DOI:
10.1039/D2QM00833E
(Research Article)
Mater. Chem. Front., 2022,
6, 3442-3449
Thermally activated delayed fluorescence dendrimers achieving 20% external quantum efficiency for solution-processed OLEDs†
Received
17th August 2022
, Accepted 27th September 2022
First published on 28th September 2022
Abstract
Two multi-carbazole-encapsulated TADF dendrimers were obtained by coupling tercarbazole (tBuTCz or MeOTCz) and di(pyridin-3-yl)methanone (DPyM) units. Both tBuTCz-DPyM and MeOTCz-DPyM show small singlet–triplet energy gap (ΔEST) values (0.01 vs. 0.02 eV) and high photoluminescence quantum yield (PLQY) values (66.2 vs. 55.0%). High-performance solution-processed OLEDs based on tBuTCz-DPyM and MeOTCz-DPyM as the emissive layer were fabricated. The tBuTCz-DPyM-based solution-processed device exhibited a maximum current efficiency (CEmax) of 52.6 cd A−1, a maximum external quantum efficiency (EQEmax) of 20.4% and a maximum luminance (Lmax) of 6165 cd m−2 with a low turn-on voltage of 3.2 V. The MeOTCz-DPyM-based solution-processed device also showed an efficient performance with a CEmax of 27.0 cd A−1, an EQEmax of 9.2%, an Lmax of 8169 cd m−2 and a low turn-on voltage of 3.4 V. The tBuTCz-DPyM-based device (I) and the MeOTCz-DPyM-based device (II) show Commission internationale de l'éclairage (CIE) coordinates of (0.25, 0.48) and (0.37, 0.54), respectively. Both tBuTCz-DPyM and MeOTCz-DPyM demonstrate a high performance among solution-processed OLEDs with TADF dendrimers.
1. Introduction
Due to their wide viewing angle, high response speed, high brightness and lightweight properties, organic light-emitting diodes (OLEDs) had been developed as commercial technologies for next-generation displays and lighting.1–5 Generally, the fabrication of OLEDs can be divided into vacuum-deposited and solution-processed devices. The construction of multiple organic functional layers for vacuum-deposited OLEDs has been proved to be efficient.6–8 However, due to the limited deposited space, low material utilization and high manufacturing costs, the development of vacuum-deposited OLEDs has been restricted. Therefore, it is more important to develop solution-processed OLEDs. At this stage, great progress has been made with solution-processed OLEDs.9–16 Nonetheless, the performance of solution-processed OLEDs still needs to be greatly improved, and comparison with that of vacuum-deposited OLEDs is difficult. In recent years, as a third-generation light-emitting material in OLEDs, based on their advantages of being heavy metal-free, low cost and achieving a 100% internal quantum efficiency (IQE) through efficient reverse intersystem crossing (RISC) procedures, thermally activated delayed fluorescence (TADF) emitters have received great attention.17–22 Thus, it is particularly important to develop high-performance solution-processed TADF materials.
Small-molecule TADF emitters have the disadvantages of being low-molecular-weight compounds, with poor solution-processability and a high crystallization tendency, which are not suitable for solution-processed OLEDs.23,24 In recent years, polymers and dendritic TADF molecules have been widely developed as solution-processed OLED materials.25–30 In particular, due to their definite structural composition, good film-forming properties and high purity, dendrimers are a type of ideal solution-processing OLED material.31–34 Because multi-carbazole-encapsulated molecules have good solubility, a good hole-transport capacity, amorphous film-forming properties and good thermal stability, these are suit for solution-processed OLEDs. Therefore, a suitable design strategy for multi-carbazole-encapsulated molecules for the construction of TADF dendrimers could be beneficial for solution-processed OLEDs.35–39 In this paper, we report two TADF dendrimers with multi-carbazole encapsulation, named tBuTCz-DPyM and MeOTCz-DPyM, which comprise di(pyridin-3-yl)methanone (DPyM) as the acceptor core coupled with 3,3′′,6,6′′-tetra-tert-butyl-9′H-9,3′:6′,9′′-tercarbazole (tBuTCz) or 3,3′′,6,6′′-tetramethoxy-9′H-9,3′:6′,9′′-tercarbazole (MeOTCz) as the donor units. The solution-processed devices based on tBuTCz-DPyM and MeOTCz-DPyM as the doped emissive layer show a high performance with maximum current efficiency (CEmax) values of 52.6 and 27.0 cd A−1, maximum external quantum efficiency (EQEmax) values of 20.4 and 9.2%, maximum luminance (Lmax) values of 6165 and 8169 cd m−2 at 8 V, and low turn-on voltages of 3.2 and 3.4 V, respectively. In addition, the full-width at half-maximum (FWHM) of the EL spectrum for tBuTCz-DPyM is narrow, at 84 nm.
2. Results and discussion
2.1. Synthesis and structural characterization
As shown in Scheme S1 (ESI†), the target compounds for tBuTCz-DPyM and MeOTCz-DPyM were prepared via the Ullmann C–N coupling reaction of DPyM with tBuTCz or MeOTCz, respectively.40,41 The chemical structures of the two dendrimers were confirmed using 1H NMR and 13C NMR and high-resolution MS.
2.2. Thermal properties and film-forming properties
The two materials showed good thermal stability, which was indicated by their high decomposition temperature (Td, corresponding to 5% weight loss) values of 443 and 446 °C for tBuTCz-DPyM and MeOTCz-DPyM, respectively, and the glass transition temperature (Tg) for tBuTCz-DPyM and MeOTCz-DPyM reached 260 and 268 °C, respectively (Fig. S1, ESI†). Moreover, in order to evaluate the morphological stability of the target compounds, atomic force microscopy (AFM) was utilized to explore the surface images of their neat films. As shown in Fig. S2 (ESI†), the surfaces of the two films were smooth and pinhole-free, and the root-mean-square (RMS) roughness was 0.44 nm and 0.46 nm for tBuTCz-DPyM and MeOTCz-DPyM, respectively. Therefore, the two emitters can provide uniform amorphous films for solution-processed devices, and the introduction of the tert-butyl group into the molecule provides better film-forming properties than for the methoxy-containing emitter.
2.3. Electrochemical and computational properties
The electrochemical behavior of the dendrimers was investigated using cyclic voltammetry (CV). As shown in Fig. S3 (ESI†), both of the compounds displayed one oxidation wave. According to the onset potentials, the highest occupied molecular orbital (HOMO) energy levels of tBuTCz-DPyM and MeOTCz-DPyM were estimated to be −5.47 and −5.12 eV, respectively. According to the optical bandgaps, the lowest unoccupied molecular orbital (LUMO) energy levels of tBuTCz-DPyM and MeOTCz-DPyM were calculated to be −2.70 eV and −2.52 eV, respectively. To gain insight into the electronic nature and geometry of the emitters, density functional theory (DFT) calculations were performed at the B3LYP/6-31G* level. As shown in Fig. 1, the HOMO and LUMO of the two compounds were located on the electron-donating multi-carbazole unit and the electron-withdrawing dipyridyl ketone unit, respectively. It was shown that the two molecules have a small HOMO–LUMO overlap, which indicates strong charge-transfer (CT) character and a small electron-exchange energy.42,43 The calculated HOMO levels of tBuTCz-DPyM and MeOTCz-DPyM were −5.17 and −4.88 eV, while the calculated LUMO levels were −2.41 and −2.40 eV, respectively. Due to the stronger electron-donating ability of the methoxy group over the tert-butyl group, the HOMO level of MeOTCz-DPyM is shallower than that of tBuTCz-DPyM.16 This matches the experimental values obtained from the CV curves.
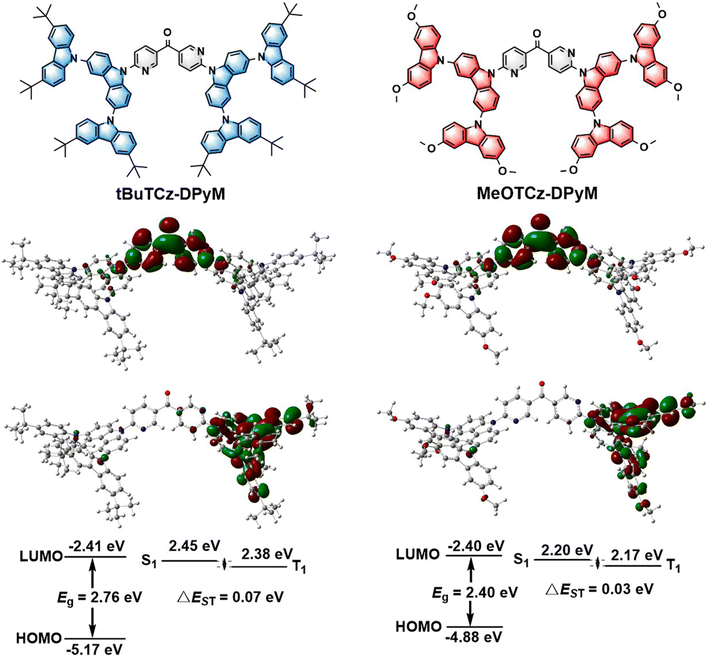 |
| Fig. 1 Chemical structures (top), B3LYP/6-31G*-optimized molecular orbitals for the HOMO (lower middle) and LUMO (upper middle), and the energy levels (bottom) of the tBuTCz-DPyM (left) and MeOTCz-DPyM (right) emitters. | |
2.4. Photophysical properties
The two materials displayed similar absorption spectra. The weak absorption bands in the range of 320–450 nm assigned to the CT transitions from the tBuTCz or MeOTCz as donor units to DPyM as the acceptor unit. The tBuTCz-DPyM and MeOTCz-DPyM materials showed emission peaks at 521 and 554 nm in solution, respectively. Fig. 2b shows the fluorescence and phosphorescence spectra of the two compounds in their film states. According to the onsets of the fluorescence and phosphorescence spectra, ΔEST was calculated to be 0.01 eV for tBuTCz-DPyM, and 0.02 eV for MeOTCz-DPyM. The small ΔEST values indicated that an up-conversion process of the triplet excitons into their singlet state could occur efficiently through RISC, which results in the TADF phenomenon.42,43 The corresponding data are summarized in Table 1.
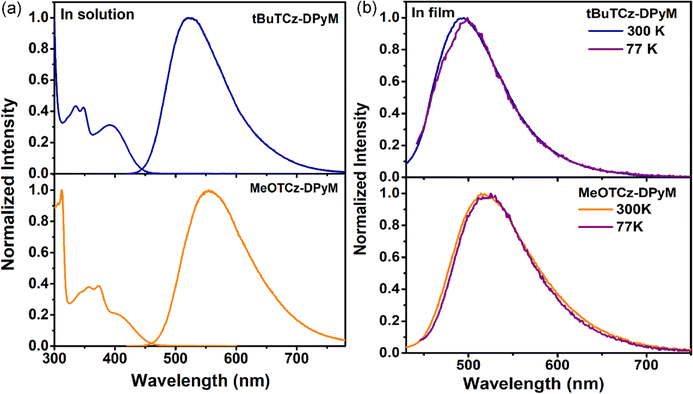 |
| Fig. 2 UV-Vis absorption and PL spectra of tBuTCz-DPyM and MeOTCz-DPyM in (a) dilute toluene solution, and (b) the film state (8 wt% doped in mCBP). Fluorescence spectra at room temperature (300 K) and phosphorescence spectra at 77 K for tBuTCz-DPyM and MeOTCz-DPyM in doped films (8 wt% doped in mCBP). | |
Table 1 Photophysical, electrochemical and thermal stability data of tBuTCz-DPyM and MeOTCz-DPyM
Compound |
λ
abs
(nm) |
λ
PL
/λPLb (nm) |
Φ
PL
(%) |
HOMOc/HOMOd (eV) |
LUMOe/LUMOd (eV) |
ΔESTf/ΔESTd (eV) |
k
RISC
(s−1) |
τ
p/τdh [ns]/[μs] |
T
d/Tgi (°C) |
Measured in toluene solution.
Measured in the film state.
HOMO energy levels deduced from the equation HOMO = −(4.8 + Eoxons).
Obtained from quantum calculations using B3LYP/6-31G*.
LUMO energy levels obtained from the equation LUMO = HOMO + Egopt.
Estimated from the onset of fluorescence spectrum at 300 K and phosphorescence spectrum at 77 K.
k
RISC: the rate constant for RISC from the triplet excited state to the singlet excited state.
Measured in thin films on a quartz substrate at 300 K.
Measured via TGA/DSC at a heating rate of 10 °C min−1.
|
tBuTCz-DPyM
|
392 |
521/495 |
63.5 |
−5.47/−5.17 |
−2.70/−2.41 |
0.01/0.07 |
1.8 × 105 |
7.0/9.5 |
443/260 |
MeOTCz-DPyM
|
405 |
554/514 |
55.0 |
−5.12/−4.88 |
−2.52/−2.40 |
0.02/0.03 |
2.4 × 105 |
15.7/7.9 |
446/268 |
Moreover, the two dendrimers also showed small calculated ΔEST values of 0.07 and 0.03 eV for tBuTCz-DPyM and MeOTCz-DPyM, respectively. The dendrimers of tBuTCz-DPyM and MeOTCz-DPyM have a high PLQY in the films of 63.5 and 55.0%, respectively, which indicated that, compared with MeOTCz-DPyM with methoxy groups, tBuTCz-DPyM with tert-butyl groups can better reduce the concentration quenching caused by molecular aggregation. As shown in Fig. 3, transient PL measurements were carried out to prove the TADF features of the two dendrimers in the film state at room temperature. Both target compounds showed a multi-exponential decay with a short lifetime of 7.0 ns and a long lifetime of 9.5 μs for tBuTCz-DPyM, and a short lifetime of 15.7 ns and a long lifetime of 7.9 μs for MeOTCz-DPyM, which is attributed to TADF.36 In order to gain a deeper insight into the RISC process, the rate constants of the two materials were calculated using reported methods.44 As shown in Table S1 (ESI†), the rate constant for radiative decay from the S1 state (kr, S) of tBuTCz-DPyM and MeOTCz-DPyM was calculated to be 5.0 × 107 and 1.9 × 107 s−1, respectively. This is equivalent to the value of conventional fluorescent materials. Moreover, the rate constant of non-radiative decay from the S1 state (knr,S) of tBuTCz-DPyM and MeOTCz-DPyM was estimated to be 2.9 × 107 and 1.6 × 107 s−1, respectively. The ratio of kr, S to knr,S is 1.7 for tBuTCz-DPyM and 1.2 for MeOTCz-DPyM. Therefore, the ratio of kr, S to knr,S for tBuTCz-DPyM is slightly higher than that of MeOTCz-DPyM, which leads to a slightly higher PLQY value for tBuTCz-DPyM compared with MeOTCz-DPyM.45 Furthermore, the kRISC values of the two emitters were relatively fast, with the corresponding values of 1.8 × 105 s−1 for tBuTCz-DPyM and 2.4 × 105 s−1 for MeOTCz-DPyM, which exhibited an effective RISC process, leading to small ΔEST values.
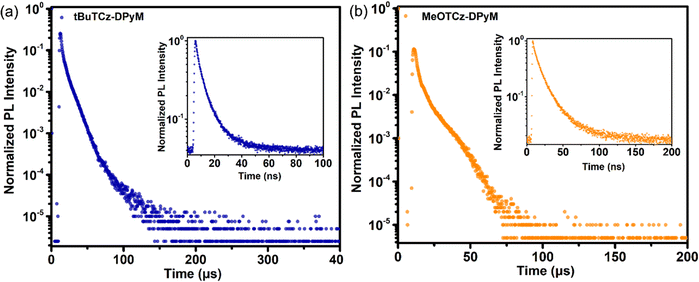 |
| Fig. 3 Transient decay curves of tBuTCz-DPyM (a) and MeOTCz-DPyM (b) in doped films (8 wt% doped in mCBP) at 300 K. Inset: prompt fluorescence. | |
2.5. Electroluminescent properties
As shown in Fig. 4, the relative energy-level alignments, the electroluminescence (EL) spectra, current density–voltage–luminance (J–V–L) characteristics, and the external quantum efficiency–current density curves of the optimized devices are displayed, and the corresponding parameters are summarized in Table 2. Both of the solution-processed devices were fabricated with the following structures: ITO/PEDOT:PSS (40 nm)/PVK (5 nm)/mCBP: emitter (8 wt%, 60 nm)/TPBI (40 nm)/Yb (5 nm)/Ag (120 nm). PEDOT:PSS is poly(3,4-ethylene-dioxythiophene):poly(styrenesulfonic acid), which act as the hole-injection layer; PVK is poly(N-vinylcarbazole), which acts as the hole-transporting layer; and TPBI is 1,3,5-tris(N-phenyl-benzimiazole-2-yl)benzene, which serves as the electron-transporting layer. The EQE–current density curves show CEmax = 52.6 cd A−1, and EQEmax = 20.4% for the tBuTCz-DPyM-based device (device I), and CEmax = 27.0 cd A−1, and EQEmax = 9.2% for the MeOTCz-DPyM-based device (device II). In addition, the full-width at half-maximum (FWHM) of the EL spectrum of device I is only 84 nm, narrower than that of device II (115 nm), and the EL spectrum for devices I and II exhibits, respectively, bluish-green and green emission with a maximum at 503 and 530 nm and Commission internationale de l'éclairage (CIE) coordinates of (0.25, 0.48) and (0.37, 0.54). Both emitters have small ΔEST and high PLQY values, which can not only ensure an effective RISC process but also provide high-efficiency emission. Hence, both materials could be used to obtain high-performance devices.37 Moreover, because the tert-butyl group has good solubility and electrochemical stability, the tBuTCz-DPyM emitter, which contains tert-butyl groups, is more suitable for solution-processed OLEDs than MeOTCz-DPyM, which will lead to a better performance.38,39
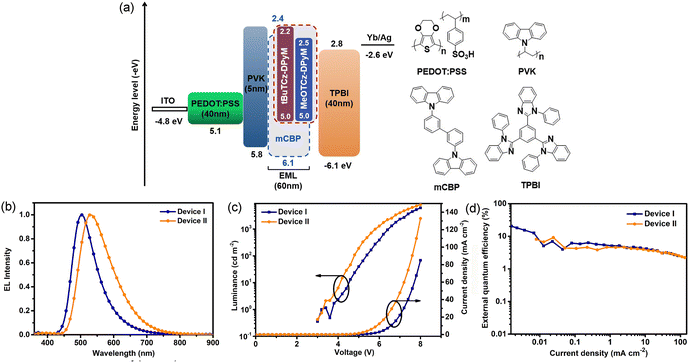 |
| Fig. 4 (a) Relative energy-level alignment of the devices (left) and chemical structures of the materials (right); (b) EL spectra of the devices; (c) current density–voltage–luminance (J–V–L) characteristics of the devices; and (d) external quantum efficiency–current density characteristics of the devices. | |
Table 2 EL characteristics of devices I and II
Device |
λ
ELmax
(nm) |
FWHM (nm) |
V
on
(V) |
L
max
(cd m−2) |
CEmaxd (cd A−1) |
EQEmaxe (%) |
CIEf (x, y) |
EL emission peak.
Turn-on voltage recorded at 1 cd m−2.
Maximum luminance at 8 V.
The maximum current efficiency.
The maximum external quantum efficiency.
Commission internationale de l'éclairage coordinates.
|
I |
503 |
84 |
3.2 |
6165 |
52.6 |
20.4 |
0.25, 0.48 |
II |
530 |
115 |
3.4 |
8169 |
27.0 |
9.2 |
0.37, 0.54 |
3. Conclusions
In conclusion, we have developed two new TADF dendrimers based on tercarbazole as the donor and di(pyridin-3-yl)methanone as the accepter. Both tBuTCz-DPyM and MeOTCz-DPyM have excellent thermal stability, good film-forming properties, a small ΔEST value, and a relatively high PLQY. Two solution-processed devices based on tBuTCz-DPyM and MeOTCz-DPyM as the emitter showed relatively high values, respectively, for CEmax of 52.6 and 27.0 cd A−1, EQEmax of 20.4% and 9.2%, Lmax of 6165 and 8169 cd m−2 at 8 V, and with low a turn-on voltage of 3.2 and 3.4 V. In particular, the tBuTCz-DPyM-based solution-processed device showed a bluish-green emission with CIE coordinates of (0.25, 0.48), which possess a narrow FWHM of 84 nm. By contrast, the MeOTCz-DPyM-based solution-processed device showed a green emission with CIE coordinates of (0.37, 0.54). These are high efficiencies among solution-processed TADF dendrimer OLEDs. High-performance, solution-processable TADF dendrimers have been developed that are beneficial for the practical application of large-area manufacturing based on solution processing.
4. Experimental section
4.1. General information
Unless otherwise stated, all commercial chemicals are used directly without further purification. All solvents are analytical grade and freshly distilled before use. Anhydrous toluene was refluxed with sodium and anhydrous dichloromethane was refluxed with calcium hydride, followed by fresh distillation before use. 1H NMR and 13C NMR spectra were recorded in CDCl3 with tetramethylsilane (TMS) as the internal standard using a Bruker Avance 600 MHz spectrometer. HRMS (Q-TOF) spectra were obtained using an Impact II spectrometer (Bruker Daltonics Inc.). UV-Vis absorption spectra were collected using a Mapada UV-1800PC recording spectrophotometer. Photoluminescence (PL) spectra were recorded using a PerkinElmer LS-55 fluorescence spectrophotometer. The absolute PLQY values of the emitters in the solid state were measured using an Edinburgh FLS 980 spectrophotometer with an integrating sphere under ambient conditions. TGA was carried out using a Hitachi STA7300 instrument at a heating rate of 10 °C min−1 under a nitrogen atmosphere. DSC was carried out using a Mettler Toledo (DSC1) instrument at a heating rate of 10 °C min−1 under a nitrogen flow. CV was measured at room temperature using a CorrTest electrochemical workstation. The CV system was constructed using a platinum plate as the working electrode, a platinum wire as the auxiliary electrode and Ag/AgNO3 (0.1 mol L−1 in acetonitrile) as the quasi-reference electrode with ferrocenium/ferrocene (Fc+/Fc) as the external standard. Tetrabutylammonium perchlorate (0.1 M) was used as the supporting electrolyte.
The synthesis of tBuTCz, MeOTCz and DPyM was completed according to the reference methods.36,40,46
Synthesis of tBuTCz-DPyM.
tBuTCz (346 mg, 0.48 mmol), DPyM (80 mg, 0.23 mmol), CuI (9.2 mg, 0.05 mmol), K3PO4 (1.0 g, 4.8 mmol) and 1,2-diaminocyclohexane (8.0 mg, 0.07 mmol) were added to the system, which was then purged with argon 3 times. The dioxane (4 ml) was added to the system. The reaction was stirred at 110 °C for 12 h. After the reaction, the mixture was filtered using a filter paper. The crude product was obtained after removing the solvent, and was purified via column chromatography (silica, hexane/ethyl acetate, v/v 5
:
1) to afford the tBuTCz-DPyM compound as a yellow solid with a yield of 52%. 1H NMR (600 MHz, CDCl3) δ 9.38 (d, J = 1.8 Hz, 2H), 8.64 (dd, J = 8.4, 1.8 Hz, 2H), 8.33 (d, J = 8.4 Hz, 4H), 8.26 (s, 4H), 8.17 (s, 8H), 8.10 (d, J = 8.4 Hz, 2H), 7.74 (dd, J = 9.0, 1.8 Hz, 4H), 7.48 (d, J = 9.6 Hz, 8H), 7.38 (d, J = 9.0 Hz, 8H), 1.47 (s, 72H). 13C NMR (150 MHz, CDCl3) δ 190.65, 154.78, 151.55, 142.81, 140.12, 139.83, 138.33, 132.83, 129.69, 126.36, 125.89, 123.67, 123.26, 119.13, 117.76, 116.30, 113.36, 109.03, 34.75, 32.03, 29.71. HRMS (APCI) m/z: [M + H]+ calcd for C115H115N8O+, 1623.9188; found, 1623.9267.
Synthesis of MeOTCz-DPyM.
A procedure similar to that used for tBuTCz-DPyM was followed but using MeOTCz instead of tBuTCz to afford the compound MeOTCz-DPyM as a yellow solid with a yield of 56%. 1H NMR (600 MHz, CDCl3) δ 9.35 (d, J = 1.8 Hz, 2H), 8.61 (dd, J = 8.4, 1.8 Hz, 2H), 8.31 (d, J = 9.0 Hz, 4H), 8.26 (d, J = 1.8 Hz, 4H), 8.06 (d, J = 8.4 Hz, 2H), 7.70 (dd, J = 8.4, 1.8 Hz, 4H), 7.58 (d, J = 2.4 Hz, 8H), 7.33 (d, J = 9.0 Hz, 8H), 7.04 (dd, J = 9.0, 2.4 Hz, 8H), 3.95 (s, 24H). 13C NMR (150 MHz, CDCl3) δ 190.57, 154.67, 154.00, 151.49, 140.09, 138.29, 136.94, 132.79, 129.66, 126.37, 125.82, 123.49, 119.12, 117.66, 115.23, 113.42, 110.50, 102.99, 56.12. HRMS (APCI) m/z: [M + H]+ calcd for C91H67N8O9+, 1415.5026; found, 1415.5074.
OLED device fabrication and characterization.
ITO patterned glass substrates (size 12 mm × 8 mm) were cleaned in an ultrasonic bath using acetone, isopropyl alcohol, and DI water for 15 min, respectively. After UV-ozone treatment for 3 min, a 40-nm-thick PEDOT:PSS layer was spin coated onto the ITO substrate, which was then heated at 150 °C for 15 min. Then, a 5-nm-thick PVK interlayer was spin coated on top of the PEDOT:PSS layer under nitrogen and annealed at 110 °C for 10 min. Subsequently, an emissive layer of the TADF dendrimer was spin coated from a chlorobenzene solution and annealed at 100 °C for 5 min. Finally, TPBI (40 nm), Yb (5 nm) and silver (120 nm) cathodes were deposited via thermal evaporation under vacuum conditions (1 × 10−7 torr). Characterization of the device performance was measured using a Keithley 2400 meter with a BM-7AS luminance colorimeter in an F-star optical measurement system. The EL spectra and CIE coordinates were recorded using a PR-788 photometer.
Author contributions
Cheng Zhang: methodology, data curation, and writing – review and editing. Hao Yan: OLED device fabrication and characterization and writing – review and editing. Yuting He: conceptualization, investigation, and writing – original draft. Yongshuai Chai: software, data curation, and validation. Deyun Zhou: project administration, supervision, and writing – review and editing.
Conflicts of interest
There are no conflicts to declare.
Acknowledgements
This work was supported by the Natural Science Basic Research Plan in Shaanxi Province of China (Program No. 2021JQ-664), and the Natural Science Foundation of Shaanxi Provincial Department of Education (Program No. 19JK0379).
Notes and references
- Y. W. Xu, P. Xu, D. H. Hu and Y. G. Ma, Recent progress in hot exciton materials for organic light-emitting diodes, Chem. Soc. Rev., 2021, 50, 1030–1069 RSC.
- X. C. Fan, K. Wang, Y. Z. Shi, J. X. Chen, F. Huang, H. Wang, Y. N. Hu, Y. Tsuchiya, X. M. Ou, J. Yu, C. Adachi and X. H. Zhang, Managing intersegmental charge-transfer and multiple resonance alignments of D3-A typed TADF emitters for red OLEDs with improved efficiency and color purity, Adv. Opt. Mater., 2021, 10, 2101789 CrossRef.
- C. Wu, C. S. Shi, Y. Y. Zheng, J. Y. Zhang, Y. F. Wang, N. Sun, Q. Wang and Z. H. Lu, Multifunctional luminophores with dual emitting cores: TADF emitters with AIE properties for efficient solution- and evaporation-processed doped and non-doped OLEDs, Chem. Eng. J., 2022, 431, 133249 CrossRef CAS.
- B. Li, Z. Yang, W. Q. Gong, X. H. Chen, D. W. Bruce, S. Y. Wang, H. L. Ma, Y. Liu, W. G. Zhu, Z. G. Chi and Y. F. Wang, Intramolecular through-space charge transfer based TADF-active multifunctional emitters for high efficiency solution-processed OLED, Adv. Opt. Mater., 2021, 9, 2100180 CrossRef CAS.
- J. N. Sun, J. Zhang, Q. Q. Liang, Y. Wei, C. B. Duan, C. M. Han and H. Xu, Charge-transfer exciton manipulation based on hydrogen bond for efficient white thermally activated delayed fluorescence, Adv. Funct. Mater., 2020, 30, 1908568 CrossRef CAS.
- Y. Liu, X. Xiao, Y. Ran, Z. Y. Bin and J. S. You, Molecular design of thermally activated delayed fluorescent emitters for narrowband orange–red OLEDs boosted by a cyano-functionalization strategy, Chem. Sci., 2021, 12, 9408–9412 RSC.
- F. T. Liu, Z. Cheng, L. Wan, Z. J. Feng, H. Liu, H. X. Jin, L. Gao, P. Lu and W. S. Yang, Highly efficient multi-resonance thermally activated delayed fluorescence material with a narrow full width at half-maximum of 0.14 eV, Small, 2021, 18, 2106462 CrossRef PubMed.
- M. L. Yang, S. Shikita, H. Min, I. S. Park, H. Shibata, N. Amanokura and T. Yasuda, Wide-range color tuning of narrowband emission in multi-resonance organoboron delayed fluorescence materials through rational imine/amine functionalization, Angew. Chem., Int. Ed., 2021, 60, 23142–23147 CrossRef CAS PubMed.
- Y. Zou, S. L. Gong, G. H. Xie and C. L. Yang, Design strategy for solution-processable thermally activated delayed fluorescence emitters and their applications in organic light-emitting diodes, Adv. Opt. Mater., 2018, 6, 1800568 CrossRef.
- S. Y. Lee, T. Yasuda, H. Komiyama, J. Lee and C. Adachi, Thermally activated delayed fluorescence polymers for efficient solution-processed organic light-emitting diodes, Adv. Mater., 2016, 28, 4019–4024 CrossRef CAS PubMed.
- Y. K. Yang, S. M. Wang, Y. H. Zhu, Y. J. Wang, H. M. Zhan and Y. X. Cheng, Thermally activated delayed fluorescence conjugated polymers with backbone-donor/pendant-acceptor architecture for nondoped OLEDs with high external quantum efficiency and low roll-off, Adv. Funct. Mater., 2018, 28, 1706916 CrossRef.
- C. S. Li, Y. W. Xu, Y. C. Liu, Z. J. Ren, Y. G. Ma and S. K. Yan, Highly efficient white-emitting thermally activated delayed fluorescence polymers: Synthesis, non-doped white OLEDs and electroluminescent mechanism, Nano Energy, 2019, 65, 104057 CrossRef CAS.
- Y. Z. Shi, H. Wu, K. Wang, J. Yu, X. M. Ou and X. H. Zhang, Recent progress in thermally activated delayed fluorescence emitters for nondoped organic light-emitting diodes, Chem. Sci., 2022, 13, 3625–3651 RSC.
- C. Zhang, H. Zeng, Q. Y. Huang, Y. Wang, Y. S. Chai, Y. Huang, S. L. Zhao and Z. Y. Lu, High-performance red electrophosphorescent devices based on all-solution-processed hydrogen-bonded supramolecular material, J. Mater. Chem. C, 2018, 6, 4095–4105 RSC.
- C. Zhang, Y. Wang, Q. Y. Huang, J. Zhou, Y. Huang, S. L. Zhao and Z. Y. Lu, A novel H-bonding self-assembly heteromeric molecular duplex bearing host and guest energy transfer units as high-performance electroluminescent material, Dyes Pigm., 2018, 149, 755–763 CrossRef CAS.
- Y. T. He, D. Y. Zhou, C. Zhang and H. Yan, Yongshuai Chai, Orange-red and saturated red thermally activated delayed fluorescent dendrimers for non-doped solution-processed OLEDs, Dyes Pigm., 2022, 203, 110385 CrossRef CAS.
- X. Yin, Y. He, X. Wang, Z. X. Wu, E. B. Pang, J. Xu and J. A. Wang, Recent advances in thermally activated delayed fluorescent polymer-molecular designing strategies, Front. Chem., 2020, 8, 725 CrossRef CAS PubMed.
- Q. Xue and G. H. Xie, Thermally activated delayed fluorescence beyond through-bond charge transfer for high-performance OLEDs, Adv. Opt. Mater., 2021, 9, 2002204 CrossRef CAS.
- T. C. Jiang, Y. C. Liu, Z. J. Ren and S. K. Yan, The design, synthesis and performance of thermally activated delayed fluorescence macromolecules, Polym. Chem., 2020, 11, 1555–1571 RSC.
- F. M. Xie, J. X. Zhou, Y. Q. Li and J. X. Tang, Effects of the relative position and number of donors and acceptors on the properties of TADF materials, J. Mater. Chem. C, 2020, 8, 9476–9494 RSC.
- S. K. Jeon, H. L. Lee, K. S. Yook and J. Y. Lee, Recent progress of the lifetime of organic light-Emitting diodes based on thermally activated delayed fluorescent material, Adv. Mater., 2019, 31, 34 Search PubMed.
- Y. Zou, S. L. Gong, G. H. Xie and C. L. Yang, Design strategy for solution-processable thermally activated delayed fluorescence emitters and their applications in organic light-emitting diodes, Adv. Opt. Mater., 2018, 6, 1800568 CrossRef.
- M. Godumala, S. Choi, M. J. Cho and D. H. Choi, Recent breakthroughs in thermally activated delayed fluorescence organic light emitting diodes containing non-doped emitting layers, J. Mater. Chem. C, 2019, 7, 2172–2198 RSC.
- X. X. Ban, W. Jiang, K. Y. Sun, B. P. Lin and Y. M. Sun, Self-host blue dendrimer comprised of thermally activated delayed fluorescence core and bipolar dendrons for efficient solution-processable nondoped electroluminescence, ACS Appl. Mater. Interfaces, 2017, 9, 7339–7346 CrossRef CAS PubMed.
- C. S. Li, Z. J. Ren, X. L. Sun, H. H. Li and S. K. Yan, Deep-blue thermally activated delayed fluorescence polymers for nondoped solution-processed organic light-emitting diodes, Macromolecules, 2019, 52, 2296–2303 CrossRef CAS.
- C. S. Li, A. K. Harrison, Y. C. Liu, Z. N. Zhao, C. Zeng, F. B. Dias, Z. J. Ren, S. Yan and M. R. Bryce, Asymmetrical-dendronized TADF emitters for efficient non-doped solution-processed OLEDs by eliminating degenerate excited states and creating solely thermal equilibrium routes, Angew. Chem., Int. Ed., 2022, 61, e202115140 CAS.
- P. S. Ngo, M. K. Hung, K. W. Tsai, S. Sharma and S. A. Chen, Highly efficient solution-processed thermally activated delayed fluorescence bluish-green and hybrid white organic light-emitting diodes using novel bipolar host materials, ACS Appl. Mater. Interfaces, 2019, 11, 45939–45948 CrossRef CAS PubMed.
- J. H. Lee, C. H. Chen, P. H. Lee, H. Y. Lin, M. Y. Leung, T. L. Chiu and C. F. Lin, Blue organic light-emitting diodes: current status, challenges, and future outlook, J. Mater. Chem. C, 2019, 7, 5874–5888 RSC.
- B. H. Zhang and Y. X. Cheng, Recent advances in conjugated TADF polymer featuring in backbone-donor/pendant-acceptor structure: material and device perspectives, Chem. Rec., 2019, 19, 1624–1643 CrossRef CAS PubMed.
- C. S. Li, R. S. Nobuyasu, Y. K. Wang, F. B. Dias, Z. J. Ren, M. R. Bryce and S. K. Yan, Solution-processable thermally activated delayed fluorescence white OLEDs based on dual-emission polymers with tunable emission colors and aggregation-enhanced emission properties, Adv. Opt. Mater., 2017, 5, 1700435 CrossRef.
- Y. Zou, S. L. Gong, G. H. Xie and C. L. Yang, Design strategy for solution-processable thermally activated delayed fluorescence emitters and their applications in organic light-emitting diodes, Adv. Opt. Mater., 2018, 6, 1800568 CrossRef.
- C. Yin, D. D. Zhang and L. Duan, A perspective on blue TADF materials based on carbazole-benzonitrile derivatives for efficient and stable OLEDs, Appl. Phys. Lett., 2020, 116, 120503 CrossRef CAS.
- X. J. Zheng, R. J. Huang, C. Zhong, G. H. Xie, W. M. Ning, M. L. Huang, F. Ni, F. B. Dias and C. L. Yang, Achieving 21% external quantum efficiency for nondoped solution-processed sky-blue thermally activated delayed fluorescence OLEDs by means of multi-(donor/acceptor) emitter with through-space/-bond charge transfer, Adv. Sci., 2020, 7, 1902087 CrossRef CAS PubMed.
- J. Hwang, H. Kang, J. E. Jeong, H. Y. Woo, M. J. Cho, S. Park and D. H. Choi, Donor engineered deep-blue emitters for tuning luminescence mechanism in solution-processed OLEDs, Chem. Eng. J., 2021, 416, 129185 CrossRef CAS.
- B. Wex and B. R. Kaafarani, Perspective on carbazole-based organic compounds as emitters and hosts in TADF applications, J. Mater. Chem. C, 2017, 5, 8622–8653 RSC.
- K. Matsuoka, K. Albrecht, A. Nakayama, K. Yamamoto and K. Fujita, Highly efficient thermally activated delayed fluorescence organic light-emitting diodes with fully solution-processed organic multilayered architecture: impact of terminal substitution on carbazole-benzophenone dendrimer and interfacial engineering, ACS Appl. Mater. Interfaces, 2018, 10, 33343–33352 CrossRef CAS PubMed.
- J. J. Luo, S. L. Gong, Y. Gu, T. H. Chen, Y. F. Li, C. Zhong, G. H. Xie and C. L. Yang, Multi-carbazole encapsulation as a simple strategy for the construction of solution-processed, non-doped thermally activated delayed fluorescence emitters, J. Mater. Chem. C, 2016, 4, 2442–2446 RSC.
- K. Albrecht, E. Matsuoka, D. Yokoyama, Y. Sakai, A. Nakayama, K. Fujita and K. Yamamoto, Thermally activated delayed fluorescence OLEDs with fully solution processed organic layers exhibiting nearly 10% external quantum efficiency, Chem. Commun., 2017, 53, 2439–2442 RSC.
- Y. F. Li, G. H. Xie, S. L. Gong, K. L. Wu and C. L. Yang, Dendronized delayed fluorescence emitters for non-doped, solution-processed organic light-emitting diodes with high efficiency and low efficiency roll-off simultaneously: two parallel emissive channels, Chem. Sci., 2016, 7, 5441–5447 RSC.
- B. Huang, X. X. Ban, K. Y. Sun, Z. M. Ma, Y. N. Mei, W. Jiang, B. P. Lin and Y. M. Sun, Thermally activated delayed fluorescence materials based on benzophenone derivative as emitter for efficient solution-processed non-doped green OLED, Dyes Pigm., 2016, 133, 380–386 CrossRef CAS.
- P. Rajamalli, D. Y. Chen, W. B. Li, I. D. W. Samuel, B. C. David, M. Z. S. Alexandra and E. Zysman-Colman, Enhanced thermally activated delayed fluorescence through bridge modification in sulfone-based emitters employed in deep blue organic light-emitting diodes, J. Mater. Chem. C, 2019, 7, 6664–6671 RSC.
- H. Uoyama, K. Goushi, K. Shizu, H. Nomura and C. Adachi, Highly efficient organic light-emitting diodes from delayed fluorescence, Nature, 2012, 492, 234–238 CrossRef CAS PubMed.
- S. Hirata, Y. Sakai, K. Masui, H. Tanaka, S. Y. Lee, H. Nomura, N. Nakamura, M. Yasumatsu, H. Nakanotani, Q. S. Zhang, K. Shizu, H. Miyazaki and C. Adachi, Highly efficient blue electroluminescence based on thermally activated delayed
fluorescence, Nat. Mater., 2015, 14, 330–336 CrossRef CAS PubMed.
- K. C. Pan, S. W. Li, Y. Y. Ho, Y. J. shiu, W. L. Tsai, M. Jiao, W. K. Lee, C. C. Wu, C. L. Chung, T. Chatterjee, Y. S. Li, K. T. Wong, H. C. Hu, C. C. Chen and M.-T. Lee, Efficient and tunable thermally activated delayed fluorescence emitters having orientation-adjustable CN-substituted pyridine and pyrimidine acceptor units, Adv. Funct. Mater., 2016, 26, 7560–7571 CrossRef CAS.
- X. Zeng, K. C. Pan, W. K. Lee, S. L. Gong, F. Ni, X. Xiao, W. X. Zeng, Y. P. Xiang, L. S. Zhan, Y. Zhang, C. C. Wu and C. L. Yang, High-efficiency pure blue thermally activated delayed fluorescence emitters with a preferentially horizontal emitting dipole orientation via a spiro-linked double D-A molecular architecture, J. Mater. Chem. C, 2019, 7, 10851–10859 RSC.
- S. Y. Hsieh, Y. Tang, S. Crotti, E. A. Stone and S. J. Miller, Catalytic enantioselective pyridine N-oxidation, J. Am. Chem. Soc., 2019, 141, 18624–18629 CrossRef CAS PubMed.
|
This journal is © the Partner Organisations 2022 |