DOI:
10.1039/C6RA25502G
(Paper)
RSC Adv., 2017,
7, 1233-1240
N-Halamine modified thermoplastic polyurethane with rechargeable antimicrobial function for food contact surface†
Received
19th October 2016
, Accepted 13th November 2016
First published on 4th January 2017
Abstract
A polymer blend of two N-halamine precursors was prepared and homogeneously incorporated into TPU structure via a solvent casting method, and an N-halamine modified TPU film with rechargeable antimicrobial activity resulted after treating with chlorine bleach. Antimicrobial efficacies were evaluated against both Staphylococcus aureus (S. aureus) and Escherichia coli O157:H7 (E. coli). Results showed that the N-halamine modified TPU film caused 6
log
CFU reduction of bacteria reduction within 2 hours of contact. Moreover, the N-halamine modified TPU displayed desirable rechargeability and stability, which maintained sufficient antimicrobial activity after 20 cycles of “discharge–recharge” process and over 4 weeks of storage. Besides, the tensile strength and surface tension of TPU were not adversely affected by N-halamine modification. The N-halamine modified TPU with rechargeable antimicrobial function exhibited great potential as a cheap, safe and effective food contact surface material for preventing food microbial cross-contamination.
Introduction
Centers for Disease Control and Prevention (CDC) estimated that about 48 million people get sick, 128
000 are hospitalized and 3000 die of foodborne diseases annually in the United States.1 The majority of these foodborne illnesses were caused by consuming foods contaminated with harmful microorganisms such as fungi, bacteria, viruses, etc.2 Several outbreaks can be traced back to the microbiological cross-contamination from food contact surfaces during food preparation or processing.3 Therefore, in the food industry, cross-contamination has been controlled and managed predominately in many hazard analysis-based systems. Microorganisms can attach and survive on food contact surfaces due to either poor design of equipment or insufficient sanitation. Even worse, these pathogens can accumulate on the surfaces and form biofilms, which make it even more difficult to be remove them by established sanitation practices.4 Therefore, making contact surface materials with antimicrobial activity is of great importance to reduce microbial cross-contamination and so as to ensure food safety.
Currently, one of the most popular methods to produce antimicrobial food contact surfaces is through compounding small molecular antimicrobial additives such as triclosan and metal ions (copper, silver, etc.) into the carrier materials.5,6 However, since their antimicrobial effectiveness depend on migration of antimicrobial agents to the contact surface, there are limitations of this method, such as insufficient effectiveness, diminishing of antimicrobial agents with time, higher cost, safety concern, etc. Another potential method is through grafting antimicrobial molecules onto surface of carrier materials.7 However, this method is not suitable for food contact materials used on the surface of processing equipment. Because the heavy abrasion during food processing and sanitation practices will damage or even remove the grafted functional molecules, resulting in the removal of the antimicrobial function layer. Until now, there are very few antimicrobial surface materials that are suitable for food processing applications in the markets. Therefore, there is a continuing need to introduce novel antimicrobial technology to the food industry for solving microbial cross-contamination problems.
N-Halamine, as an emerging antimicrobial technology, has attracted a great deal of interest in recent years due to their superior antimicrobial efficacy against a broad spectrum of microorganisms, non-toxicity to human, good stability and low cost.7–9 N-Halamines are a group of compounds containing one or more nitrogen–halogen covalent bonds (N–Cl) that are normally formed by the halogenation of imide, amide or amine groups; and the antimicrobial activity of N-halamines was due to the oxidation state of halide atoms in chloramine or bromamine groups.10 One important feature makes N-halamines different from any other biocides is that their antimicrobial activity can be recovered after consuming through simply treating with chlorine bleach, which can be perfectly incorporated into current sanitation practices established in the food industry.11 All these features make N-halamines an attractive antimicrobial technology for food contact surface materials. A recent review by Bastarrachea et al. also highlighted the great potential of applying N-halamine polymers on equipment coating in food industry.7 Previously, intensive research focused on the application of N-halamine technology in textiles,12,13 biomedical materials,10,14 paints15 and water treatment materials16,17 by collaborators within our group. However, applications of these N-halamine compounds for direct food contact surfaces have seldom been explored.
Food contact surfaces can be made of various materials such as modular plastics, metals, fabrics and polyurethanes based on specific applications. In recent years, polyurethanes are attracting more and more interest in food processing industry especially for food conveyor belting.18 This is mainly because polyurethane belts are easy to clean, which can reduce both water consumption and sanitation cost. Therefore, TPU was selected as a representative material for food contact surfaces in this research. Previously, Julie M. Goddard's group worked intensively on applying N-halamines to food contact surfaces; however, they mainly focused on grafting method and on some other materials including polypropylene (PP),19,20 polyethylene (PE),21 stainless steel,22 etc. Although Yuyu Sun's team worked intensively on polyurethane, they also focused on grafting method and their research targeted to biomedical applications.9,23 Our group focused on synthesizing new N-halamine compounds and many new N-halamine polymers have been successfully developed and tested in the past few years.14,24–31 This allows us to select the suitable N-halamine polymers for food contact surface applications based on efficiency, safety and cost.
Recently, Liu et al. from our group synthesized two novel N-halamine polyelectrolytes that have shown strong antimicrobial efficacy.32 Compared with previous non-electrolyte N-halamine precursor polymers, these two N-halamine polyelectrolytes were synthesized from lower-cost acyclic monomers that were already commercially available in the market and the procedure was simple and clean. This will help to lower the cost and make it easier to pass future safety examinations for food contact surface applications. Also the combined polymer is not dissolved either in water or in many organic solvents, which it means the polymer blend is not easy to leak out after long time use in food processing environment. Moreover, compared with hydrophilic antimicrobial compounds, this polymer blend will also be not expected to lower the surface energy too much after compounding into hydrophobic carrier materials. All these properties are quite attractive for food contact surface applications. Therefore, we explored a new approach to apply these two new N-halamine precursor polymers in TPU elastomer aimed for food contact surface applications, and we addressed the rechargeable antimicrobial function of the new N-halamine modified TPU elastomer. This research is the first time to investigate the potential of N-halamine modified TPU as an effective antimicrobial surface material and highlighted the advantage of N-halamines to provide rechargeable antimicrobial function.
Experimental
1. Materials and instrumentation
Cationic monomer, (3-acrylamidopropyl) trimethylammonium chloride (APTMAC, 74–76 wt% in H2O), was purchased from Tokyo Chemical Industry Co., Ltd (Tokyo, Japan). Anionic monomer, 2-acrylamido-2-methylpropane sulfonic acid sodium salt solution (AMPSS, 50 wt% in H2O), was purchased from Sigma-Aldrich (St. Louis, MO). Sodium persulfate (Na2O8S2) was purchased from Acros Organics (Fair Lawn, NJ). Sodium thiosulfate (Na2O3S2) was purchased from Alfa Aesar (Heysham, LA3 2XY, England). Potassium iodide (KI) and isopropyl alcohol were purchased from Fisher Scientific (Fair Lawn, NJ). Tetrahydrofuran (THF) was purchased from EMD Millipore Corporation (Billerica, MA). Chlorine bleach was purchased from Walmart (Great Value™, Sodium hypochlorite: 8.25%). Polyether-based thermoplastic polyurethane pellets (TPU, IROGRAN® A 85 P 4394) was kindly supplied by Huntsman Corporation USA (Derry, NH). Nuclear Magnetic Resonance (NMR) spectra were collected using an AVANCE III 400 MHz Digital NMR spectrometer (Bruker AXS GmbH, Karlsruhe, Germany). NEXUS 470 spectrometer (Nicolet Instrument Corporation, Madison, WI) with an attenuated total reflectance (ATR) accessory was used to collect Fourier transform infrared (FTIR) data.
2. Preparation of N-halamine precursor polymers and their modified TPU elastomer
A cationic polyelectrolyte, poly[(3-acrylamidopropyl) trimethylammonium chloride] (pAPTMAC) and an anionic polyelectrolyte, poly(2-acrylamido-2-methylpropane sulfonic acid sodium salt) (pAMPSS), were synthesized by free radical polymerization method following the protocol of Liu et al.27 Briefly, for cationic polyelectrolyte (pAPTMAC), 27.56 g (0.1 mol) of (3-acrylamidopropyl)trimethylammonium chloride, 0.1 g of Na2O8S2 and 300 mL of DI water were stirred at 65 °C for 5 h under the protection of nitrogen gas in a one liter three-neck flask. A yellowish cationic homopolymer with a yield of 95% was resulted after separation, washing with isopropyl alcohol and drying in the vacuum overnight. For anionic polyelectrolyte (pAMPSS), 20.72 g (0.1 mol) of 2-acrylamido-2-methylpropane sulfonic acid sodium salt solution, 0.1 g of Na2O8S2 and 300 mL of DI water were reacted under the same polymerization conditions as mentioned above. A white homopolymer with a yield of 90% was obtained after separation, washing with 95% ethanol and drying in the vacuum overnight. The structures of two polymer products were confirmed by NMR characterization as indicated in the same literature27 and were shown in Fig. 1. The cationic polymer (pAPTMAC) has Mη = 6.6 × 104 Dalton and the anionic polymer (pAMPSS) has Mη = 7.4 × 104 Dalton. Then these two N-halamine precursor polymers were dissolved into deionized water to final concentration at 200 mg mL−1, and equal volume of these two polymer solutions were mixed together with stirring. A new precipitate polymer blend was obtained and recorded as N-halamine polymer precursor (NPH) in this study, and the blending mechanism was shown in Fig. 2. After that, this new polymer was dried completely in oven at 80 °C for about 6 h and grinded into fine powder (diameter ≤ 53 μm).
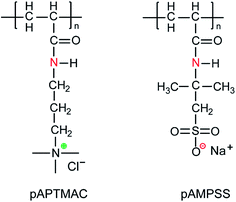 |
| Fig. 1 Structures of synthesized cationic (pAPTMAC) and anionic (pAMPSS) polyelectrolytes. | |
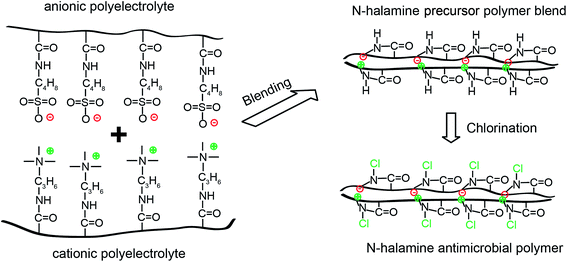 |
| Fig. 2 Preparation of N-halamine precursor polymer blend and formation of N-halamine structure through chlorination. | |
An N-halamine precursor polymer modified TPU film was prepared via a solution casting method following the protocol of Luo et al. with modification.9 Briefly, 4.5 g of pre-dried polyether-based TPU pellets were dissolved in 45 mL of THF at room temperature and stirred until homogeneous. Then pre-weighed polymer particles were added into the TPU THF solution with vigorous stirring to disperse the particles homogeneously. The solution was vacuumed to remove air bubbles, quickly poured into a glass mold (size: 6′′ × 12′′ × 0.5′′) and dried in a fume hood overnight. The film was uniform with a thickness around 0.30 mm and further cured in a vacuum oven at 45 °C for 24 h to remove any residual solvent. The obtained N-halamine precursor polymer modified TPU was recorded as TPU–NPH. Three different content of N-halamine precursor polymer modified TPU were prepared in this study (1%, 2% and 4%). TPU control film was prepared following the similar procedures except that no polymer particles was added into the TPU solution. The presence of N-halamine precursor polymers in the modified TPU was confirmed with FT-IR.
3. Chlorination and titration
The N-halamine precursor polymer modified TPU films (TPU–NPH) with different polymer contents (1%, 2% and 4%) were chlorinated by soaking in a 5% commercial aqueous bleach solution (1058 ± 102 ppm of available chlorine, pH = 7.0/HCl) for 1 h. After chlorination, the films were washed thoroughly with deionized water and dried in a 45 °C vacuum oven overnight to remove any free chlorine residuals. The chlorinated film containing N-halamine functional group (N–Cl) was recorded as TPU–NPCl. The original unmodified TPU film was treated with the same procedures to serve as chlorination treatment control and recorded as TPU–Cl. The presence of oxidative chlorines was confirmed with iodometric/thiosulfate titration as described in the following procedure. The chlorination conditions at 1% and 0.2% bleach, and treatment time of 1 h and 5 h were also investigated, respectively.
The oxidative chlorine contents of these TPU films were determined using an iodometric/thiosulfate titration method.27 Briefly, chlorinated TPU films were cut into the size of one square inch pieces (6.45 cm2). One piece of each film was put into a flask containing 20 mL of water, 1 mL of 0.1 N of acetic acid, and 0.25 g of KI, and stirred at room temperature for 1 h to form I2. Then 0.5% starch solution was added into the sample and titrated by 0.001 N of sodium thiosulfate aqueous solution from blue to colorless. The active chlorine contents in the chlorinated films were calculated using the following formula: [Cl+] (μg cm−2) = (N × V × 35.5)/2A, where N and V are the normality (equiv. L−1) and volume (L) of the titrant sodium thiosulfate and A is the total surface area of the chlorinated samples (cm2).
4. Antimicrobial efficacy test
The antimicrobial efficacy of N-halamine modified films was performed using a “sandwich” test method as described previously.27 A Gram negative E. coli O157:H7 (ATCC 43895) and a Gram positive S. aureus (ATCC 6538) were used as challenge bacteria in this study. Briefly, a single colony of each strain was transferred into 15 mL of trypticase soy broth (TSB, BD Co., MD) and incubated at 37 °C, 120 rpm for 16 h. The culture was washed twice with Butterfield's phosphate buffer (BPB) through centrifugation and re-suspended in the BPB buffer. Bacterial population was estimated by the spectrometer at O.D.640 nm and the inoculum with appropriated population was prepared. Then, an aliquot of 25 μL of the inoculum was added into the center of a square film, and a second identical film was placed on the top. A sterile weight was placed on the top to ensure complete contact with inoculated bacteria. At the contact time of 30 min, 1 h, and 2 h, the films were put into with Na2O3S2 solution (0.05 N) and vortexed to remove any oxidative chlorine residuals. Ten-fold serial dilutions were made for all samples and each dilution was plated on Trypticase agar (TSA) plates. The plates were incubated at 37 °C for 24 h and bacterial colonies were enumerated and recorded for antimicrobial efficacy analysis. All experiments were performed three times. Antimicrobial efficacy test was performed on unchlorinated (TPU) and chlorinated control films (TPU–Cl), unchlorinated (TPU–4% NPH) and chlorinated N-halamine precursor polymers modified TPU (TPU–1% NPCl, TPU–2% NPCl and TPU–4% NPCl).
5. Rechargeability and stability test
Based on the results from antimicrobial efficacy test, the 4% N-halamine modified TPU film (TPU–4% NPCl) was selected for the rechargeability test. To simulate the real application, the chlorinated film (TPU–4% NPCl) was immersed into 0.1 N of Na2O3S2 solution for 1 h to remove all the oxidative chlorines in the N–Cl structure of the film. This process was named “discharge”. Then some of these films were washed thoroughly with DI water and chlorinated with 5% bleach for 1 h to form the N–Cl structure. This process was named “recharge” and the film was recorded as R1. These “discharge–recharge” procedure was repeated for 5 cycles, 10 cycles and 20 cycles and they were recorded as R5, R10, and R20, respectively. The rechargeability were evaluated by both antimicrobial efficacy and total available chlorine content.
The TPU–4% NPCl films were selected for stability test under three different conditions of fluorescent light, dark, and water. For each condition, the TPU–4% NPCl films were cut into the size of one square inches (6.45 cm2) and stored in dark, under florescent light, or immersed in 100 mL of deionized water, respectively. For each condition, triplicate films were taken out and titrated for oxidative chlorine content at day 1, 3, 7, 14, 21 and 28. The chlorine content of immersion water was also titrated at each day. At day 28, all films were chlorinated with 5% bleach for 1 h, washed, and titrated to determine the oxidative chlorine content recovered from each group.
6. Physical properties test
The physical properties considered in this study are those related to the film performance and durability during use. On the other hand, it is to evaluate the deterioration of the film due to the additives and charging/recharging processing. These properties are strength and contact angle. Tensile strength was determined using an Instron® Universal Testing Machine Model 5565 (Instron®, Norwood, MA) at standard conditions of temperature and relative humidity of 21 °C ± 2 °C and 65% ± 5%, respectively. Rectangular specimens (76.2 mm × 25.4 mm) were stretched until breaking at a gauge length of 20 mm and a crosshead speed of 100 mm min−1. Tensile strength as the maximum load divided by the specimen's cross-sectional area was recorded in MPa. Nine independent measurements were performed for each type of samples.
The contact angle was measured using a ramé-hart Standard Contact Angle Goniometer Model 200 (ramé-hart, Inc. Mountain Lakes, NJ). This system consists of a microscope, camera, x–y–z moving stage, dropping mechanism and a computer equipped with DROPimage software. The water contact angle values were measured using the sessile drop method. The measurement error of this system is specified as ±2°. Three independent measurements were performed for each type of samples. Contact angle is a measure of the hydrophobicity of the film.
Results and discussion
1. Characterization of the N-halamine modified TPU
The synthesized N-halamine polymer precursors and their modified TPU elastomer were confirmed with optical observation and FT-IR. Compared with control TPU film, small particles were observed homogeneously dispersed in the TPU–4% NPH TPU film structure. FT-IR spectra of N-halamine polymer precursors (NP), unmodified TPU control film (TPU) and N-halamine precursor polymer modified TPU (TPU–4% NPH) were collected and included in the ESI.† All these results confirmed that the N-halamine precursor polymer blend was successfully incorporated into the TPU film. It should be highlighted that this N-halamine precursor polymer blend was not dissolved in either water or any tested organic solvents (THF, phenol, DMSO, petroleum ether, acetonitrile, benzene), which is ideal for food contact surface applications. In addition, water was the only solvent used through the whole procedure for preparing the polymer blend and sodium persulfate as water soluble catalyst was easily to be removed after synthesis procedure. This process is clean and simple, which means good potential for scale-up industrial production and also fulfills the trend of green chemistry.
2. Chlorination and chlorine content determination
The TPU film modified with different contents of N-halamine polymers and under different chlorination conditions were shown in Fig. 3(a). The control TPU film was determined to have 0.03 μg cm−2 oxidative chlorines on the surface. This is because polyurethane also has amine group (N–H), which can also be halogenated into N-halamine (N–Cl). However, usually the chlorine content in halogenated polyurethane was very low and not stable, which is in good agreement with the observation from Luo et al.9 After modified with 4% N-halamine polymers, the oxidative chlorine content increased dramatically to 2.7 μg cm−2. According to previous research, this concentration of oxidative chlorine was enough to make the surface with potent antimicrobial activities.27,33
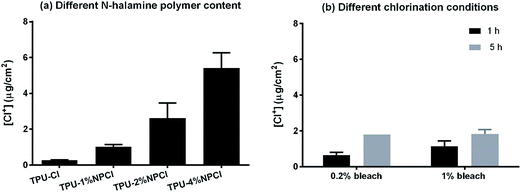 |
| Fig. 3 Titration of oxidative chlorine content in: (a) TPU films with different N-halamine polymer content (0%, 1%, 2% and 4%) treated with 5% bleach (pH = 7.0, 1058 ± 102 ppm of available chorine); (b) films with 4% N-halamine polymer content (TPU–4% NP) chlorinated with different concentrations of bleach (1% and 0.2% bleach) and treatment time (1 h and 5 h). | |
In this research, however, TPU film was observed not resistant to high concentration of bleach, which damage could be observed in the TPU structure after 20 rechlorination cycles (5% bleach for 1 h of each rechlorination cycle). Therefore, the film was also chlorinated using lower concentrations of bleach (1% and 0.2%). The oxidation chlorine contents were titrated at 278 ± 42 ppm [Cl+] (1% bleach) and 53 ± 0 ppm [Cl+] (0.2% bleach), respectively. As shown in Fig. 3(b), TPU–4% NPH could achieve enough oxidative chlorines (0.643 μg cm−2) on the surface even chlorinated with as low as 0.2% bleach (53 ± 0 ppm [Cl+]) for 1 h. After the time extended to 5 h, the oxidative chlorine content could reach at 1.79 μg cm−2. These two bleach concentrations (1% and 0.2%) were addressed in this research because they were of great importance to the food industry. According to federal regulations (21 CFR part 178), the 200 ppm of available chlorine is the maximum concentration that can be used for sanitizing food contact surfaces without further rinsing step. In the real application environment, even lower concentration, usually between 20 and 50 ppm, was preferred by industry because it can minimize the potential corrosive effects of bleach to contact materials including TPU and stainless steels.34 Based on the results, this N-halamine modified TPU film could be chlorinated to achieve enough antimicrobial activity even at very low concentration of bleach (0.2%, 50 ppm of [Cl+]) for using in food processing plant.
3. Antimicrobial efficacy
Antimicrobial efficacies of the N-halamine modified TPU were shown in Fig. 4. As shown, unchlorinated control film and unchlorinated TPU–4% NPH film did not display antimicrobial activity within 2 h of contact for either E. coli O157:H7 (Gram−) or S. aureus (Gram+). Although TPU control film showed slight increase of antimicrobial reduction after 2 h contact time for both bacteria, the efficacy was not satisfactory. For N-halamine modified TPU films, a dose–response phenomenon could be observed between NP content and antimicrobial efficacy in both of tested bacteria: higher NP content resulted in better antimicrobial efficacy. When the NP content increased to 4%, it had a total kill of the inoculum (about 106 CFU/6.45 cm2) for both bacteria within 2 h of contact.
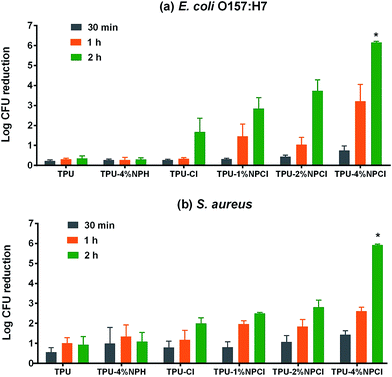 |
| Fig. 4 Antimicrobial efficacy of N-halamine modified TPU film against (a) E. coli O157:H7 (inoculum: 6.15 ± 0.06 log CFU per sample) and (b) S. aureus (inoculum: 5.84 ± 0.13 log CFU per sample). * indicates a total kill of all inoculated bacteria. | |
These results are in good accordance with previous research which N-halamines usually have very potent antimicrobial efficacy for killing of all inoculated bacteria (106 to 108 CFU/6.45 cm2) within 30 min or even shorter.23,27,32,35,36 However, TPU was very hydrophobic, which would prevent the sufficient contact of all bacteria with antimicrobial functional groups on the surface within a short period of time. Therefore, it needs longer time to have a 100% kill. For an ideal antimicrobial surface material, both high surface tension (hydrophobic) and strong biocidal activity are desired. In this way the overall ability to prevent microbial contamination will be optimized. This N-halamine modified TPU film has both hydrophobic and potent biocidal activity.
4. Rechargeability
The rechargeability was investigated using both titration and antimicrobial activity testing methods. As shown in Fig. 5, N-halamine modified TPU could still have a total kill of 6
log
CFU of inoculated E. coli O157:H7 within 2 h of contact after 20 cycles of “discharge–recharge” process. While the quenched film did not have any antimicrobial activity, which confirmed that the antimicrobial activity of N-halamine modified TPU film was due to the N-halamine functional groups (N–Cl). This rechargeability was also confirmed with chlorine content titration. After 20 cycles of “discharge-recharge” process, only a slight decrease of oxidative chlorine content was observed. The results indicated that this N-halamine modified TPU film has desirable rechargeability, which will create great potential for food contact surface applications. In this way, the contact surface will always keep high antimicrobial activity after treating with diluted chlorine bleach during sanitation.
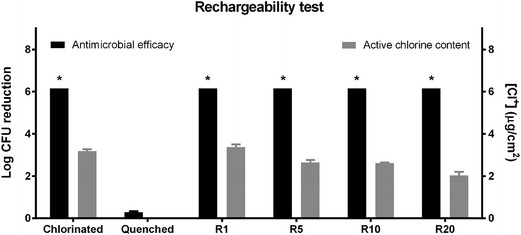 |
| Fig. 5 Antimicrobial efficacies and oxidative chlorine contents of rechargeable N-halamine modified TPU films. Inoculum: E. coli O157:H7 (6.15 log CFU/6.45 cm2). * indicates a total kill of all inoculated bacteria. | |
5. Stability
Generally, N-halamine functional group (N–Cl) was reported to be not very stable under light or in water environment,36 therefore the stability of antimicrobial functional groups of this N-halamine modified TPU was also investigated. As shown in Fig. 6, the N–Cl bond in the N-halamine modified films remained unchanged after storage in dark for 4 weeks. Under fluorescent light, it experienced a slight decrease after 3 weeks. This result was in good accordance with previous research performed by Liu et al. which these two N-halamine polyelectrolytes were quite stable under fluorescent light.27 However, the intensity of N-halamine functional group (N–Cl) decreased faster in the water environment: the oxidative chlorine content decreased to less than 1.0 μg cm−2 in the water after 28 days of storage. However the N–Cl bonds could almost be totally recovered after rechlorination at day 28 (28R). The other fact is that many food processing plant will do the sanitation every day, which means rechlorination (or “recharge” process) can be performed daily. Therefore, when combined with rechargeability result, this stability is far more enough to support its application as food antimicrobial surface material, which will always keep sufficient antimicrobial functional groups after regular treatment of diluted bleach during sanitation.
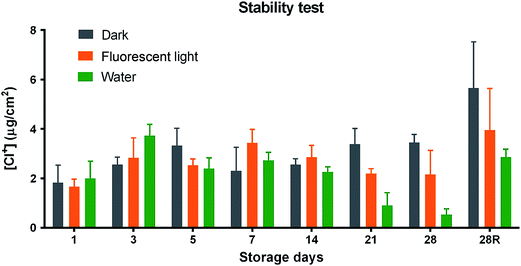 |
| Fig. 6 Oxidative chlorine contents of N-halamine modified TPUs stored in dark, under fluorescent light and in water for 28 days and rechlorinated after 28 days of storage (28R). | |
6. Physical properties
A successful antimicrobial functional modification should not sacrifice other important application functions of food contact materials. For example, tensile strength and hydrophobicity of conveyor belts should not be compromised too much to achieve the antimicrobial activity. Therefore, in this research, we also tested some physical properties of this N-halamine modified TPU at four different cases: TPU only (TPU-Ctrl) as a control, TPU charged with chlorine (TPU-Ctrl–Cl), N-halamine modified TPU (TPU–4% NP), and N-halamine modified TPU charged with chlorine (TPU–4% NP–Cl). As shown in Table 1, the tensile strength did not decrease evidently after mixing with 4% NPH polymers. Compared to the TPU only control film, N-halamine modified films lost around 10% of the tensile strength. After treatment/charging with chlorine (5% bleach), films lost around 20% of their strength. However, this loss is not considered a sever deterioration and the change in tensile strength would be expected to be further minimized through using low concentration of bleach (0.2%) in food processing application environment. For contact angle, there was a certain decrease in values observed for N-halamine modified TPU after chlorination. This could be explained by the compounding method applied in this research.
Table 1 Physical properties of N-halamine modified TPU films
Samples |
Thickness (mm) |
Tensile strength at maximum load (MPa) |
Contact angle (deg) |
TPU-Ctrl |
0.301 ± 0.033 |
20.9 ± 6.2 |
90.3 ± 1.0 |
TPU-Ctrl–Cl |
0.319 ± 0.055 |
14.3 ± 6.1 |
100.5 ± 3.3 |
TPU–4% NP |
0.311 ± 0.039 |
18.3 ± 3.7 |
89.9 ± 1.8 |
TPU–4% NP–Cl |
0.340 ± 0.045 |
14.5 ± 6.3 |
77.5 ± 1.2 |
For the purpose of research convenience, we chose the solvent casting method in this study. However this method also has drawbacks: the surface was not very smooth due to solvent evaporation and the particles were not distributed evenly in the film as those made in the industry through heat extrusion method. All these would contribute to lower number of contact angle as well as big variance of chorine content between different films. However, the surface tension test indicated this N-halamine modified TPU was still hydrophobic. The contact angle is also expected to be improved after better engineering method such as hot blending and extrusion method was applied in the future when a large quantity of production was desired. All these characteristics will be positive and accepted by industry for the application as food contact materials.
For these materials proposed for food contact surface applications, several criteria should be comprehensively considered and well balanced: the raw materials should be cheap and easily accessible; the synthesizing procedures need to be simple and clean, and less toxic processing aid agents should be used; the effectiveness and durability should be good enough. This N-halamine modified polyurethane can adequately fulfill all the criteria mentioned above. It should be remarked here that only water was used during the whole synthesis procedures of N-halamine precursor polymers, which means it is safe and green. This will also contribute to making it easier for passing the regulatory examination for applying on the food contact surfaces in the future. Most importantly, the antimicrobial activity can be recharged, which can fulfill both effectiveness and durability requirements of antimicrobial material as desired by the food industry.
Conclusions
In this study, a simple, clean and efficient method to modify thermoplastic polyurethane (TPU) with rechargeable antimicrobial function is reported. This N-halamine modified TPU film caused 6
log bacterial reduction within 2 hours of contact. The antimicrobial N-halamine functional groups were also stable enough for the application. Moreover, the N-halamine modified TPU displayed desirable rechargeability, which maintained sufficient antimicrobial activity after 20 cycles of “discharge–recharge” process. Therefore, this new N-halamine modified TPU has a great potential to be used as food contact surface material with rechargeable antimicrobial function. Ultimately, antimicrobial functionalized food contact surface will help to prevent microbial cross-contamination and biofilm formation during food processing and improve food safety.
References
- S. Elaine, M. H. Robert, J. A. Frederick, V. T. Robert, W. Marc-Alain, L. R. Sharon, L. J. Jeffery and M. G. Patricia, Emerging Infectious Disease journal, 2011, 17, 7 CrossRef PubMed.
- Centers for Disease Control and Prevention, Morbidity and Mortality Weekly Report, 2007, vol. 59, p. 6 Search PubMed.
- K. A. Jackson, M. Biggerstaff, M. Tobin-D'Angelo, D. Sweat, R. Klos, J. Nosari, O. Garrison, E. Boothe, L. Saathoff-Huber, L. Hainstock and R. P. Fagan, J. Food Prot., 2011, 74, 949–953 CrossRef CAS PubMed.
- R. A. N. Chmielewski and J. F. Frank, Compr. Rev. Food Sci. Food Saf., 2003, 2, 22–32 CrossRef CAS.
- D. Chung, S. E. Papadakis and K. L. Yam, Int. J. Food Sci. Technol., 2003, 38, 165–169 CrossRef CAS.
- H. J. Lee, S. G. Lee, E. J. Oh, H. Y. Chung, S. I. Han, E. J. Kim, S. Y. Seo, H. Do Ghim, J. H. Yeum and J. H. Choi, Colloids Surf., B, 2011, 88, 505–511 CrossRef CAS PubMed.
- L. J. Bastarrachea, A. Denis-Rohr and J. M. Goddard, Annu. Rev. Food Sci. Technol., 2015, 6, 97–118 CrossRef CAS PubMed.
- E.-R. Kenawy, S. D. Worley and R. Broughton, Biomacromolecules, 2007, 8, 1359–1384 CrossRef CAS PubMed.
- J. Luo, N. Porteous and Y. Sun, ACS Appl. Mater. Interfaces, 2011, 3, 2895–2903 CAS.
- B. Demir, I. Cerkez, S. D. Worley, R. M. Broughton and T.-S. Huang, ACS Appl. Mater. Interfaces, 2015, 7, 1752–1757 CAS.
- H. B. Kocer, I. Cerkez, S. D. Worley, R. M. Broughton and T. S. Huang, ACS Appl. Mater. Interfaces, 2011, 3, 2845–2850 CAS.
- L. Lin, M. Kaikai, L. Yin, L. Ying, L. Rong, R. Xuehong and H. Tung-Shi, Journal of Engineered Fabrics & Fibers, 2016, 11, 23–30 Search PubMed.
- X. Cheng, R. Li, J. Du, J. Sheng, K. Ma, X. Ren and T.-S. Huang, Polym. Adv. Technol., 2015, 26, 99–103 CrossRef CAS.
- X. Ren, L. Kou, H. B. Kocer, S. D. Worley, R. M. Broughton, Y. M. Tzou and T. S. Huang, J. Biomed. Mater. Res., Part B, 2009, 89, 475–480 CrossRef PubMed.
- H. B. Kocer, I. Cerkez, S. D. Worley, R. M. Broughton and T. S. Huang, ACS Appl. Mater. Interfaces, 2011, 3, 3189–3194 CAS.
- Z. Jiang, B. Demir, R. M. Broughton, X. Ren, T. S. Huang and S. D. Worley, J. Appl. Polym. Sci., 2016, 133 DOI:10.1002/app.43413.
- S. D. Worley, D. E. Williams and R. A. Crawford, Crit. Rev. Environ. Control, 1988, 18, 133–175 CrossRef CAS.
- N. Chaitiemwong, W. C. Hazeleger and R. R. Beumer, Int. J. Food Microbiol., 2010, 142, 260–263 CrossRef CAS PubMed.
- A. Denis-Rohr, L. J. Bastarrachea and J. M. Goddard, Food Bioprod. Process., 2015, 96, 12–19 CrossRef CAS.
- L. J. Bastarrachea and J. M. Goddard, J. Agric. Food Chem., 2015, 63, 4243–4251 CrossRef CAS PubMed.
- L. J. Bastarrachea, M. Peleg, L. A. McLandsborough and J. M. Goddard, J. Food Eng., 2013, 117, 52–58 CrossRef CAS.
- L. J. Bastarrachea and J. M. Goddard, J. Appl. Polym. Sci., 2013, 127, 821–831 CrossRef CAS.
- Y. Liu, K. Ma, R. Li, X. Ren and T. S. Huang, Cellulose, 2013, 20, 3123–3130 CrossRef CAS.
- I. Cerkez, H. B. Kocer, S. D. Worley, R. M. Broughton and T. S. Huang, React. Funct. Polym., 2012, 72, 673–679 CrossRef CAS.
- H. B. Kocer, S. D. Worley, R. M. Broughton and T. S. Huang, React. Funct. Polym., 2011, 71, 561–568 CrossRef CAS.
- X. Ren, C. Zhu, L. Kou, S. D. Worley, H. B. Kocer, R. M. Broughton and T. S. Huang, J. Bioact. Compat. Polym., 2010, 25, 392–405 CrossRef CAS.
- Y. Liu, J. Li, X. Cheng, X. Ren and T. Huang, J. Mater. Chem. B, 2015, 3, 1446–1454 RSC.
- M. Yin, X. Chen, R. Li, D. Huang, X. Fan, X. Ren and T.-S. Huang, J. Appl. Polym. Sci., 2016 DOI:10.1002/app.44204.
- X. Fan, X. Ren, T.-S. Huang and Y. Sun, RSC Adv., 2016, 6, 42600–42610 RSC.
- X. Li, Y. Liu, Z. Jiang, R. Li, X. Ren and T. S. Huang, Cellulose, 2015, 22, 3609–3617 CrossRef CAS.
- K. Ma, Y. Liu, Z. Xie, R. Li, Z. Jiang, X. Ren and T.-S. Huang, Ind. Eng. Chem. Res., 2013, 52, 7413–7418 CrossRef CAS.
- Z. Jiang, K. Ma, J. Du, R. Li, X. Ren and T. S. Huang, Appl. Surf. Sci., 2014, 288, 518–523 CrossRef CAS.
- J. Luo, N. Porteous, J. Lin and Y. Sun, J. Bioact. Compat. Polym., 2015, 30, 157–166 CrossRef CAS PubMed.
- M. H. Moayed and M. Golestanipour, Mater. Corros., 2005, 56, 39–43 CrossRef CAS.
- I. Cerkez, S. D. Worley, R. M. Broughton and T. S. Huang, React. Funct. Polym., 2013, 73, 1412–1419 CrossRef CAS.
- F. Hui and C. Debiemme-Chouvy, Biomacromolecules, 2013, 14, 585–601 CrossRef CAS PubMed.
Footnote |
† Electronic supplementary information (ESI) available. See DOI: 10.1039/c6ra25502g |
|
This journal is © The Royal Society of Chemistry 2017 |
Click here to see how this site uses Cookies. View our privacy policy here.