DOI:
10.1039/C4QI00189C
(Review Article)
Inorg. Chem. Front., 2015,
2, 10-27
Magnetic clusters based on octacyanidometallates
Received
30th October 2014
, Accepted 25th November 2014
First published on 26th November 2014
Abstract
Octacyanidometallates make an important branch of cyanide-based molecular magnets that not only follow the trends in modern magnetochemistry and materials science but also have stimulated these fields from the very beginning and still blaze a trail by introducing new concepts such as heterotrimetallic systems, new functionalities and cross-effects like photo-switching of magneto-optical properties and setting new records in magnetic ordering temperatures or magnetic exchange interactions. The following paper focuses on a special class of molecular magnets which, to the best of our knowledge, have not been reviewed so far: octacyanide-based magnetic clusters. A complete list of all known molecules incorporating octacyanides with an extensive discussion of their structures, topologies and magnetic properties, with special attention paid to multifunctional systems, is provided. Several milestone-clusters are discussed thoroughly to emphasize their particular importance in the development of crystal engineering and molecular magnetism.
 Dawid Pinkowicz | Dawid Pinkowicz has been an Associate Professor at Jagiellonian University, Faculty of Chemistry since 2012. He received his PhD from Jagiellonian University with Prof. Barbara Sieklucka and then moved to Prof. Masahiro Yamashita's group for the research project ‘Photo-Switchable Single-Molecule Quantum Magnets’ within the Matsumae International Fellowship Program. Afterwards he joined Prof. Kim Dunbar for the research project ‘Multifunctional Molecular Materials through Cyanide Chemistry’ within the Marie Curie International Outgoing Fellowship funded by the European Commission within the 7th Framework Programme. His recent research interests cover the design of tailor-made functional ligands for the construction of multifunctional molecular materials. |
 Robert Podgajny | Robert Podgajny received his PhD from Jagiellonian University in 2002. He developed his skills in molecular magnetism at P. & M. Curie University in Paris and in handling metallic nanoparticles in LCC CNRS in Toulouse (France). In 2013 he received his habilitation (post-doctoral) degree from Jagiellonian University. His area of expertise involves crystal engineering of magnetic coordination polynuclear assemblies. Currently he is developing methods for construction of molecular materials using polynuclear clusters of tuneable composition, structure and magnetic properties, and studying the role of non-covalent anion–π interactions. |
 Beata Nowicka | Beata Nowicka completed her PhD in coordination chemistry at Jagiellonian University in 1998. She gained experience from the synthetic chemistry laboratories at the University of Erlangen-Nürnberg in Germany and the Institute of Physical and Chemical Research (RIKEN) in Japan. In 2005 she joined the Inorganic Molecular Materials Group and she is now working on guest-responsive porous magnetic networks. |
 Szymon Chorazy | Szymon Chorazy received his PhD from Jagiellonian University, Faculty of Chemistry in 2014 for the investigation of multifunctional chiral, luminescent, and spin transition molecule-based magnets based on polycyanidometallates. During the PhD studies, he realized the International PhD Study Programme in the cooperation between Jagiellonian University (Inorganic Molecular Materials Group, Prof. Barbara Sieklucka) and The University of Tokyo (Solid State Physical Chemistry Group, Prof. Shin-ichi Ohkoshi). He is now continuing his research within this cooperation, investigating a variety of luminescence, non-linear optical and spin transition phenomena in the magnetically ordered state of novel cyanido-bridged coordination polymers. |
 Mateusz Reczyński | Mateusz Reczyński is a PhD student and a member of the Inorganic Molecular Materials Group. His area of interest includes the study of the stimulus–response relationship in dynamic molecular networks based on polycyanometallates and 3d-ion complexes. He is a grantee of the Diamond Grant Programme aimed at accelerating the scientific career of outstanding students in Poland. |
 Barbara Sieklucka | Barbara Sieklucka is a Full Professor of Inorganic Chemistry at Jagiellonian University in Krakow, Poland and the Head of the Inorganic Molecular Materials Group, a co-founder of the European Institute of Molecular Magnetism and a co-author of 106 research papers. Her research focuses on the crystal engineering of highly structured functional magnetic materials on the basis of polynuclear cyanido-bridged coordination compounds, which allow imposing specific functionalities (dynamics, sorption, magnetism, photomagnetism, porosity, luminescence, chirality, non-linear optics etc.) on the target material, with the ultimate goal of achieving multifunctionality and efficient engineering of the nanospace within the crystal network. |
1. Introduction
Molecular magnetic clusters1–5 are obtained through chemical assembly of appropriate geometrically restricted spin-carriers, organic radicals (p-centres)6,7 and/or paramagnetic metal ions (d- or f-centres),8–10 connected in such a way that they form closed discrete structures with magnetic interactions transmitted through intervening overlapping magnetic orbitals of diamagnetic bridges (superexchange) or directly (exchange). In addition to paramagnetic building blocks other molecules bearing various additional functionalities, chirality,11 redox activity,12,13 photochromism14 or luminescence,15,16 can be incorporated. Proper ‘chemical blend’ of functional units can lead to a multifunctional molecule/compound,17 which apart from interesting magnetism may also exhibit additional properties in response to external factors including light,18–21 pressure,22–25 electric field,26–29 and guest molecules/solvent vapors.21,30–34 Research on multifunctional magnetic molecules that incorporates basic concepts of chemistry, physics, and materials science has much to offer to the future of science and technology and the elaboration of new types of devices on the nanoscale. The evolution of the field has been described in detail in several reviews and books.2,5,35–52
The modular approach53 is the most efficient strategy that allows the construction of larger molecules ‘by design’ from specific molecular fragments with properties strongly influenced by individual molecular modules. This step-wise method enables the synthesis of families of structurally related multinuclear molecules with tailored magnetic, electronic, optical and redox properties. Cyanidometallates are perfect candidates for such a modular strategy because of a large variety of known stable homoleptic cyanide complexes, which can be used to link to other metal centres resulting in basically an infinite number of heterometallic54,55 combinations. Moreover, the cyanide anion is well known for providing short and effective pathways for ferromagnetic or antiferromagnetic superexchange interactions56 between metal ions depending on the symmetry of the overlapping magnetic orbitals in the linear or bent M–CN–M′ linkage.50,57–67 The ligand field at each metal centre and therefore their electronic properties can be controlled by the choice of suitable blocking ligands. Indeed this strategy has led to well-defined and robust multinuclear molecules that mimic extended Prussian blue analogs and allowed the systematic evaluation and modeling of properties due to their discrete nature.60,66,68–70 Octacyanidometallates are a specific class of cyanidometallates which are able to form up to eight CN-bridges with other metal centres and, unlike other cyanide complexes, can easily adopt various geometries, which allows uncommon topologies of the resulting CN-bridged assemblies.71,72 The topology of the heterometallic molecular assemblies is usually controlled by the auxiliary ligands at the metal centre (blocking and bridging).73–75
In the following contribution we will focus on clusters based on all octacyanidometallates: [NbIV(CN)8]4−, [MoIV/V(CN)8]4−/3−, [WIV/V(CN)8]4−/3− and [ReV(CN)8]3−. They will be reviewed according to their topology-class with particular attention paid to their functionality. Throughout this review a multinuclear molecule being ‘a group of metal centres with direct or indirect interactions i.e. through bridging ligands’ will be referred to as a cluster.76
2. Topology
Heterometallic cyanido-bridged clusters show a variety of topologies, which depend mostly on (i) the coordination number and geometry of the polycyanidometallate, (ii) the presence of blocking ligands decreasing the number of bridging CN− groups, (iii) the number and spatial arrangement of labile ligands on the cationic building block, and (iv) relative charges of the building blocks. The most common cluster topologies are presented in Fig. 1. They comprise simple and decorated squares (for 4–8 metal centres), trigonal bipyramids (5), octahedra (6), cubes (8), face-centred cubes (14) and six-capped body-centred cubes (15).
 |
| Fig. 1 Typical topologies of cyanido-bridged clusters (cyanidometallate [M(CN)x] centres – blue spheres, secondary transition metal centres [M′(L)y(solv)z] – red spheres). | |
The simplest M′2M2square motif composed of two cyanidometallate anions M and two metal cations M′ is very common. It can be encountered in clusters based on planar tetracyanidometallates,77–79 as well as hexa-80–86 and octacoordinated87–93 cyanido complexes, both with or without blocking ligands at M′ centres. Due to the rigid geometry and 90° angle between cis-CN groups in square-planar and octahedral complexes, the clusters based on tetra- and hexacyanidometallates are usually regular squares.77,80,81,94 In some cases distortions are caused by the presence of bulky blocking ligands.79,95–98 For octacyanidometallate-based squares, out-of-plane distortion to the rhombic or rhomboid shape is a rule, as the angle between CN-bridges is lower than 90°. Square clusters based on divalent metal cations M′ and [M(CN)6/8]3−/4− ions are negatively charged and require the presence of additional cations in the structure. Neutral square clusters are formed if two CN groups are replaced by neutral ligands in hexacyanidometallates(II)99 or octacyanidometallates(IV).100 The square motif often appears in larger clusters composed of two101 or three89vertex-sharing squares and/or containing one or two side-chains.101 The so-called decorated squares may have single additional ion bound to cyanidometallates or cationic building blocks,101–106 but sometimes the side-chains consist of two107 or three108 elements.
Pentanuclear trigonal bipyramid clusters M′3M2 are typically composed of two axially arranged polycyanidometallates forming bridges with three equatorially located octahedral metal complexes with four coordination sites blocked by chelating ligands. In this arrangement the cyanidometallate bridges through three facial CN ligands, therefore this kind of cluster is easily formed by hexacyanidometallates, as well as hexa-coordinated tricyanidometallates. This topology is very common for [M(CN)6]3− ions with divalent 3d metals, as it results in neutral clusters. For octacyanidometallates there are only two known isomorphic trigonal bipyramids, both [Ni(tmphen)2]3[M(CN)8]2.109 Among larger clusters this topological motif appears in the form of decorated and vertex-sharing trigonal bipyramids. Interestingly, both apical110 and equatorial111 vertex-shared structures are known. In the first case the connecting metal centre is a hexacyanidometallate, in the second case it is the tetrahedral Co2+ ion.
Octahedral topology is unique for hexanuclear clusters based on octacyanidometallates. This topology requires a building block with four CN-bridges in facial arrangement, which cannot be achieved in hexacyanidometallates. The known structures are neutral clusters of the M′4M2 type that consist of two [M(CN)8]4− ions in axial positions bound to four divalent M′ cations in the equatorial plane.112–116
Conversely, the topology of a cube is attainable only for octahedral hexa-coordinated cyanidometallates. In M′4M4 clusters metal ions are placed alternatingly in the corners of a cube, with each metal connected to three neighbours. This topology is most often realised by the use of octahedral tricyanidometallates.117–124 Ligands blocking three facial positions at the cationic building blocks118,125–128 or pentadienyl tricyanido-stool-type complexes are also utilised.98,129–132 Cube-shaped cluster structures are numerous. They are widely studied as models of Prussian-blue type structures. A variation of this topology is shown by vertex sharing cubes of the M′7M8 type.133
Among larger clusters, comprising more than ten metal centres, the most common is the highly symmetrical topology of face-centred cubes. It is characteristic of M′8M6 type clusters, where eight metal cations are placed at the corners of a cube and each is connected to three cyanidometallates located at the six faces, thus forming an octahedron. This type of cluster is often formed by planar tetracyanidometallates.119,134–137 The same topology can be realised by reversed placement of building blocks in M′6M8 clusters. In this type of structure eight hexa-coordinated cyanidometallates containing a tridentate facial blocking ligand occupy the corners of the cube. The six cations at the centres of each face can have octahedral138 or square-pyramid coordination geometry.119,139 If the face-centred metal ions have no ligand pointing inside the cube (for square-planar or square-pyramid geometry), clusters with this topology can accommodate guest molecules inside.119,134 Like in the case of trigonal bipyramid or cube topology, there is also an example of a larger cluster composed of vertex-sharing face-centred cubes.140
The face-centred cube topology clusters are not known to form octacyanidometallates. However, [M(CN)8]3− ions combined with divalent cations in alcoholic media commonly form pentadecanuclear clusters M′9M6, which can be treated as an equivalent of face-centred cube topology appended by one additional cation inside. In this type of cluster M′ cations located at the corners of the cube are connected to three octacyanidometallates each. The [M(CN)8]3− anions, usually in distorted dodecahedral coordination geometry, are located above the faces of the cube, therefore the topology is more accurately described as a six-capped cube rather than a face-centred cube. Three CN groups of each octacyanidometallate point outside the cube and one inside the cube, binding the additional cation in the centre. The body-centred six-capped cubic topology is unique to octacyanidometallates. Above twenty characterised structures make M′9M6 type clusters the most abundant group among discrete octacyanidometallate-based assemblies. Additionally, the clusters, which are stable in solution, have been employed as secondary building units (SBU) for the construction of extended structures.141–143
The second largest cluster type based on octacyanidometallates is octadecanuclear M′12M6 assembly, which was obtained by the use of a tetradentate macrocyclic Schiff base blocking ligand on Ni(II). Two such structures based on Nb(IV) and W(IV), respectively, are known.144,145 They show the topology of the hexadecorated dodecanuclear ring.
3. Discussion of the topology and properties
3.1 Squares and square-motif based clusters
Molecular squares are the simplest examples of polycyanidometallate-based clusters. The M′2M2 core, where metal centres are connected with CN bridges, can be expanded to more complex discrete systems containing more than four metal centres, such as decorated146 or vertex-sharing89,114 squares, or more intricate metal-capped squares,147,148 where additional metal ions are incorporated in compartmental ligands. The M′2M2 motive can also be recognised in almost every kind of cluster incorporating octacyanidometallate moieties. All square-based clusters are gathered in Table 1.
Table 1 Molecular squares and square-motive based clusters
Centres |
Formula/metallic core |
Shape |
Functionality |
Ref. |
Type |
Description |
HL1 = pentadentate ligand – see ref. 73.
L2 = bis(2-aminoethyl)amine.
|
4 |
[Cu(phen)3]2{[Cu(phen)2]2[W(CN)8]2}ClO4·10H2O/Cu2W2 |
Square |
— |
— |
88
|
[Mn(tptz)(ac)(H2O)2]2{[Mn(tptz)(MeOH)1.58(H2O)0.48]2[W(CN)8]2}·5MeOH·9.85H2O/Mn2W2 |
HSM |
S = 8/2 |
89
|
[Co(tpm)2]{[Co(H2O)(tpm)]2[W(CN)8]2}·4H2O/Co2W2 |
HSM |
S = 8/2 |
90
|
[Mn(tpm)2]{[Mn(H2O)(tpm)]2[W(CN)8]2}·4H2O/Mn2W2 |
Spin-glass |
τ
0 = 7.1 × 10−16 s ΔE/kB = 63.1 K |
90
|
{[Ni(HL1a)]2[W(CN)8]2}·5H2O/Ni2W2 |
HSM |
S = 8/2 |
91
|
{[Mn(hmp)2]2Cl2}{[Mn(hmp)2W(CN)8]2}·5H2O/Mn2W2 |
HSM |
S = 8/2 |
149
|
{[Mn(bpy)2]2(ox)}{[Mn(bpy)2W(CN)8]2}·4H2O/Mn2W2 |
Spin-glass |
τ
0 = 6.3 × 10−15 s ΔE/kB = 63.4 K |
92
|
[Mn(phen)3]2[Mn(phen)2(μ-NC)2W(CN)6]2(ClO4)2·9H2O/Mn2W2 |
— |
— |
93
|
K2{[Co(tren)]2[W(CN)8]2}·9H2O/Co2W2 |
— |
— |
87
|
{[Ni(bpy)2]2[W(CN)6(bpy)]2}·12H2O/Ni2W2 |
— |
— |
100
|
{[Cu(dpa)]2[W(CN)6(bpy)]2}·8H2O/Cu2W2 |
— |
— |
100
|
{[Fe(bik)2]2[Mo(CN)8]2}(HMeIm)2·5H2O/Fe2Mo2 |
SCO/photomagnetism |
Gradual |
150
|
6 |
[Co(bik)3]{[W(CN)8]3[Co(bik)2]3}·2H2O·13MeCN/Co3W3 |
Decorated square |
CTIST/photomagnetism |
Gradual |
146
|
{[Mn(tptz)(MeOH)(NO3)]2[Mn(tptz)(MeOH)(DMF)]2 [W(CN)8]2}·6MeOH/Mn4W2 |
Double-decorated square |
HSM |
S = 18/2 |
89
|
{Cu(L2b)]2[Cu(tren)]2[Mo(CN)8]2}·12H2O/Cu4Mo2 |
— |
— |
151
|
{[((valen)M′)Ln(OH2)2(μM(CN)8)]2·2[M′′(tpy)2]}(ClO4)2 (Ln = GdIII, TbIII; M′ = CuII, NiII; M = MoIV, WIV; M′′ = NiII, RuII, OsII)/M′2Ln2M2 |
Metal-capped square |
SMM |
— |
147
|
9 |
[{Mn(phen)2}6{M(CN)8}3(H2O)2]·nH2O (M = W, Nb; n = 21, 24)/Mn6M3 |
Double decorated two vertex-sharing squares |
HSM (M = Nb) |
S = 27/2 |
114
|
10 |
{[Mn6(tptz)6(MeOH)4(DMF)2W4(CN)32]}·8.2H2O·2.3MeOH/Mn6W4 |
Three vertex-sharing squares |
HSM |
S = 26/2 |
89
|
{[((valen)M)Tb(H2O)2]4[Mo(CN)8]2}[Mo(CN)8]·nH2O/(M = NiII, CuII; n = 21, 19)/Cu4Tb4Mo2 |
Double-decorated metal-capped square |
SMM (M = Cu) |
τ
0 = 2.12 × 10−6 s ΔE/kB = 19.3 K |
148
|
Simplicity and finite sizes of square-core based systems make them excellent objects for theoretical studies and modelling of magnetic exchange between metal centres through the cyanido bridge and intermolecular magnetic interactions. Several models explaining experimental data were proposed and investigated with the spin Hamiltonian,88,90,114 many-body electronic model Hamiltonian91 or DFT calculations88etc. The comparison of calculation results with structural details gives better understanding of magneto-structural correlations which allows prediction of the character of magnetic interactions.
Most of the square-motive based clusters are high spin molecules. The highest value of the ground spin state S = 27/2 was observed for the nonanuclear {[Mn(phen)2]6[Nb(CN)8]3(H2O)2} cluster114 exhibiting unique topology of double-decorated two vertex-sharing squares. SMM behaviour was observed in a large group of hexanuclear M′2Ln2M2 (M′ = Ni, Cu; M = Mo, W; Ln = Gd, Tb) assemblies with metal-capped square topology, where lanthanide centres from the Ln2M2 core are coordinated by a complex of the valen ligand and M′.147 M′2Ln2M2 species were applied to obtain a new wide class of isostructural hetero-tetrametallic {[((valen)M′)Ln(OH2)2(μ-M(CN)8)]2·2[M′′(tpy)2]}(ClO4)2 systems (M′′ = Ni, Ru, Os; Fig. 2) exhibiting field-induced SMM behaviour at 1.6 kOe, with τ0 = 3.93 × 10−8 s and ΔE/kB = 16.8 K for tungsten derivatives.
 |
| Fig. 2 Structural diagram showing the structure of a heterotetrametallic system {[((valen)M′)Ln(OH2)2(μ-M(CN)8)]2·2[M′′(tpy)2]}(ClO4)2 with four different metal centres.147 | |
The M′2Ln2M2 unit was also observed in another SMM {[((valen)Cu)Tb(OH2)2]4[Mo(CN)8]2}[Mo(CN)8] system,148 where the central metal-capped square is decorated with two [((valen)Cu)Tb(H2O)2] units connected by CN bridges. Its SMM character is described by the relaxation time τ0 = 2.12 × 10−6 s and the magnetisation reversal barrier ΔE/kB = 19.3 K. The frequency dependence of the AC magnetic susceptibility was also noted in two systems incorporating MnII2WV2 molecular squares.90,92 Its character could be rather described as a spin-glass behaviour rather than an SMM one.
There are two examples of photo-switchable square-motif-based compounds: [Co(bik)3]{[W(CN)8]3[Co(bik)2]3}·2H2O·13MeCN146 and {[Fe(bik)2]2[Mo(CN)8]2}(HMeIm)2.5H2O.150 The structure of [Co(bik)3]{[W(CN)8]3[Co(bik)2]3}·2H2O·13MeCN contains a kite-like (square decorated with a bimetallic tail) mixed-valence {(CoII/IIIWIV/V)2CoIIIWV} molecule (Fig. 3). This complex exhibits thermally induced electron transfer in CoII/III–WIV/V pairs accompanied by the HS ↔ LS transition on Co centres. Interestingly, the transition occurs only on metal centres within the square core. The low-temperature {(CoIIILSWIV)2CoIIILSWV} form exhibits a significant photomagnetic effect upon irradiation at various wavelengths. The proposed mechanism suggests photo-induced CTIST from the CoIIILS–WIV to CoIIHS–WV state. {[Fe(bik)2]2[Mo(CN)8]2}(HMeIm)2.5H2O, on the other hand, shows a gradual thermal SCO transition and photomagnetic effect based on LIESST occurring at the FeII centres.
 |
| Fig. 3 A kite-like molecule of {[W(CN)8]3[Co(bik)2]3}·2H2O·13MeCN.146 | |
3.2 Trigonal bipyramidal clusters
Trigonal bipyramidal clusters of the M′3M2 type based on hexacyanidometallates53,68,109,118,152 exhibit numerous magnetic and optical functionalities, including high spin molecules, SMM behaviour, thermal- and photo-induced SCO and CTIST phenomena and linkage isomerism. Among octacyanidometallate-based clusters there are only two examples of trigonal bipyramid topology: {[Ni(tmphen)2]3[W(CN)8]2}·MeOH·2H2O and its Mo(V) analogue.109 In both clusters ferromagnetic interactions between Ni(II) and M(V) centres result in the ground spin state S = 4, however no SMM behaviour was detected. The [Ni(tmphen)2(NC)2] units are chirality centres. Similar to many hexacyanidometallate-based clusters with diimine blocking ligands they adopt one configuration within one cluster resulting in chiral ΔΔΔ or ΛΛΛ clusters. Unfortunately both enantiomers are present in the centrosymmetric lattice, which excludes the possibility to observe the optical activity of these compounds. However, trigonal bipyramidal clusters form a great molecular platform for the implementation of chirality in magnetic materials and possible observation of magneto-optical phenomena. Their potential in that field should be exploited.
3.3 Octahedral clusters
Octahedral clusters (also referred to as hexanuclear cages; Fig. 4) are specific for non-rigid octacyanidometallates and are not known for other polycyanidometallates due to the geometrical restrictions. Their design and synthesis relies on the same principles as in the case of trigonal bipyramidal clusters discussed in the previous section. Combination of the divalent 3d transition metal complexes M′ with two chelating blocking groups and two ‘open coordination sites’ in cis-geometry [M′(L)(solv)2]2+ with the flexible octacyanidometallate(IV) [M(CN)8]4− allows the formation of 90°-bent M′–CN–M linkages and consecutively closed CN-bridged architectures – octahedrons, where apical positions are occupied by octacyanidometallates and the equatorial ones – by 3d transition metals.
 |
| Fig. 4 Fragment of the crystal structure of {[MnII(bpy)2]4[MoIV(CN)8]2} showing the overall geometry and topology typical of the hexanuclear octahedral cluster family.112 | |
There are only three isostructural octahedral clusters known so far featuring [MnII(bpy)2] and [M(CN)8] (M = Mo,112,113 W,112,113 Nb114) moieties as building blocks. Note that {[MnII(bpy)2]4[MoIV(CN)8]2} has been reported twice after its discovery by Sieklucka et al. in 2000112 with different crystallization solvent contents.115,116
Chronologically, {[MnII(bpy)2]4[MoIV(CN)8]2} and {[MnII(bpy)2]4[WIV(CN)8]2} are the two octahedral clusters that were reported first. Their magnetic properties, however, are rather trivial due to the diamagnetism of octacyanidomolybdate(IV) and octacyanidotungstate(IV). Both show paramagnetic behaviour typical of MnII (S = 5/2) centres with very weak inter/intramolecular antiferromagnetic interactions between them (through space or through the diamagnetic –NC–Mo–CN– linkage). Both compounds have been subjected to detailed photomagnetic studies which revealed a strong photomagnetic effect upon UV light irradiation (Fig. 5).113 The observed photo-induced magnetization was rationalized on the basis of inter-valence charge transfer from the MnII–NC–MIV to the MnI–NC–MV motif involving an intra-ligand π*←π transition within the [MnII(bpy)2(μ-NC)2] moiety.
 |
| Fig. 5 ‘Photomagnetic effect’ for {[MnII(bpy)2]4[MoIV(CN)8]2} in the form of magnetic susceptibility vs. UV light irradiation time dependence showing the characteristic increase when the light is switched off after ca. 16 h of constant irradiation at T = 10 K and HDC = 5 kOe. | |
The third octahedral cluster {[MnII(bpy)2]4[NbIV(CN)8]2}114 is a high-spin molecule with S = 18/2 ground state. Such high total spin is due to significant antiferromagnetic interactions between the two apical NbIV and four equatorial MnII centres estimated to be −9.1 cm−1 (with the exchange coupling Hamiltonian defined as H = −2J12S1S2). Molecules with such a high spin ground state can be used for magnetic cooling at very low temperatures.3
3.4 Pentadecanuclear clusters
3.4.1 Molecular structures.
A family of pentadecanuclear six-capped-body-centred cube clusters are easily formed without the polydentate chelating ligands required for other cluster types. The general exact formula of the solvated clusters is {M′[M′(solv)3]8[M(CN)8]6}, or {M′9M6} in short, where M′ represents divalent 3d metal ions and M represents pentavalent 4d or 5d ions forming octacyanidometallate complexes (M = WV MoV or ReV; Fig. 6 and 1). The central M′ ions coordinate six [M′(μ-CN)5(CN)3]3− units with M centres located in the corners of an octahedron. Each face of the octahedron is capped by a fac-[M′(μ-NC)3(solv)3] moiety with M′ centres located in the corners of a concentric cube. Clusters with the following combination of metal centres have been reported: M′ = MnII, FeII, CoII or NiII and M = WV, MoV or ReV (Table 2).153–157 The use of FeCl2·4H2O and CoCl2·6H2O in the same synthesis has been demonstrated to result in a mixed-metal trimetallic {Fe6Co3W6}158 analogue. The diamagnetic [ReV(CN)8]3− has also been used by Long and co-workers for substitution of paramagnetic [M(CN)8]3− to give bimetallic {Mn9Re6} and mixed-metal trimetallic {M′9M6−xRex} clusters.159
 |
| Fig. 6 The molecular structure of the solvated pentadecanuclear six-capped-body-centred cubes {M′[M′(ROH)3]8[M(CN)8]6}. | |
Table 2 Molecular pentadecanuclear six-capped-body-centred cubes and higher nuclearity clusters
Formula/metallic core |
Topology |
Functionality |
Ref. |
Type |
Description |
It was later proved that intracluster MnII–MoV coupling is rather of AF nature leading to SGS = 39/2 as shown for analogous Mn9W6.159,164
It was later proved that intracluster CoII–WV and CoII–MoV coupling is rather of F nature leading to SGS = 15/2 assuming the effective CoII spin of 1/2 with an isotropic average g-value of 13/3 operating at low temperatures.143
It was later shown that intercluster CoII–WV and CoII–MoV couplings are rather of F nature with the effective CoII spin of 1/2 with an isotropic average g-value of 13/3 operated for CoII at low temperatures.143
|
15-centred clusters with solvent blocking ligands, 15 compounds
|
{Mn[Mn(EtOH)3]8[W(CN)8]6}·12EtOH/Mn9W6 |
Six-capped body centred cube |
HSM |
MnII–WV AF coupling leading to SGS = 39/2 |
153
|
{Mn[Mn(MeOH)3]8[Mo(CN)8]6}·5MeOH·2H2O/Mn9Mo6 |
Six-capped body centred cube |
HSM |
MnII–MoV F leading to SGS = 51/2a |
154
|
{Mn[Mn(EtOH)3]8[Mo(CN)8]6}·6EtOH·3iPrOH·3H2O/Mn9Mo6 |
Six-capped body centred cube |
HSM |
MnII–MoV AF coupling leading to SGS = 39/2 |
164
|
{Mn[Mn(MeOH)3]8[Re(CN)8]6}·MeOH·7.8H2O/Mn9Re6 |
Six-capped body centred cube |
Paramagnet |
— |
159
|
{Mn[Mn(MeOH)3]8[W(CN)8]5[Re(CN)8]}·nMeOH·mH2O/Mn9W5Re1 |
Six-capped body centred cube |
HSM |
MnII–WV AF coupling leading to SGS = 38/2 |
159
|
{Mn[Mn(MeOH)3]8[Mo(CN)8]5[Re(CN)8]}·5.5MeOH·4.5H2O/Mn9Mo5Re1 |
Six-capped body centred cube |
HSM |
MnII–MoV AF coupling leading to SGS = 38/2 |
159
|
{Mn[Mn(MeOH)3]8[Mo(CN)8]3[Re(CN)8]3}·0.5MeOH·6H2O/Mn9Mo3Re3 |
Six-capped body centred cube |
HSM |
MnII–MoV AF coupling leading to SGS = 36/2 |
159
|
{Co[Co(MeOH)3]8[W(CN)8]6}·19H2O/Co9W6 |
Six-capped body centred cube |
HSM, SMM behaviour |
CoII–WV AF coupling leading to SGS = 21/2b/τ0 = 7.39 × 10−11 s, ΔE/kB = 27.8 K |
156
|
{Co[Co(MeOH)3]8[W(CN)8]6}·4,4′-bpdo·MeOH·2H2O/Co9W6 |
Six-capped body centred cube |
HSM, SMM behaviour |
CoII–WV F coupling leading to SGS = 15/2; τ0 = 4(1) × 10−9 s, ΔE/kB = 10.3(5) K |
143
|
{Co[Co(MeOH)3]8[Mo(CN)8]6}·4MeOH·16H2O/Co9Mo6 |
Six-capped body centred cube |
HSM |
CoII–MoV AF coupling leading to SGS = 21/2b |
156
|
{Co[Co(MeOH)3]8[W(CN)8]5[ReV(CN)8]}·nMeOH·mH2O/Co9W5Re1 |
Six-capped body centred cube |
HSM |
CoII–WV AF coupling leading to SGS = 20/2c |
159
|
{Co[Co(MeOH)3]8[Mo(CN)8]5[Re(CN)8]}·3MeOH·8H2O/Co9Mo5Re1 |
Six-capped body centred cube |
HSM |
CoII–MoV AF coupling leading to SGS = 20/2c |
159
|
{Ni[Ni(MeOH)3]8[W(CN)8]6}·15MeOH/Ni9W6 |
Six-capped body centred cube |
HSM |
NiII–WV F coupling leading to SGS = 24/2 |
155
|
{Fe[Fe(MeOH)3]8[W(CN)8]6}·nMeOH/Fe9W6 |
Six-capped body centred cube |
CT phase transition |
Thermal CT: FeII-HS–WV (HT) to FeIII-HS–WIV (LT); thermal hysteresis loop of 8 K (Tc↓ = 206 K, Tc↑ = 214 K) |
157
|
{Co[Co(MeOH)3]2[Fe(MeOH)3]6[W(CN)8]6}·nMeOH/Co3Fe6W6 |
Six-capped body centred cube |
CT/CTIST phase transition |
Thermal CT: FeII-HS–WV (HT) to FeIII-HS–W (LT) concomitant with thermal CTIST: CoII-HS–WV (HT) to CoIII-LS–WIV (LT); thermal hysteresis loop of 14 K (Tc↓ = 193 K, Tc↑ = 207 K) |
158
|
15-centred clusters with bidentate blocking ligands, 9 compounds
|
{Ni[Ni(btz)(MeOH)]8[W(CN)8]6}·6MeOH·18H2O/Ni9W6 |
Six-capped body centred cube |
HSM |
NiII–WV F coupling leading to SGS = 24/2 |
162
|
{Ni[Ni(2,2′-bpy)(H2O)]8[W(CN)8]6}·41H2O/Ni9W6 |
Six-capped body centred cube |
HSM, SMM behaviour |
NiII–WV F coupling leading to SGS = 24/2;/τ0 = 1.5 × 10−13 s, ΔE/kB = 47 K |
160
|
{Ni[Ni(2,2′-bpy)(H2O)]8[W(CN)8]6}·16H2O/Ni9W6 |
Six-capped body centred cube |
HSM |
NiII–WV F coupling leading to SGS = 24/2 |
163
|
{Ni[Ni(tmphen)(MeOH)]6[Ni(H2O)3]2[WV(CN)8]6}·6DMF/Ni9W6 |
Six-capped body centred cube |
HSM, SMM behaviour |
NiII–WV F coupling leading to SGS = 24/2; hysteresis loop below TB = 0.7 K |
109
|
{Ni[Ni(4,4′-dmbpy)(H2O)]8[W(CN)8]6}·18H2O/Ni9W6 |
Six-capped body centred cube |
HSM |
NiII–WV F coupling leading to SGS = 24/2 |
163
|
{Ni[Ni(5,5′-dmbpy)(H2O)]8[W(CN)8]6}·8H2O/Ni9W6 |
Six-capped body centred cube |
HSM |
NiII–WV F coupling leading to SGS = 24/2 |
163
|
{Ni[Ni(4,4′-dtbpy)(H2O)]8[W(CN)8]6}·17H2O/Ni9W6 |
Six-capped body centred cube |
HSM |
NiII–WV F coupling leading to SGS = 24/2 |
163
|
{Ni[Ni(2,2′-bpy)(H2O)]8[Mo(CN)8]6}·12H2O/Ni9Mo6 |
Six-capped body centred cube |
HSM |
NiII–MoV F coupling leading to SGS = 24/2 |
161
|
{Ni[Ni(tmphen)(MeOH)]6[Ni(H2O)3]2[Mo(CN)8]6}·6DMF/Ni9Mo6 |
Six-capped body centred cube |
HSM |
NiII–MoV F coupling leading to SGS = 24/2 |
109
|
15-centred clusters in the coordination network, 3 compounds
|
{Mn[Mn(4,4′-bpy)(EtOH)]2[Mn(4,4′-bpy)0.5(EtOH)2]2[Mn(4,4′-bpy)0.5(EtOH)(H2O)]2[Mn(EtOH)2(H2O)]2[W(CN)8]6}·10EtOH/Mn9W6 |
Six-capped body centred cube in I0O2 network |
HSM embedded in I0O2 network |
MnII–WV AF coupling leading to SGS = 39/2 |
141
|
{Mn[Mn(dpe)]4[Mn(dpe)0.5(MeOH)2]2[Mn(MeOH)3]2[W(CN)8]6}·14MeOH/Mn9W6 |
Six-capped body centred cube in I1O2 network |
Superpara-magnetic chain of Mn9W6 clusters in I1O2 network |
MnII–WV AF coupling along the chain built of Mn9W6 clusters, weak AF interchain interactions |
142
|
{Co[Co(4,4′-bpdo)1.5(MeOH)]8[W(CN)8]6}·2H2O/Co9W6 |
Six-capped body centred cube in I0O1 network |
HSM, embedded in I0O1 network |
CoII–WV F coupling leading to SGS = 15/2 |
143
|
18-centred clusters, 2 compounds
|
{[Ni(L1)]6[Ni(L1)(H2O)]6[Nb(CN)8]6}·100H2O/Ni12Nb6 |
12-Metallic Ni6Nb6 ring decorated by 6NiII centres |
Paramagnet |
Overall AF interactions |
144
|
{[Ni(L1)]6[Ni(L1)(H2O)]6[W(CN)8]6}·36H2O/Ni12W6 |
12-Metallic Ni6W6 ring decorated by 6NiII centres |
Paramagnet |
— |
145
|
20-centred clusters, 1 compound
|
{[Cu(Me3tacn)]12[Cu(H2O)][W(CN)8]2[W(CN)8]5}·24H2O (Me3tacn = 1,4,7-trimethyl-1,4,7-triazacyclononane)/Cu13W7 |
20-Metallic open winged cage |
Paramagnet |
Overall F interactions |
165
|
M′ centres can be coordinated by solvent molecules (solv = H2O, MeOH, EtOH) or bidentate chelating N-donor or N,O-donor ligands L forming [M′(μ-NC)3(L)(solv)] units (Fig. 7a).160–163 However, in {NiII[NiII(tmphen)(MeOH)]6[Ni(H2O)3]2[μ-CN]30[WV(CN)3]6} two NiII centres located at the opposite external M′ sites do not coordinate 3,4,7,8-tmphen, preserving the original composition: [M′(μ-NC)3(solv)3]. The remaining six NiII centres adopt [Ni(μ-NC)3(tmphen)(solv)] coordination, with all six ligands located on the perimeter and oriented almost perfectly parallel to each other (pancake bonds). This results in a disc-like shape with the molecular symmetry lowered from Oh to approximate C3v (Fig. 7b).109
 |
| Fig. 7 Molecular structures of {Ni9W6(4,4′-dmbpy)8} (a) and {Ni9W6(tmphen)6} (b) presented perpendicular (left) and along (right) the C3 axis. Colour code: {Ni(solv)x} – red, {W(CN)3} – blue, CN− bridges – grey, organic blocking ligands – green. | |
3.4.2 Isomerism.
The consequence of the presence of [M′(μ-NC)3(L)(solv)] moieties is stereoisomerism as was presented by Nowicka et al.163 A total number of possible spatial arrangements of external ligands on eight independent [M′(μ-NC)3(L)(solv)] moieties per cluster can be calculated as 37 = 2187 (variations with repetition, three combinations per one M′ site). In the case of four symmetrically independent external Ni cations observed for centrosymmetric clusters the number of possible combinations is reduced to 33 = 27, with the independent crystallographic unit being “half” of the cluster. As several combinations are identical (clusters can be superimposed) six different isomers were identified and their point symmetry was ascribed as D4h (one), C4v (one), C2h (two) and Ci (two combinations; Fig. 8). [M′(μ-NC)3(L)(solv)]-based isomerism was identified in the case of Ni9W6 clusters with bidentate ligands: 2,2′-bpy and its alkyl-substituted derivatives (Table 3).163
 |
| Fig. 8 Geometrical isomers of centrosymmetric {M′[M′X2Y]8[M(CN)8]6} type clusters; X – blue sticks = identical monodentate or symmetrical bidentate ligands (bpy-derivatives), Y – red balls = ligand (solvent). For clarity only one half of each cluster is depicted.163 Reproduced from ref. 163 with permission from The Royal Society of Chemistry. | |
Table 3 Comparison of structural data and isomeric forms for known pentadecanuclear clusters with eight symmetrical bidentate ligands
Cluster formula |
Cell volume, space group P![[1 with combining macron]](https://www.rsc.org/images/entities/char_0031_0304.gif) |
No. of symmetry non-equivalent clusters |
Effective cell volume per cluster and solvent molecules |
Isomers |
Ref. |
{Ni[Ni(bpy)(solv)]8[W(CN)8]6} |
8536 |
2 |
4268 |
III and IV |
163
|
|
9392 |
2 |
4696 |
III and IV |
163
|
|
9593 |
2 |
4797 |
IV |
160
|
{Ni[Ni(bpy)(H2O)]8[Mo(CN)8]6} |
9666 |
2 |
4833 |
IV |
161
|
{Ni[Ni(btz)(H2O)]8[W(CN)8]6} |
5036 |
1 |
5036 |
IV |
162
|
{Ni[Ni(4,4′-dmbpy)(solv)]8[W(CN)8]6} |
6273 |
1 |
6273 |
VI |
163
|
|
6026 |
1 |
6026 |
II and VI |
163
|
{Ni[Ni(5,5′-dmbpy)(solv)]8[W(CN)8]6} |
5828 |
1 |
5828 |
IV |
163
|
|
12 054 |
2 |
6027 |
IV |
163
|
{Ni[Ni(4,4′-dtbpy)(solv)]8[W(CN)8]6} |
7615 |
1 |
7615 |
II |
163
|
3.4.3 Secondary building blocks.
M′9M6 clusters can be used as secondary building blocks (SBB) in the construction of more complex supramolecular systems (Fig. 9).124,166–168 The fac-[M′(μ-NC)3(HOR)3] groups of a M′9M6 cluster located in the corners of a cube can be considered as open coordination sites and [M(CN)8] groups located in the corners of an octahedron with their terminal CN− ligands can act as molecular bridges (Fig. 9a). The use of 4,4′-bpy and its derivatives like dpe led to the formation of extended hybrid coordination polymers unprecedented in cyanide chemistry like the 2-D I0O2 (I – inorganic connectivity with its dimensionality in the superscript, O – organic connectivity with its dimensionality in the superscript)169 {Mn9W6-(4,4′-bpy)}∞ polymer revealing double 4,4′-bpy intercluster bridging (Fig. 9b)141 or the 3-D I1O2 {Mn9W6-(dpe)}∞ showing unique quadruple intercluster cyanide-bridging towards a coordination nanowire (Fig. 9c). The observed hybrid organic–inorganic coordination resulted in the hexagonal arrangement of the nanowires.142 The use of 4,4′-bpdo and CoII centres leads to two different products depending on the bpdo
:
Co molar ratio. The 1
:
1 ratio gives 1-D supramolecular zig-zag chains {Co9W6⋯(bpdo)}∞ with hydrogen bonding (MeOH)2⋯bpdo⋯(HOMe)2 patterns (Fig. 9d). The excess of bpdo gave the 1-D coordination nanochain I0O1 {Co9W6-(bpdo)}∞ with quadruple linear connectivity and additional monodentate side coordination of bpdo (Fig. 9e).143
 |
| Fig. 9 Observed reactivity of {M′[M′(ROH)3]8[W(CN)8]6} (M′ = Mn or Co) molecules in ROH media. {M′(solv)x} – red, {W(CN)3} – blue, CN− bridges – grey, organic linkers – green. The green-to-red arrows and blue-to-red arrows indicate the propagation directions of the M′9M6 coordination skeleton; (a) M′9M6 cluster; (b) {Mn9W6-(4,4′-bpy)}∞ polymer; (c) {Mn9W6-(dpe)}∞ coordination polymer; (d) {Co9W6⋯(bpdo)}∞ H-bonded chain; (e) {Co9W6-(bpdo)}∞ quadruple chain. For details see the text. | |
3.4.4 Magnetic properties and functionalities.
The most important magnetic properties of pentadecanuclear clusters and the relevant parameters are presented in Table 2. The high spin ground states S = 39/2 for Mn9M6,153,154S = 12 for Ni9M6,155,160,161,163S = 15/2 for Co9M6 (M = Mo, W)143,156S = 18 for Mn9W5Re and Mn9Mo5Re and S = 17 for Mn9Mo3Re3
159 have been recognized due to the strong magnetic interactions between the CN-bridged metal ions. The magnetic coupling constants JM′M were calculated from direct fitting or simulation of magnetic data as well as using DFT methods for cyanide-bridged fragments of Mn9M6 and Ni9M6 clusters. These JM′M values are within the 0 to −18 cm−1 range for Mn analogues170,171 and 0 to +16 cm−1 for Ni analogues (for the following exchange Hamiltonian: Ĥ = −2JM′MŜ1Ŝ2).109,155,171 The antiferromagnetic interactions in Mn9M6 clusters were also confirmed by polarized neutron diffraction.170 The slow magnetic relaxation was reported for Co9W6,156 Co9W5Re,159 Co9W6⋯(bpdo)143 and Ni9M6(bpy)8 (M = W, Mo).160,161 The SMM character has been confirmed only for Ni9W6(tmphen)6 by measuring a magnetic hysteresis loop below T = 0.7 K.109 In the hybrid Mn9W6-(bpy) material the magnetic properties related to the high spin ground state of S = 39/2 are preserved,141 while in the Mn9W6-(dpe) long range antiferromagnetic ordering below Tc = 3.5 K and double metamagnetic transitions at H = 100 Oe and 480 Oe are observed.142 The onset of slow magnetic relaxation for the supramolecular Co9W6-(bpdo) network below 4 K allowed us to calculate the relaxation time τ0 = 2 × 10−7 s and the effective energy barrier ΔE/kB = 19 K (S = 15/2) being very similar to those found for the original solvated Co9M6 clusters. The onset of magnetic relaxation for the coordination polymer Co9W6-(bpdo) was shifted below 3 K disabling its reliable analysis. This difference was correlated with the differences in local structural symmetry of the external CoII centres, structural distortion of the cyanido-bridged skeleton, possible anisotropy of magnetic WV–CN–CoII interactions and intercluster magnetic interactions.143 The influence of spatial orientation of three terminal MeOH ligands coordinated to the external M′ sites (M′ = Co, Ni) on the magnetic anisotropy was tested theoretically using DFT methods.172
Introduction of FeII ions as M′ sites in the M′9M6 clusters did not lead to a high spin ground state because the obtained compounds Fe9W6 and Fe6Co3W6 undergo a reversible charge-transfer assisted solid state phase transition based on FeIIWV (HT)⇄FeIIIWIV (LT) equilibrium. The transitions occur with a thermal hysteresis loop with Tc↓ = 208 K and Tc↑ = 214 K for Fe9W6,157 and with Tc↓ = 194 K and Tc↑ = 207 K for Fe6Co3W6,158 which was consequently shown by monocrystalline and powder X-ray diffraction, DSC and magnetic measurements. It was also shown that in Fe6Co3W6 electron transfer occurs simultaneously along two different channels: (NC)7WV–CN–HSFeII⇄(NC)7WIV–CN–HSFeIII (pure electron transfer) and (NC)7WV–CN–HSCoII⇄(NC)7WIV–CN–LSCoIII (electron transfer combined with the HSCoIII⇄LSCoIII spin transition), the first process being most probably a driving force for the second one (Fig. 10a). The related structural solid state transformation consisted of (i) a local isotropic change in Co–N bond-lengths of ca. 0.16 Å at the central Co site and in M′–O and M′–N bond-lengths of 0.08 Å at external Co or Fe sites (Fig. 10b), (ii) moderate and nearly isotropic cluster compression/expansion of ca. 0.2–0.3 Å (up to 2%, measured between the most remote atoms of terminal ligands) (Fig. 10c) and (iii) shortening/elongation of intercluster distances through hydrogen bonds (up to 4%). It was suggested that the spin transition HSCoIII⇄LSCoIII took place only at the central Co site, while the HSFeII⇄HSFeIII processes together with a simple shortening of bond lengths in [CoII(μ-NC)3(MeOH)3] moieties occurred in the external M′ corners. Interestingly, the identical change in the crystallographic cell volume between ca. 16
000 and ca. 17
000 Å3 was observed for both Fe9W6 and Fe6Co3W6, parallel to different local changes at M′ sites.
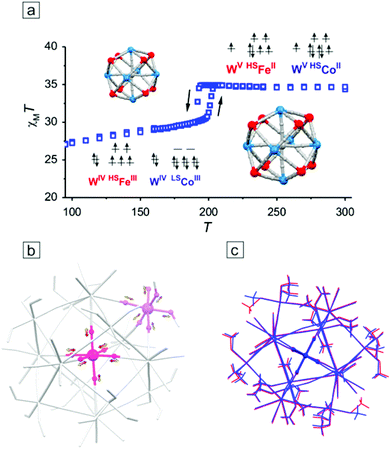 |
| Fig. 10 Structural and spin transition in {Fe6Co3W6}: (a) χMT(T) dependence at H = 1 kOe and electronic configurations of involved d metal ions (tungsten – blue, Co/Fe – red), (b) shortening/elongation of bond-lengths at Co/Fe sites (pink) and (c) changes in the molecular structure (red – HT phase, blue – LT phase). | |
3.5 Octadecanuclear decorated molecular wheels
The efficient approach towards octacyanidometallate-based coordination clusters with the nuclearity even higher than the pentadecanuclear molecules was presented first by Sutter and co-workers in 2003.144 They exploited the potential of 3d metal complexes with pyridine-based macrocyclic Schiff bases occupying the equatorial coordination sites, leaving only two axial positions to grow the coordination skeleton. The spontaneous assembly of such an equatorially blocked {NiIIL} unit with octacyanidoniobate(IV) gave the very unique octadecanuclear {[NiII(L)]6[NiII(L)(H2O)]6[NbIV(CN)8]6}·100H2O, composed of cyclic {Ni12Nb6} molecules. A 12-centred cyclic core {Ni6Nb6} resembles a giant cyclohexane in the chair conformation with six NbIV centres located on the vertexes, and six CN–Ni–NC linkages forming the sides of this spatial structure. The cyclic core is further decorated by six additional {NiIIL(H2O)} modules through the cyanide-bridges (Fig. 11a). The longest Ni–Ni and Nb–Nb distances within the Ni6Nb6 core are around 15 Å, and the largest size of the whole molecule is defined by the distance of 30 Å between O atoms of the opposite {NiIIL(H2O)} modules.
 |
| Fig. 11 Topology and dynamic properties of 18-metallic {[NiII(L)]6[NiII(L)(H2O)]6[MIV(CN)8]6}·nH2O, {Ni12M6} (L – pyridine-based macrocyclic Schiff base shown in the top right corner, M = Nb, n = 100 or M = W, n = 36) cyclic motifs: (a) the structural views along c (left) and a (right) directions (Ni – dark green, W – gold, CN – green sticks, N, O – red, C – yellow), and (b) the scheme of water-controlled syntheses and single-crystal transformations exploring dynamic properties of {Ni12M6} clusters.144,145 | |
The presence of both paramagnetic NiII and NbIV centres connected by cyanide bridges in the 18-metallic cluster suggested the possible observation of a high spin ground state S = 15 or S = 9 in the case of ferromagnetic or antiferromagnetic coupling, respectively. Surprisingly, the magnetic measurements did not prove the high-spin nature, showing only weak overall antiferromagnetic interactions operating in the crystal lattice.
The special structural feature of {Ni12Nb6} molecules is the presence of two types of NiII complexes: the bridging {NiIIL(μ-NC)2} and the terminal {NiIIL(H2O)(μ-NC)} for which the coordinated water prevents the further growth of the cyanido-bridged skeleton. This suggests the existence of a very subtle equilibrium between cyanides and water coordinated at the axial positions of NiII complexes. In fact, it was shown for the WIV-containing analogues that depending on the amount of water used in the synthesis it is possible to obtain repeatedly {[NiII(L)]6[NiII(L)(H2O)]6[WIV(CN)8]6}·36H2O, {Ni12W6} molecules, or two types of three-dimensional cyanido-bridged {[NiII(L)]2[WIV(CN)8]}n networks of Cc or Ia
d symmetry, both built of {Ni12W6} molecular subunits (Fig. 11b).145 Moreover, these 3D networks can be post-synthetically transformed to 0D {Ni12W6} decorated cyclic molecules by the addition of water to the mother solution containing single crystals. The observed solvent-mediated transformations are accompanied by the unprecedentedly significant change of the crystal shape from a flower with diamond-like leaves for the Ia
d network to block crystals for the Cc network, and needles for {Ni12W6} molecules. This observation points to the possible dynamic properties of 18-metallic decorated {Ni12M6} cyclic molecules, which can be further explored in the reversible synthetic switching between different cyanido-bridged magnetic frameworks of various dimensionalities.
3.6 Eicosanuclear open winged cages
The divalent 3d cations of the octahedral geometry combined with octacyanidometallates (Mo, W, Re) strongly prefer the construction of 15-centred M′9[M(CN)8]6 clusters of a six-capped body-centred cube topology, especially in alcoholic media. However, such molecules are not known for CuII ions probably due to the strong Jahn–Teller effect leading to the preferable D4h or C4v symmetry which strongly hampers the growth of Cu9[M(CN)8]6 clusters. To prevent the formation of 3-D coordination polymers and to impose the C3v symmetry obtained typically at fac-[M′(solv)3(μ-NC)3] moieties the self-assembly between CuII and [WV(CN)8]3− was carried out with the preprogrammed tridentate Me3tacn ligand (Me3tacn = 1,4,7-trimethyl-1,4,7-triazacyclononane) in a methanolic solution. Instead of 15-centred molecules much larger 20-metallic {[CuII(Me3tacn)]12[CuII(H2O)][WV(CN)8]2[WIV(CN)8]5}·24H2O, {Cu13W7} clusters of a very specific open winged molecular cage topology were obtained (Fig. 12).165 The opening cage part of this molecule consists of nine CuII ions constructing a body-centred cube which is essentially the same as in M′9[M(CN)8]6 clusters but only five [M(CN)8] units cover the cube which is due to the strong axial elongation of the central CuII ion. The resulting {Cu9W5} units adopt a five-capped body-centred cube topology which is an open-cage structure different from spatially closed M′9[M(CN)8]6 clusters. In addition, four trinuclear {Cu2W} wings with half-occupancies are bonded to four W and four Cu centres of {Cu9W5} cages giving the unique eicosanuclear {Cu13W7} cluster core, which is the largest among [M(CN)8]-based polynuclear molecules. Such a sophisticated spatial structure was primarily caused by the geometrical preferences of CuII complexes but the reported partial reduction of WV to WIV plays also a crucial role in the successful formation of a neutral 20-metallic cluster. This, however, strongly limits the cyanido-mediated intracluster magnetic interactions, as five diamagnetic [WIV(CN)8]4− are embedded in each {Cu13W7} molecule. For the remaining paramagnetic CuII and WV centres, an expected ferromagnetic coupling was detected but this does not lead to the high ground state spin of the whole molecule due to the partial isolation of the spin carriers. Despite this, it is clearly proved that the application of geometrically flexible CuII complexes together with flexible and redox active [WIV/V(CN)8]4−/3− ions can be a successful synthetic pathway towards very high nuclearity clusters.165
 |
| Fig. 12 Topology of the largest [M(CN)8]-based cluster – 20-metallic {[CuII(Me3tacn)]12[CuII(H2O)][WV(CN)8]2[WIV(CN)8]5}·24H2O, {Cu13W7} (Me3tacn = 1,4,7-trimethyl-1,4,7-triazacyclononane) open winged molecular cage in the views along a (top) and c (bottom) axes (left panel). The 15-centred {M′[M′(L)3]8[M(CN)8]6}·nsolv, {M′9M6} (M′ = Mn–Ni; M = Mo, W, Re) cluster was shown as the comparison (right panel) in the corresponding structural views. The blue balls and sticks present the identical parts for both types of clusters, while the orange part represents the differences between these two topologies. Note that all four trinuclear Cu2W wings of {Cu13W7} clusters reveal only half crystallographic occupancies.153,165 | |
4. Conclusions
Octacyanidometallates are unique building blocks that are extremely useful in the construction of various low dimensional magnetic clusters ranging from tetranuclear squares, pentanuclear trigonal bipyramids, hexanuclear octahedrons, to pentadecanuclear six-capped-body-centred cubes and even larger molecules. Some of these cluster topologies are unique in the sense that they are not known for other cyanidometallates ([M(CN)6]n− or [M(CN)7]n−) as it is in the case of hexanuclear and pentadecanuclear cluster families. Owing to the intrinsic geometrical flexibility, octacyanidometallates can easily adopt different coordination modes resulting in all possible topologies which are relatively easy to control by the use of appropriate ‘protecting groups’ – blocking ligands at the 3d metal centre.
Combination of various metal centres in octacyanidometallate-based clusters enables observation of many functionalities: thermal charge transfer,158 spin cross-over150 and photomagnetic effects18 (due to the availability of at least two oxidation states at the metal centres), magnetic bistability and SMM-type slow magnetic relaxation109 (thanks to significant magnetic anisotropy originating from strong spin–orbit coupling of the building blocks) or high spin ground states leading to the observation of the magnetocaloric effect (due to strong exchange coupling within the M–CN–M′ linkages of a multinuclear system and weak intermolecular interactions).173 Therefore, octacyanidometallate-based clusters can be considered as perfect molecular platforms for the development of molecular devices for future applications in spintronics and molecular electronics, nanoscale information storage, molecular sensors or light-triggered molecular switching devices.
A systematic study of octacyanidometallate-based clusters led to the discovery of new physical and chemical phenomena like simultaneous electron transfer through two different charge transfer pathways in Fe6Co3W6,158 or the FeII–WV⇄FeIII–WIV charge transfer equilibrium in Fe9W6
157 clusters or the strong photomagnetic effect in Mn4Mo2 and Mn4W2 hexanuclear octahedral molecules that is not based on charge transfer within the CuII–MoIV or CoII–WV structural motifs.113 It is expected that further in-depth research on similar heterometallic systems will lead to the discovery of other unknown phenomena and functionalities important in the development of chemistry, physics and materials science.
Abbreviations
1-D | One dimensional |
2,2′-bpy | 2,2′-Bipyridine |
2-D | Two dimensional |
3-D | Three dimensional |
4,4′-bpdo | 4,4′-Bipyridine-N,N′-dioxide |
4,4′-dmbpy | 4,4′-Dimethyl-2,2′-bipyridine |
4,4′-dtbpy | 4,4′-Ditertbutyl-2,2′-bipyridine |
5,5′-dmbpy | 5,5′-Dimethyl-2,2′-bipyridine |
ac | Acetate |
AC | Alternating current |
AF | Antiferromagnetic |
bik | Bis(1-methylimidazole-2-yl)ketone |
bpy | 2,2′-Bipyridine |
btz | 2,2′-Bis(4,5-dihydrothiazine) |
CT | Charge transfer |
CTIST | Charge transfer induced spin transition |
DFT | Density functional theory |
DMF |
N,N′-Dimethylformamide |
DMSO | Dimethylsulfoxide |
dpa | Di-(2-picolyl)amine |
dpe | 1,2-Di(4-pyridyl)ethylene |
DSC | Differential scanning calorimetry |
EtOH | Ethanol |
F | Ferromagnetic |
HMeIm |
N-Methyl imidazole |
hmp | 2-Hydroxymethylpyridine |
HS | High spin |
HSM | High spin molecule |
HT | High temperature |
iPrOH | Isopropanol |
LS | Low spin |
LT | Low temperature |
Me3tacn | 1,4,7-Trimethyl-1,4,7-triazacyclononane |
MeCN | Acetonitrile |
MeOH | Methanol |
NOA | Natural optical activity |
ox | Oxalate |
phen | 1,10-Phenanthroline |
PrOH | Propanol |
SCO | Spin crossover |
SMM | Single molecule magnet |
solv | Solvent |
T
c
| Critical temperature |
tmphen | 3,4,7,8-Tetramethyl-1,10-phenanthroline |
tpm | Tri(1H-pyrazol-1-yl)methane |
tptz | 2,4,6-Tris(2-pyridyl)-1,3,5-triazine |
tpy | 2,2′:6′,2′′-Terpyridine |
tren | Tris(2-aminoethyl)amine |
valen |
N,N′-Bis(3-methoxy-salicylidene)-ethylenediamine |
Acknowledgements
This work was partially financed by the Polish National Science Centre within the Research Project DEC-2011/01/B/ST5/00716. DP gratefully acknowledges the financial support of the EC REA within the Marie Curie International Outgoing Fellowship, project MultiCyChem (grant agreement no. PIOF-GA-2011-298569) and the financial support of the Polish Ministry of Science and Higher Education within the Fellowship for Outstanding Young Scientists programme (2014 edition). SC gratefully acknowledges the financial support of the Foundation for Polish Science within the START fellowship. MR gratefully acknowledges the financial support of the Polish Ministry of Science and Higher Education within the Diamond Grant (grant agreement no. 0195/DIA/2013/42).
References
- D. Gatteschi, J. Alloys Compd., 2001, 317–318, 8–12 CrossRef CAS.
-
D. Gatteschi, R. Sessoli and R. Villain, Molecular Nanomagnets, Oxford University Press, New York, 2006 Search PubMed.
- R. Sessoli, Angew. Chem., Int. Ed., 2012, 51, 43–45 CrossRef CAS PubMed.
- M. Yamashita, Dalton Trans., 2012, 41, 13555 RSC.
- K. R. Dunbar, Inorg. Chem., 2012, 51, 12055–12058 CrossRef CAS PubMed.
- D. Pinkowicz, Z. Li, P. Pietrzyk and M. Rams, Cryst. Growth Des., 2014, 14, 4878–4881 CAS.
- I. Ratera and J. Veciana, Chem. Soc. Rev., 2012, 41, 303–349 RSC.
- L. B. L. Escobar, G. P. Guedes, S. Soriano, N. L. Speziali, A. K. Jordão, A. C. Cunha, V. F. Ferreira, C. Maxim, M. A. Novak, M. Andruh and M. G. F. Vaz, Inorg. Chem., 2014, 53, 7508–7517 CrossRef CAS PubMed.
- R. A. Layfield, Organometallics, 2014, 33, 1084–1099 CrossRef CAS.
- D. N. Woodruff, R. E. P. Winpenny and R. A. Layfield, Chem. Rev., 2013, 113, 5110–5148 CrossRef CAS PubMed.
- C. Train, R. Gheorghe, V. Krstic, L.-M. Chamoreau, N. S. Ovanesyan, G. L. J. A. Rikken, M. Gruselle and M. Verdaguer, Nat. Mater., 2008, 7, 729–734 CrossRef CAS PubMed.
- F. Pointillart, B. L. Guennic, S. Golhen, O. Cador, O. Maury and L. Ouahab, Chem. Commun., 2013, 49, 615–617 RSC.
- S. Fortier, J. J. Le Roy, C.-H. Chen, V. Vieru, M. Murugesu, L. F. Chibotaru, D. J. Mindiola and K. G. Caulton, J. Am. Chem. Soc., 2013, 135, 14670–14678 CrossRef CAS PubMed.
- D. Pinkowicz, M. Ren, L.-M. Zheng, S. Sato, M. Hasegawa, M. Morimoto, M. Irie, B. K. Breedlove, G. Cosquer, K. Katoh and M. Yamashita, Chem. – Eur. J., 2014, 20, 12502–12513 CAS.
- S. Chorazy, K. Nakabayashi, S.-i. Ohkoshi and B. Sieklucka, Chem. Mater., 2014, 26, 4072–4075 CrossRef CAS.
- S. Chorazy, K. Nakabayashi, M. Arczynski, R. Pełka, S.-i. Ohkoshi and B. Sieklucka, Chem. – Eur. J., 2014, 20, 7144–7159 CrossRef CAS PubMed.
- D. Pinkowicz, R. Podgajny, W. Nitek, M. Rams, A. M. Majcher, T. Nuida, S.-i. Ohkoshi and B. Sieklucka, Chem. Mater., 2010, 23, 21–31 CrossRef.
- A. Bleuzen, V. Marvaud, C. Mathoniere, B. Sieklucka and M. Verdaguer, Inorg. Chem., 2009, 48, 3453–3466 CrossRef CAS PubMed.
- I. Y. Barskaya, E. V. Tretyakov, R. Z. Sagdeev, V. I. Ovcharenko, E. G. Bagryanskaya, K. Y. Maryunina, T. Takui, K. Sato and M. V. Fedin, J. Am. Chem. Soc., 2014, 136, 10132–10138 CrossRef CAS PubMed.
- T. Liu, H. Zheng, S. Kang, Y. Shiota, S. Hayami, M. Mito, O. Sato, K. Yoshizawa, S. Kanegawa and C. Duan, Nat. Commun., 2013, 4 Search PubMed.
- S.-i. Ohkoshi and H. Tokoro, Acc. Chem. Res., 2012, 45, 1749–1758 CrossRef CAS PubMed.
- D. Pinkowicz, K. Kurpiewska, K. Lewinski, M. Balanda, M. Mihalik, M. Zentkova and B. Sieklucka, CrystEngComm, 2012, 14, 5224–5229 RSC.
- G. Seber, G. J. Halder, J. A. Schlueter and P. M. Lahti, Cryst. Growth Des., 2011, 11, 4261–4266 CAS.
- M. Ohba, W. Kaneko, S. Kitagawa, T. Maeda and M. Mito, J. Am. Chem. Soc., 2008, 130, 4475–4484 CrossRef CAS PubMed.
- P. Gütlich, A. B. Gaspar, Y. Garcia and V. Ksenofontov, C. R. Chim., 2007, 10, 21–36 CrossRef PubMed.
- T. Mahfoud, G. Molnár, S. Bonhommeau, S. Cobo, L. Salmon, P. Demont, H. Tokoro, S.-I. Ohkoshi, K. Boukheddaden and A. Bousseksou, J. Am. Chem. Soc., 2009, 131, 15049–15054 CrossRef CAS PubMed.
- S. Thiele, F. Balestro, R. Ballou, S. Klyatskaya, M. Ruben and W. Wernsdorfer, Science, 2014, 344, 1135–1138 CrossRef CAS PubMed.
- S. Loth, S. Baumann, C. P. Lutz, D. M. Eigler and A. J. Heinrich, Science, 2012, 335, 196–199 CrossRef CAS PubMed.
- M. Fitta, M. Bałanda, R. Pełka, P. Konieczny, D. Pinkowicz and B. Sieklucka, J. Phys.: Condens. Matter, 2013, 25 Search PubMed.
-
O. Sato, J. Tao and Y. Z. Zhang, Angew. Chem., Int. Ed., 2007, 46, 2152–2187 Search PubMed.
- S. Shimomura, R. Matsuda, T. Tsujino, T. Kawamura and S. Kitagawa, J. Am. Chem. Soc., 2006, 128, 16416–16417 CrossRef CAS PubMed.
- D. Pinkowicz, R. Podgajny, B. Gaweł, W. Nitek, W. Łasocha, M. Oszajca, M. Czapla, M. Makarewicz, M. Bałanda and B. Sieklucka, Angew. Chem., Int. Ed., 2011, 50, 3973–3977 CrossRef CAS PubMed.
- M. Ren, D. Pinkowicz, M. Yoon, K. Kim, L.-M. Zheng, B. K. Breedlove and M. Yamashita, Inorg. Chem., 2013, 52, 8342–8348 CrossRef CAS PubMed.
- M.-H. Zeng, Z. Yin, Y.-X. Tan, W.-X. Zhang, Y.-P. He and M. Kurmoo, J. Am. Chem. Soc., 2014, 136, 4680–4688 CrossRef CAS PubMed.
- A. K. Powell, Nat. Chem., 2010, 2, 351–352 CrossRef CAS.
- E. Rentschler, M. Affronte, C. Massobrio and P. Rabu, Solid State Sci., 2009, 11, 970–971 CrossRef CAS PubMed.
- M. Kurmoo, Chem. Soc. Rev., 2009, 38, 1353–1379 RSC.
- D. Gatteschi, L. Bogani, A. Cornia, M. Mannini, L. Sorace and R. Sessoli, Solid State Sci., 2008, 10, 1701–1709 CrossRef CAS PubMed.
- M. Murrie and D. J. Price, Ann. Rep., Sect. A: Inorg. Chem., 2007, 103, 20 RSC.
- E. Coronado and K. R. Dunbar, Inorg. Chem., 2009, 3293–3896 CrossRef CAS PubMed.
- O. Waldmann, Coord. Chem. Rev., 2005, 249, 2550–2566 CrossRef CAS PubMed.
- J. Mroziński, Coord.
Chem. Rev., 2005, 249, 2534–2548 CrossRef PubMed.
- J. V. Yakhmi, Macromol. Symp., 2004, 212, 141–158 CrossRef CAS.
- D. Gatteschi and R. Sessoli, J. Magn. Magn. Mater., 2004, 272, 1030–1036 CrossRef PubMed.
- D. Gatteschi and R. Sessoli, Angew. Chem., Int. Ed., 2003, 42, 268–297 CrossRef CAS PubMed.
- J. S. Miller and A. J. Epstein, MRS Bull., 2000, 25, 21–28 CrossRef CAS.
- G. Christou, D. Gatteschi, D. N. Hendrickson and R. Sessoli, MRS Bull., 2000, 25, 66–71 CrossRef CAS.
-
R. Boca, Theoretical Foundations of Molecular Magnetism, Elsevier, 1999 Search PubMed.
- J. M. Clemente-Juan and E. Coronado, Coord. Chem. Rev., 1999, 193–5, 361–394 CrossRef.
-
O. Kahn, Molecular Magnetism, Wiley, New York, NY, 1993 Search PubMed.
- J. S. Miller, A. J. Epstein and W. M. Reiff, Acc. Chem. Res., 1988, 21, 114–120 CrossRef CAS.
-
R. Willett, D. Gatteschi and O. Kahn, Magneto-structural Correlation in Exchange Coupled Systems, Kluwer, Dordrecht, 1985 Search PubMed.
- J. A. Smith, J.-R. Galán-Mascarós, R. Clérac, J.-S. Sun, X. Ouyang and K. R. Dunbar, Polyhedron, 2001, 20, 1727–1734 CrossRef CAS.
- M.-G. Alexandru, D. Visinescu, S. Shova, F. Lloret, M. Julve and M. Andruh, Inorg. Chem., 2013, 52, 11627–11637 CrossRef CAS PubMed.
- D. Visinescu, A. M. Madalan, M. Andruh, C. Duhayon, J.-P. Sutter, L. Ungur, W. Van den Heuvel and L. F. Chibotaru, Chem. – Eur. J., 2009, 15, 11808–11814 CrossRef CAS PubMed.
- D. Pinkowicz, H. Southerland, X.-Y. Wang and K. R. Dunbar, J. Am. Chem. Soc., 2014, 136, 9922–9924 CrossRef CAS PubMed.
- S. Wang, X. H. Ding, Y. H. Li and W. Huang, Coord. Chem. Rev., 2012, 256, 439–464 CrossRef CAS PubMed.
- S. Wang, X.-H. Ding, J.-L. Zuo, X.-Z. You and W. Huang, Coord. Chem. Rev., 2011, 255, 1713–1732 CrossRef CAS PubMed.
- B. Sieklucka, R. Podgajny, P. Przychodzen and T. Korzeniak, Coord. Chem. Rev., 2005, 249, 2203–2221 CrossRef CAS PubMed.
- R. Lescouezec, L. M. Toma, J. Vaissermann, M. Verdaguer, F. S. Delgado, C. Ruiz-Perez, F. Lloret and M. Julve, Coord. Chem. Rev., 2005, 249, 2691–2729 CrossRef CAS PubMed.
- J. T. Culp, J. H. Park, F. Frye, Y. D. Huh, M. W. Meisel and D. R. Talham, Coord. Chem. Rev., 2005, 249, 2642–2648 CrossRef CAS PubMed.
- V. Marvaud, J. M. Herrera, T. Barilero, F. Tuyeras, R. Garde, A. Scuiller, C. Decroix, M. Cantuel and C. Desplanches, Monatsh. Chem., 2003, 134, 149–163 CrossRef CAS PubMed.
- J. Cernak, M. Orendac, I. Potocnak, J. Chomic, A. Orendacova, J. Skorsepa and A. Feher, Coord. Chem. Rev., 2002, 224, 51–66 CrossRef CAS.
- J. S. Miller and J. L. Manson, Acc. Chem. Res., 2001, 34, 563–570 CrossRef CAS PubMed.
- M. Ohba and H. Okawa, Coord. Chem.
Rev., 2000, 198, 313–328 CrossRef CAS.
- M. Verdaguer, A. Bleuzen, V. Marvaud, J. Vaissermann, M. Seuleiman, C. Desplanches, A. Scuiller, C. Train, R. Garde, G. Gelly, C. Lomenech, I. Rosenman, P. Veillet, C. Cartier and F. Villain, Coord. Chem. Rev., 1999, 192, 1023–1047 CrossRef.
- D. Pinkowicz, R. Pełka, O. Drath, W. Nitek, M. Bałanda, A. M. Majcher, G. Poneti and B. Sieklucka, Inorg. Chem., 2010, 49, 7565–7576 CrossRef CAS PubMed.
- K. E. Funck, M. G. Hilfiger, C. P. Berlinguette, M. Shatruk, W. Wernsdorfer and K. R. Dunbar, Inorg. Chem., 2009, 48, 3438–3452 CrossRef CAS PubMed.
-
K. R. Dunbar, C. Achim and M. Shatruk, in Spin-Crossover Materials, John Wiley & Sons Ltd, 2013, pp. 171–202 Search PubMed.
-
M. Verdaguer and G. S. Girolami, in Magnetism: Molecules to Materials V, Wiley-VCH Verlag GmbH & Co. KGaA, 2005, pp. 283–346 Search PubMed.
- B. Sieklucka, R. Podgajny, T. Korzeniak, B. Nowicka, D. Pinkowicz and M. Kozieł, Eur. J. Inorg. Chem., 2011, 2011, 305–326 CrossRef.
- B. Sieklucka, R. Podgajny, D. Pinkowicz, B. Nowicka, T. Korzeniak, M. Balanda, T. Wasiutynski, R. Pelka, M. Makarewicz, M. Czapla, M. Rams, B. Gawel and W. Lasocha, CrystEngComm, 2009, 11, 2032–2039 RSC.
- R. Podgajny, D. Pinkowicz, B. Czarnecki, M. Kozieł, S. Chorąży, M. Wis, W. Nitek, M. Rams and B. Sieklucka, Cryst. Growth Des., 2014, 14, 4030–4040 CAS.
- B. Nowicka, T. Korzeniak, O. Stefańczyk, D. Pinkowicz, S. Chorąży, R. Podgajny and B. Sieklucka, Coord. Chem. Rev., 2012, 256, 1946–1971 CrossRef CAS PubMed.
- D. Pinkowicz, M. Rams, W. Nitek, B. Czarnecki and B. Sieklucka, Chem. Commun., 2012, 48, 8323–8325 RSC.
- M. W. G. DeBolster, Pure Appl. Chem., 1997, 69, 1251–1303 CAS.
- W.-F. Yeung, H.-K. Kwong, T.-C. Lau, S. Gao, L. Szeto and W.-T. Wong, Polyhedron, 2006, 25, 1256–1262 CrossRef CAS PubMed.
- L. R. Falvello and M. Tomas, Chem. Commun., 1999, 273–274 RSC.
- L. Jiang, T.-B. Lu and X.-L. Feng, Inorg. Chem., 2005, 44, 7056–7062 CrossRef CAS PubMed.
- A. Mondal, Y. Li, M. Seuleiman, M. Julve, L. Toupet, M. Buron-Le Cointe and R. Lescouëzec, J. Am. Chem. Soc., 2013, 135, 1653–1656 CrossRef CAS PubMed.
- Y.-H. Peng, Y.-F. Meng, L. Hu, Q.-X. Li, Y.-Z. Li, J.-L. Zuo and X.-Z. You, Inorg. Chem., 2010, 49, 1905–1912 CrossRef CAS PubMed.
- E. Pardo, M. Verdaguer, P. Herson, H. Rousselière, J. Cano, M. Julve, F. Lloret and R. Lescouëzec, Inorg. Chem., 2011, 50, 6250–6262 CrossRef CAS PubMed.
- D. Li, S. Parkin, G. Wang, G. T. Yee, A. V. Prosvirin and S. M. Holmes, Inorg. Chem., 2005, 44, 4903–4905 CrossRef CAS PubMed.
- A. Mondal, Y. Li, P. Herson, M. Seuleiman, M.-L. Boillot, E. Riviere, M. Julve, L. Rechignat, A. Bousseksou and R. Lescouezec, Chem. Commun., 2012, 48, 5653–5655 RSC.
- C.-F. Wang, W. Liu, Y. Song, X.-H. Zhou, J.-L. Zuo and X.-Z. You, Eur. J. Inorg. Chem., 2008, 2008, 717–727 CrossRef.
- Y.-Z. Zhang, U. P. Mallik, R. Clérac, N. P. Rath and S. M. Holmes, Polyhedron, 2013, 52, 115–121 CrossRef CAS PubMed.
- R. Kania, K. Lewinski and B. Sieklucka, Dalton Trans., 2003, 1033–1040 RSC.
- T. Korzeniak, C. Desplanches, R. Podgajny, C. Giménez-Saiz, K. Stadnicka, M. Rams and B. Sieklucka, Inorg. Chem., 2009, 48, 2865–2872 CrossRef CAS PubMed.
- H. Zhao, M. Shatruk, A. V. Prosvirin and K. R. Dunbar, Chem. – Eur. J., 2007, 13, 6573–6589 CrossRef CAS PubMed.
- J. Wang, Y.-L. Xu, H.-B. Zhou, H.-S. Wang, X.-J. Song, Y. Song and X.-Z. You, Dalton Trans., 2010, 39, 3489–3494 RSC.
- T. S. Venkatakrishnan, C. Desplanches, R. Rajamani, P. Guionneau, L. Ducasse, S. Ramasesha and J.-P. Sutter, Inorg. Chem., 2008, 47, 4854–4860 CrossRef CAS PubMed.
- Z.-X. Wang, L. Li, T.-W. Wang, Y.-Z. Li, S.-i. Ohkoshi, K. Hashimoto, Y. Song and X.-Z. You, Inorg. Chem., 2007, 46, 10990–10995 CrossRef CAS PubMed.
- R. Podgajny, T. Korzeniak, P. Przychodzeń, C. Gimenez-Saiz, M. Rams, M. Kwaśniak and B. Sieklucka, Eur. J. Inorg. Chem., 2010, 2010, 4166–4174 CrossRef.
- X.-D. Zheng, Y.-L. Hua, R.-G. Xiong, J.-Z. Ge and T.-B. Lu, Cryst. Growth Des., 2010, 11, 302–310 Search PubMed.
- N. Hoshino, Y. Sekine, M. Nihei and H. Oshio, Chem. Commun., 2010, 46, 6117–6119 RSC.
- M.-X. Yao, Z.-Y. Wei, Z.-G. Gu, Q. Zheng, Y. Xu and J.-L. Zuo, Inorg. Chem., 2011, 50, 8636–8644 CrossRef CAS PubMed.
- J.-N. Rebilly, L. Catala, G. Charron, G. Rogez, E. Riviere, R. Guillot, P. Thuery, A.-L. Barra and T. Mallah, Dalton Trans., 2006, 2818–2828 RSC.
- K. K. Klausmeyer, T. B. Rauchfuss and S. R. Wilson, Angew. Chem., Int. Ed., 1998, 37, 1694–1696 CrossRef CAS.
- H. Xiang, S.-J. Wang, L. Jiang, X.-L. Feng and T.-B. Lu, Eur. J. Inorg. Chem., 2009, 2009, 2074–2082 CrossRef.
- T. Korzeniak, B. Nowicka, K. Stadnicka, W. Nitek, A. M. Majcher and B. Sieklucka, Polyhedron, 2013, 52, 442–447 CrossRef CAS PubMed.
- Y.-Z. Zhang, U. P. Mallik, R. Clerac, N. P. Rath and S. M. Holmes, Chem. Commun., 2011, 47, 7194–7196 RSC.
- M. Nihei, Y. Okamoto, Y. Sekine, N. Hoshino, T. Shiga, I. P.-C. Liu and H. Oshio, Angew. Chem., Int. Ed., 2012, 51, 6361–6364 CrossRef CAS PubMed.
- D. Leznoff, C. Shorrock and R. Batchelor, Gold Bull., 2007, 40, 36–39 CrossRef CAS.
- R. Gheorghe, M. Kalisz, R. Clérac, C. Mathonière, P. Herson, Y. Li, M. Seuleiman, R. Lescouëzec, F. Lloret and M. Julve, Inorg. Chem., 2010, 49, 11045–11056 CrossRef CAS PubMed.
- J. Kim, S. Han, K. I. Pokhodnya, J. M. Migliori and J. S. Miller, Inorg. Chem., 2005, 44, 6983–6988 CrossRef CAS PubMed.
- Y.-J. Zhang, T. Liu, S. Kanegawa and O. Sato, J. Am. Chem. Soc., 2009, 131, 7942–7943 CrossRef CAS PubMed.
- Y. Zhang, U. P. Mallik, N. Rath, G. T. Yee, R. Clerac and S. M. Holmes, Chem. Commun., 2010, 46, 4953–4955 RSC.
- C. P. Berlinguette, J. A. Smith, J. R. Galán-Mascarós and K. R. Dunbar, C. R. Chim., 2002, 5, 665–672 CrossRef CAS.
- M. G. Hilfiger, H. Zhao, A. Prosvirin, W. Wernsdorfer and K. R. Dunbar, Dalton Trans., 2009, 5155–5163 RSC.
- J. L. Heinrich, J. J. Sokol, A. G. Hee and J. R. Long, J. Solid State Chem., 2001, 159, 293–301 CrossRef CAS.
- N. Hoshino, F. Iijima, G. N. Newton, N. Yoshida, T. Shiga, H. Nojiri, A. Nakao, R. Kumai, Y. Murakami and H. Oshio, Nat. Chem., 2012, 4, 921–926 CrossRef CAS PubMed.
- B. Sieklucka, J. Szklarzewicz, T. J. Kemp and W. Errington, Inorg. Chem., 2000, 39, 5156–5158 CrossRef CAS.
- C. Mathonière, R. Podgajny, P. Guionneau, C. Labrugere and B. Sieklucka, Chem. Mater., 2004, 17, 442–449 CrossRef.
- T. S. Venkatakrishnan, R. Rajamani, S. Ramasesha and J.-P. Sutter, Inorg. Chem., 2007, 46, 9569–9574 CrossRef CAS PubMed.
- S.-L. Ma, Y. Ma, S. Ren, S.-P. Yan and D.-Z. Liao, Struct. Chem., 2008, 19, 329–338 CrossRef CAS.
- X. Chen, P. Yang, S. L. Ma, S. Ren, M. Y. Tang, Y. Yang, Z. J. Guo and L. Z. Liu, J. Struct. Chem., 2009, 50, 495–499 CrossRef CAS PubMed.
- J. L. Heinrich, P. A. Berseth and J. R. Long, Chem. Commun., 1998, 1231–1232 RSC.
- Z.-G. Gu, W. Liu, Q.-F. Yang, X.-H. Zhou, J.-L. Zuo and X.-Z. You, Inorg. Chem., 2007, 46, 3236–3244 CrossRef CAS PubMed.
- J. Y. Yang, M. P. Shores, J. J. Sokol and J. R. Long, Inorg. Chem., 2003, 42, 1403–1419 CrossRef CAS PubMed.
- E. J. Schelter, F. Karadas, C. Avendano, A. V. Prosvirin, W. Wernsdorfer and K. R. Dunbar, J. Am. Chem. Soc., 2007, 129, 8139–8149 CrossRef CAS PubMed.
- M. Nihei, M. Ui, N. Hoshino and H. Oshio, Inorg. Chem., 2008, 47, 6106–6108 CrossRef CAS PubMed.
- E. J. Schelter, A. V. Prosvirin, W. M. Reiff and K. R. Dunbar, Angew. Chem., Int. Ed., 2004, 43, 4912–4915 CrossRef CAS PubMed.
- E. J. Schelter, A. V. Prosvirin and K. R. Dunbar, J. Am. Chem. Soc., 2004, 126, 15004–15005 CrossRef CAS PubMed.
- F. Karadas, C. Avendano, M. G. Hilfiger, A. V. Prosvirin and K. R. Dunbar, Dalton Trans., 2010, 39, 4968–4977 RSC.
- D. Li, S. Parkin, R. Clérac and S. M. Holmes, Inorg. Chem., 2006, 45, 7569–7571 CrossRef CAS PubMed.
- D. Li, S. Parkin, G. Wang, G. T. Yee, R. Clérac, W. Wernsdorfer and S. M. Holmes, J. Am. Chem. Soc., 2006, 128, 4214–4215 CrossRef CAS PubMed.
- K. Mitsumoto, H. Nishikawa, G. N. Newton and H. Oshio, Dalton Trans., 2012, 41, 13601–13608 RSC.
- D. Li, R. Clérac, O. Roubeau, E. Harté, C. Mathonière, R. Le Bris and S. M. Holmes, J. Am. Chem. Soc., 2007, 130, 252–258 CrossRef PubMed.
- M. L. Kuhlman and T. B. Rauchfuss, Inorg. Chem., 2003, 43, 430–435 CrossRef PubMed.
- J. L. Boyer, H. Yao, M. L. Kuhlman, T. B. Rauchfuss and S. Wilson, Eur. J. Inorg. Chem., 2007, 2007, 2721–2728 CrossRef.
- K. K. Klausmeyer, S. R. Wilson and T. B. Rauchfuss, J. Am. Chem. Soc., 1999, 121, 2705–2711 CrossRef CAS.
- S. C. N. Hsu, M. Ramesh, J. H. Espenson and T. B. Rauchfuss, Angew. Chem., Int. Ed., 2003, 42, 2663–2666 CrossRef CAS PubMed.
- S. M. Contakes, M. L. Kuhlman, M. Ramesh, S. R. Wilson and T. B. Rauchfuss, Proc. Natl. Acad. Sci. U. S. A., 2002, 99, 4889–4893 CrossRef CAS PubMed.
- J. J. Sokol, M. P. Shores and J. R. Long, Angew. Chem., Int. Ed., 2001, 40, 236–239 CrossRef CAS.
- P. A. Berseth, J. J. Sokol, M. P. Shores, J. L. Heinrich and J. R. Long, J. Am. Chem. Soc., 2000, 122, 9655–9662 CrossRef CAS.
- M. P. Shores, J. J. Sokol and J. R. Long, J. Am. Chem. Soc., 2002, 124, 2279–2292 CrossRef CAS PubMed.
- L. C. Beltran, J. Sokol and J. Long, J. Cluster Sci., 2007, 18, 575–596 CrossRef CAS PubMed.
- L. Jiang, H. J. Choi, X.-L. Feng, T.-B. Lu and J. R. Long, Inorg. Chem., 2007, 46, 2181–2186 CrossRef CAS PubMed.
- S. Wang, J.-L. Zuo, H.-C. Zhou, H. J. Choi, Y. Ke, J. R. Long and X.-Z. You, Angew. Chem., Int. Ed., 2004, 43, 5940–5943 CrossRef CAS PubMed.
- J. J. Sokol, M. P. Shores and J. R. Long, Inorg. Chem., 2002, 41, 3052–3054 CrossRef CAS PubMed.
- R. Podgajny, W. Nitek, M. Rams and B. Sieklucka, Cryst. Growth Des., 2008, 8, 3817–3821 CAS.
- R. Podgajny, S. Chorazy, W. Nitek, M. Rams, M. Bałanda and B. Sieklucka, Cryst. Growth Des., 2010, 10, 4693–4696 CAS.
- S. Chorazy, R. Podgajny, W. Nitek, M. Rams, S.-i. Ohkoshi and B. Sieklucka, Cryst. Growth Des., 2013, 13, 3036–3045 CAS.
- R. Pradhan, C. Desplanches, P. Guionneau and J.-P. Sutter, Inorg. Chem., 2003, 42, 6607–6609 CrossRef CAS PubMed.
- D.-P. Zhang, L.-F. Zhang, G.-L. Li and Z.-H. Ni, Chem. Commun., 2013, 49, 9582–9584 RSC.
- A. Mondal, L.-M. Chamoreau, Y. Li, Y. Journaux, M. Seuleiman and R. Lescouëzec, Chem. – Eur. J., 2013, 19, 7682–7685 CrossRef CAS PubMed.
- N. Bridonneau, L.-M. Chamoreau, P. P. Laine, W. Wernsdorfer and V. Marvaud, Chem. Commun., 2013, 49, 9476–9478 RSC.
- J. Long, L.-M. Chamoreau and V. Marvaud, Dalton Trans., 2010, 39, 2188–2190 RSC.
- J.-W. Zhang, H.-S. Wang and Y. Song, Inorg. Chem. Commun., 2011, 14, 56–60 CrossRef CAS PubMed.
- A. Mondal, Y. Li, L.-M. Chamoreau, M. Seuleiman, L. Rechignat, A. Bousseksou, M.-L. Boillot and R. Lescouezec, Chem. Commun., 2014, 50, 2893–2895 RSC.
- W. Zhang, H.-L. Sun and O. Sato, CrystEngComm, 2010, 12, 4045–4047 RSC.
- Z.-G. Gu, Q.-F. Yang, W. Liu, Y. Song, Y.-Z. Li, J.-L. Zuo and X.-Z. You, Inorg. Chem., 2006, 45, 8895–8901 CrossRef CAS PubMed.
- Z. J. Zhong, H. Seino, Y. Mizobe, M. Hidai, A. Fujishima, S.-i. Ohkoshi and K. Hashimoto, J. Am. Chem. Soc., 2000, 122, 2952–2953 CrossRef CAS.
- J. Larionova, M. Gross, M. Pilkington, H. Andres, H. Stoeckli-Evans, H. U. Güdel and S. Decurtins, Angew. Chem., Int. Ed., 2000, 39, 1605–1609 CrossRef CAS.
- F. Bonadio, M. Gross, H. Stoeckli-Evans and S. Decurtins, Inorg. Chem., 2002, 41, 5891–5896 CrossRef CAS PubMed.
- Y. Song, P. Zhang, X.-M. Ren, X.-F. Shen, Y.-Z. Li and X.-Z. You, J. Am. Chem. Soc., 2005, 127, 3708–3709 CrossRef CAS PubMed.
- S. Chorazy, R. Podgajny, W. Nogas, W. Nitek, M. Koziel, M. Rams, E. Juszynska-Galazka, J. Zukrowski, C. Kapusta, K. Nakabayashi, T. Fujimoto, S.-i. Ohkoshi and B. Sieklucka, Chem. Commun., 2014, 50, 3484–3487 RSC.
- R. Podgajny, S. Chorazy, W. Nitek, M. Rams, A. M. Majcher, B. Marszałek, J. Żukrowski, C. Kapusta and B. Sieklucka, Angew. Chem., Int. Ed., 2013, 52, 896–900 CrossRef CAS PubMed.
- D. E. Freedman, M. V. Bennett and J. R. Long, Dalton Trans., 2006, 2829–2834 RSC.
- J. H. Lim, J. H. Yoon, H. C. Kim and C. S. Hong, Angew. Chem., Int. Ed., 2006, 45, 7424–7426 CrossRef CAS PubMed.
- J. H. Lim, H. S. Yoo, J. H. Yoon, E. K. Koh, H. C. Kim and C. S. Hong, Polyhedron, 2008, 27, 299–303 CrossRef CAS PubMed.
- J. H. Lim, H. S. Yoo, J. I. Kim, J. H. Yoon, N. Yang, E. K. Koh, J.-G. Park and C. S. Hong, Eur. J. Inorg. Chem., 2008, 2008, 3428–3431 CrossRef.
- B. Nowicka, K. Stadnicka, W. Nitek, M. Rams and B. Sieklucka, CrystEngComm, 2012, 14, 6559–6564 RSC.
- S.-L. Ma, S. Ren, Y. Ma, D.-Z. Liao and S.-P. Yan, Struct. Chem., 2009, 20, 161–167 CrossRef CAS PubMed.
- J. Wang, Z.-C. Zhang, H.-S. Wang, L.-C. Kang, H.-B. Zhou, Y. Song and X.-Z. You, Inorg. Chem., 2010, 49, 3101–3103 CrossRef CAS PubMed.
- L. M. C. Beltran and J. R. Long, Acc. Chem. Res., 2005, 38, 325–334 CrossRef CAS PubMed.
- C. P. Berlinguette and K. R. Dunbar, Chem. Commun., 2005, 2451–2453 RSC.
- A. Lachgar, J. J. Zhang, S. A. Gamboa and B. J. Davis, Adv. Mater. Sci. Eng., 2009, 2009 Search PubMed.
- A. K. Cheetham, C. N. R. Rao and R. K. Feller, Chem.Commun., 2006, 4780–4795 RSC.
- E. Ruiz, G. Rajaraman, S. Alvarez, B. Gillon, J. Stride, R. Clérac, J. Larionova and S. Decurtins, Angew. Chem., Int. Ed., 2005, 44, 2711–2715 CrossRef CAS PubMed.
- Y.-Q. Zhang and C.-L. Luo, Dalton Trans., 2008, 4575–4584 RSC.
- Y.-Q. Zhang and C.-L. Luo, Inorg. Chem., 2009, 48, 10486–10488 CrossRef CAS PubMed.
- M. Fitta, M. Bałanda, M. Mihalik, R. Pełka, D. Pinkowicz, B. Sieklucka and M. Zentkova, J. Phys.: Condens. Matter, 2012, 24 Search PubMed.
|
This journal is © the Partner Organisations 2015 |
Click here to see how this site uses Cookies. View our privacy policy here.