pigs, J. Agric. Food Chem., 2008, 56, 705–712 CrossRef CAS PubMed.
- A. K. Sandhu, Y. Huang, D. Xiao, E. Park, I. Edirisinghe and B. Burton-Freeman, Pharmacokinetic characterization and bioavailability of strawberry anthocyanins relative to meal intake, J. Agric. Food Chem., 2016, 64, 4891–4899 CrossRef CAS PubMed.
- W. Mullen, C. A. Edwards, M. Serafini and A. Crozier, Bioavailability of pelargonidin-3-O-glucoside and its metabolites in humans following the ingestion of strawberries with and without cream, J. Agric. Food Chem., 2008, 56, 713–719 CrossRef CAS PubMed.
- W. Kalt, Anthocyanins and their C(6)-C(3)-C(6) metabolites in humans and animals, Molecules, 2019, 24, 4024 CrossRef CAS PubMed.
- J. Fang, Some anthocyanins could be efficiently absorbed across the gastrointestinal mucosa: Extensive presystemic metabolism reduces apparent bioavailability, J. Agric. Food Chem., 2014, 62, 3904–3911 CrossRef CAS PubMed.
- C. Czank, A. Cassidy, Q. Zhang, D. J. Morrison, T. Preston, P. A. Kroon, N. P. Botting and C. D. Kay, Human metabolism and elimination of the anthocyanin, cyanidin-3-glucoside: a (13)C-tracer study, Am. J. Clin. Nutr., 2013, 97, 995–1003 CrossRef CAS PubMed.
- A. Costabile, A. Klinder, F. Fava, A. Napolitano, V. Fogliano, C. Leonard, G. R. Gibson and K. M. Tuohy, Whole-grain wheat breakfast cereal has a prebiotic effect on the human gut microbiota: a double-blind, placebo-controlled, crossover study, Br. J. Nutr., 2008, 99, 110–120 CrossRef CAS PubMed.
- A. Rodriguez-Mateos, R. Del Pino-García, T. W. George, A. Vidal-Diez, C. Heiss and J. P. Spencer, Impact of processing on the bioavailability and vascular effects of blueberry (poly)phenols, Mol. Nutr. Food Res., 2014, 58, 1952–1961 CrossRef CAS PubMed.
- C. Vetrani, A. A. Rivellese, G. Annuzzi, M. Adiels, J. Borén, I. Mattila, M. Orešič and A. M. Aura, Metabolic transformations of dietary polyphenols: comparison between in vitro colonic and hepatic models and in vivo urinary metabolites, J. Nutr. Biochem., 2016, 33, 111–118 CrossRef CAS PubMed.
- K. Hanhineva, M. A. Lankinen, A. Pedret, U. Schwab, M. Kolehmainen, J. Paananen, V. de Mello, R. Sola, M. Lehtonen, K. Poutanen, M. Uusitupa and H. Mykkänen, Nontargeted metabolite profiling discriminates diet-specific biomarkers for consumption of whole grains, fatty fish, and bilberries in a randomized controlled trial, J. Nutr., 2015, 145, 7–17 CrossRef CAS PubMed.
- K. J. Penczynski, D. Krupp, A. Bring, K. Bolzenius, T. Remer and A. E. Buyken, Relative validation of 24 h urinary hippuric acid excretion as a biomarker for dietary flavonoid intake from fruit and vegetables in healthy adolescents, Eur. J. Nutr., 2017, 56, 757–766 CrossRef CAS PubMed.
- R. M. de Ferrars, C. Czank, Q. Zhang, N. P. Botting, P. A. Kroon, A. Cassidy and C. D. Kay, The pharmacokinetics of anthocyanins and their metabolites in humans, Br. J. Pharmacol., 2014, 171, 3268–3282 CrossRef CAS PubMed.
- A. Angelini, C. Bendini, F. Neviani, L. Bergamini, B. Manni, T. Trenti, R. Rovati and M. Neri, Insulin-like growth factor-1 (IGF-1): Relation with cognitive functioning and neuroimaging marker of brain damage in a sample of hypertensive elderly subjects, Arch. Gerontol. Geriatr., 2009, 49, 5–12 CrossRef CAS PubMed.
- M. Picillo, R. Pivonello, G. Santangelo, C. Pivonello, R. Savastano, R. Auriemma, M. Amboni, S. Scannapieco, A. Pierro, A. Colao, P. Barone and M. T. Pellecchia, Serum IGF-1 is associated with cognitive functions in early, drug-naïve Parkinson's disease, PLoS One, 2017, 12, e0186508 CrossRef PubMed.
- H. Shimada, H. Makizako, T. Doi, D. Yoshida, K. Tsutsumimoto, Y. Anan, K. Uemura, S. Lee, H. Park and T. Suzuki, A large, cross-sectional observational study of serum BDNF, cognitive function, and mild cognitive impairment in the elderly, Front. Aging Neurosci., 2014, 6, 69–69 CrossRef PubMed.
Footnote |
† Electronic supplementary information (ESI) available. See DOI: 10.1039/d0fo02125c |
|
This journal is © The Royal Society of Chemistry 2021 |