DOI:
10.1039/D1QM01540K
(Research Article)
Mater. Chem. Front., 2022,
6, 440-449
Synthesis of broadband NIR garnet phosphor Ca4ZrGe3O12: Cr3+, Yb3+ for NIR pc-LED applications
Received
20th November 2021
, Accepted 4th January 2022
First published on 5th January 2022
Abstract
There are outstanding application prospects for near-infrared phosphor-converted light-emitting diodes (NIR pc-LEDs) in many fields such as non-destructive detection, bio-imaging, and modern agriculture, in which the development of NIR-emitting phosphors with large full width at half maximum (FWHM), high efficiency, and excellent thermal stability is the challenge of current research. Herein, a broadband NIR-emitting phosphor Ca4ZrGe3O12: Cr3+ (CZGO: Cr3+) was successfully synthesized, which ranged from 650 to 1250 nm with 160 nm FWHM under the excitation of blue light at 477 nm. Based on the first-principles theory calculation, the origination of its matrix luminescence was accurately predicted, and the site-selective occupancy and the formation of various charge transfer bands (CTBs) have also been revealed in detail. With the introduction of Yb3+ into CGZO: Cr3+, the FWHM was broadened to 230 nm, and the thermal stability was also significantly increased. Furthermore, a NIR pc-LED device was fabricated by combining CZGO: Cr3+/Yb3+ with a 450 nm commercial LED chip, and it demonstrated satisfactory performance in night vision and bio-imaging.
1. Introduction
There is currently great interest in broadband near-infrared (NIR) light sources due to their numerous applications in bio-imaging, non-destructive detection, and night vision.1–5 Low efficiency and large size are always associated with traditional NIR light sources such as halogen lamps and tungsten filaments, while AlGaAs light-emitting diodes (LEDs) can achieve a NIR light with excellent efficiency and small size.6,7 However, bio-imaging and composition detection require a broadband NIR light source to expand the testing scope and obtain additional information,8–10 but the narrow full width at half maximum (FWHM) of AlGaAs LEDs (FWHM < 50 nm) limits their use in various practical applications. Thus, there has been increased interest in the newly emerged NIR phosphor-converted light-emitting diodes (pc-LEDs).11 By combining a commercial blue LED chip with a NIR-emitting phosphor, the fabricated LED device can realize a broadband NIR light with the advantages of high efficiency, low cost, and small size. However, there is a very large Stokes shift of the luminous center because of the huge energy difference between the emission and excitation light, which will inevitably produce a strong thermal effect, chromatic drift, and reduction in luminous intensity.12 Therefore, a thermally stable broadband NIR phosphor has become the main challenge at this stage.
Currently, a large number of Cr3+-doped NIR phosphors have been reported that can easily achieve a broadband NIR emission in a weak crystal field environment, such as Ca2LuScGa2Ge2O12: Cr3+ (FWHM = 150 nm) and LiInSi2O6: Cr3+ (FWHM = 143 nm).13,14 Multi-site occupancy of Cr3+ can also realize a broadband NIR emission, but the weak crystal field environment is still essential. Xie's group created La2MgZrO6: Cr3+ (FWHM = 210 nm), in which Cr3+ simultaneously occupied the Mg/ZrO6 octahedron sites and consequently emitted a broadband NIR light.15 Additionally, Yb3+ is always used to broaden the FWHM by the efficient energy transfer from Cr3+ to Yb3+, and thermal stability can also be increased because of its simple energy levels, which effectively avoid the energy loss from cross-relaxation between different energy levels.16–19
As Zhang's group reported, the FWHM of the garnet-structured phosphor Ca2LuZr2Al3O12: Cr3+ can be broadened from approximately 160 to 340 nm by the efficient energy transfer from Cr3+ to Yb3+, and the thermal stability (T0.5) can also be greatly increased from 450 to 650 K by introducing a high concentration of Yb3+.20 Notably, the garnet structure is always considered as an excellent and stable thermal matrix, and it is expected to be an ideal host for overcoming severe thermal quenching resulting from the huge Stokes shift of Cr3+, such as in Na3In2Li3F12: Cr3+ (82%@423 K)21 and Gd3Sc2Ga3O12: Cr3+ (92%@423 K).22 Therefore, Cr3+ and Yb3+ co-doped garnet-structured hosts are ideal for exploring broadband NIR-emitting phosphors with satisfactory thermal stability.
In this work, a garnet-structured phosphor CZGO: Cr3+, Yb3+ was prepared, in which the site selection occupancy of Cr3+ and Yb3+ was confirmed by the first-principles theory calculation. According to the partial density of states (PDOS) of the CZGO host, the host luminescence was identified as the electron transition generated by orbital coupling between the Zr and O atoms. Under the excitation of blue light at 477 nm, CZGO: Cr3+ showed a broadband (FWHM = 160 nm) NIR emission from 650 to 1250 nm, centered at 840 nm. Utilizing the introduction of Yb3+, the FWHM was broadened to 230 nm, and then, the thermal stability significantly increased. The energy transfer process between Cr3+ and Yb3+ was subsequently investigated by spectroscopy and decay curves. Combined with a commercial 450 nm LED chip, the fabricated LED device output a bright NIR light that provided a satisfactory performance when used for night vision and bio-penetration. These results illustrate that CZGO: Cr3+, Yb3+ possesses the potential for application in night vision and bio-imaging.
2. Experimental
2.1 Synthesis of samples
Phosphors CZGO: x% Cr3+, y% Yb3+ (x = 0, 0.6, 0.8, 1.0, 1.2, 1.5, 2.0; y = 0, 1, 2, 3, 4) were synthesized via a high-temperature solid-state reaction method. The stoichiometric analytical reagent (A. R.) raw materials CaCO3, ZrO2, Cr2O3, and high purity (99.99%) GeO2 and Yb2O3 were first ground for 20 min with a small amount of ethanol. Next, the mixture powder was pre-sintered at 650 °C for 2 h and sintered at 1400 °C for 4 h in air. Finally, the samples were obtained after natural cooling to room temperature.
2.2 Characterization
Powder X-ray diffraction (XRD) data was collected on Bruker D8 Advance powder diffractometer (Bruker Corporation, Germany) at 40 kV and 30 mA with Cu Kα radiation (λ = 1.5405 Å), and the step size of 2θ was 0.02°. Rietveld refinement was performed by General Structure Analysis System (GSAS) software.23 The diffusion reflection spectra (DRS) were measured by a ultraviolet-visible (UV-Vis) spectrophotometer (Cary 5000). The photoluminescence (PL) and excitation (PLE) spectra as well as the decay curves were obtained using an Edinburgh FLS920 fluorescence spectrophotometer (Edinburgh Instruments Ltd, UK) equipped with 450 W Xe lamp and a microsecond flash-lamp (uF920H) as a light source, respectively. The quantum yield (QY) was tested by the integrating sphere on the same Edinburgh FLS920 fluorescence spectrophotometer with BaSO4 as a reference. The temperature-dependent PL spectra (280–470 K) and a low-temperature spectrum at 4 K were obtained with an Oxford OptistatDN2 nitrogen cryogenics temperature controller and the Oxford OptistatDry system, respectively.
2.3 Computational details
The computation was performed by CASTEP.24 The band structure, partial density of states (PDOS), and formation energy were calculated by density functional theory (DFT) in the form of the generalized gradient approximation (GGA) Perdew–Burke–Ernzerhof (PBE) function.25 A Monkhorst–Pack 3 × 3 × 2 κ mesh was used as the Brillouin zone, and the kinetic energy cutoff and SCF were set as 500 eV and 10−5 eV per atom, respectively.
3. Results and discussion
3.1 Phase and structure
To verify the phase purity, powder XRD Rietveld refinements of blank and Cr3+/Yb3+ co-doped CZGO were representatively performed. Fig. 1a and b show that all Bragg reflections coincided well with those of the calculated data, and residual factors were low (Rwp = 8.3% and Rp = 6.1% for the blank sample; Rwp = 9.0% and Rp = 6.5% for Cr3+/Yb3+ co-doped CZGO). Accordingly, the samples were successfully synthesized, and Cr3+/Yb3+ entered into the host. The crystallographic parameters are listed in Table 1. As depicted in Fig. 1c, the garnet structure CZGO exhibits characteristics of a cubic phase with the Ia
d space group. There are four Wyckoff sites, 24c, 16a, 24d, and 96h, which are occupied by Ca1, Ca2/Zr, Ge, and O, respectively. In particularly, Ca1 is coordinated with 8 oxygen atoms, and Ca2 and Zr equally share the 16a site with a coordination number (CN) of 6. Theoretically, Cr3+/Yb3+ [CN = 6, r(Cr3+) = 0.615 Å, r(Yb3+) = 0.868 Å; CN = 8, r(Yb3+) = 0.985 Å] can occupy the Ca1O8 [CN = 8, r(Ca2+) = 1.12 Å] and Ca2/ZrO6 [CN = 6, r(Ca2+) = 1.00, r(Zr4+) = 0.720 Å] sites due to their close radius and valence.26 To confirm the site occupancy selection of Cr3+ and Yb3+, the formation energy (Eform) was computed by the first-principles theory calculation according to the following equation: | Eform = Edoped − Epure − n × Edopant + m × Eatom | (1) |
where Edoped and Epure represent the total energy of the host with or without dopant, respectively; Edopant and Eatom denote the atomic energy of the dopant and the atom replaced by dopant, respectively; n and m denote the number of the dopant and the atom replaced by dopant, respectively. Obviously, the Cr substituting for Zr possesses the lowest Eform (−9.5 eV), implying that it is most likely that Cr3+ will occupy the ZrO6 site.27 Likewise, the Yb3+ prefers to enter into the Ca1O8 (Eform = −6.5 eV) site than others (Fig. 1d).
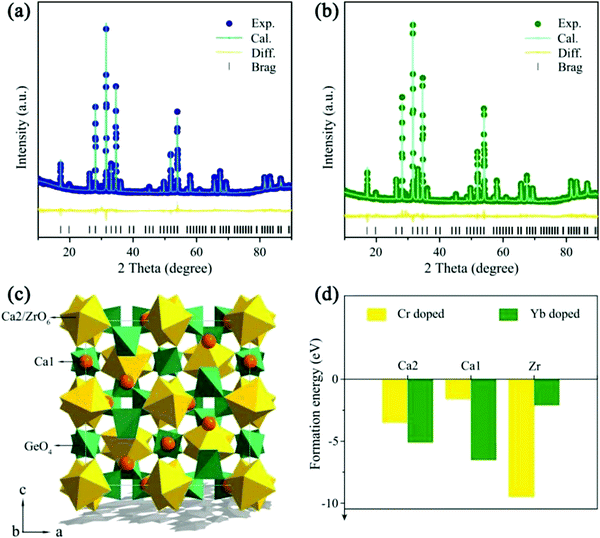 |
| Fig. 1 Powder XRD Rietveld refinements of (a) blank CZGO and (b) CZGO: 1% Cr3+, 1% Yb3+; (c) the crystalline structure and cationic sites in CZGO; (d) the formation energy of Cr or Yb doped into the different cationic sites Ca1O8, Ca2/ZrO6, and GeO4. | |
Table 1 Rietveld refinement and crystallographic parameters of the CZGO blank and CZGO: 1% Cr3+ and 1% Yb3+
Compound |
CZGO |
CZGO: 1% Cr3+ and 1% Yb3+ |
Space group |
Ia d |
Ia d |
a/c, Å |
12.7 |
12.7 |
V, Å3 |
2057.5 |
2055.3 |
R
wp, % |
8.3 |
9.0 |
R
p, % |
6.1 |
6.5 |
χ
2
|
2.1 |
2.3 |
The diffusion reflection spectra (DRS) of the blank, and CZGO singly or co-doped with Cr3+/Yb3+ are illustrated in Fig. 2a. Except for the matrix absorption band at approximately 220 nm, a Zr–O charge transfer band (CTB) is observed at 280 nm in the CZGO host. The DRS of Yb-doped CZGO is similar to that of the blank host, but a slight absorption enhancement at 280 nm is likely caused by Yb–O CTB. On the contrary, a significant absorption band occurs at 260 nm in the Cr3+-doped sample that belongs to Cr–O CTB. Other absorption bands at 390, 477, and 680 nm correspond to transition 4A2(G)–4T1(P), 4A2(G)–4T1(F), and 4A2(G)–4T2(F) of Cr3+, respectively. The Cr3+ singly doped and Cr3+/Yb3+ co-doped samples exhibit similar DRS, meaning that there is efficient energy transfer from the Cr3+ to Yb3+ ions. The optical band gap can be estimated by the Kubelka–Munk function:28
| 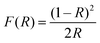 | (2) |
| [hνF(R∞)]2 = A(hν − Eg) | (4) |
where
R denotes the diffuse reflectance,
hν denotes the photon energy, and
A represents an absorption constant. For the direct and indirect band gap,
n is equal to 2 and 1/2, respectively. Based on the following first-principles theory calculation, CZGO is characterized by a direct band gap. The band gap was calculated to be 5.2, 5.1, 4.9, and 4.8 eV for the blank, Yb
3+-doped, Cr
3+-doped, and Cr
3+/Yb
3+ co-doped sample, respectively (
Fig. 2b).
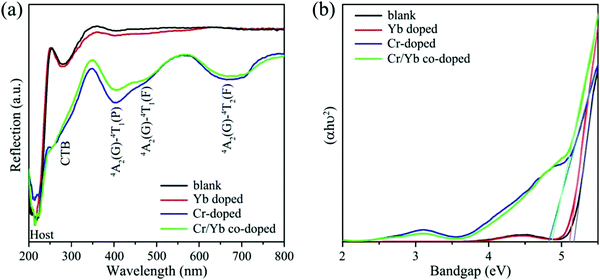 |
| Fig. 2 The (a) DRS and (b) Tucker curves of the blank, and CZGO singly or co-doped with Cr3+/Yb3+. | |
To further confirm the contribution of CTBs in Fig. 2a and reveal the composition of the conduction (CB) and valence band (VB), the band structure and PDOS were calculated by the first-principles theory calculation. As shown in Fig. 3a, the CZGO is characterized by a direct band gap, and the band gap (Eg) is 3.50 eV, which is smaller than that of the experimental value due to the underestimated interaction between electrons arrived at by the GGA-PBE method.29 Obviously, the CB is mainly constructed by the d orbital of Ca and Zr, yet the p orbital of O and Zr form the VB. Notably, there is a similar p orbital for Zr and O, suggesting that their p orbital is coupled. Therefore, the electron of the Zr d orbital transferred to the O p orbital, and a Zr–O bond was formed.
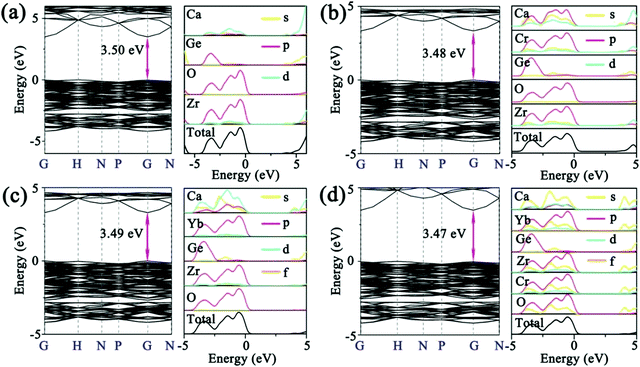 |
| Fig. 3 The band structure and PDOS of (a) the blank, and (b) Cr-doped, (c) Yb-doped, and (d) Cr/Yb co-doped CZGO. | |
When the host is excited by n-UV light, it is possible for electrons to jump from the O p orbital (VB) to the Zr d orbital (CB), relax to the natural defect energy level between band gaps, and then finally travel back to the VB and emit host luminescence. Thus, a CTB might be generated between Zr and O atoms, and the host is expected to emit matrix luminescence. Additionally, the partial p and d orbitals of Ca are similar to that of Zr, resulting from Ca2 and Zr equally sharing the 16a Wyckoff site, in which Zr and Ca possess the same symmetry and energy level splitting. After the host was doped with 1% Cr, the band gap slightly decreased to 3.48 eV (Fig. 3b). Apparently, the PDOS of Cr is similar to that of Zr, resulting from their same crystal field environment. The p orbital of Cr and O were also coupled, which might generate Cr–O CTB. Similarly, Yb was also orbitally coupled with O, indicating that the excitation of Yb3+ in CZGO most likely simultaneously originated from the Yb–O CTB and the energy transfer from the host or Zr–O (Fig. 3c). When the CZGO host was co-doped with 1% Cr3+ and 1% Yb3+, a similar PDOS was obtained for Cr, Yb, Ca, and Zr because of their site sharing (Fig. 3d). Additionally, there is a similar orbital couple of Cr and O that is expected to transfer energy to Yb3+ in the same manner as that to the Zr–O CTB. Additionally, the band gap reached the lowest value of 3.47 eV because a greater number of defects might be introduced in the co-doped host as compared to the singly doped host.
3.2 Luminescence properties
The PL and PLE spectra for CZGO: 1% Cr3+ are displayed in Fig. 4a. Under the excitation of 480 nm light, Cr3+ emits a broad NIR band (650–1250 nm) centered at 840 nm with a 160 nm large FWHM, corresponding to a 4T2(F)–4A2(F) transition. The PLE spectrum monitored at 840 nm consists of four bands at 280, 300, 477, and 680 nm, originating from the CTB, and 4A2(F)–4T1(P), 4A2(F)–4T1(F), and 4A2(F)–4T2(F) transitions, respectively. To optimize the luminescence intensity, various concentrations of CZGO: x% Cr3+ (x = 0.6, 0.8, 1.0, 1.2, 1.5, and 2.0) were synthesized, and their normalized integrated emission intensities and quantum yield (QY) are listed in Table 2. The emission intensity continuously increases until the concentration of Cr3+ becomes greater than 1.0%, which occurs due to the concentration quenching effect. The QY reaches the maximum value of 35% when the amount of Cr3+ equals 1%.
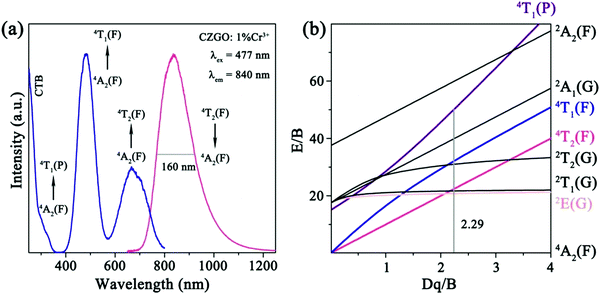 |
| Fig. 4 (a) The PL and PLE spectra together with (b) a Tanabe–Sugano energy level diagram of Cr3+ in CZGO. | |
Table 2 The normalized emission intensity and internal quantum yield of CZGO: x%Cr3+
x (%)
|
0.6
|
0.8
|
1.0
|
1.2
|
1.5
|
2.0
|
Normalized intensity |
0.4 |
0.7 |
1.0 |
0.8 |
0.7 |
0.5 |
QY (%) |
14 |
23 |
35 |
26 |
22 |
18 |
The emission of Cr3+ is strongly dependent on the crystal field environment of the octahedron (Oh symmetry), in which the crystal field strength and splitting can be roughly estimated by the Tanabe–Sugano theory using the following equations:30
where
Dq denotes the crystal field strength, and the Rach parameter
B can be calculated by:
|  | (6) |
in which
x is defined as:
| 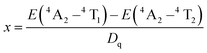 | (7) |
E(
4T
1–
4A
2) and
E(
4T
2–
4A
2) were determined to be 20
![[thin space (1/6-em)]](https://www.rsc.org/images/entities/char_2009.gif)
964 and 14
![[thin space (1/6-em)]](https://www.rsc.org/images/entities/char_2009.gif)
706 cm
−1, respectively. Accordingly,
Dq,
B, and
Dq/
B were calculated to be 1470 cm
−1, 642 cm
−1, and 2.29, respectively (
Fig. 4b). The
Dq/
B value was slightly less than 2.3, which indicates that the crystal field strength for Cr
3+ in CZGO is weak, and
4T
2(F) is below the
2E(G) level.
31 Consequently, Cr
3+ emits a broad NIR emission band, derived from the
4T
2(F)–
4A
2(F) transition.
As predicted above by the first-principles theory calculation in Fig. 3a, host emission is expected, and a band emission centered at 740 nm was displayed (Fig. 5a). Additionally, the PLE spectrum includes a strong excitation band centered at 280 nm, which was assigned to the electron transition from the O-p to Zr-d orbital in the Zr–O CTB. The corresponding experimental band gap is approximately 5.1 eV, which is greater than that obtained by theoretical calculation (Eg = 3.5 eV) due to the underestimates of the atomic reaction. However, the emission peak (740 nm, 1.68 eV) is far less than the value of the band gap, and this can be inferred as a defect energy level that is caused by an oxygen vacancy.
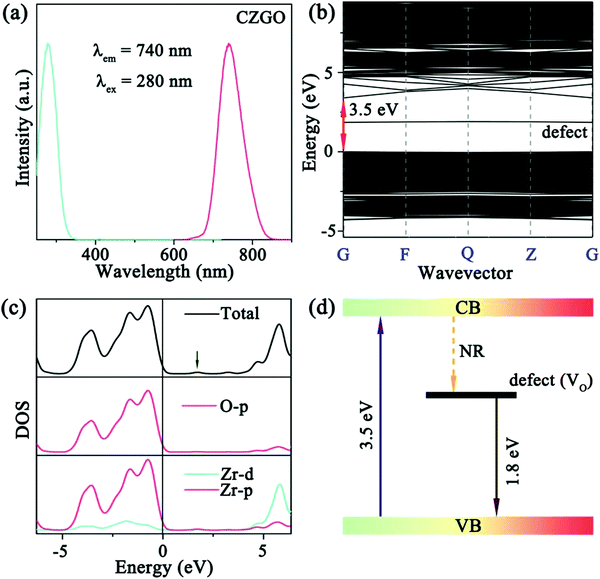 |
| Fig. 5 (a) The PL and PLE spectra of the blank; (b) the band structure and (c) DOS of CZGO with an oxygen vacancy (Vo) together with (d) the mechanism of the matrix luminescence. | |
To confirm our speculation and reveal the mechanism of matrix luminescence, the band structure and DOS of the matrix with one oxygen vacancy were calculated. As shown in Fig. 5b and c, a defect energy level can be easily observed after introducing an oxygen vacancy (Vo), and the energy difference between the defect and the VB was calculated to be 1.8 eV (approximately 689 nm), which is very close to the emission peak (740 nm). Accordingly, the coupling of the orbitals constructs the Zr–O CTB, which corresponds to the excitation band. The defect energy level most likely originates from the oxygen vacancy, and the transition between the defect and VB forms the emission band. Consequently, the detailed mechanism of the matrix luminescence consists of an electron that is pumped from the VB (O-p orbital) to the CB (Zr-d orbital) by n-UV light (280 nm), transfers to the defect energy level (Vo) by non-radiation relaxation (NR), and then immediately returns to the VB and subsequently releases far-red light (Fig. 5d).
To further refine the practical application value, Yb3+ was introduced into CZGO: Cr3+ to broaden the FWHM by energy transfer from the Cr3+ to Yb3+ ion. For a comprehensive understanding of the energy transfer between the host or Cr3+ and Yb3+, the PL and PLE spectra of the blank, and Yb3+-doped and Cr3+–Yb3+ co-doped samples are displayed in Fig. 6a. The Yb3+ singly doped CZGO exhibits two emission bands at 750 nm – one from the host, and one typical emission band centered at 968 nm from the 2F7/2 → 2F5/2 transition of Yb3+. A comparison of the PL and PLE spectra of the host and CZGO: 1% Yb3+ confirms that the excitation bands of singly doped Yb3+ are the same as those of the host, which implies that energy was transferred from the Zr–O CTB to Yb3+.
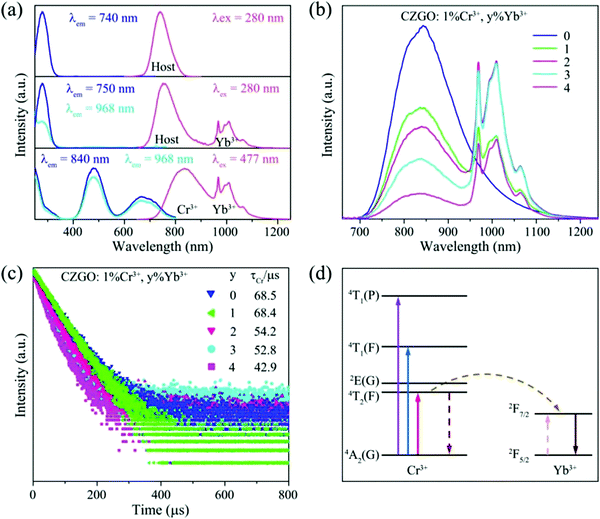 |
| Fig. 6 (a) The PL and PLE spectra of the blank, and Yb3+-doped and Cr3+/Yb3+-co-doped CZGO; (b) PL spectra and (c) decay curves of CZGO: 1% Cr3+, y% Yb3+ (y = 0, 1, 2, 3, 4) excited by 480 nm light together with (d) an energy transfer schematic diagram between Cr3+ and Yb3+. | |
Although there is no overlap between the excitation band of Yb3+ and the emission band of Cr3+, there is also a significant energy transfer process from Cr3+ to Yb3+ because the PLE spectra of Yb3+ is the same as that of Cr3+, except for the intensity in Cr3+–Yb3+ co-doped CZGO. As mentioned above, similar to Zr, Cr3+ enters into the ZrO6 site and couples with O in the p orbital, so that Cr3+ can also realize energy transfer to Yb3+ in the same manner as that which occurred with Zr–O CTB. To obtain the most optimal luminescence properties, the emission spectra of CZGO: 1% Cr3+, y% Yb3+ (y = 0, 1, 2, 3, 4) were measured and are provided in Fig. 6b as a function of the concentration of Yb3+. With increasing Yb3+, the FWHM increased from 160 to 230 nm after doping 1% Yb3+ into CZGO: 1% Cr3+, and the integrated emission intensity was maintained at 73% of CZGO singly doped with Cr3+.
To further verify the energy transfer process between Cr3+ and Yb3+, decay curves of Cr3+ in CZGO: 1% Cr3+, y% Yb3+ (y = 0, 1, 2, 3, 4) were measured and are depicted in Fig. 6c. These can be well fitted by second-order exponential functions according to the following equation:32
| 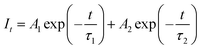 | (8) |
where
It represents the luminescence intensity at time
t,
A1 and
A2 denote constants, and
τ1 and
τ2 denote the decay times for exponential components. The average lifetime can be estimated by:
| 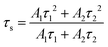 | (9) |
As the concentration of Yb3+ increased from 0 to 4%, the calculated average lifetime of Cr3+ monitored at 840 nm gradually decreased from 68.5 to 42.9 μs, verifying the existence of an efficient energy transfer between Cr3+ and Yb3+. As shown in Fig. 6d, under near-ultraviolet or blue/red light irradiation, the electrons of Cr3+ jump from ground state 4A2(G) to excited states, and then relax to the lowest excited state 4T2(F). Next, some of them return to the ground state 4A2(G) and emit a broad NIR band centered at 840 nm, while others transfer their energy to Yb3+ ions, and the electrons at 2F5/2 are excited to 2F7/2. Finally, the electrons return to 2F5/2 and also release a wide emission band with peaks at 968 and 1009 nm. Consequently, the FWHM of CZGO: Cr3+ is considerably broadened from 160 to 230 nm by the introduction of Yb3+.
3.3 Thermal stability
The thermal stability of phosphors coated on LED chips plays an important role in the process of deriving practical applications, because the operating temperature of LEDs is always greater than 100 °C (373 K). To evaluate the thermal stability, the temperature-dependent emission spectra of singly doped Cr3+ and Cr3+/Yb3+ co-doped CZGO were obtained and are displayed in Fig. 7a and b, respectively. With increasing temperature, the emission intensity of Cr3+-doped CZGO gradually decreased, but the emission position did not change. After CZGO: Cr3+ was doped with Yb3+, the Cr3+ in the co-doped sample exhibited the same thermal quenching behavior as the singly doped sample, but higher thermal resistance was exhibited by Yb3+. As shown in Fig. 7c, at the operating temperature of LEDs (373 K), the emission intensity of CZGO: Cr3+ and CZGO: Cr3+/Yb3+ was maintained 69% and 73% of the initial intensity at room temperature, respectively. Obviously, the thermal stability was increased with the introduction of Yb3+, which also occurred with Ca2LuZr2Al3O12.15 The thermal quenching mechanism is illustrated in the configuration coordinate diagram shown in Fig. 7d. Electrons at ground state 4A2(F) are pumped to excited states and then relax to the lowest excited state 4T2(F), where a portion of them absorb additional thermal vibration energy to reach the cross point between 4A2(F) and 4T2(F). When the electrons return to the ground state by cross-relaxation, thermal quenching ensues. The additional energy is defined as thermal activation energy ΔEa, which can be estimated by the following equation:33 | 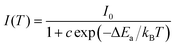 | (10) |
where I0 and I(T) represent the integrated emission intensity at the initial temperature and temperature T, c denotes a constant, and kB denotes the Boltzmann constant (kB = 8.62 × 10−5 eV). The calculated ΔEa of CZGO: Cr3+/Yb3+ is 4.3 eV, which is higher than that of CZGO: Cr3+ (3.8 eV). However, the Cr3+ emission exhibits the same thermal quenching behavior in the CZGO: Cr3+ and CZGO: Cr3+/Yb3+ systems, suggesting that the enhancement of thermal resistance is due to the introduction of Yb3+ instead of the improvement in ΔEa. This occurs because Yb3+ possesses the simple ground state 2F5/2 and excited state 2F7/2, which effectively prevents energy loss due to cross-relaxation between different energy levels. On the contrary, the thermal quenching behavior of Yb3+ is mainly dominated by multi-phonon emission, and the thermal quenching intensity is always less than that of the cross-relaxation effect.14 Therefore, the increased thermal stability is described as the transfer of energy from Cr3+ to the more stable Yb3+. It can also be predicted that the thermal stability of the sample will be further increased with increasing Yb3+, but the emission intensity and FWHM will decrease.
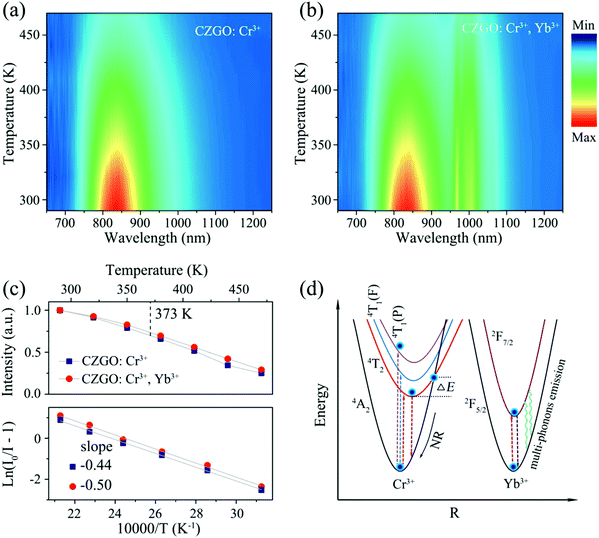 |
| Fig. 7 The temperature-dependent PL spectra of (a) 1% Cr3+ and (b) 1% Cr3+/1% Yb3+ co-doped CZGO; (c) normalized integrated intensity and a plot of ln(I0/I – 1) vs. 10 000/T for Cr3+ and Cr3+/Yb3+ co-doped CZGO; (d) a configuration coordinate diagram for Cr3+ and Yb3+ in the CZGO host. | |
3.4 Packaged NIR pc-LED application characteristics
NIR light sources have been widely applied for night vision and bio-imaging because they are invisible to human eyes and possess satisfactory bio-penetration.34 Aimed at these applications, we fabricated a NIR pc-LED by combining a 450 nm commercial chip with CZGO: Cr3+/Yb3+, which consists of a blue and broadband NIR spectra (Fig. 8a). Fig. 8b shows photographs of fruits and a hand illuminated by the white LED and fabricated NIR pc-LED light sources. The fruits are colorful under the white LED illumination, while a black and white image appears when they are illuminated by the fabricated NIR pc-LED. Additionally, the hand is covering the fabricated NIR pc-LED light source, and some blood vessels in the hand can be clearly observed by a Canon camera with a 760 nm filter, which confirms that the bio-penetration of the NIR light source is satisfactory. These features indicate that CZGO: Cr3+/Yb3+ possesses considerable potential for use in night vision and bio-imaging.
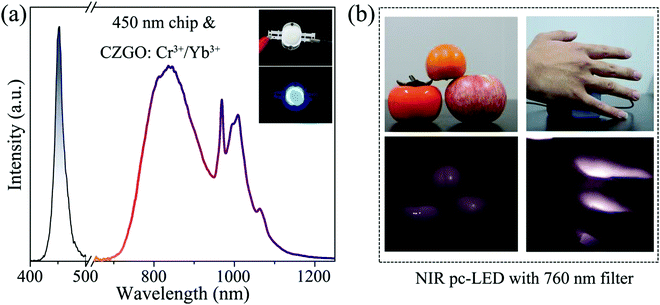 |
| Fig. 8 (a) The output spectrum of fabricated NIR pc-LED packaged by combining a 450 nm chip with CZGO: Cr3+/Yb3+. The inset shows the fabricated LED devices. (b) Photographs of various fruit and a hand under a white LED and NIR pc-LED with a 760 nm filter. | |
4. Conclusions
Using a high-temperature solid-state reaction method, we developed a NIR phosphor CZGO: Cr3+ that exhibits a broad emission band ranging from 650 to 1250 nm with a FWHM of 160 nm. Based on first-principles theory calculation, it was determined that the formation of CTBs occurred due to the p orbital coupling of Zr4+, Cr3+, Yb3+, and O2− atoms, and the host luminescence most likely originated from the electron transition between the d orbital of Zr4+ and the p orbital of O2−.
By comparing the formation energy, the site-selective occupancy was revealed as the Cr3+ and Yb3+ preferring to enter into the ZrO6 and CaO8 polyhedrons, respectively. Utilizing the introduction of Yb3+, the FWHM of Cr3+ was further broadened to 230 nm, and the thermal stability was also increased by effective energy transfer from Cr3+ to the more stable Yb3+ ions. The energy transfer process was also investigated by spectroscopy and decay curves. Finally, a NIR pc-LED was fabricated by combining CZGO: Cr3+, Yb3+ with a 460 nm LED chip, which exhibited a satisfactory bio-penetration ability and night vision features. These results illustrate that there is potential application for CZGO: Cr3+, Yb3+ in non-destructive detection and night vision.
Author contributions
Jinmeng Xiang was responsible for performing the experiments, analyzing the data, and writing the first draft. The contributor's roles were conceptualization, investigation, and writing the original draft. Jiming Zheng was responsible for assisting with theoretical calculations. Xiaoqi Zhao was responsible for testing the low-temperature spectrum and packaging the LED device. The contributor's role was providing partial resources. Xue Zhou was responsible for assisting with partial experiments. The contributor's role was data curation. Changheng Chen was responsible for analyzing the thermal quenching mechanism. The contributor's role was validation. Minkun Jin was responsible for assisting with partial graphics. The contributor's role was software. Chongfeng Guo was responsible for the supervision and leadership of the overall work. The contributor's roles were conceptualization, funding acquisition, project administration, supervision, and writing-review and editing.
Conflicts of interest
There are no conflicts to declare.
Acknowledgements
This work was supported by the National Natural Science Foundation of China (No. 51672215 and 11274251), Natural Science Basic Research Program of Shaanxi (Program No. 2019JZ-32), and The Youth Innovation Team of Shaanxi Universities.
References
- E. H. Song, H. Ming, Y. Y. Zhou, F. Q. He, J. C. Wu, Z. G. Xia and Q. Y. Zhang, Cr3+-doped Sc-based fluoride enabling highly efficient near infrared luminescence: a case study of K2NaScF6: Cr3+, Laser Photonics Rev., 2021, 15, 2000410 CrossRef CAS.
- Y. Wei, Z. Y. Gao, X. H. Yun, H. Yang, Y. X. Liu and G. G. Li, Abnormal Bi3+-activated NIR emission in highly symmetric XAl12O19 (X = Ba, Sr, Ca) by selective sites occupation, Chem. Mater., 2020, 32, 8747–8753 CrossRef CAS.
- Z. Y. Yang, Y. F. Zhao, Y. Y. Zhou, J. W. Qiao, Y. C. Chuang, M. S. Molokeev and Z. G. Xia, Giant red-shifted emission in (Sr,Ba)Y2O4: Eu2+ phosphor toward broadband near-infrared luminescence, Adv. Funct. Mater., 2021, 2103928–2103939 Search PubMed.
- B. B. Su, M. Z. Li, E. H. Song and Z. G. Xia, Sb3+-doping in cesium zinc halide single crystals enabling high-efficiency near-infrared emission, Adv. Funct. Mater., 2021, 31, 2105316 CrossRef CAS.
- J. W. Qiao, G. J. Zhou, Y. Y. Zhou, Q. Y. Zhang and Z. G. Xia, Divalent europium-doped near-infrared-emitting phosphor for light-emitting diodes, Nat. Commun., 2019, 10, 5267–5273 CrossRef.
- Z. W. Jia, C. X. Yuan, Y. F. Liu, X. J. Wang, P. Sun, L. Wang, H. C. Jiang and J. Jiang, Strategies to approach high performance in Cr3+-doped phosphors for high-power NIR-LED light sources, Light: Sci. Appl., 2020, 9, 86–94 CrossRef CAS.
- X. F. Zhou, W. Y. Geng, J. Y. Li, Y. C. Wang, J. Y. Ding and Y. H. Wang, An ultraviolet-visible and near-infrared-responded broadband NIR phosphor and its NIR spectroscopy application, Adv. Opt. Mater., 2020, 1902003 CrossRef CAS.
- C. P. Wang, X. M. Wang, Y. Zhou Yang, S. Zhang, C. Li, D. F. Hu, L. Xu and H. Jiao, An ultra-broadband near-infrared Cr3+-activated gallogermanate Mg3Ga2GeO8 phosphor as light sources for food analysis, ACS Appl. Electron. Mater., 2019, 1, 1046–1053 CrossRef CAS.
- V. Rajendran, M. Fang, N. D. G. Guzman, T. Lesniewski, S. Mahlik, M. Grinberg, G. Leniec, M. S. Kaczmarek, Y. Lin, K. Lu, C. Lin, H. Chang, S. Hu and R. S. Liu, Super broadband near-infrared phosphors with high radiant flux as future light sources for spectroscopy applications, ACS Energy Lett., 2018, 3, 2679–2684 CrossRef CAS.
- L. L. Zhang, D. D. Wang, Z. D. Hao, X. Zhang, G. H. Pan, H. J. Wu and J. H. Zhang, Cr3+-doped broadband NIR garnet phosphor with enhanced luminescence and its application in NIR spectroscopy, Adv. Opt. Mater., 2019, 1900185 CrossRef.
- D. C. Yu, Y. S. Zhou, C. S. Ma, J. M. Melman, K. M. Baroudi, M. LaCapra and R. E. Riman, Non-rare-earth Na3AlF6: Cr3+ phosphors for far-red light-emitting diodes, ACS Appl. Electron. Mater., 2019, 1, 2325–2333 CrossRef CAS PubMed.
- Q. Wei, J. Y. Ding and Y. H. Wang, A novel tunable extra-broad yellow-emitting nitride phosphor with zero-thermal-quenching property, Chem. Eng. J., 2020, 386, 124004 CrossRef CAS.
- B. Bai, P. P. Dang, D. Y. Huang, H. Z. Lian and J. Lin, Broadband near-infrared emitting Ca2LuScGa2Ge2O12: Cr3+ phosphors: luminescence properties and application in light-emitting diodes, Inorg. Chem., 2020, 59, 13481–13488 CrossRef CAS PubMed.
- X. X. Xu, Q. Y. Shao, L. Q. Yao, Y. Dong and J. Q. Jiang, Highly efficient and thermally stable Cr3+-activated silicate phosphors for broadband near-infrared LED applications, Chem. Eng. J., 2020, 383, 123108 CrossRef CAS.
- H. T. Zeng, T. L. Zhou, L. Wang and R. J. Xie, Two-site occupation for exploring ultra-broadband near-infrared phosphor-double-perovskite La2MgZrO6: Cr3+, Chem. Mater., 2019, 31, 5245–5253 CrossRef CAS.
- G. C. Liu, T. Hu, S. Maxim Molokeev and Z. G. Xia, Li/Na substitution and Yb3+ co-doping enabling tunable near-infrared emission in LiIn2SbO6: Cr3+ phosphors for lighting-emitting diodes, iScience, 2021, 24, 102250 CrossRef CAS.
- L. Q. Yao, Q. Y. Shao, S. Y. Han, C. Liang, J. H. He and J. Q. Jiang, Enhancing near-infrared photoluminescence intensity and spectral properties in Yb3+ co-doped LiScP2O7: Cr3+, Chem. Mater., 2020, 32, 2430–2439 CrossRef CAS.
- L. Q. Yao, Q. Y. Shao, X. X. Xue, Y. Dong, C. Liang, J. H. He and J. Q. Jiang, Broadband emission of single-phase Ca3Sc2Si3O12: Cr3+/Ln3+ (Ln = Nb, Yb, Ce) phosphors for novel solid-state light sources with visible to near-infrared light output, Ceram. Int., 2019, 45, 14249–14255 CrossRef CAS.
- Q. Y. Shao, H. Ding, L. Q. Yao, J. F. Xu, C. Liang, Z. H. Li, Y. Dong and J. Q. Jiang, Broadband near-infrared light source derived from Cr3+-doped phosphors and a blue LED chip, Opt. Lett., 2018, 43, 5251–5254 CrossRef CAS PubMed.
- S. He, L. L. Zhang, H. Wu, H. J. Wu, G. H. Pan, Z. D. Hao, X. Zhang, L. G. Zhang, H. Zhang and J. H. Zhang, Efficient super broadband NIR Ca2LuZr2Al3O12: Cr3+, Yb3+ garnet phosphor for pc-LED light source toward NIR spectroscopy applications, Adv. Opt. Mater., 2020, 1901684 CrossRef CAS.
- W. D. Nie, Y. Li, J. X. Zuo, Y. K. Kong, W. F. Zou, G. Chen, J. Q. Peng, F. Du, L. Han and X. Y. Ye, Cr3+-activated Na3X2Li3F12 (X = Al, Ga, In) garnet phosphors with broadband NIR emission and high luminescence efficiency for potential biomedical application, J. Mater. Chem. C, 2021, 9, 15230–15241 RSC.
- E. T. Basore, W. G. Xiao, X. F. Liu, J. H. Wu and J. R. Qiu, Broadband near-infrared garnet phosphors with near-unity internal quantum efficiency, Adv. Opt. Mater., 2020, 2000296 CrossRef CAS.
- B. H. Toby, EXPGUI, a graphical user interface for GASA, J. Appl. Crystallogr., 2001, 34, 210–213 CrossRef CAS.
- M. D. Segall, P. J. D. Lindan, M. J. Probert, C. J. Pickard, P. J. Hasnip, S. J. Clark and M. C. Payne, First-principles simulation: ideas, illustrations and the CASTEP code, J. Phys.: Condens. Matter, 2002, 14, 2717–2744 CrossRef CAS.
- M. Ernzerhof and G. E. Scuseria, Assessment of the Perdew-Burke-Ernzerhof exchange-correlation functional, J. Chem. Phys., 1999, 110, 5029–5036 CrossRef CAS.
- R. D. Shannon, Revised effective ionic radii and systematic studies of interatomic distance in halides and chalcogenides, Acta Crystallogr., Sect. A: Cryst. Phys., Diffr., Theor. Gen. Crystallogr., 1976, 32, 751–767 CrossRef.
- J. W. Lee, S. P. Singh, M. Kim, S. U. Hong, W. B. Park and K. S. Sohn, Metaheuristics-assisted combinatorial screening of Eu2+-doped Ca-Sr-Ba-Li-Mg-Al-Si-Ge-N compositional space in search of a narrow-band green emitting phosphor and density functional theory calculations, Inorg. Chem., 2017, 56, 9814–9824 CrossRef CAS.
- A. A. Christy, O. M. Kvalheim and R. A. Velapoldi, Quantitative analysis in diffuse reflectance spectrometry: A modified Kubelka-Munk equation, Vib. Spectrosc., 1995, 9, 19–27 CrossRef CAS.
- J. Zhu, M. H. Yang, Y. Che, Z. H. Zhang, X. C. Ge, J. Y. Xiang, Y. Zhou, K. Xiong and H. W. Yu, Structure-optical behavior correlation, optimized photoluminescence, and DFT calculation of La7O6(BO3)(PO4)2: Sm3+ micropowder for solid state lighting, ACS Appl. Electron. Mater., 2019, 1, 1688–1697 CrossRef CAS.
- Z. W. Zhou, J. M. Zheng, R. Shi, N. M. Zhang, J. Y. Chen, R. Y. Zhang, H. Suo, G. M. Ewa and C. F. Guo,
Ab initio site Occupancy and far-red emission of Mn4+ in cubic-phase La(MgTi)1/2O3 for plant cultivation, ACS Appl. Mater. Interfaces, 2017, 9, 6177–6185 CrossRef CAS PubMed.
- H. H. Lin, G. X. Bai, T. Yu, M. K. Tsang, Q. Y. Zhang and J. H. Hao, Site occupancy and near-infrared luminescence in Ca3Ga2Ge3O12: Cr3+ persistent phosphor, Adv. Opt. Mater., 2017, 5, 1700227 CrossRef.
- J. M. Xiang, J. M. Zheng, Z. W. Zhou, H. Suo, X. Q. Zhao, X. J. Zhou, N. M. Zhang, M. S. Molokeev and C. F. Guo, Enhancement of red emission and site analysis in Eu2+ doped new-type structure Ba3CaK(PO4)3 for plant growth white LEDs, Chem. Eng. J., 2019, 356, 236–244 CrossRef CAS.
- H. J. Yu, J. Chen, R. Y. Mi, J. Y. Yang and Y. G. Liu, Broadband near-infrared emission of K3ScF6: Cr3+ phosphors for night vision imaging system sources, Chem. Eng. J., 2021, 417, 129271 CrossRef CAS.
- X. Zhou, J. M. Xiang, J. M. Zheng, X. Q. Zhao, H. Suo and C. F. Guo,
Ab initio two-sites occupancy and broadband near-infrared emission of Cr3+ in Li2MgZrO4, Mater. Chem. Front., 2021, 5, 4334–4342 RSC.
|
This journal is © the Partner Organisations 2022 |
Click here to see how this site uses Cookies. View our privacy policy here.