DOI:
10.1039/C4RA11678J
(Paper)
RSC Adv., 2015,
5, 10119-10124
A dual-functional Cd(II)–organic-framework demonstrating selective sensing of Zn2+ and Fe3+ ions exclusively and size-selective catalysis towards cyanosilylation†
Received
2nd October 2014
, Accepted 5th January 2015
First published on 5th January 2015
Abstract
A dual-functional complex with the formula Cd2(L)(DMF)2(H2O)2 (L = 2,3′,5,5′-biphenyl tetracarboxylic acid) has been successfully synthesized under solvothermal conditions and characterized by thermogravimetric analyses, IR spectroscopy, X-ray powder diffraction and single crystal X-ray diffraction. This material can selectively sense Zn2+ and Fe3+ ions over mixed metal ions through fluorescence enhancement and quenching, respectively. The cyanosilylation of aldehydes reaction studied used activated complex as a catalyst; the results showed that this complex with Lewis acid sites can act as a stable heterogeneous catalyst.
Introduction
Over the past decade, functional metal–organic frameworks (MOFs) have attracted much attention, not only for their compositional and structural diversities, but also for their potential applications in the areas of catalysis,1 luminescence,2 gas adsorption and separation,3 ion exchange,4 magnetism5 and so on. It should be noted that study on MOFs as chemosensors is one of the hot topics.6,7 Most probes are sensitive to certain metal ions such as Zn2+, Al3+ and Fe3+,8,9 which follow the principle that there exist coordination sites in the structure to postsynthetically interact with special metal ions, and then exert an effect on the luminescent intensity of the material.10
Zn2+ and Fe3+ are widely used in many areas such as industry, agriculture and especially in medicine. However, they have different influences on the human body and other biological tissues. Fe3+ ion plays an essential part in many vital cell functions such as hemoglobin formation, muscle and brain function, electron transfer processes in DNA and RNA synthesis.11 But excess amounts of Fe3+ in a living cell may cause damage to nucleic acids and proteins through catalyzing the production of reactive oxygen species.12 Zn2+ ion is the second most abundant transition metal essential for the human body with a concentration ranging from sub-nM to ∼0.3 mM.13 Recent work showed that Zn2+ is closely associated with severe pathological disease, such as Alzheimer's disease and familial amyotrophic lateral sclerosis,14 and its intracellular concentration is under tight control.15 Hence, selective detection or sensing of Fe3+ and Zn2+ over other mixed metal ions seems to be very important for human health.
The studies on MOFs as catalysts have also caused considerable attention due to their potential properties including porosity, extraordinarily robust and high internal surface area.16 The catalytic mechanism of MOFs results from the metal center in the structure, or the catalytically-active functional organic sites of the organic ligand.17 However, MOFs with Lewis acid sites could also serve as size- and shape-selective heterogeneous catalysts, which is one of the hot topics.18
In this work, we report the synthesis, structural characterization and applications of a unique porous MOF with the formula Cd2(L)(DMF)2(H2O)2 (1) using L ligand (L = 2,3′,5,5′-biphenyl tetracarboxylic acid) as organic linker. Complex 1 is sensitive to Zn2+ and Fe3+ ions without the interference of other mixed ions. More interestingly, activated 1 with Lewis acid sites shows size-selective catalytic activity toward the cyanosilylation of aldehydes.
Experimental section
Materials and methods
All reagents and solvents employed were commercially available and used without further purification. The C, H, and N microanalyses were carried out with Perkin-Elmer 240 elemental analyzer. Thermogravimetric analyses (TGA) were taken on a Perkin-Elmer Pyrisl (25–800 °C, 10 °C min−1, flowing N2 (g)). The FT-IR spectra were recorded from KBr pellets in the range 4000–400 cm−1 on a Nicolet 5DX spectrometer. X-ray powder diffraction was recorded with a Bruker AXS D8advanced automated diffractometer with Cu-Kα radiation. Luminescence spectra for the solid samples and liquid samples were investigated with a HitachiF-4500 fluorescence spectrophotometer and Varian Cary Eclipse Fluorescence spectrophotometer, respectively. The products of catalysis reaction are monitored by gas chromatography with a SP-2100A Gas chromatograph.
Solvothermal synthesis
Cd2(L)(DMF)2(H2O)2 (1). Cd(NO3)2·4H2O (25 mg, 0.26 mmol) was added to the DMF/CH3CH2OH/H2O (3
:
3
:
2, 4 ml) solution of H4L (15 mg, 0.45 mmol), and the mixture was stirred for ca. 20 min in air. Then it was heated at 75 °C for 2 days, followed by slow cooling (5 °C h−1). The resulting light yellow crystals were washed with distilled water and dried in air (yield: ca. 60%). Elemental analysis calcd (%) for 1 C22H24Cd2N2O12 (%): C: 36.01; H: 3.27; N: 3.82; found: C: 35.96; H: 3.34; N: 3.86. IR (KBr, cm−1): 3400(s), 1652(s), 1574(s), 1385(vs), 1242(w), 1108(w), 828(w), 773(w), 724(w).
X-ray crystallography
Crystallographic data of 1 were collected at 173 K with a Bruker Apex CCD II diffractometer with Mo-Kα radiation (λ = 0.71073 Å) and graphite monochromator using ω-scan mode. The structure was solved by direct methods and refined on F2 by full-matrix least squares using SHELXTL.19 This was a twinned structure and the values of Rint, provided by TWINABS, are 0.0351 and 0.0400 corresponding to the two crystal domains. Crystallographic data and experimental details for structural analyses are summarized in Table 1.†
Table 1 Crystallographic data for 1
Results and discussion
Crystal structure
The single-crystal X-ray analysis reveals that 1 crystallizes in a chiral space group P21. The asymmetric unit of 1 contains two crystallographically unique Cd(II) ions, one L ligand, two coordinated DMF molecules and two aqua ligands. As shown in Fig. 1a, the Cd1 atom is six-coordinate with five carboxylate oxygen atoms from four individual L ligands and one oxygen atom from one coordinated DMF molecule, Like Cd1, Cd2 atom is also six-coordinate with three carboxylate oxygen atoms from three individual L ligands and one oxygen atoms from one coordinated DMF molecule and two aqua ligands, respectively. Cd1 is linked to Cd2 via two μ2-η2:η1 bidentate carboxylate groups and one μ2-η1:η1carboxylate group to form a dinuclear Cd cluster. The Cd clusters are bridged by L ligands to form a three-dimensional (3D) framework. It is noted that four carboxylate groups in the L ligand exhibit three kinds of connection modes: monodentate, bidentate bridging and chelating/bridging mode and all the carboxylate groups are deprotonated (Fig. 1b). It is worth mentioning that regardless of the coordinated solvent molecules, it showed 1D channels with the size of ca. 11.4 × 9.7 Å2 along the b axis (Fig. 1c and d). With omitting the coordinated solvent molecules, PLATON analysis20 revealed that the 3D framework was composed of voids of 562.2 Å3, which represent 44.1% per unit cell volume.
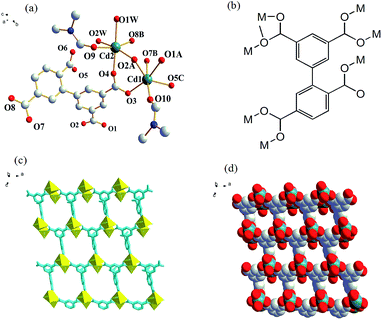 |
| Fig. 1 (a) Coordination environments of Cd(II) in 1. Symmetry mode: A: x − 1, y, z; B: x − 1, y, z − 1; C: −x, 0.5 + y, −z + 1. (b) The coordination modes of L ligand in 1. (c) 3D single network of 1 with removing coordinated DMF molecule. (d) Space-filling representation of 3D structure of 1 along the b-axis (DMF molecules were omitted for clarify). | |
Thermal stability
The simulated and experimental PXRD patterns of 1 are shown in Fig. S1a.† Their peak positions are in good agreement with each other, indicating the phase purity of the products. The differences in the intensity may be due to the preferred orientation of the powder sample.
The TGA curve (Fig. S2†) of 1 shows the first weight loss of 4.94% from ca. 29.0 °C to 118.0 °C, corresponding to the loss of two coordinated water molecules (calculated 4.91%). The weight loss of 20.44% from 118 °C to 330 °C, which corresponds to the loss of two coordinated DMF molecules (calculated 19.91%). The residue was CdO (experimental: 35.36% and calculated: 35.02%).
Photoluminescence properties
Previous studies have shown that Cd(II) coordination polymers exhibit photoluminescent properties.21,22 So we investigated the luminescent properties of 1 in the solid state and in methanol suspension at room temperature.
As expected, 1 exhibits an intense emission band at ca. 420 nm upon excitation at 368 nm (Fig. S3a†). It should be noted that there is a emission band at 420 nm for free L ligand under the same experimental conditions (Fig. S3b†). Thus the emission may be assigned to the ligand-centered π–π* transition because their fluorescent emission bands are same to that of the free ligand.23
We also investigated the effects of the metal ions such as K+, Ca2+, Na+, Fe3+, Co2+, Ni2+, Cu2+, Zn2+, Pb2+, Mg2+, Cr3+, Ag+, Mn2+, Hg2+ and Cd2+ on the fluorescent intensity of 1 in methanol suspension at room temperature. The emission intensity of 1 enhanced distinctly with Zn2+ doping in. More remarkably, with increasing Zn2+ concentration, the emission intensities of 1 increased gradually upon addition of different equiv. of Zn2+ with respect to 1 (Fig. 2). When adding 10 equiv. of Zn2+, the highest peak at 378 nm is more than twice as intense as the corresponding band in the solution without Zn2+, whereas adding Fe3+ can almost quench the fluorescence intensity of 1 and others had no evident effect on the emission intensity of 1 (Fig. 3). In order to check the high sensitivity to Zn2+ and Fe3+ over other metal ions, the experiments were performed for the introduction of other metal ions (K+, Ca2+, Na+, Co2+, Ni2+, Mg2+, Ag+, Mn2+, Hg2+) into the system. More importantly, the selectivity to Zn2+ and Fe3+ is not interfered by some other metal ions (Fig. 4 and S4 and S5†).
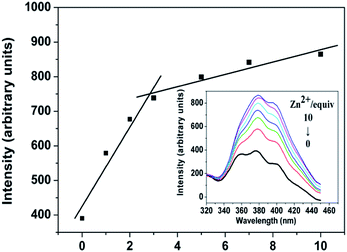 |
| Fig. 2 Emission spectra of the complex 1 in methanol at room temperature in the presence of different equiv of Zn2+ ions with respect to 1, respectively, excited at 250 nm. | |
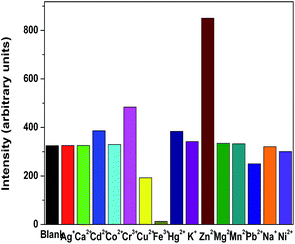 |
| Fig. 3 Room-temperature luminescent intensity of 1 at 378 nm in methanol upon addition of 10 equiv. of various metal ions (excited at 250 nm). | |
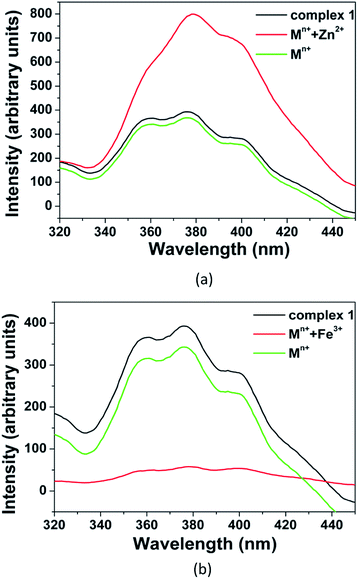 |
| Fig. 4 Comparison of the photoluminescence intensity of 1 in methanol suspension with the introduction of other metal ions (K+, Ca2+, Na+, Co2+, Ni2+, Mg2+, Ag+, Mn2+, Hg2+) in the absence and presence of 5 equiv. (a) Zn2+ (b) Fe3+. | |
As mentioned above, the exoteric Zn2+ can sensitize the luminescence of 1. The enhancement of luminescent intensity may result from more effective intramolecular energy transfer between the L ligand and the Cd2+. With the addition of Zn2+ and Fe3+, the results indicate that the energy transfer from the organic ligands to metal ions is changed, and the energy transfer is not interfered by some other metal ions.
Catalysis properties
Metal–organic frameworks as catalysts with Lewis acid sites have caused much attention due to their metallic components, which either provide the coordinatively unsaturated nodes or form the active metal complexes incorporated into the linker ligand.24 In order to probe the Lewis acid catalytical activity of complex 1 the cyanosilylation reaction was chosen. This reaction provides a convenient route to cyanohydrins, which are key derivatives in the synthesis of fine chemicals and pharmaceuticals.25 To expose the active metal sites, the coordinated molecules of complex 1 have been removed by heating to 150 °C in vacuum condition, and the block shape of the crystal did not change (see Fig. S1a†). In order to investigate the size selective catalysis of 1, we took a systematic approach by varying the size and shape of the substrates. So, five different sizes of aldehydes were performed in the cyanosilylation reaction.
A solution of equivalent amounts of cyanotrimethylsilane (4 mmol) and aldehyde (4 mmol) was mixed in CH2Cl2. This solution was added at 40 °C to suspension of activated 1 in CH2Cl2 (Scheme 1) and the progress of the reaction was monitored by using gas chromatography (see Fig. S7†). As shown in Table 2 and Fig. 5, benzaldehyde (8.9 × 6.7 Å2) had high sensitive to the catalyst. After 1.5 h, a yield of 97.44% (isolated yield: 88.65%) and a TOF value of 64.96 h−1 was achieved. Compared to the MOF Lewis catalyst, the complex 1 exhibited obvious performance (Table S1†).26 After 2 hours, n-heptaldehyde and p-anisaldehyde (10.4 × 6.1 Å2) had a yield of 96.36% (TOF: 48.18 h−1) and 94.52% (TOF: 47.26 h−1), respectively. However, for the larger size aromatic aldehydes, for example, 1-naphthaldehyde (9.6 × 8.6 Å2) and 4-benzyloxybenzaldehyde (16.1 × 6.1 Å2), only a relative low yield of 81.41% (TOF: 27.14 h−1) and 34.22% (TOF: 11.41 h−1) was achieved after 3 hours. A control filtration test was carried out to demonstrate whether the catalytic activity of the Cd metal sites is due to active sites in the solid phase or to any active species leaching into the liquid phase (Fig. S8†). Only small increase of the conversion after filtration could be attributed to a thermally activated slow reaction. We have also carried out the reaction (benzaldehyde) using Cd(NO3)2 or H4L as catalyst, respectively. The yield of 64.32% was achieved when using Cd(NO3)2 as catalyst, which is higher than the control filtration test. No activity was observed when using H4Las catalyst (Fig. S11†). This demonstrates that no homogeneous catalyst species exists in the reaction solution.
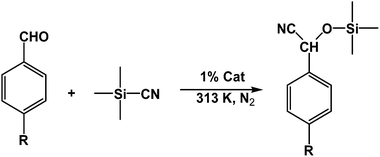 |
| Scheme 1 Cyanosilylation of aldehydes reaction catalyzed by 1. | |
Table 2 Cyanosilylation reaction catalyzed by 1
Run |
Substrate |
Time (h) |
Conversiona (%) |
TOFb (h−1) |
Conversion determined by GC. TOF: % conversion (mmol of substrate per mmol of cat. per h). |
1 |
 |
1.5 h |
97.44 |
64.96 |
2 |
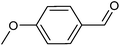 |
2 h |
94.52 |
47.26 |
3 |
 |
2 h |
96.36 |
48.18 |
4 |
 |
3 h |
81.41 |
27.14 |
5 |
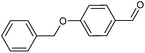 |
3 h |
34.22 |
11.41 |
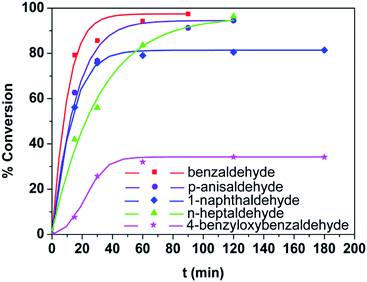 |
| Fig. 5 Conversion (%) versus time (min) for cyanosilylation for different aldehydes for 1. | |
The catalytical activity differences among the five reactions may be two reasons below: first, the reactants could access into the inside of the catalyst through the channels (11.4 × 9.7 Å2) of 1. Therefore, larger size of aldehydes were difficult to access into the channel and results in the relative low conversion and TOF value such as 4-benzyloxybenzaldehyde. Second, there were considerable active sites on the surface of the activated catalyst. So even the relative large size aldehydes, for example, 1-naphthaldehyde also performed high conversion. It should be noted that complex 1 as catalyst is easily isolated from the reaction suspension by a simple filtration. The PXRD (powder X-ray diffraction) pattern of 1 after the reactions were collected and examined, which is complete to the one before the reaction (see Fig. S1b†), indicating the stability of the catalyst. And the activity of the catalyst didn't loss after 5 cycles' reactions (Fig. S9†).
Conclusions
In summary, a new MOF based on L ligand has been successfully designed and synthesized. It exhibits strong fluorescent emission in the solid state and in methanol suspension at room temperature. 1 displayed high performance selectivity for Zn2+ and Fe3+ over other metal ions, which implies that it may be used as luminescent probes of Zn2+ and Fe3+. After removing the coordinated solvent molecules, the Lewis acid sites in the activated 1 were used as catalyst for cyanosilylation both on the surface and inside the channel of complex 1. These results will bring more attention on the potential properties of metal–organic-framework.
Acknowledgements
This work was granted financial support from National Natural Science Foundation of China (20871063, 21271096), the Program for Liaoning Excellent Talents in University (LR2011001), Liaoning Natural Science Foundation of China (201102079) and the Liaoning Innovative Team Project in University (LT2011001).
Notes and references
-
(a) J. Y. Lee, O. K. Farha, J. Roberts, K. A. Scheidt, S. T. Nguyen and J. T. Hupp, Chem. Soc. Rev., 2009, 38, 1450 RSC;
(b) J.-P. Zhang, Y.-B. Zhang, J.-B. Lin and X.-M. Chen, Chem. Rev., 2012, 112, 1001 CrossRef CAS PubMed;
(c) L. Ma, C. Abney and W. Lin, Chem. Soc. Rev., 2009, 38, 1248 RSC.
-
(a) M. D. Allendorf, C. A. Bauer, R. K. Bhaktaa and R. J. T. Houka, Chem. Soc. Rev., 2009, 38, 1330 RSC;
(b) Y. Cui, Y. Yue, G. Qian and B. Chen, Chem. Rev., 2012, 112, 1126 CrossRef CAS PubMed;
(c) J. Heine and K. Müller-Buschbaum, Chem. Soc. Rev., 2013, 42, 9232 RSC;
(d) D. I. Alexandropoulos, A. Fournet, L. Cunha-Silva, A. M. Mowson, V. Bekiari, C. Christou and T. C. Stamatatos, Inorg. Chem., 2014, 53, 3220 CrossRef CAS PubMed.
-
(a) J.-R. Li, R. J. Kuppler and H.-C. Zhou, Chem. Soc. Rev., 2009, 38, 1477 RSC;
(b) S. Chaemchuen, N. A. Kabir, K. Zhou and F. Verpoort, Chem. Soc. Rev., 2013, 42, 9304 RSC;
(c) S.-H. Yang, X. Lin, A. J. Blake, K. M. Thomas, P. Hubberstey, N. R. Champness and M. Schröder, Chem. Commun., 2008, 6108 RSC.
-
(a) J. An, C. M. Shade, D. A. Chengelis-Czegan, S. Petoud and N. L. Rosi, J. Am. Chem. Soc., 2011, 133(5), 1220 CrossRef CAS PubMed;
(b) Z.-Z. Lin, F.-L. Jiang, L. Chen, C.-Y. Yue, D.-Q. Yuan, A.-J. Lan and M.-C. Hong, Cryst. Growth Des., 2007, 7, 1712 CrossRef CAS.
-
(a) M. Kurmoo, Chem. Soc. Rev., 2009, 38, 1353 RSC;
(b) A. Bousseksou, G. Molnar, L. Salmon and W. Nicolazzi, Chem. Soc. Rev., 2011, 40, 3313 RSC;
(c) M.-H. Zeng, Z. Yin, Y.-X. Tan, W.-X. Zhang, Y.-P. He and M. Kurmoo, J. Am. Chem. Soc., 2014, 136, 4680 CrossRef CAS PubMed;
(d) Q. Chen, M.-H. Zeng, Y.-L. Zhou, H.-H. Zou and M. Kurmoo, Chem. Mater., 2010, 22, 2114 CrossRef CAS.
-
(a) N. Sabbatini, M. Guardigli and J. M. Lehn, Coord. Chem. Rev., 1993, 123, 201 CrossRef CAS;
(b) A. W. Czarnik, Acc. Chem. Res., 1994, 27, 302 CrossRef CAS;
(c) D. Parker, P. K. Senanayake and J. A. G. Williams, J. Chem. Soc., Perkin Trans. 2, 1998, 2129 RSC.
-
(a) P. Wardman, Free Radical Biol. Med., 2007, 43, 995 CrossRef CAS PubMed;
(b) B. Gole, A. K. Bar and P. S. Mukherjee, Chem. Commun., 2011, 47, 12137 RSC;
(c) J. Cui, Z. Lu, Y. Li, Z. Guo and H. Zheng, Chem. Commun., 2012, 48, 7967 RSC.
-
(a) S. Dang, E. Ma, Z. M. Sun and H. J. Zhang, J. Mater. Chem., 2012, 22, 16920 RSC;
(b) K. Jayaramulu, R. P. Narayanan, S. J. George and T. K. Maji, Inorg. Chem., 2012, 51, 10089 CrossRef CAS PubMed.
- Z. Chen, Y.-W. Sun, L.-L. Zhang, D. Sun, F.-L. Liu, Q.-G. Meng, R.-M. Wang and D.-F. Sun, Chem. Commun., 2013, 49, 11557 RSC.
-
(a) W. Liu, T. Jiao, Y. Li, Q. Liu, M. Tan, H. Wang and L. Wang, J. Am. Chem. Soc., 2004, 126, 2280 CrossRef CAS PubMed;
(b) B. Zhao, X.-Y. Chen, P. Cheng, D.-Z. Liao, S.-P. Yan and Z.-H. Jiang, J. Am. Chem. Soc., 2004, 126, 15394 CrossRef CAS PubMed.
-
(a) N. C. Andrews, N. Engl. J. Med., 1999, 341, 1986 CrossRef CAS PubMed;
(b) A. Barba-Bon, A. M. Coster and S. Gil, Chem. Commun., 2012, 48, 3000 RSC;
(c) J. L. Bricks, A. Kovalchuk, C. Trieflinger, M. Nofz, M. Buschel, A. I. Tolmachev, J. Daub and K. Rurack, J. Am. Chem. Soc., 2005, 127, 13522 CrossRef CAS PubMed.
- G. Alez, Iron Overload: Haemosiderosis, Causes, Primary and Secondary Haemochromatosis, Treatment, and More, Webster's Digital Services, 2012, pp. 1–204 Search PubMed.
- S. J. Lippard and J. M. Berg, Principles of Bioinorganic Chemistry, University Science Book, Mill Valley, CA, 1994, pp. 10, 14 and 78–183 Search PubMed.
- A. I. Bush, Curr. Opin. Chem. Biol., 2000, 4, 184 CrossRef CAS.
- C. E. Outten and T. V. O'Halloran, Science, 2001, 292, 2488 CrossRef CAS PubMed.
-
(a) S. H. Cho, B. Ma, S. T. Nguyen, J. T. Hupp and T. E. Albrecht-Schmitt, Chem. Commun., 2006, 2563 RSC;
(b) C. D. Wu, A. Hu, L. Zhang and W. Lin, J. Am. Chem. Soc., 2005, 127, 8940 CrossRef CAS PubMed;
(c) S. Horike, M. Dinca, K. Tamaki and J. R. Long, J. Am. Chem. Soc., 2008, 130, 5854 CrossRef CAS PubMed;
(d) Q. Han, X. Sun, J. Li, P. Ma and J. Niu, Inorg. Chem., 2014, 53, 6107 CrossRef CAS PubMed.
-
(a) A. R. Burgoyne and R. Meijboom, Catal. Lett., 2013, 143, 563 CrossRef CAS;
(b) Y. Hwang, D.-Y. Hong, J.-S. Chang, S. H. Jhung, Y.-K. Seo, J. Kim, A. Vimont, M. Daturi, C. Serre and G. Férey, Angew. Chem., Int. Ed., 2008, 47, 4144 CrossRef CAS PubMed.
-
(a) M. Fujita, Y. J. Kwon, S. Washizu and K. Ogura, J. Am. Chem. Soc., 1994, 116, 1151 CrossRef CAS;
(b) D. Dang, P. Wu, C. He, Z. Xie and C. Duan, J. Am. Chem. Soc., 2010, 132, 14321 CrossRef CAS PubMed;
(c) A. Henschel, K. Gedrich, R. Kraehnert and S. Kaskel, Chem. Commun., 2008, 4192 RSC;
(d) M. R. Lohe, K. Gedrich, T. Freudenberg and E. Kockrick, Chem. Commun., 2011, 47, 3075 RSC.
- SHELXTL 6.10, Bruker Analytical Instrumentation, Madison, Wisconsin, USA, 2000 Search PubMed.
- V. A. Blatov, L. Carlucci, G. Ciani and D. M. Proserpio, CrystEngComm, 2004, 6, 377 RSC.
-
(a) X. Zhang, H. Gorohmaru, M. Kadowaki, T. Kobayashi, T. Ishi-i, T. Thiemann and S. Mataka, J. Mater. Chem., 2004, 14, 1901 RSC;
(b) S.-N. Wang, Coord. Chem. Rev., 2001, 215, 79 CrossRef CAS;
(c) N. Wei, Y.-R. Zhang and Z.-B. Han, CrystEngComm, 2013, 15, 8883 RSC.
-
(a) G. Yu, S. Yin, Y. Liu, Z. Shuai and D. Zhu, J. Am. Chem. Soc., 2003, 125, 14816 CrossRef CAS PubMed;
(b) Y.-P. Tong, S.-L. Zheng and X.-M. Chen, Eur. J. Inorg. Chem., 2005, 3734 CrossRef CAS;
(c) N. Wei, M.-Y. Zhang, X.-N. Zhang, G.-M. Li, X.-D. Zhang and Z.-B. Han, Cryst. Growth Des., 2014, 14(6), 3002 CrossRef CAS.
-
(a) J. Wu, H. W. Hou, H. Y. Han and Y. Fan, Inorg. Chem., 2007, 46, 7960 CrossRef CAS PubMed;
(b) Z. Z. Lai, R. B. Fu, S. M. Hu and X. T. Wu, Eur. J. Inorg. Chem., 2007, 34, 5439 CrossRef;
(c) J. L. Song, H. H. Zhao, J. G. Mao and K. R. Dunbar, Chem. Mater., 2004, 16, 1884 CrossRef CAS.
-
(a) Q.-R. Fang, D.-Q. Yuan, J. Sculley, J.-R. Li, Z.-B. Han and H.-C. Zhou, Inorg. Chem., 2010, 49, 11637 CrossRef CAS PubMed;
(b) J. Gascon, U. Aktay, M. D. Hernandez-Alonso, G. P. M. van Klink and F. Kapteijn, J. Catal., 2009, 261, 75 CrossRef CAS PubMed;
(c) J. Canivet, S. Aguado, Y. Schuurman and D. Farrusseng, J. Am. Chem. Soc., 2013, 135, 4195 CrossRef CAS PubMed.
-
(a) K. Higuchi, M. Onaka and Y. Izumi, Bull. Chem. Soc. Jpn., 1993, 66, 2016 CrossRef CAS;
(b) R. J. H. Gregory, Chem. Rev., 1999, 99, 3649 CrossRef CAS PubMed;
(c) M. North, Tetrahedron: Asymmetry, 2003, 14, 147 CrossRef CAS;
(d) B. Thirupathi, M. K. Patil and B. M. Reddy, Appl. Catal., A, 2010, 384, 147 CrossRef CAS PubMed.
-
(a) K. Schlichte, T. Kratzke and S. Kaskel, Microporous Mesoporous Mater., 2004, 73, 81 CrossRef CAS PubMed;
(b) R. F. D'Vries, V. A. Peña-O'Shea, N. Snejko, M. Iglesias, E. Gutiérrez-Puebla and M. A. Monge, J. Am. Chem. Soc., 2013, 135, 5782 CrossRef PubMed;
(c) G. Kumar and R. Gupta, Inorg. Chem., 2013, 52, 10773 CrossRef CAS PubMed;
(d) P. Phuengphai, S. Youngme, P. Gamez and J. Reedijk, Dalton Trans., 2010, 39, 7936 RSC.
Footnote |
† Electronic supplementary information (ESI) available: Experimental procedures, structure figures, a TGA plot, simulated and experimental X-ray powder diffraction patterns, fluorescence studies, catalysis studies and crystallographic data (CIF) for 1. CCDC 1014278. For ESI and crystallographic data in CIF or other electronic format see DOI: 10.1039/c4ra11678j |
|
This journal is © The Royal Society of Chemistry 2015 |