DOI:
10.1039/C8RA04460K
(Paper)
RSC Adv., 2018,
8, 26029-26035
Immunomagnetic bead-based biotin-streptavidin system for highly efficient detection of aflatoxin B1 in agricultural products†
Received
25th May 2018
, Accepted 1st July 2018
First published on 19th July 2018
Abstract
The potential homogeneous assay employing immunomagnetic beads (IMB) has been receiving attention as a screening tool in food-safety control; the method is simple, efficient, and does not require long incubation times or complex separation steps. In this study, a homogeneous immunoassay has been successfully developed and applied in the determination of aflatoxin B1 (AFB1) contamination in agricultural products by coupling IMB and the biotin-streptavidin (BSA) (BSA-IMB) system. Under optimal conditions, the limit of detection (LOD, IC10), half-maximal inhibition concentration (IC50) and detection range (IC20–IC80) of BSA-IMB are 0.00579, 0.573 and 0.0183–17.9 ng mL−1, respectively, for AFB1. The detection of AFB1 by BSA-IMB can be achieved in 40 min (ELISA needs at least 180 min). The cross-reactivities of BSA-IMB with its analogues are negligible (<3.82%); these results indicate high selectivity. The spiked recoveries are in the range from 89.6 to 118.2% with relative standard deviations (RSDs) of 3.4 to 13.2% for AFB1 in agricultural product samples. Furthermore, the results of BSA-IMB for authentic samples show reliability and high correlation of 0.9928 with an HPLC-fluorescence detector. The proposed BSA-IMB system is demonstrated to be a satisfactory tool for homogeneous, efficient, sensitive, and alternative detection of AFB1 in a wide detection range for agricultural product samples.
1 Introduction
Aflatoxins are highly toxic secondary metabolites produced by a number of different fungi, and they are present in various agricultural products and feeds; they have significance because of their deleterious effects on human beings, livestock and poultry.1–3 Aflatoxin B1 (AFB1) is the predominant and most toxic aflatoxin, and it has been used to establish maximum limits (MLs) by various government agencies.4 In the European Union, the rigorous legal limit for AFB1 in groundnuts, nuts, dried fruits, and cereal has been regulated at 2 μg kg−1.5,6 In China, MLs of AFB1 are set below 10 μg kg−1 in rice and below 5 μg kg−1 in infant foods.7 Assessing contamination of AFB1 in food and feed producing chain is of great importance and necessity.
Various analytical methods have been reported for the determination of AFB1; these mainly include chromatographic methods and immunochemical assays such as detection using a HPLC-fluorescence detector (FLD),5,8 HPLC-MS/MS,9–11 enzyme-linked immunosorbent assays (ELISA)1 and gold immunochromatographic assays.12 The chromatographic methods are standardized, high precision and sensitive, but they are also time-consuming, expensive and unsuitable for screening purposes. Immunoassays are increasingly considered as alternatives or complementary methods for AFB1 analysis as they have significant advantages such as high selectivity and sensitivity, simplicity, rapidity, and cost-effectiveness.13 ELISA is the most commonly used microplate-based immunochemical assay, and it is a heterogeneous method, which requires extensive pipetting, washing and incubation steps; therefore, it is time-consuming (typically more than 2 h for an analysis) and complex. A very promising way to overcome these problems of immunoassays is a shift from heterogeneous methods to homogeneous assays.14
Immunomagnetic beads (IMB), which are used in a potential homogeneous assay, are receiving attention as a new immobile phase pattern, as they can diffuse freely in the reaction mixture, separate easily with the aid of an external magnet, and have an efficient reaction that does not require long incubation time.15,16 Moreover, IMB have good biocompatibility for coupling with antibodies or small molecules and larger surface area than the flat base of a microtiter plate, which immobilizes a higher number of ‘active molecules’ and enhances the sensitivity of the enzymatic immunoassay.15,17,18 The biotin-streptavidin (BSA) system is a potential and valuable approach for achieving signal amplification and improving sensitivity.19 This system has been used for developing analytical methods, which can solve the limitation of labeling HRP amount on target proteins, thus obtaining perfect results.20 With the help of BSA technology, a large amount of HRP, which conjugates with streptavidin, can be reflected by substrate catalysis and chromogenic reaction.21 Hence, highly efficient and sensitive detection can be easily achieved using the IMB system coupled with BAS system.
The present study aims to use IMB system coupled with BAS system to develop a homogeneous and efficient immunoassay (BSA-IMB) for assessing AFB1 contamination in agricultural product samples (Fig. 1). For the determination of AFB1, IMB and BSA-IMB based on monoclonal antibody (McAb) were developed and reported. BSA-IMB showed higher sensitivity and wider detection range and thus, it was chosen as the ideal system for detecting AFB1. The matrix effects of 5 agricultural product samples for BSA-IMB were evaluated. Moreover, BSA-IMB was applied to detect AFB1 in authentic samples of agricultural products, and the results were confirmed by HPLC-FLD.
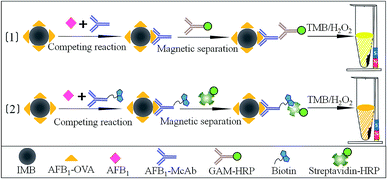 |
| Fig. 1 Schematic illustrations of IMB (1) and BSA-IMB (2) for detecting AFB1. | |
2 Materials and methods
2.1 Reagents and equipment
Carboxylic group-modified magnetic microspheres (200 nm) were purchased from Suzhou Vdo Biotech Co., Ltd. (Suzhou, China). Analytical standards of AFB1, its analogues (AFB2, AFG1, AFG2, and AFM1), and goat anti-mouse immunoglobulin horseradish peroxidase (GAM-HRP) were purchased from Sigma Chemical Co. (St. Louis, USA). Commercial antigen (AFB1-OVA) of AFB1 was obtained from Wuxi Determine Bio-Tech Co., Ltd. (Wuxi, China). Streptavidin conjugated with HRP (streptavidin-HRP) was purchased from Beijing Biodragon Immunotechnologies Co., Ltd. (Beijing, China). N-Hydroxysulfosuccinimide sodium salt (sulfo-NHS) and 1-ethyl-3-(3-dimethylaminopropyl) carbodiimide hydrochloride (EDC) were supplied by Adamas-beta Reagent Co., Ltd. (Shanghai, China). Biotinyl-N-hydroxy-succinimide (BNHS), 2-morpholinoethanesulfonic acid (MES), 3′,5,5′-tetramethyl benzidine (TMB), H2O2, bovine serum albumin, ovalbumin (OVA), polyoxyethylene sorbitan monolaurate (Tween-20) and other chemical reagents were purchased from Aladdin (Shanghai, China). Anti-AFB1 McAb was prepared and stored in our laboratory.22
2-Morpholino-ethanesulfonic acid buffer (MES, 0.05 mol L−1, pH 5.2), carbonate-buffered saline buffer (CBS, 0.05 mol L−1, pH 9.6), phosphate-buffered saline buffer (PBS, 0.01 mol L−1, pH 7.4) and phosphate-buffered saline containing 0.05% Tween-20 (PBST) were prepared and stored in our laboratory. Concentrated aqueous solutions of sulfo-NHS (94.3 mg mL−1) and EDC (50 mg mL−1) were prepared in our laboratory before use. TMB solution contained 0.4 mmol L−1 TMB and 3 mmol L−1 H2O2 in citrate buffer (pH 5.0).
Milli-Q purified water was obtained from the Milli-Q purification system (Bedford, MA, USA). Absorbance was detected using an Infinite M1000 Pro microtiter plate reader (Tecan, Switzerland). Centrifugation was performed on a Neofuge 18R centrifuge (Hongkong, China). The KQ2200 ultrasonic apparatus was provided by Kunshan Ultrasonic Instruments Co., Ltd (Kunshan, China). Shake-incubation was carried out on a TS-A shaker (Jintan, China), and magnetic separation was performed on a magnetic separator (Tianjin, China). The results of BSA-IMB were validated with Aglient 1260 HPLC equipped with a fluorescence detector (Wilmington, DE, USA).
2.2 Preparation of magnetic microspheres-antigen
The bioconjugation strategy between carboxylic group-modified magnetic microspheres and amino-groups of the antigen AFB1-OVA was achieved via the EDC/NHS method.17,23 Briefly, carboxylic group-modified magnetic microspheres (50 μL, 50 mg/10 mL) were first ultrasonically dispersed and activated by incubating with EDC (200 μL, 50 mg mL−1) and sulfo-NHS (200 μL, 94.3 mg mL−1) in 550 μL MES buffer. The mixture was stirred for 30 min at room temperature and then magnetically separated; next, the supernatant was discarded. Activated magnetic microspheres were resuspended and washed twice with 2 mL MES buffer and then resuspended with 950 μL MES buffer. AFB1-OVA (dialyzed against MES buffer, 50 μL, 6 μg mL−1) was added and stirred overnight at 4 °C. After another step of separation and washing, 2% bovine serum albumin solution in PBS buffer (1 mL, containing 0.05% sodiumazide) was added to block nonspecific sites on magnetic microspheres for 2 h at 4 °C and then, the product was stored at 4 °C.
2.3 Preparation of biotinylated-McAb
Biotinylated-McAb was prepared according to a previously reported method with modifications.20 Anti-AFB1 McAb was dissolved and dialyzed in CBS (0.1 mol L−1, pH 9.2) for 4 h. BNHS was dissolved in 200 μL DMSO and adjusted to 38 mg mL−1; then, 10-fold molar excess against 2 mg mL−1 McAb was added. The mixture solution was stirred at room temperature for 6 h and dialyzed against PBS buffer overnight at 4 °C. Finally, the biotinylated-McAb sample was preserved in PBS buffer (containing 3% bovine serum albumin, 50% glycerol) and stored at −20 °C.
2.4 Procedures of immunoassays
For ELISA. The procedures of ELISA were followed according to the classic method.21 After the coating and blocking steps, either standard serial concentrations or samples of AFB1 in PBS containing methanol (50 μL per well) were added, followed by addition of optimal McAb dilution (50 μL per well, in PBS) for 1 h at 37 °C. After further washing, GAM-HRP dilution (100 μL per well, in PBS) was dispensed into each well and incubated for 1 h at 37 °C. Then, the plates were washed again. TMB solution (100 μL per well) was added to the plates and incubated for 15 min at 37 °C. Then, the reaction was stopped with 2 mol L−1 sulfuric acid (50 μL per well), and the absorbance was measured at 450 nm.
For IMB. The magnetic microsphere-antigen dilution (50 μL per tube) was added, followed by adding standard serial concentrations or samples of AFB1 in PBS containing methanol (50 μL per tube) and optimal McAb dilution (100 μL per tube, in PBS) together for 20 min at 37 °C. After magnetic separation and washing with PBST, GAM-HRP dilution (100 μL per tube, in PBS) was added and incubated for 20 min at 37 °C. After another separation and washing, the HRP activity of complexes was studied by adding TMB solution (100 μL per tube). The reaction was stopped with 2 mol L−1 sulfuric acid (50 μL per tube) after 10 min of incubation at 37 °C, and the absorbance was measured at a wavelength of 450 nm.
For BSA-IMB. The schematic diagram of the BSA-IMB procedures for determination of AFB1 is shown in Fig. 1. The optimal dilutions of magnetic microspheres-antigen (50 μL per tube), standard serial concentrations or samples of AFB1 (50 μL per tube), and biotinylated-McAb (100 μL per tube) were added in order, and competing reactions proceeded for 20 min at 37 °C. Following another signal amplification reaction of the BSA system by adding streptavidin-HRP dilution (100 μL per tube, in PBS) and incubating for 10 min at 37 °C, peroxidase activity was revealed, and the absorbance was measured using the same procedure as that used in the above-mentioned method.
Standard curves. A series of AFB1 standards were prepared by diluting AFB1 standards in PBS buffer containing methanol. Determinations were carried out in triplicate, and the mean values of B/B0 (B: absorbance signal with analytes; B0: absorbance signal in the absence of analytes) were plotted against the logarithm of analyte concentration to obtain the competitive curves. The half-maximal inhibition concentration (IC50), limit of detection (LOD, IC10) and detection range (IC20–IC80) were obtained from a four-parameter logistic equation of the sigmoidal curves using the Origin Pro 7.0 software.
Optimization of experimental parameters. The experimental parameters such as concentrations of magnetic microspheres-antigen, McAb, biotinylated-McAb, GAM-HRP, and streptavidin-HRP as well as ionic strength, contents of organic solvents and pH were studied to improve the sensitivity of immunoassays. Solutions with series concentrations of analytes and varied experimental parameters were tested. The B0/IC50 ratio and IC50 values were used as primary criteria to evaluate immunoassay performances; the highest ratio of B0/IC50 and the lowest value of IC50 were the most desirable.
Cross-reactivities. Additionally, cross-reactivities (CRs) for compounds structurally related to AFB1 were used to evaluate the selectivity of BSA-IMB. The CR values were calculated as follows:
CR% = (IC50 of analyte/IC50 of analogue) × 100 |
2.6 Analysis of spiked samples by BSA-IMB
Agricultural product samples (rice flour, wheat flour, corn flour, corn and peanuts) certified as free of AFB1 were used for matrix effect and recovery studies. These homogenized agricultural product samples were finely chopped. Then, the agricultural product samples (5 g) were spiked with AFB1 at 0.1, 0.5, 2.5, and 10 ng g−1 and stored overnight to allow drug–matrix interaction. Next, the extraction solution (20 mL of methanol-PBS, v/v, 3
:
2; and 20 mL of n-hexane) was added. The tubes were shaken with a vortex mixer for 20 min and then allowed to stand for 30 min. The solutions were filtered, diluted to an appropriate multiple using the optimized working solution, and analyzed by BSA-IMB. Each analysis was performed in triplicate.
To analyze the effects of matrix on sensitivity, the extracted agricultural product samples were analysed by a series of dilutions with PBS (containing 5% methanol). The matrix effects were determined by comparing standard curves of AFB1 prepared in matrix extract and standard curves of AFB1 prepared in PBS buffer free of matrix.
2.7 Evaluation of BSA-IMB with HPLC-FLD
To test the effectiveness of the developed BSA-IMB, authentic agricultural product samples were chosen from flour factories and marketplaces and prepared with the same procedure described above. Then, each sample was divided into two portions: one was analyzed using BSA-IMB, and the other using HPLC-FLD. For HPLC-FLD, the extracted solutions were adjusted to pH of about 6.0–7.0 and then cleaned and concentrated through AflaTest immuno-affinity columns (Vicam, USA). In the step of derivatisation, 200 μL of n-hexane and 100 μL of trifluoroacetic acid were added to the purified and evaporated residue; after 30 s of vortex mixing and 15 min of derivatisation at 40 °C in a water bath, it was dried under nitrogen. The residue was dissolved in 200 μL of acetonitrile and filtered through a membrane filter (0.22 μm). Then, 100 μL supernatant was injected into HPLC-FLD for analysis. HPLC-FLD analysis was performed on an Eclipse XDB2-C18 column (250 mm × 4.6 mm × 5 μm) using a mixture of water, methanol and acetonitrile (11
:
4:5, v/v) as the mobile phase at a flow rate of 1.0 mL min−1 at 35 °C. The excitation wavelength and detection wavelength were set at 355 nm and 430 nm, respectively.
3 Results and discussion
3.1 Optimization of immunoassay conditions
As shown in Table 1, the parameters for IMB and BSA-IMB were optimized. The concentrations of biochemical reagents were first optimized. The optimal concentrations of magnetic microspheres-antigen were 0.2 ng mL−1 for IMB and 0.1 ng mL−1 for BSA-IMB. The optimal concentrations of McAb and biotinylated-McAb were 1.3 ng mL−1 and 0.6 ng mL−1 for IMB and BSA-IMB, respectively. We used 1
:
8000 of GAM-HRP and 1
:
38
000 of streptavidin-HRP for IMB and BSA-IMB, respectively.
Table 1 Optimization of the parameters for immunoassays
Factors |
IMB |
Factors |
BSA-IMB |
Magnetic microspheres-antigen |
0.2 ng mL−1 |
Magnetic microspheres-antigen |
0.1 ng mL−1 |
McAb |
1.3 ng mL−1 |
Biotinylated-McAb |
0.6 ng mL−1 |
GAM-IgG-HRP |
1 : 8000 |
Streptavidin-HRP |
1 : 38 000 |
Methanol (v/v, %) |
5 |
Methanol (v/v, %) |
5 |
Na+ (mol L−1) |
0.5 |
Na+ (mol L−1) |
0.5 |
pH value |
7.4 |
pH value |
7.4 |
Organic solvent, ionic strength and pH were investigated to optimize immunoassays (Fig. S1†), and the optimal results are summarized in Table 1. Methanol was selected to improve the solubility of analytes and evaluate its effect on the immunoassays. The values of B0/IC50 tended to decrease with the increase in methanol, and the IC50 values showed drastic increase when the methanol content was above 5% for IMB and BSA-IMB. The change in Na+ concentration from 0.1 to 0.6 mol L−1 influenced immunoassays dramatically. The highest B0/IC50 and lowest IC50 values were acquired at 0.5 mol L−1 Na+ for IMB and BSA-IMB. In addition, pH did not have a notable effect on the sensitivity of the immunoassays. On the basis of these results, 5% methanol, 0.5 mol L−1 Na+ and pH 7.4 were chosen as optimal IMB and BSA-IMB conditions.
3.2 Sensitivities
The calibration curves of AFB1 using immunoassays were constructed under optimum conditions. The graph between percent binding (% B/B0) and the logarithm of concentration of AFB1 (ng mL−1) was plotted (Fig. 2). IMB for AFB1 was shown to have LOD of 0.0335 ng mL−1, IC50 of 0.606 ng mL−1, and detection range (IC20–IC80) of 0.0690–5.32 ng mL−1. BSA-IMB showed higher sensitivity, with LOD value, IC50 value and detection range of 0.00579, 0.573 and 0.0183–17.9 ng mL−1, respectively. Using same McAb, the ELISA results for AFB1 exhibited LOD of 0.0440 ng mL−1, IC50 of 0.245 ng mL−1, and a detection range of 0.0681–0.879 ng mL−1. The chromogenic results of BAS-IMB in standard serial concentrations of AFB1 are shown in Fig. S3.† As can be seen from the diagram, the colours of solutions gradually deepened with the decrease in AFB1 concentration, and these results were reversed by increasing the concentrations of AFB1. Finally, the developed BSA-IMB method was selected for follow-up research and determination.
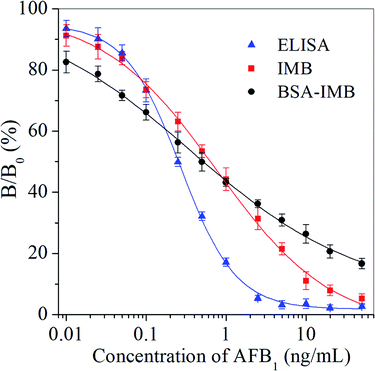 |
| Fig. 2 Immunoassay calibration curves for AFB1. | |
As LODs of ELISA, IMB and BSA-IMB were below MLs of AFB1, the sensitivity of the developed immunoassays can meet the requirements for detecting AFB1. Using popular materials of magnetic microspheres, new load and separation techniques have been achieved. Thus, the detection patterns of heterogeneous ELISA can be changed to those of homogeneous IMB and BSA-IMB in this study. Through the use of new labeling techniques and signal amplification strategies, the detection efficiency and convenience of immunoassays can be improved, which are popular requirements desirable in the detection of harmful substances.
In this study, LODs showed clear improvements (LODs of BSA-IMB improved 7.6-fold and 5.8-fold over those of ELISA and IMB, respectively). Moreover, the detection range of BSA-IMB (covers 4 orders of magnitude) was wider than those of IMB and ELISA, which is more convenient and practical for detecting AFB1. Without the use of special instruments and expensive reagents, higher sensitivity and wider detection range of BSA-IMB could be achieved after coupling with the BSA system, which has strong signal amplification capability. Compared to the reported instrument-based detection methods and immunoassays for AFB1, the developed BSA-IMB method possessed high sensitivity, which should be feasible and worthy of wide use. Therefore, the proposed BSA-IMB format was selected for further research and applications in the detection of AFB1 in agricultural product samples.
Objectively speaking, instrument-based detection methods often require complicated cleanup procedures, and it is difficult to achieve high-throughput screening for a large number of samples; ELISAs need a long period of analysis time and complex separation processes. Compared with the ELISA for AFB1, which requires 180 min of analysis time by multistep reactions (5 steps), homogeneous BSA-IMB can be achieved in 3 steps in 40 min without complex incubation and washing steps. Thus, the developed BSA-IMB method exhibits great improvement with regard to shortening the overall testing time and analytical procedures (Table 2).
Table 2 The parameters of immunoassays for AFB1 using the same McAb
Immunoassay |
LOD (IC10, ng mL−1) |
IC50 (ng mL−1) |
Detection range (IC20–IC80, ng mL−1) |
Detection time (min) |
Detection step |
ELISA |
0.0440 |
0.245 |
0.0681–0.879 |
180 |
5 |
IMB |
0.0335 |
0.606 |
0.0690–5.32 |
50 |
3 |
BSA-IMB |
0.00579 |
0.573 |
0.0183–17.9 |
40 |
3 |
The reasons for these observations might be that McAb binds more easily to the target compounds in BSA-IMB because they are distributed more uniformly in the liquid reaction system than in the solid-phase ELISA system. In addition, the BSA-IMB system, as an immobile phase pattern, has larger surface area than the flat base of a microtiter plate, which can immobilize a high number of antibodies.18,24 Moreover, the volume of the sample loaded in magnetic microspheres is larger than that in conventional ELISA, which enables more free targets to occupy the antibody binding sites and improves the sensitivity of the immunoassay. In summary, due to numerous potential advantages of BSA-IMB, it is a straightforward, rapid and highly efficient testing method.
3.3 Selectivity
BSA-IMB showed negligible CRs with analogues (Table 3). Different levels of CRs were found between AFB1 and its analogues in some reports.25–27 In this BSA-IMB study, CRs of AFB1 for AFM1, AFG1 and AFB2 were 3.82%, 3.67% and 0.53%, respectively; the CR value was lower than 0.06% for AFG2. Therefore, negligible CRs between AFB1 and its analogues guaranteed the use of BSA-IMB for specific determination of AFB1.
Table 3 Cross-reactivity of AFB1 toward its analogues by BSA-IMB
Compound |
Structure |
IC50 (ng mL−1) |
CR (%) |
AFB1 |
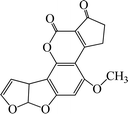 |
0.573 |
100 |
AFM1 |
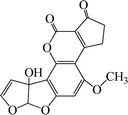 |
15.0 |
3.82 |
AFG1 |
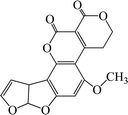 |
15.6 |
3.67 |
AFB2 |
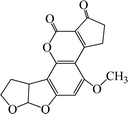 |
108.1 |
0.53 |
AFG2 |
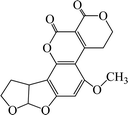 |
>1000 |
<0.06 |
3.4 Matrix effects
Matrix effects are one of the most common challenges while performing immunoassays on complex samples. Sample dilution is the easiest and most immediate way to minimize matrix effects. Different sample matrices have different effects on the sensitivity of immunoassays. The matrix effects of 5 agricultural product samples on the sensitivity of BSA-IMB are shown in Fig. S2.† With the increasing dilution multiples, the matrix effects on sensitivity were reduced. The scheme of dilution was as follows: 8-fold dilution of rice flour sample and 10-fold dilution of wheat flour, corn flour, corn and peanut samples. These dilution schemes were also applied for subsequent experiments.
3.5 Accuracy and precision
Recoveries and relative standard deviations (RSDs) were calculated to evaluate accuracy and precision of BSA-IMB. As illustrated in Table 4, the recoveries of AFB1 for BSA-IMB ranged from 89.6 to 118.2% with RSDs between 3.4 and 13.2%. These results indicated that the accuracy and precision of the developed BSA-IMB were satisfactory for the qualitative and quantitative determination of AFB1 in agricultural products.
Table 4 Accuracy and precision of AFB1 in agricultural products by BSA-IMB
Sample |
Spiked (ng g−1, n = 3) |
Dilution times |
Mean recovery ± SD (%) |
RSD (%) |
Rice flour |
0.1 |
8 |
94.8 ± 6.2 |
6.5 |
0.5 |
103.0 ± 5.1 |
4.9 |
2.5 |
101.9 ± 10.7 |
10.5 |
10 |
102.4 ± 12.3 |
12.0 |
Wheat flour |
0.1 |
10 |
99.9 ± 9.5 |
9.5 |
0.5 |
109.6 ± 6.2 |
5.6 |
2.5 |
95.6 ± 12.6 |
13.2 |
10 |
114.1 ± 5.7 |
5.0 |
Corn flour |
0.1 |
10 |
105.3 ± 6.5 |
6.2 |
0.5 |
115.7 ± 4.0 |
3.4 |
2.5 |
115.6 ± 3.9 |
3.4 |
10 |
103.9 ± 8.0 |
7.7 |
Corn |
0.1 |
10 |
108.8 ± 7.5 |
6.9 |
0.5 |
89.6 ± 5.8 |
6.5 |
2.5 |
96.1 ± 7.9 |
8.2 |
10 |
90.2 ± 8.8 |
9.8 |
Peanut |
0.1 |
10 |
97.6 ± 3.8 |
3.9 |
0.5 |
104.8 ± 3.7 |
3.5 |
2.5 |
118.2 ± 6.1 |
5.2 |
10 |
101.6 ± 11.8 |
11.6 |
3.6 Correlation of BSA-IMB with HPLC-FLD
Comparative analyses of the samples of agricultural products naturally contaminated with AFB1 were performed by both the developed BSA-IMB method and reference method of HPLC-FLD. Using BSA-IMB, we found that the samples suffered varying degrees of contamination by AFB1, which ranged from 1.25 to 425.8 ng g−1. Subsequent HPLC-FLD gave largely consistent results when compared with BSA-IMB, and the positive results ranged from 1.73 to 400.2 ng g−1. Good correlations were obtained between the results of BSA-IMB and HPLC-FLD (y = 0.9885x + 2.0781, R2 = 0.9928) (Fig. 3). These results further demonstrated that AFB1 in authentic samples can be simply, rapidly, homogeneously and efficiently detected by the proposed BSA-IMB method.
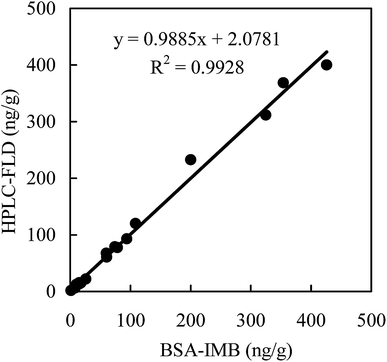 |
| Fig. 3 Correlation between BSA-IMB and HPLC-FLD for detecting AFB1 in authentic samples (n = 3). | |
4 Conclusions
In summary, a simple and efficient BSA-IMB method for the determination of AFB1 was successfully developed by coupling the BSA system with the IMB system. Using this simple BSA-IMB system, the detection of AFB1 contamination was realized in a simple, efficient, and sensitive manner in a wide detection range. The detection of AFB1 by BSA-IMB was achieved in 40 min (ELISA needs at least 180 min). The accuracy and precision of BSA-IMB met the requirements of AFB1 analysis. The studies of agricultural product samples were conducted using both BSA-IMB and HPLC-FLD to demonstrate the reliability of BSA-IMB in AFB1 assessment. Moreover, the developed BSA-IMB method was found to be ideal as less use of antigen and antibody was observed. The developed BSA-IMB method is a sensitive and economical method for large-scale screening and monitoring of AFB1 in agricultural product samples. In future studies, BSA-IMB can be developed to assay more analytes and different matrix samples; thus, the determination of harmful substances will be achieved through a more sensitive, inexpensive and alternative method.
Conflicts of interest
There are no conflicts to declare.
Acknowledgements
This work was supported by the National Key Research Development Program of China (2017YFC1200100), the National Natural Science Foundation of China (31701687, 31570414, 31770446), the Natural Science Foundation of Jiangsu Province (BK20170537), the China Postdoctoral Science Foundation (2016M601745), the Senior Talent Scientific Research Initial Funding Project of Jiangsu University (16JDG035), the Project Funded by the Priority Academic Program Development of Jiangsu Higher Education Institutions (PAPD) and the the Project Funded by the Priority Academic Program Development of Jiangsu Higher Education Institutions (PAPD).
References
- C. N. Rossi, C. P. Takabayashi, M. A. Ono, G. H. Saito, E. N. Itano, O. Kawamura, E. Y. Hirooka and E. Y. S. Ono, Food Chem., 2012, 132, 2211–2216 CrossRef.
- J. Xie, Y. Z. Sun, Y. J. Zheng, C. Wang, S. J. Sun, J. C. Li, S. Y. Ding, X. Xia and H. Y. Jiang, Food Control, 2017, 73, 445–451 CrossRef.
- M. W. Yao, L. Y. Wang and C. Z. Fang, Luminescence, 2017, 32, 661–665 CrossRef PubMed.
- Y. Zhang, Z. Y. Liao, Y. J. Liu, Y. J. Wan, J. Chang and H. J. Wang, Microchim. Acta, 2017, 184, 1471–1479 CrossRef.
- F. F. Chen, C. L. Luan, L. Wang, S. Wang and L. H. Shao, J. Sci. Food Agric., 2017, 97, 1805–1810 CrossRef PubMed.
- European Commission, Commission Regulation No. 1881/2006, Official J. European Union, 2006, L364, 5–24.
- P. R. China National Standard No. GB2761-2017, Ministry of Health of China, Beijing, 2017.
- O. Golge, F. Hepsag and B. Kabak, Food Control, 2016, 59, 731–736 CrossRef.
- S. Hickert, J. Gerding, E. Ncube, F. Hübner, B. Flett, B. Cramer and H. U. Humpf, Mycotoxin Res., 2015, 31, 109–115 CrossRef PubMed.
- S. F. Fan, Q. Li, L. Sun, Y. S. Du, J. Xia and Y. Zhang, Food Addit. Contam. A, 2015, 32, 1175–1184 CrossRef PubMed.
- Z. Zhang, X. Hu, Q. Zhang and P. W. Li, J. Chromatogr. B: Anal. Technol. Biomed. Life Sci., 2016, 1021, 145–152 CrossRef PubMed.
- Y. L. Yuan, X. D. Hua, M. Li, W. Yin, H. Y. Shi and M. H. Wang, RSC Adv., 2014, 4, 24406–24411 RSC.
- G. F. Lin, H. Zhao, T. C. Liu, J. J. Hou, Z. Q. Ren, Y. S. Wu, W. Q. Dong and W. H. Huang, RSC Adv., 2014, 4, 55229–55236 RSC.
- D. S. Smith and S. A. Eremin, Anal. Bioanal. Chem., 2008, 391, 1499–1507 CrossRef PubMed.
- X. Wang, R. Niessner and D. Knopp, Sensors, 2014, 14, 21535–21548 CrossRef PubMed.
- X. Wang, R. Niessner, D. Tang and D. Knopp, Anal. Chim. Acta, 2016, 912, 10–23 CrossRef PubMed.
- F. Song, Y. Zhou, Y. S. Li, X. M. Meng, X. Y. Meng, J. Q. Liu, S. Y. Lu, H. L. Ren, P. Hu, Z. S. Liu, Y. Y. Zhang and J. H. Zhang, Food Chem., 2014, 158, 445–448 CrossRef PubMed.
- S. C. Yu, F. Yu, Y. P. Li, L. Liu, Q. H. Zhang, L. B. Qu and Y. J. Wu, Food Control, 2016, 60, 500–504 CrossRef.
- J. L. Yuan, S. J. Wu, N. Duan, X. Y. Ma, Y. Xia, J. Chen, Z. S. Ding and Z. P. Wang, Talanta, 2014, 127, 163–168 CrossRef PubMed.
- Q. K. Fang, L. M. Wang, Q. Cheng, Y. L. Wang, S. Y. Wang, J. Cai and F. Q. Liu, Food Anal. methods, 2015, 8, 1248–1257 CrossRef.
- M. Li, E. Z. Sheng, L. J. Cong and M. H. Wang, J. Agric. Food Chem., 2013, 61, 3619–3623 CrossRef PubMed.
- Y. Y. Zhang, M. Li, Y. Cui, X. Hong and D. L. Du, Food Anal. methods, 2018, 11, 2553–2560 CrossRef.
- J. H. Lei, W. Q. Liu, C. S. Sun, C. L. Tang, M. J. Li, Y. L. Chen and Y. L. Li, Acta Trop., 2009, 111, 39–43 CrossRef PubMed.
- X. Y. Zhang, M. R. Song, X. Z. Yu, Z. H. Wang, Y. B. Ke, H. Y. Jiang, J. C. Li, J. Z. Shen and K. Wen, Food Control, 2017a, 79, 309–316 Search PubMed.
- D. H. Zhang, P. W. Li, Q. Zhang, W. Zhang, Y. L. Huang, X. X. Ding and J. Jiang, Anal. Chim. Acta, 2009, 636, 63–69 CrossRef PubMed.
- Z. Xiao, P. W. Li, Q. Zhang, W. Zhang and X. X. Ding, Chin. J. Oil Crop Sci., 2011, 33, 66–70 Search PubMed.
- X. Li, P. W. Li, Q. Zhang, Y. Y. Li, W. Zhang and X. X. Ding, Anal. Chem., 2012, 84, 5229–5235 CrossRef PubMed.
Footnote |
† Electronic supplementary information (ESI) available. See DOI: 10.1039/c8ra04460k |
|
This journal is © The Royal Society of Chemistry 2018 |
Click here to see how this site uses Cookies. View our privacy policy here.