DOI:
10.1039/C5RA16289K
(Paper)
RSC Adv., 2015,
5, 75555-75568
Catalytic applications of {[HMIM]C(NO2)3}: as a nano ionic liquid for the synthesis of pyrazole derivatives under green conditions and a mechanistic investigation with a new approach†
Received
12th August 2015
, Accepted 28th August 2015
First published on 28th August 2015
Abstract
Catalytic applications of 1-methylimidazolium trinitromethanide {[HMIM]C(NO2)3}as the first nano ionic liquid (NIL) was studied. The described NIL was efficiently tested in the synthesis of 5-amino-pyrazole-4-carbonitrile derivatives in good to excellent yields on the three-component condensation of various aromatic aldehydes, malononitrile and phenylhydrazine at room temperature under solvent-free and ambient conditions. In the next study, 1,4-dihydropyrano-[2,3-c]-pyrazole derivatives were also synthesized via four-component condensation of a good range of aromatic aldehydes, malononitrile, phenylhydrazine and ethyl acetoacetate in the presence of NIL under the same reaction conditions. The described reactions were in good agreement with the green chemistry disciplines and their major advantages are short reaction times, good yields, ease of separation, recycling and reusability of NIL. A new mechanistic approach was proposed for the final step of the pyrazoles synthesis which was supported by theoretical studies.
Introduction
Ionic liquids (ILs) are commonly introduced as liquid electrolytes composed completely of ions, and occasionally a melting point criterion has been suggested to identify between molten salts and ionic liquids (mp 100 °C). Although, both molten salts and ionic liquids are better defined as salts that show ionic covalent crystalline structures. Over the last few years, ionic liquids have effectively been useful as alternative solvents for homogeneous biphasic catalysis.1–6 The increased interest in ionic liquids through chemists and technologists clearly is due to the efficiency of ionic liquids as solvents in chemical processes and counting catalytic reactions. The industrial for “green” solvents is more demand. Many chemists now understand that ionic liquids have dual roles and could be acts both as solvent and catalyst. Ionic liquids are materials that are entirely composed of ions and are liquid at or close to room temperature.7 Attention in these compounds, frequently heralded as the green, their thermal stability,8 is still increasing rapidly,9 stems from their near-zero vapor pressure,10 and their broadly harmonic properties as concerns polarity, hydrophobicity, and solvent miscibility behavior via suitable modification of the cation and the anion.
Multi-component reactions (MCRs) have occurred as a powerful tool for the construction of new and complex molecular structures because of their advantages over conventional multi-step synthesis. The major advantages of MCRs contain shorter reaction times, lower costs, high atom-economy, energy saving, the avoidance of time consuming and expensive purification procedures. It is recognized that MCRs are commonly much more eco-friendly, and suggestion rapid availability to large compound libraries with diverse functionalities.11–14
Pyrazoles are the significant kind of heterocyclic compounds that feature in several pharmaceutical targets and natural products of medicinal interest. Pyrazole derivatives have showed a wide variety of biological activities counting anti HIV,15 anti-malarial,16 antibacterial and antifungal,17 anticancer,18 anti-inflammatory,19 antioxidant,17,20 and anti-depressant activities.21 The prominence and importance of pyrazoles in drug discovery has frequently concerned the following for to improved methods.22 Recent processes for the synthesis of 5-amino-pyrazole-4-carbonitrile and 1,4-dihydropyrano-[2,3-c]-pyrazole contain synthesis based on the procedure of Ti(NMe2)2(PyPyr)2,23 I2,24 Sc(OTf)3,25 water or ethanol media,26–28 ZrO2 nanoparticles,29 LiOH,30 CuO/ZrO2,31 [BMIM]OH32 and triethylbenzylammonium chloride.33
Very recently, we have been reported the first nano ionic liquid (NIL) namely 1-methylimidazolium trinitromethanide {[HMIM]C(NO2)3} and its catalytic uses for Hantzsch four component condensation.34 In continuation of our previously reported procedure for the preparation of 1,3,5-trisubstituted pyrazolines35 and pyranopyrazoles,36 here, we wish to describe an efficient and benign synthesis of 5-amino-pyrazole-4-carbonitrile (5) via one-pot three-component condensation of various aromatic aldehydes (1), malononitrile (2) and phenylhydrazine (3) at room temperature under solvent-free and ambient conditions (Scheme 1a). Furthermore, synthesis of 1,4-dihydropyrano-[2,3-c]-pyrazole (6) by a one-pot four-component approach the reaction between several aromatic aldehydes (1), malononitrile (2), phenylhydrazine (3) and ethyl acetoacetate (4) under same conditions, was studied (Scheme 1b).
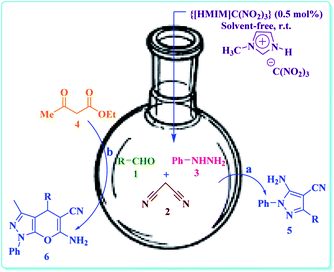 |
| Scheme 1 The synthesis of 5-amino-pyrazole-4-carbonitrile derivatives (a) and 1,4-dihydropyrano-[2,3-c]-pyrazole (b) in the presence of {[HMIM]C(NO2)3} as nano structure ionic liquid catalyst. | |
Results and discussion
Development of task specific ionic liquids (TSILs) and their structural diversity could be achieved via designing and synthesis of novel cationic cores with suitable anionic counterparts. By considering the above-mentioned synthetic strategy, in the course of a decade of our investigation on designing and synthesis of TSILs lead to report a good range of TSILs.34,37
Firstly, 1-methylimidazolium trinitromethanide {[HMIM]C(NO2)3} as a first reported nano ionic liquid (NIL), has been prepared according to our previously reported procedure. Then, optimization of the reaction conditions was followed. Finally, the influence of the nano ionic liquid catalyst in the synthesis of 5-amino-pyrazole-4-carbonitrile derivatives and 1,4-dihydropyrano-[2,3-c]-pyrazole derivatives was investigated (Scheme 1). For this intention, as a model, the condensation reaction of naphthalene-1-carbaldehyde, malononitrile and phenylhydrazine (in the synthesis of 5-amino-pyrazole-4-carbonitrile derivatives) and naphthalene-1-carbaldehyde, malononitrile, phenylhydrazine and ethyl acetoacetate (in the synthesis of 1,4-dihydropyrano-[2,3-c]-pyrazole derivatives) were considered using various amounts of the catalyst at range of 25–100 °C under solvent-free conditions (Table 1). As Table 1, show that, the best results were attained when the reaction was achieved in the presence of 0.5 mol% of NIL as a catalyst at room temperature (Table 1, entry 3). In the absence of catalyst a low yield of the products were achieved after 1 h (Table 1, entries 1 and 2). Increasing the reaction temperature and catalyst loading did not improve the rate of the reaction (Table 1, entries 4–14).
Table 1 Effect of amount of the catalyst and temperature under solvent-free conditionsa
Entry |
Catalyst loading (mol%) |
Reaction temperature (°C) |
Reaction time (min) |
Yieldb (%) |
Pathway a |
Pathway b |
Pathway a |
Pathway b |
Reaction conditions: pathway a: naphthalene-1-carbaldehyde (1 mmol), malononitrile (1 mmol), phenylhydrazine (1 mmol); pathway b: naphthalene-1-carbaldehyde (1 mmol), malononitrile (1 mmol), phenylhydrazine (1 mmol), ethyl acetoacetate (1 mmol). Isolate yield. |
1 |
Catalyst-free |
r.t. |
60 |
60 |
18 |
15 |
2 |
Catalyst-free |
100 |
60 |
60 |
21 |
17 |
3 |
0.5 |
r.t. |
10 |
12 |
95 |
94 |
4 |
0.5 |
50 |
10 |
12 |
95 |
94 |
5 |
0.5 |
75 |
10 |
12 |
95 |
94 |
6 |
0.5 |
100 |
10 |
12 |
95 |
94 |
7 |
1 |
r.t. |
10 |
12 |
95 |
94 |
8 |
1 |
100 |
10 |
12 |
95 |
94 |
9 |
2 |
r.t. |
10 |
12 |
95 |
94 |
10 |
2 |
100 |
10 |
12 |
95 |
94 |
11 |
5 |
r.t. |
10 |
12 |
94 |
92 |
12 |
5 |
100 |
10 |
12 |
94 |
92 |
13 |
10 |
r.t. |
15 |
15 |
93 |
90 |
14 |
10 |
100 |
15 |
15 |
93 |
90 |
To compare the proficiency of the solution versus solvent-free conditions, a mixture of naphthalene-1-carbaldehyde, malononitrile and phenylhydrazine (in the synthesis of 5-amino-pyrazole-4-carbonitrile derivatives) and naphthalene-1-carbaldehyde, malononitrile, phenylhydrazine and ethyl acetoacetate (in the synthesis of 1,4-dihydropyrano-[2,3-c]-pyrazole derivatives) as model reaction, in the presence of 0.5 mol% of NIL as a catalyst in some several solvents such as H2O, C2H5OH, CH2Cl2, CH3CN, CH3CO2Et and toluene was considered at room temperature. The results are summarized in Table 2. As it can be realized in Table 2, solvent-free condition was the best conditions in this reaction.
Table 2 The effect of various solvents in the presence of 0.5 mol% of nano structure ionic liquid at room temperaturea
Entry |
Solvent |
Reaction time (min) |
Yieldb (%) |
Pathway a |
Pathway b |
Pathway a |
Pathway b |
Reaction conditions: pathway a: naphthalene-1-carbaldehyde (1 mmol), malononitrile (1 mmol), phenylhydrazine (1 mmol); pathway b: naphthalene-1-carbaldehyde (1 mmol), malononitrile (1 mmol), phenylhydrazine (1 mmol), ethyl acetoacetate (1 mmol). Isolate yield. |
1 |
Solvent-free |
10 |
12 |
95 |
94 |
2 |
H2O |
15 |
20 |
95 |
94 |
3 |
C2H5OH |
15 |
20 |
95 |
94 |
4 |
CH3CN |
20 |
25 |
94 |
92 |
5 |
CH2Cl2 |
30 |
30 |
88 |
85 |
6 |
CH3CO2Et |
45 |
45 |
85 |
80 |
7 |
Toluene |
60 |
60 |
75 |
70 |
Encouraged by the considerable results and with the aim of verification to overview the scope of this main process, several 5-amino-pyrazole-4-carbonitrile derivatives were synthesized from three-component condensation reaction of a range of aromatic aldehyde, malononitrile and phenylhydrazine under solvent-free conditions at room temperature in the presence of a catalytic amount of NIL as a catalyst. Consequently, various 1,4-dihydropyrano-[2,3-c]-pyrazole derivatives were synthesized via four-component condensation reaction of several aromatic aldehyde, malononitrile, phenylhydrazine and ethyl acetoacetate under same reaction conditions. The results have been represented in Tables 3 and 4. The effect of substituents on the aromatic ring shows estimated strong effects in terms of yields under these reaction conditions. All aromatic aldehydes with electron-withdrawing and electron-releasing groups on their aromatic ring afforded the related products in high to excellent yields in short reaction times. The reaction times of aromatic aldehydes having electron withdrawing groups were rather faster than electron donating groups.
Table 3 The three-component synthesis of 5-amino-pyrazole-4-carbonitrile derivatives in the presence of 0.5 mol% of {[HMIM]C(NO2)3} as a nano structure ionic liquid catalysta
Entry |
Aldehyde |
Product |
Time (min) |
Yieldb (%) |
Mp (°C) [Lit.]Ref. |
Reaction conditions: aldehyde (1 mmol), malononitrile (1 mmol), phenylhydrazine (1 mmol). Isolate yield. |
1 |
 |
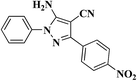 |
8 |
97 |
172–174 [164–166]24 |
2 |
 |
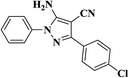 |
10 |
95 |
227–229 [128–130]24 |
3 |
 |
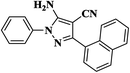 |
10 |
95 |
162–164 |
4 |
 |
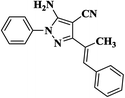 |
20 |
91 |
237–239 |
5 |
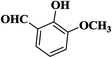 |
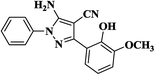 |
20 |
92 |
281–283 |
6 |
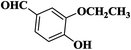 |
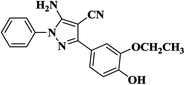 |
20 |
92 |
221–223 |
7 |
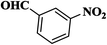 |
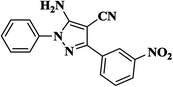 |
12 |
94 |
178–180 [128–130]24 |
8 |
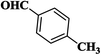 |
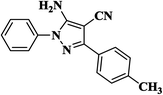 |
15 |
93 |
173–175 [118–120]24 |
9 |
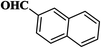 |
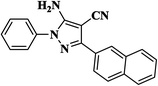 |
10 |
94 |
275–277 |
10 |
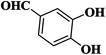 |
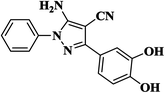 |
25 |
91 |
246–248 [212–213]31 |
11 |
 |
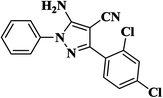 |
15 |
94 |
245–247 |
12 |
 |
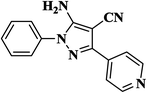 |
25 |
91 |
224–226 |
Table 4 The four-component synthesis of 1,4-dihydropyrano-[2,3-c]-pyrazole derivatives in the presence of 0.5 mol% of {[HMIM]C(NO2)3} as a nano structure ionic liquid catalysta
Entry |
Aldehyde |
Product |
Time (min) |
Yieldb (%) |
Mp (°C) [Lit.]Ref. |
Reaction conditions: aldehyde (1 mmol), malononitrile (1 mmol), phenylhydrazine (1 mmol), ethyl acetoacetate (1 mmol). Isolate yield. |
1 |
 |
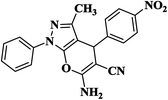 |
10 |
95 |
204–206 [192–194]33 |
2 |
 |
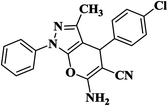 |
15 |
93 |
183–185 [177–178]33 |
3 |
 |
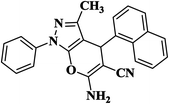 |
12 |
94 |
235–237 [222–224]29 |
4 |
 |
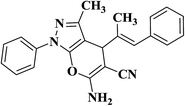 |
25 |
89 |
267–269 |
5 |
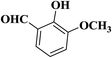 |
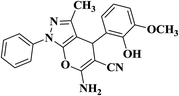 |
30 |
90 |
297–299 |
6 |
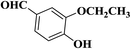 |
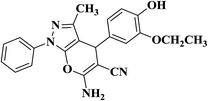 |
30 |
89 |
175–177 |
7 |
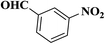 |
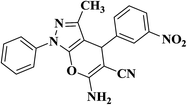 |
15 |
93 |
207–209 |
8 |
 |
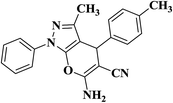 |
18 |
91 |
179–181 [173–175]33 |
9 |
 |
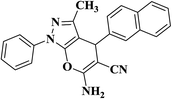 |
15 |
93 |
206–208 |
10 |
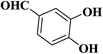 |
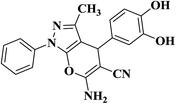 |
35 |
88 |
189–191 |
11 |
 |
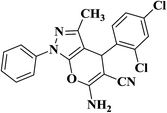 |
17 |
91 |
231–233 [182–184]33 |
12 |
 |
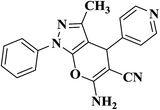 |
30 |
88 |
249–251 |
In Scheme 2 we introduce a possible mechanism for the synthesis of 5-amino-pyrazole-4-carbonitrile derivatives (5) in the presence of {[HMIM]C(NO2)3} as a nano structure ionic liquid catalyst. The reaction occurs via initial formation of arylidene malononitrile (10) in quantitative yield through the Knoevenagel addition of activated malononitrile (2) to the activated aromatic aldehyde (1) and followed by loss of water molecules. The formation of the 5-amino-pyrazole-4-carbonitrile derivatives (5) is suggested to include the reaction between arylidene malononitrile (10) and phenylhydrazine (3), followed by Michael addition, intermolecular cyclisation. Previously reported studies have been suggested aerobic auto oxidation of 5-amino-pyrazolidine-4-carbonitrile (14) to its corresponding 5-amino-pyrazole-4-carbonitrile (5). In contrast to the previously reported mechanistic description for the final step of the above described organic synthesis,24,25,31 we believed that, this step might be proceed through unusual hydride transfer as well as Cannizzaro reaction (Fig. S98†)38 and H2 releasing from tricyclic orthoamide (16) which presented in Scheme 3.39 For improving of this idea, reaction was occurred under nitrogen atmosphere and in the absence of any molecular oxygen. It was observed that, the reaction proceeded under atmosphere of nitrogen as well as normal reaction condition in the presence of oxygen. By considering the above-mentioned evidence, conversion of 5-amino-pyrazolidine-4-carbonitrile (14) to its corresponding 5-amino-pyrazole-4-carbonitrile (5) might be occurred through unusual hydride transfer and releasing of molecular hydrogen (H2). The C–H bond is so weakened by electron donation from the nitrogen lone pairs into the anti-bonding of C–H (σ*C–H orbital) which it can be broken via reaction with a proton to give molecular hydrogen. As we know, this phenomena has been named as anomeric effect.
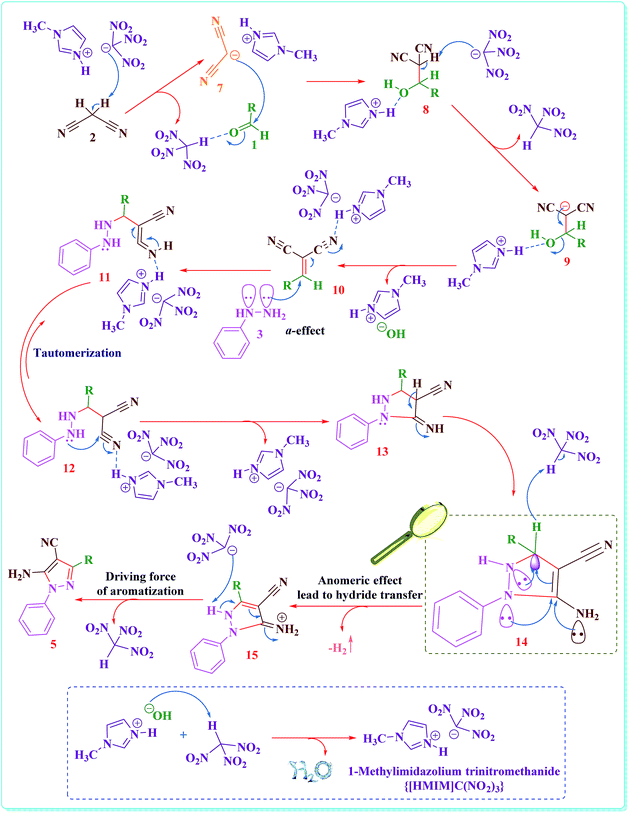 |
| Scheme 2 The suggested mechanism for the synthesis of 5-amino-pyrazole-4-carbonitrile derivatives in the presence of {[HMIM]C(NO2)3} as a nano structure ionic liquid catalyst. | |
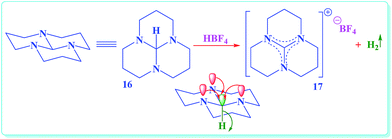 |
| Scheme 3 A striking example which had been observed for an unusual hydride transfer from tricyclic orthoamide (16) through anomeric effect.39 | |
To improvement a deeper kinetic and thermodynamic understanding of synthesis of 5-amino-pyrazole-4-carbonitrile derivatives DFT calculations were performed (Fig. 1). Starting from 13, two different orientations of adjacent N–H and C–H groups on pyrazole ring with respect to each other give cis and trans like isomers. Our calculations show that the trans like isomer is about 2 kcal mol−1 more stable than cis one. Fig. 1 shows energy profile for more stable trans isomer. As can be seen, at first the intermediate 14 forms which are slightly more stable than 13. Then through the formation of a logical transition structure and then removing the molecular hydrogen (H2) the zwitterionic intermediate 15 will be formed. According to values of calculated Gibbs free energies this reaction is about −3.10 and −2.27 kcal mol−1 exothermic for cis and trans isomers, respectively. On the other hand, because of the delocalization of lone pair of adjacent nitrogen atom and π electrons to σ* C–H bond (the anomeric effect) the C–H bond in compound 14 is so weakened and the activation energy is relatively small. The NBO analysis of donor–acceptor interactions identified that the anomeric effect due to delocalization of lone pair of nitrogen atom to σ* C–H bond for cis and trans isomers of intermediate 14 is 3.84 and 3.82 kcal mol−1, respectively. The delocalization from the π electron of adjacent double bond is 4.69 and 4.43 kcal mol−1 for cis and trans isomers, respectively. The main donor–acceptor interaction for pyrazole ring in intermediate 14 is shown in Table 5. In the final step of the reaction the zwitterionic intermediate 15 converts to 5-amino-pyrazole-4-carbonitrile (5) through an exothermic process (ΔG = −19.85 kcal mol−1). In conclusion, the whole process of conversion of 13 to 5 through the releasing molecular hydrogen is exothermic (ΔG = −24.64 kcal mol−1). Thus the above theoretical studies support our suggested mechanism and shows that releasing molecular hydrogen (H2) is quite possible in such systems. The optimized structure of all compounds involved in our suggested mechanism is shown in Fig. 2.
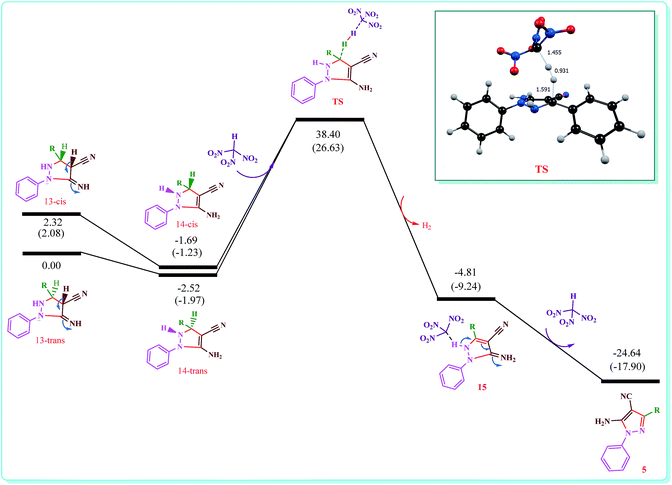 |
| Fig. 1 Energy profile calculated for synthesis of 5-amino-pyrazole-4-carbonitrile derivatives (5) beginning from compound 13 (see Scheme 2). The relative Gibbs free energy and corrected electronic energies (Figures in parentheses) obtained from the B3LYP/TZVP calculations both are given in kcal mol−1. The phenyl group is shown as R. | |
Table 5 The main second order perturbation energies (kcal mol−1) calculated for pyrazol ring in intermediate 14
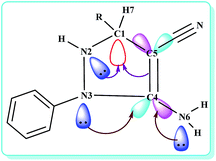
|
Donor–acceptor interactions |
14-cis |
14-trans |
nb N2 → σ* C1–H7 |
3.84 |
3.82 |
π C4–C5 → σ* C1–H7 |
4.69 |
4.43 |
nb N6 → π* C4–H5 |
38.53 |
39.79 |
nb N6 → σ* C4–H5 |
1.02 |
— |
nb N3 → π* C4–H5 |
30.94 |
35.03 |
nb N3 → σ* C4–H5 |
— |
0.52 |
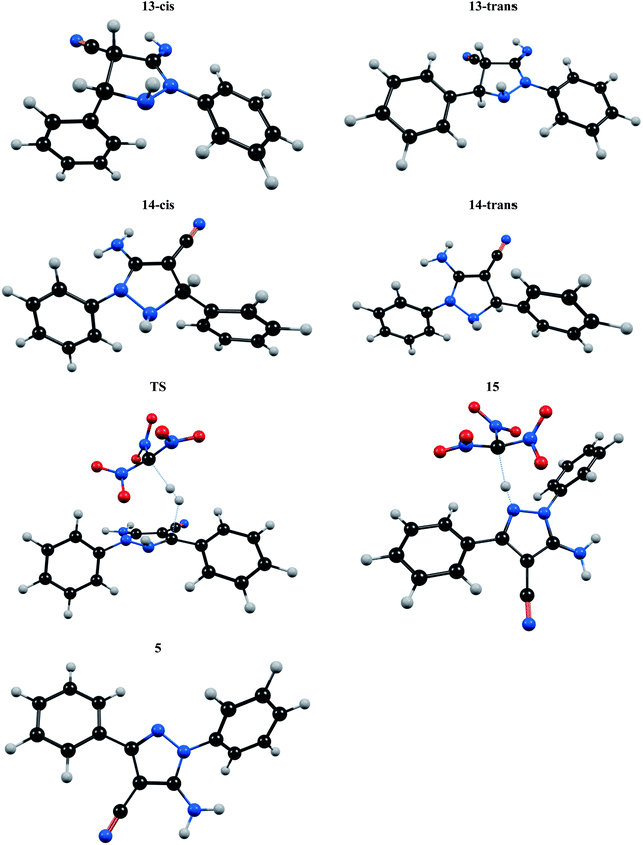 |
| Fig. 2 The optimized structure of all compounds involved in conversion of 13 to 5 according to the mechanism suggested in Fig. 1. | |
The proposed catalytic method are recommend in Scheme 4, which are dependable with literature reports.40,41 The reaction happen through primary formation of arylidene malononitrile (10) in quantitative yield via the Knoevenagel addition of activated malononitrile (2) to the activated aromatic aldehyde (1) and followed by loss of water molecules. The formation of the 1,4-dihydropyrano-[2,3-c]-pyrazole (6) is proposed to contain the following tandem reactions: pyrazolone (20) formation by reaction between phenylhydrazine (3) and activated ethyl acetoacetate (4), Michael addition of pyrazolone (20) to arylidene malononitrile (10), followed via cyclization and tautomerization (Scheme 4).
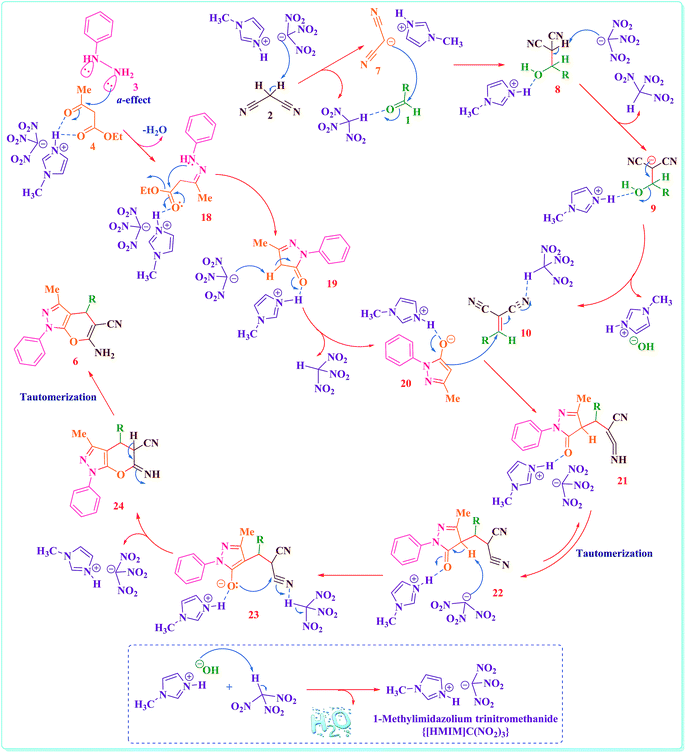 |
| Scheme 4 The proposed mechanism for the synthesis of 1,4-dihydropyrano-[2,3-c]-pyrazole derivatives in the presence of {[HMIM]C(NO2)3} as a nano structure ionic liquid catalyst. | |
Additionally, reusability of the NIL catalyst was corroborated aboard the condensation of naphthalene-1-carbaldehyde, malononitrile and phenylhydrazine (in the synthesis of 5-amino-pyrazole-4-carbonitrile derivatives) and naphthalene-1-carbaldehyde, malononitrile, phenylhydrazine and ethyl acetoacetate (in the synthesis of 1,4-dihydropyrano-[2,3-c]-pyrazole derivatives). At the end of the reaction, ethyl acetate was added to the reaction mixture and heated to extract product from remained starting materials. This solution was washed with water to separate catalyst from other materials (the product is soluble in hot ethyl acetate and NIL catalyst is soluble in water). The aqueous layer was decanted, separated and used for further reaction after removing of water. It have been detected that the catalytic activity of the catalyst was restored within the limits of the experimental errors for five continuous runs (Fig. 3). The deactivation of the nano ionic liquid catalyst is low, while reactant was expected. The reaction was scaled up to 10 mmol of reactants in the presence of 5 mol% of NIL catalyst at room temperature. The yield of the reaction was 95% after 10 min and 91% after the fifth run (in the synthesis of 5-amino-pyrazole-4-carbonitrile derivatives) and 94% after 12 min and 89% after the fifth run (in the synthesis of 1,4-dihydropyrano-[2,3-c]-pyrazole derivatives). The results were summarized in Fig. 3.
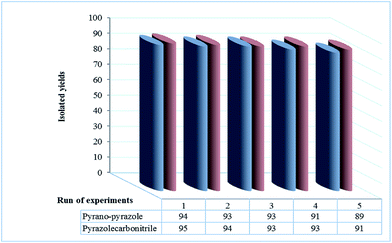 |
| Fig. 3 Reusability of the nano structure ionic liquid catalyst in the synthesis of 5-amino-pyrazole-4-carbonitrile derivatives (in 10 minutes) and in the synthesis of 1,4-dihydropyrano-[2,3-c]-pyrazole derivatives (in 12 minutes). | |
In continuation of our study into the application of nano structure ionic liquid catalyst in the synthesis of 5-amino-pyrazole-4-carbonitrile derivatives, we studied the efficacy of this ionic liquid catalyst (an approval of suggested mechanism) is comparable with various catalysts. To optimize the reaction conditions, the reaction between 3-ethoxy-4-hydroxybenzaldehyde, malononitrile and phenylhydrazine under N2 atmosphere at room temperature was used as a typical (Table 6).
Table 6 The synthesis of 5-amino-pyrazole-4-carbonitrile in the presence of different catalyst for an approval of suggested mechanisma
Entry |
Catalyst |
Amount of catalyst (mol%) |
Time (min) |
Yieldb (%) |
Reaction conditions: 3-ethoxy-4-hydroxybenzaldehyde (1 mmol), malononitrile (1 mmol), phenylhydrazine (1 mmol), under N2 atmosphere, r.t. Isolate yield. |
1 |
{[HMIM]C(NO2)3} |
0.5 |
20 |
92 |
2 |
HBF4 |
10 |
30 |
85 |
3 |
Ce(HSO4)3·7H2O |
10 |
60 |
83 |
4 |
Fe(HSO4)3 |
12 |
60 |
79 |
5 |
NH2SO3H |
10 |
60 |
85 |
6 |
[MSIM]Cl |
1 |
60 |
87 |
7 |
Zn(HSO4)2 |
10 |
120 |
51 |
8 |
Ca(HSO4)2 |
15 |
120 |
47 |
9 |
BiCl3 |
10 |
120 |
41 |
10 |
H3PW12O40 |
1 |
180 |
— |
11 |
ZrOCl2 |
10 |
180 |
— |
12 |
B(OH)3 |
10 |
180 |
— |
13 |
Oxone |
5 |
180 |
— |
14 |
Bi(HSO4)3 |
10 |
180 |
— |
15 |
Al(HSO4)3 |
12 |
180 |
— |
Conclusions
In summary, a green, effective and environmentally friendly NIL catalyst namely 1-methylimidazolium trinitromethanide {[HMIM]C(NO2)3} was used and considered in the synthesis of 5-amino-pyrazole-4-carbonitrile derivatives by three-component condensation reaction of various aromatic aldehydes, malononitrile and phenylhydrazine under solvent-free conditions at room temperature. Subsequently, 1,4-dihydropyrano-[2,3-c]-pyrazole derivatives were also synthesized via four-component condensation between several aromatic aldehydes, malononitrile, phenylhydrazine and ethyl acetoacetate under same reaction conditions. The presented mechanisms revealed that the buffer ability of nano ionic liquid, probably plays the key and dual catalytic roles in the described reactions. Finally, considerable advantages of the presented methodology and/or investigation are reasonably high yield, cleaner reaction profile, short reaction time, simple work up, recycle and reusability of the nano structure ionic liquid catalyst which makes it in close agreement with the green chemistry disciplines. A new mechanistic approach was also proposed for the final step of the pyrazoles synthesis which was supported by theoretical studies. Therefore, we thought that the suggested mechanism have potential for entering into the graduate text book in the future.
Experimental
General procedure for the preparation of nano structure ionic liquid catalyst: 1-methylimidazolium trinitromethanide
The nano ionic liquid {[HMIM]C(NO2)3} as a catalyst was synthesized according to our previously reported procedure.34 A yellow solid was formed in high purity and then the physical data of these known nano structure ionic liquid was found to be identical. Mp: 54–56 °C; yield: 97% (0.679 g); spectral data: IR (KBr): ν 3433, 3144, 1584, 1383 cm−1; 1H NMR (400 MHz, DMSO-d6): δ 3.87 (s, 3H, –CH3), 7.68 (d, 1H, J = 3.2 Hz, –CH); 7.71 (d, 1H, J = 3.2 Hz, –CH), 9.08 (s, 1H, –CH), 14.23 (brs, 1H, –NH); 13C NMR (100 MHz, DMSO-d6): δ 35.8, 46.5, 120.2, 123.6, 136.3; MS: m/z = 233 [M]+, 234 [M + H]+.
General procedure for the synthesis of 5-amino-pyrazole-4-carbonitrile derivatives
A mixture of various aromatic aldehydes (1 mmol), malononitrile (1 mmol) and phenylhydrazine (1 mmol) in a round bottom flask, was added {[HMIM]C(NO2)3} as a nano structure ionic liquid catalyst (0.5 mol%; 0.117 g), and the subsequent mixture was firstly stirred magnetically under solvent-free conditions at room temperature. After completion of the reaction, as monitored by TLC n-hexane/ethyl acetate (5
:
3), ethyl acetate (10 mL) was added to reaction mixture, stirred and refluxed for 3 min, and then was washed with water (10 mL) and decanted to separate catalyst from the other materials (the reaction mixture was soluble in hot ethyl acetate and nano structure molten salt catalyst was soluble in water). The aqueous layer was decanted and catalyst separated after removing of water. The remained catalyst was used for alternative reaction. The solvent of organic layer was evaporated and the crude product was purified by recrystallization from ethanol/water (10
:
1). In this study, nano structured ionic liquid catalyst was recycled and reused for five times without significant loss of its catalytic activity.
General procedure for the synthesis of 1,4-dihydropyrano-[2,3-c]-pyrazole derivatives
A mixture of various aromatic aldehydes (1 mmol), malononitrile (1 mmol), phenylhydrazine (1 mmol) and ethyl acetoacetate (1 mmol) in a round bottom flask, was added {[HMIM]C(NO2)3} as a nano structure ionic liquid catalyst (0.5 mol%; 0.117 g), and the subsequent mixture was firstly stirred magnetically under solvent-free conditions at room temperature. After completion of the reaction, as observed by TLC n-hexane/ethyl acetate (5
:
2), ethyl acetate (10 mL) was added to reaction mixture, stirred and refluxed for 3 min, and then was washed with water (10 mL) and decanted to separate catalyst from the other materials (the reaction mixture was soluble in hot ethyl acetate and nano structure ionic liquid catalyst was soluble in water). The aqueous layer was decanted and catalyst separated after removing of water. The remained catalyst was used for alternative reaction. The solvent of organic layer was evaporated and the crude product was purified by recrystallization from ethanol/water (10
:
1). In this work, nano structure ionic liquid catalyst was recycled and reused for five times without significant loss of its catalytic activity.
Spectral data analysis for compounds
5-Amino-3-(naphthalen-1-yl)-1-phenyl-1H-pyrazole-4-carbonitrile (Table 3, entry 3). Yellow solid; mp: 162–164 °C; yield: 95%; IR (KBr): ν 3554, 3475, 3415, 3237, 3027, 2226, 1637, 1616, 1566, 1384, 1371 cm−1; 1H NMR (400 MHz, DMSO-d6): δppm 7.71 (t, 6H, J = 7.4 Hz, ArH), 8.11 (d, 2H, J = 7.2 Hz, ArH), 8.22 (d, 1H, J = 7.2 Hz, ArH), 8.28 (d, 2H, J = 8.4 Hz, ArH), 9.40 (s, 2H, –NH2); 13C NMR (100 MHz, DMSO-d6): δppm 85.5, 100.8, 101.5, 113.7, 114.5, 124.3, 125.9, 127.7, 128.6, 129.0, 129.4, 131.1, 133.5, 134.6, 160.8, 166.1, 167.3.
5-Amino-1-phenyl-3-(1-phenylprop-1-en-2-yl)-1H-pyrazole-4-carbonitrile (Table 3, entry 4). Yellow solid; mp: 237–239 °C; yield: 91%; IR (KBr): ν 3550m, 3462, 3418, 3237, 2987, 2208, 1634, 1515, 1384, 1266 cm−1; 1H NMR (400 MHz, DMSO-d6): δppm 2.00 (s, 3H, –CH3), 3.92 (s, 1H, –CH), 7.51 (t, 6H, J = 8.0 Hz, ArH), 7.66 (d, 4H, J = 7.6 Hz, ArH), 9.60 (s, 2H, –NH2); 13C NMR (100 MHz, DMSO-d6): δppm 33.9, 67.4, 114.7, 114.9, 126.1, 126.2, 126.7, 126.9, 127.4, 127.5, 129.0, 129.3, 129.4, 129.6, 137.4, 137.5, 137.6, 166.1.
5-Amino-3-(2-hydroxy-3-methoxyphenyl)-1-phenyl-1H-pyrazole-4-carbonitrile (Table 3, entry 5). Orange solid; mp: 281–283 °C; yield: 92%; IR (KBr): ν 3545, 3483, 3412, 3237, 2217, 1638, 1618, 1498, 1347, 1246 cm−1; 1H NMR (400 MHz, DMSO-d6): δppm 3.37 (s, 3H, –OCH3), 6.55 (s, 1H, –OH), 7.39 (t, 4H, J = 5.9 Hz, ArH), 7.48 (s, 2H, –NH2), 7.77 (d, 4H, J = 5.9 Hz, ArH); 13C NMR (100 MHz, DMSO-d6): δppm 24.1, 63.9, 116.0, 116.1, 126.0, 126.1, 126.2, 126.8, 126.9, 127.1, 127.5, 127.8, 128.9, 129.3, 129.4, 129.5, 168.1.
5-Amino-3-(3-ethoxy-4-hydroxyphenyl)-1-phenyl-1H-pyrazole-4-carbonitrile (Table 3, entry 6). Orange solid; mp: 221–223 °C; yield: 92%; IR (KBr): ν 3554, 3468, 3412, 3237, 2978, 2181, 1639, 1608, 1569, 1384, 1274 cm−1; 1H NMR (400 MHz, DMSO-d6): δppm 1.33 (t, 3H, J = 7.0 Hz, –CH3), 4.04 (q, 2H, J = 6.8 Hz, –CH2), 6.54 (d, 1H, J = 8.0 Hz, ArH), 6.70 (d, 2H, J = 8.4 Hz, ArH), 6.79 (d, 1H, J = 8.0 Hz, ArH), 6.86 (s, 1H, –OH), 7.01 (t, 1H, J = 8.4 Hz, ArH), 7.45 (t, 2H, J = 8.4 Hz, ArH), 7.54 (s, 1H, ArH), 7.95 (s, 2H, –NH2); 13C NMR (100 MHz, DMSO-d6): δppm 14.6, 28.5, 64.0, 114.9, 125.4, 125.6, 126.3, 126.4, 126.5, 126.6, 128.0, 131.1, 133.2, 147.1, 151.2, 151.3, 159.0, 159.1.
5-Amino-3-(naphthalen-2-yl)-1-phenyl-1H-pyrazole-4-carbonitrile (Table 3, entry 9). Yellow solid; mp: 275–277 °C; yield: 94%; IR (KBr): ν 3550, 3470, 3411, 3358, 3237, 2983, 2219, 1638, 1559, 1384, 1283 cm−1; 1H NMR (400 MHz, DMSO-d6): δppm 7.33 (s, 1H, ArH), 7.52 (t, 5H, J = 9.2 Hz, ArH), 7.88 (d, 6H, J = 9.2 Hz, ArH), 8.87 (s, 2H, –NH2); 13C NMR (100 MHz, DMSO-d6): δppm 59.5, 144.6, 125.3, 125.4, 125.6, 126.0, 126.1, 126.3, 126.4, 126.9, 127.7, 127.8, 128.5, 131.7, 131.9, 132.0, 133.2, 133.3, 133.4, 167.4.
5-Amino-3-(2,4-dichlorophenyl)-1-phenyl-1H-pyrazole-4-carbonitrile (Table 3, entry 11). Yellow solid; mp: 245–247 °C; yield: 94%; IR (KBr): ν 3463, 3400, 3233, 2983, 2206, 1645, 1577, 1384 cm−1; 1H NMR (400 MHz, DMSO-d6): δppm 7.06 (s, 1H, ArH), 7.40 (t, 3H, J = 8.4 Hz, ArH), 7.45 (d, 1H, J = 8.4 Hz, ArH), 7.51 (d, 2H, J = 8.4 Hz, ArH), 7.61 (d, 2H, J = 8.4 Hz, ArH), 7.86 (s, 2H, –NH2); 13C NMR (100 MHz, DMSO-d6): δppm 65.0, 112.7, 112.8, 125.6, 125.7, 126.1, 127.4, 127.7, 127.8, 128.1, 132.0, 133.3, 145.3, 151.8, 152.0, 165.4.
5-Amino-1-phenyl-3-(pyridin-4-yl)-1H-pyrazole-4-carbonitrile (Table 3, entry 12). Brown solid; mp: 221–223 °C; yield: 92%; IR (KBr): ν 3550, 3462, 3416, 3229, 2987, 2194, 1639, 1615, 1384 cm−1; 1H NMR (400 MHz, DMSO-d6): δppm 7.05 (s, 2H, –NH2), 7.21 (d, 2H, J = 6.0 Hz, ArH), 7.41 (t, 1H, J = 7.8 Hz, ArH), 7.82 (d, 2H, J = 7.2 Hz, ArH), 8.46 (t, 1H, J = 6.2 Hz, ArH), 8.53 (d, 2H, J = 6.0 Hz, ArH), 8.77 (t, 1H, J = 5.6 Hz, ArH); 13C NMR (100 MHz, DMSO-d6): δppm 60.8, 123.1, 125.5, 125.7, 126.2, 126.5, 126.6, 127.0, 128.0, 131.2, 133.4, 138.4, 145.4, 150.1, 165.6.
6-Amino-3-methyl-4-(naphthalen-1-yl)-1-phenyl-1,4-dihydropyrano[2,3-c]pyrazole-5-carbonitrile (Table 4, entry 3). Yellow solid; mp: 235–237 °C; yield: 94%; IR (KBr): ν 3550, 3470, 3415, 3241, 3062, 2197, 1600, 1498, 1370 cm−1; 1H NMR (400 MHz, DMSO-d6): δppm 2.27 (s, 3H, –CH3), 5.42 (s, 1H, –CH), 7.09 (s, 2H, –NH2), 7.35 (t, 2H, J = 8.0 Hz, ArH), 7.47 (t, 2H, J = 6.8 Hz, ArH), 7.53 (t, 2H, J = 8.0 Hz, ArH), 7.75 (d, 1H, J = 8.0 Hz, ArH), 7.86 (d, 3H, J = 7.6 Hz, ArH), 8.01 (d, 1H, J = 7.2 Hz, ArH), 8.23 (d, 1H, J = 7.6 Hz, ArH); 13C NMR (100 MHz, DMSO-d6): δppm 19.0, 31.7, 56.5, 103.8, 119.9, 120.4, 123.6, 124.1, 125.4, 125.8, 126.0, 126.2, 126.6, 126.7, 128.9, 129.2, 129.3, 129.9, 131.3, 134.1, 138.1, 140.2, 145.7.
6-Amino-3-methyl-1-phenyl-4-(1-phenylprop-1-en-2-yl)-1,4-dihydropyrano[2,3-c]pyrazole-5-carbonitrile (Table 4, entry 4). Yellow solid; mp: 267–269 °C; yield: 89%; IR (KBr): ν 3545, 3470, 3414, 3333, 3061, 2925, 2204, 1599, 1499, 1383 cm−1; 1H NMR (400 MHz, DMSO-d6): δppm 1.87 (s, 3H, –CH3), 2.10 (s, 3H, –CH3), 6.41 (s, 1H, –CH), 6.84 (s, 1H, –CH), 7.06 (d, 2H, J = 7.2 Hz, ArH), 7.32 (t, 6H, J = 7.6 Hz, ArH), 7.96 (d, 2H, J = 7.2 Hz, ArH), 8.04 (s, 2H, –NH2); 13C NMR (100 MHz, DMSO-d6): δppm 21.1, 37.4, 69.4, 101.2, 111.7, 116.2, 122.9, 123.1, 125.3, 125.5, 125.6, 125.7, 126.1, 126.4, 126.6, 127.0, 128.1, 129.4, 131.1, 134.0, 138.4, 139.4, 145.9, 152.2.
6-Amino-4-(2-hydroxy-3-methoxyphenyl)-3-methyl-1-phenyl-1,4-dihydropyrano[2,3-c]pyrazole-5-carbonitrile (Table 4, entry 5). Pink solid; mp: 297–299 °C; yield: 90%; IR (KBr): ν 3462, 3420, 3333, 3231, 3062, 2945, 2201, 1640, 1599, 1497, 1361 cm−1; 1H NMR (400 MHz, DMSO-d6): δppm 1.86 (s, 3H, –CH3), 2.09 (s, 3H, –CH3), 3.82 (s, 1H, –OH), 3.98 (s, 1H, –CH), 6.88 (d, 4H, J = 8.0 Hz, ArH), 7.30 (t, 2H, J = 7.8 Hz, ArH), 7.51 (s, 2H, –NH2), 7.91 (t, 2H, J = 6.8 Hz, ArH); 13C NMR (100 MHz, DMSO-d6): δppm 21.1, 32.6, 37.2, 60.3, 100.4, 100.5, 101.4, 111.4, 116.0, 116.1, 126.1, 126.2, 126.7, 126.9, 127.5, 128.9, 129.3, 129.5, 137.3, 137.6, 140.8, 140.9, 145.0.
6-Amino-4-(3-ethoxy-4-hydroxyphenyl)-3-methyl-1-phenyl-1,4-dihydropyrano[2,3-c]pyrazole-5-carbonitrile (Table 4, entry 6). Orange solid; mp: 175–177 °C; yield: 89%; IR (KBr): ν 3420, 3331, 3229, 3195, 2984, 2186, 1647, 1597, 1516, 1494, 1395 cm−1; 1H NMR (400 MHz, DMSO-d6): δppm 1.32 (t, 3H, J = 7.0 Hz, –CH3), 1.84 (s, 3H, –CH3), 4.02 (q, 2H, J = 6.8 Hz, –CH3), 4.58 (s, 1H, –OH), 6.44 (d, 1H, J = 8.0 Hz, ArH), 6.78 (d, 1H, J = 8.0 Hz, ArH), 6.82 (s, 1H, –CH), 7.07 (t, 1H, J = 7.4 Hz, ArH), 7.17 (s, 1H, ArH), 7.30 (t, 1H, J = 7.2 Hz, ArH), 7.50 (t, 1H, J = 8.0 Hz, ArH), 7.82 (d, 1H, J = 8.0 Hz, ArH), 7.89 (d, 1H, J = 7.6 Hz, ArH), 8.08 (s, 2H, –NH2); 13C NMR (100 MHz, DMSO-d6): δppm 27.0, 34.5, 36.8, 59.1, 64.3, 119.4, 120.1, 120.3, 120.7, 120.8, 124.1, 126.5, 128.9, 129.4, 129.8, 135.0, 138.1, 144.2, 145.0, 145.9, 146.3, 146.4.
6-Amino-3-methyl-4-(3-nitrophenyl)-1-phenyl-1,4-dihydropyrano[2,3-c]pyrazole-5-carbonitrile (Table 4, entry 7). Yellow solid; mp: 207–209 °C; yield: 93%; IR (KBr): ν 3462, 3411, 3328, 3249, 3195, 3057, 2920, 2195, 1661, 1597, 1519, 1385 cm−1; 1H NMR (400 MHz, DMSO-d6): δppm 1.82 (s, 3H, –CH3), 4.80 (s, 1H, –CH), 7.35 (d, 2H, J = 7.2 Hz, ArH), 7.42 (s, 1H, ArH), 7.52 (t, 2H, J = 8.0 Hz, ArH), 7.69, 7.30 (t, 2H, J = 7.6 Hz, ArH), 7.83 (d, 2H, J = 7.6 Hz, ArH), 8.18 (s, 2H, –NH2); 13C NMR (100 MHz, DMSO-d6): δppm 13.4, 26.6, 36.7, 57.5, 119.2, 119.8, 120.3, 120.6, 120.8, 122.3, 122.7, 122.8, 123.2, 123.9, 126.8, 128.9, 129.4, 129.7, 129.8, 130.8, 134.9.
6-Amino-3-methyl-4-(naphthalen-2-yl)-1-phenyl-1,4-dihydropyrano[2,3-c]pyrazole-5-carbonitrile (Table 4, entry 9). Yellow solid; mp: 206–209 °C; yield: 93%; IR (KBr): ν 3554, 3441, 3351, 3301, 3191, 3095, 2925, 2191, 1653, 1593, 1518, 1398 cm−1; 1H NMR (400 MHz, DMSO-d6): δppm 1.77 (s, 3H, –CH3), 4.89 (s, 1H, –CH), 7.33 (d, 4H, J = 8.0 Hz, ArH), 7.53 (t, 5H, J = 8.4 Hz, ArH), 7.86 (d, 2H, J = 8.0 Hz, ArH), 7.90 (s, 1H, ArH), 7.92 (s, 2H, –NH2); 13C NMR (100 MHz, DMSO-d6): δppm 35.2, 37.5, 58.5, 124.9, 125.1, 125.2, 125.3, 125.4, 126.5, 126.6, 126.7, 126.8, 127.7, 1238.0, 128.1, 131.1, 131.2, 131.3, 133.4, 133.8, 134.0, 139.8, 142.9, 143.8.
6-Amino-4-(3,4-dihydroxyphenyl)-3-methyl-1-phenyl-1,4-dihydropyrano[2,3-c]pyrazole-5-carbonitrile (Table 4, entry 10). Orange solid; mp: 189–191 °C; yield: 88%; IR (KBr): ν 3413, 3329, 3237, 2186, 1655, 1598, 1496, 1384 cm−1; 1H NMR (400 MHz, DMSO-d6): δppm 1.90 (s, 3H, –CH3), 4.14 (s, 1H, –OH), 4.49 (s, 1H, –OH), 6.58 (d, 1H, J = 8.4 Hz, ArH), 6.71 (d, 1H, J = 8.0 Hz, ArH), 6.83 (s, 1H, –CH), 6.86 (s, 1H, ArH), 7.10 (t, 1H, J = 6.8 Hz, ArH), 7.16 (s, 2H, –NH2), 7.33 (t, 1H, J = 7.6 Hz, ArH), 7.51 (t, 1H, J = 8.0 Hz, ArH), 7.81 (d, 1H, J = 7.6 Hz, ArH), 7.90 (d, 1H, J = 7.6 Hz, ArH); 13C NMR (100 MHz, DMSO-d6): δppm 33.8, 36.6, 59.4, 99.6, 115.3, 115.5, 118.4, 119.1, 120.1, 120.3, 124.2, 126.6, 128.9, 129.9, 138.1, 145.9, 146.6, 157.7, 159.6.
6-Amino-3-methyl-1-phenyl-4-(pyridin-4-yl)-1,4-dihydropyrano[2,3-c]pyrazole-5-carbonitrile (Table 4, entry 12). Red solid; mp: 249–251 °C; yield: 88%; IR (KBr): ν 3406, 3346, 3152, 3066, 2919, 2195, 1667, 1595, 1520, 1395 cm−1; 1H NMR (400 MHz, DMSO-d6): δppm 1.82 (s, 3H, –CH3), 4.78 (s, 1H, –CH), 7.36 (d, 1H, J = 6.6 Hz, ArH), 7.41 (s, 2H, –NH2), 7.51 (t, 3H, J = 8.0 Hz, ArH), 7.65 (d, 1H, J = 8.8 Hz, ArH), 7.81 (d, 2H, J = 7.6 Hz, ArH), 8.57 (d, 2H, J = 7.0 Hz, ArH); 13C NMR (100 MHz, DMSO-d6): δppm 30.6, 41.3, 57.9, 123.4, 124.2, 125.0, 125.4, 128.2, 128.3, 128.4, 129.3, 129.8, 130.0, 131.6, 133.6, 134.1, 134.6, 142.6, 145.4.
Computational detail
Computations were performed using the Gaussian 09 program.42 Density functional theory has been used to investigate the reactions of 5-amino-pyrazole-4-carbonitrile derivatives (5) in the presence of {[HMIM]C(NO2)3} as a nanostructure ionic liquid catalyst. All geometry optimizations were performed at B3LYP/TZVP level of theory and frequency calculations were carried out at the same level. All frequencies in the non-transition states are real. We have used the corrected electronic energy and Gibbs free energy for investigation of the mechanism of the synthesis of 5-amino-pyrazole-4-carbonitrile derivatives (5). The nature of transition structure was confirmed by intrinsic reaction coordinate (IRC)43 searches and vibrational frequency calculations. The intramolecular interactions were calculated on the basis of natural bond orbital (NBO)44 analyses.
Acknowledgements
We thank Bu-Ali Sina University and Iran National Science Foundation (INSF) for financial support to our research group.
Notes and references
- P. Wasserscheid and W. Keim, Angew. Chem., Int. Ed., 2000, 39, 3773 CrossRef.
- K. R. Seddon, in Molten Salt Chemistry, ed. G. Mamantov and R. Marassi, Reidel Publishing Co., Dordrecht, The Netherlands, 1987 Search PubMed.
- J. D. Holbrey and K. R. Seddon, Clean Products and Processes, 1999, 1, 223 Search PubMed.
- T. Welton, Chem. Rev., 1999, 99, 2071 CrossRef CAS PubMed.
- J. Dupont, C. S. Consorti and J. J. Spencer, J. Braz. Chem. Soc., 2000, 11, 337 CAS.
- C. C. Tzschucke, C. Markert, W. Bannwarth, S. Roller, A. Hebel and R. Haag, Angew. Chem., Int. Ed., 2000, 41, 3964 CrossRef.
- J. S. Wilkes, J. Mol. Catal. A: Chem., 2014, 214, 11 CrossRef PubMed.
- M. Kosmulski, J. Gustafsson and J. B. Rosenholm, Thermochim. Acta, 2004, 412, 47 CrossRef CAS PubMed.
- M. Deetlefs and K. R. Seddon, Chim. Oggi, 2006, 24, 16 CAS.
- M. J. Earle, J. M. S. S. Esperanca, M. A. Gilea, J. N. Canongia-Lopes, L. P. N. Rebelo, J. W. Magee, K. R. Seddon and J. A. Widegren, Nature, 2006, 439, 831 CrossRef CAS PubMed.
- B. M. Trost, Angew. Chem., Int. Ed. Engl., 1995, 34, 259 CrossRef CAS PubMed.
- P. A. Wender, S. T. Hanhdy and D. L. Wright, Chem. Ind., 1997, 765 CAS.
- L. Weber, Curr. Opin. Chem. Biol., 2000, 4, 295 CrossRef CAS.
- A. Dömling, Curr. Opin. Chem. Biol., 2002, 6, 306 CrossRef.
- J. Kim, D. Lee, C. Park, W. So, M. Jo, T. Ok, J. Kwon, S. Kong, S. Jo, Y. Kim, J. Choi, H. C. Kim, Y. Ko, I. Choi, Y. Park, J. Yoon, M. K. Ju, J. Kim, S.-J. Han, T.-H. Kim, J. Cechetto, J. Nam, P. Sommer, M. Liuzzi, J. Lee and Z. No, ACS Med. Chem. Lett., 2012, 3, 678 CrossRef CAS PubMed.
- J. Bo, F. Wei, S. Mu-Yan, Y. Qin, W. Shu-Liang, T. Shu-Jiang and L. Guigen, J. Org. Chem., 2014, 79, 5258 CrossRef PubMed.
- R. Nagamallu and A. K. Kariyappa, Bioorg. Med. Chem. Lett., 2013, 23, 6406 CrossRef PubMed.
- I. M. El-Deeb and S. H. Lee, Bioorg. Med. Chem., 2010, 18, 3961 CrossRef CAS PubMed.
- S. Prekupec, D. Makuc, J. Plavec, L. Suman, M. Kralj, K. Pavelic, J. Balzarini, E. D. Clercq, M. Mintas and S. Raic-Malic, J. Med. Chem., 2007, 50, 3037 CrossRef CAS PubMed.
- N. K. Piyush, P. S. Shailesh and K. R. Dipak, New J. Chem., 2014, 38, 2902 RSC.
- D. M. Bailey, P. E. Hansen, A. G. Hlavac, E. R. Baizman, J. Pearl, A. F. Defelice and M. E. Feigenson, J. Med. Chem., 1985, 28, 256 CrossRef CAS.
- E. McDonald, J. Keith, A. B. Paul, M. J. Drysdale and P. Workman, Curr. Top. Med. Chem., 2006, 6, 1193 CrossRef CAS.
- A. A. Dissanayake and A. L. Odom, Chem. Commun., 2012, 48, 440 RSC.
- M. Srivastava, P. Rai, J. Singh and J. Singh, New J. Chem., 2014, 38, 302 RSC.
- K. Kumari, D. S. Raghuvanshi, V. Jouikov and K. N. Singh, Tetrahedron Lett., 2012, 53, 1130 CrossRef CAS PubMed.
- Y. Peng, G. Song and R. Dou, Green Chem., 2006, 8, 573 RSC.
- G. Vasuki and K. Kumaravel, Tetrahedron Lett., 2008, 49, 5636 CrossRef CAS PubMed.
- A. M. Shestopalov, Y. M. Emeliyanova, A. A. Shestopalov, L. A. Rodinovskaya, Z. I. Niazimbetova and D. H. Evans, Org. Lett., 2002, 4, 423 CrossRef CAS PubMed.
- A. Saha, S. Payra and S. Banerjee, Green Chem., 2015, 17, 2859 RSC.
- S. Aixue, Y. Jia-Hai, Y. Haitao, Z. Wenchao and W. Xiaolong, Tetrahedron Lett., 2014, 55, 889 CrossRef PubMed.
- S. Maddila, S. Rana, R. Pagadala, S. Kankala, S. Maddila and S. B. Jonnalagadda, Catal. Commun., 2015, 61, 26 CrossRef CAS PubMed.
- M. Srivastava, P. Rai, J. Singh and J. Singh, RSC Adv., 2013, 3, 16994 RSC.
- D. Shi, J. Mou, Q. Zhuang, L. Niu, N. Wu and X. Wang, Synth. Commun., 2004, 34, 4557 CrossRef CAS PubMed.
- M. A. Zolfigol, S. Baghery, A. R. Moosavi-Zare, S. M. Vahdat, H. Alinezhad and M. Norouzi, RSC Adv., 2014, 4, 57662 RSC.
-
(a) M. A. Zolfigol, D. Azarifar, S. Mallakpour, I. Mohammadpoor-Baltork, A. Forghaniha, B. Maleki and M. Abdollahi-Alibeik, Tetrahedron Lett., 2006, 47, 833 CrossRef CAS PubMed;
(b) D. Azarifar, M. A. Zolfigol and B. Maleki, Bull. Korean Chem. Soc., 2004, 25, 1 Search PubMed;
(c) D. Azarifar, M. A. Zolfigol and B. Maleki, Synthesis, 2004, 1744 CrossRef CAS;
(d) M. A. Zolfigol, D. Azarifar and B. Maleki, Tetrahedron Lett., 2004, 45, 2181 CrossRef CAS PubMed.
-
(a) A. R. Moosavi-Zare, M. A. Zolfigol, E. Noroozizadeh, M. Tavasoli, V. Khakyzadeh and A. Zare, New J. Chem., 2013, 37, 4089 RSC;
(b) M. A. Zolfigol, M. Tavasoli, A. R. Moosavi-Zare, P. Moosavi, H. G. Kruger, M. Shiri and V. Khakyzadeh, RSC Adv., 2013, 3, 25681 RSC.
-
(a) M. A. Zolfigol, A. Khazaeia, A. R. Moosavi-Zare and A. Zare, Org. Prep. Proced. Int., 2010, 42, 95 CrossRef CAS PubMed;
(b) A. Khazaei, M. A. Zolfigol, A. R. Moosavi-Zare and A. Zare, Sci. Iran., Trans. C, 2010, 17, 31 CAS;
(c) M. A. Zolfigol, A. Khazaei, A. R. Moosavi-Zare, A. Zare and V. Khakyzadeh, Appl. Catal., A, 2011, 400, 1 CrossRef PubMed;
(d) M. A. Zolfigol, V. Khakyzadeh, A. R. Moosavi-Zare, A. Zare, S. B. Azimi, Z. Asgari and A. Hasaninejad, C. R. Chim., 2012, 15, 719 CrossRef CAS PubMed;
(e) M. A. Zolfigol, A. Khazaei, A. R. Moosavi-Zare, A. Zare, H. G. Kruger, Z. Asgari, V. Khakyzadeh and M. Kazem-Rostami, J. Org. Chem., 2012, 77, 3640 CrossRef CAS PubMed;
(f) A. Zare, A. R. Moosavi-Zare, M. Merajoddin, M. A. Zolfigol, T. Hekmat-Zadeh, A. Hasaninejad, A. Khazaei, M. Mokhlesi, V. Khakyzadeh, F. Derakhshan-Panah, M. H. Beyzavi, E. Rostami, A. Arghoon and R. Roohandeh, J. Mol. Liq., 2012, 167, 69 CrossRef CAS PubMed;
(g) M. A. Zolfigol, A. Khazaei, A. R. Moosavi-Zare, A. Zare, Z. Asgari, V. Khakyzadeh and A. Hasaninejad, J. Ind. Eng. Chem., 2013, 19, 721 CrossRef CAS PubMed;
(h) A. R. Moosavi-Zare, M. A. Zolfigol, M. Zarei, A. Zare and V. Khakyzadeh, J. Mol. Liq., 2013, 186, 63 CrossRef CAS PubMed;
(i) A. R. Moosavi-Zare, M. A. Zolfigol, M. Zarei, A. Zare, V. Khakyzadeh and A. Hasaninejad, Appl. Catal., A, 2013, 467, 61 CrossRef CAS PubMed;
(j) M. A. Zolfigol, H. Vahedi, S. Azimi and A. R. Moosavi-Zare, Synlett, 2013, 24, 1113 CrossRef CAS;
(k) A. R. Moosavi-Zare, M. A. Zolfigol, O. Khaledian, V. Khakyzadeh, M. D. Farahani and H. G. Kruger, New J. Chem., 2014, 38, 2342 RSC;
(l) A. R. Moosavi-Zare, M. A. Zolfigol and M. Daraei, Synlett, 2014, 25, 1173 CrossRef;
(m) M. A. Zolfigol, A. R. Moosavi-Zare and M. Zarei, C. R. Chim., 2014, 17, 1264 CrossRef CAS PubMed;
(n) A. R. Moosavi-Zare, M. A. Zolfigol, V. Khakyzadeh, C. Böttcher, M. H. Beyzavi, A. Zare, A. Hasaninejad and R. Luque, J. Mater. Chem. A, 2014, 2, 770 RSC;
(o) M. A. Zolfigol, S. Baghery, A. R. Moosavi-Zare and S. M. Vahdat, RSC Adv., 2015, 5, 32933 RSC;
(p) M. A. Zolfigol, S. Baghery, A. R. Moosavi-Zare, S. M. Vahdat, H. Alinezhad and M. Norouzi, RSC Adv., 2015, 5, 45027 RSC.
- M. A. Zolfigol, H. Gholami and V. Khakyzadeh, Principles of organic synthesis with a new approach, Bu-Ali Sina University Publishers, Hamedan, Iran, 3rd edn, 2014, p. 26 Search PubMed.
-
(a) J. M. Erhardt and J. D. Wuest, J. Am. Chem. Soc., 1980, 102, 6363 CrossRef CAS;
(b) T. J. Atkins, J. Am. Chem. Soc., 1980, 102, 6364 CrossRef CAS;
(c) J. M. Erhardt, E. R. Grover and J. D. Wuest, J. Am. Chem. Soc., 1980, 102, 6365 CrossRef CAS.
- H. Sheibani and M. Babaei, Synth. Commun., 2010, 40, 257 CrossRef CAS PubMed.
- H. Mecadon, M. Rumum-Rohman, M. Rajbangshi and B. Myrboh, Tetrahedron Lett., 2011, 52, 2523 CrossRef CAS PubMed.
- M. J. Frisch, Gaussian 09, revision A.02, Gaussian, Inc., Wallingford, CT, 2009 Search PubMed.
-
(a) K. J. Fukui, J. Phys. Chem., 1970, 74, 4161 CrossRef CAS;
(b) K. Fukui, Acc. Chem. Res., 1981, 14, 363 CrossRef CAS.
- E. D. Glendening, A. E. Reed, J. A. Carpenter and F. Weinhold, NBO Version 3.1 Search PubMed.
Footnote |
† Electronic supplementary information (ESI) available. See DOI: 10.1039/c5ra16289k |
|
This journal is © The Royal Society of Chemistry 2015 |