DOI:
10.1039/C5QO00151J
(Research Article)
Org. Chem. Front., 2015,
2, 956-960
Catalytic enantioselective synthesis of quaternary 3,3′-indolyloxindoles by combination of Rh(II) complexes and chiral phosphines†
Received
7th May 2015
, Accepted 2nd June 2015
First published on 3rd June 2015
Abstract
Rh(II)/chiral phosphine combined catalysis has been developed for a highly efficient sequential C–H functionalization/asymmetric allylation or Michael addition reaction of indoles and 3-diazooxindoles with allenoates or vinyl ketones to afford a diverse range of quaternary 3,3′-indolyloxindole derivatives. Moreover, a significantly positive effect of LiCl in the sequential C–H functionalization/allylation reaction was observed, which led to an unusual ternary activation mechanism in the allylation step.
Introduction
The optically active 3,3′-bisindole represents a unique structural element in the fascinating family of indole alkaloids exemplified in Fig. 1, which have been characterized by a wide spectrum of antimicrobial, antiviral and antitumor bioactivities.1 All-carbon quaternary stereogenic center-linking patterns (QCPs) of the bisindole unit are potential sources of structural diversity that attracts great interest for its chemical synthesis, especially in a catalytic enantioselective fashion. Recent reports have described a number of intra-2 or intermolecular3–5 methodologies, and the latter chiefly centered on asymmetric substitution reactions of racemic 3,3′-disubstituted oxindoles3 and asymmetric addition reactions of 3-indolyloxindoles to electrophiles.4 Despite the great contributions that these existing methods have made in building up bisindole derivatives and total synthesis of natural alkaloids, the methods to access QCPs are still rather limited and hence novel strategies are extremely desired to circumvent daunting challenges in the highly straightforward and stereoselective construction of all-carbon quaternary stereogenic centers of this type.6
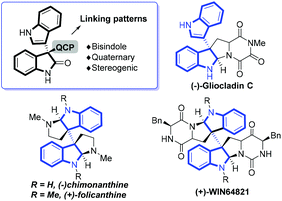 |
| Fig. 1 Natural bisindole alkaloids. | |
Nucleophilicity-featured chiral phosphines7 have recently been at the center of attention in popular asymmetric organocatalysis arising from pioneering contributions from Lu8 and driven by important findings coming from Vedejs,9 Zhang,10 Fu,11 Shi12 and others.13–17 Simple α-amino acid- or dipeptide-based bifunctional chiral phosphines, first discovered by Miller14 and Jacobsen,15 advanced by Zhao16 and Lu,17 have recently proven to be extremely effective in the promotion of a number of enantioselective transformations. For example, Lu's17g and Zhao's16c groups respectively reported asymmetric γ-additions of oxindoles or benzofuran to allenoates, providing direct access to highly enantioenriched quaternary 3,3′-indolyloxindoles or benzofuran (Scheme 1). In the last decade, asymmetric metal/organo-combined catalysis has been a valuable and versatile concept to enable the abundant creation of new transformations.4d,18–20 However, to the best of our knowledge, chiral phosphines have rarely been in this category with the exception of a few non-enantioselective examples described by Krische and Wu.21 We believe that the combination of transition metals and chiral phosphines would be able to provide more opportunities to enrich the synthetic value than either type of individual catalysts. Herein, we will demonstrate our efforts directed towards developing a novel Rh(II)/chiral phosphine sequential catalysis for the efficient synthesis of enantioenriched 3,3′-indolyloxindoles with unprecedented QCPs (Scheme 2).
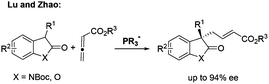 |
| Scheme 1 Phosphine mediated asymmetric γ-additions. | |
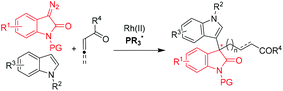 |
| Scheme 2 Rh(II)/chiral phosphine combined catalysis. | |
Results and discussion
We first investigated different conditions for a three-component reaction of 3-diazooxindole 1a, N-methyl indole 2a and allenoate 3 under the catalysis of 2 mol% of transition metal complexes and 10 mol% of organocatalysts, in CH2Cl2, at 25 °C (Table 1, entries 1–8). Since the Ru(II)/chiral squaramidebinary catalyst system previously developed4d failed to afford the desired three-component transformation, instead only a C–H functionalization product was observed (see Fig. S1 in the ESI† for details), we turned to use more nucleophilic chiral phosphines, in combination with transition metal complexes to catalyze the reaction. In the presence of 2 mol% [RuCl2(p-cymene)]2, the L-Val derived bifunctional chiral phosphine 4a successfully enabled a sequential C–H functionalization/allylation reaction to give 5a, but in a low yield with a moderate 32% ee (entry 1). The use of rhodium complexes as metal catalysts did not exert an impact on the enantioselectivity while leading to obviously higher yields (entries 2 and 3). More interestingly, the Rh2(esp)2 showed good compatibility with the chiral phosphine organocatalyst to allow the sequential reaction to give a good yield (entry 3). Then, a series of bifunctional chiral phosphines 4b–4f were evaluated, and it was found that the original α-amino acid sources and hydrogen-bond donor moieties were both important for stereocontrol (entries 4–8). In particular, the combination of Rh2(esp)2 and L-Phe derived thiourea phosphine 4d enabled the reaction to give the best results of 50% yield and 77% ee (entry 6). Notably, the reaction in CHCl3 displayed 87% ee (entry 9 and see Table S1 in the ESI† for more details). Surprisingly, even better results were obtained by lowering the loading of Rh2(esp)2 to 0.02 mol% probably due to more amounts of chiral phosphine catalyst 4d liberated from the coordination to rhodium (entry 10). The examination of additives (also see Table S1 in the ESI† for details) identified that 1.0 equivalent of LiCl continued to improve both the yield and enantioselectivity (entry 11, 81% yield and 91% ee). However, lowering the reaction temperature (0 °C) led to significantly eroded yield, albeit with a maintained enantiomeric excess (entry 12).
Table 1 Optimization of reaction conditionsa
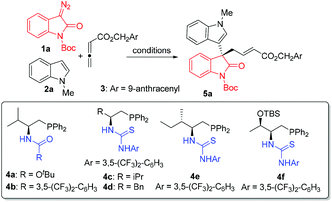
|
Entry |
[M] |
4
|
Solvent |
Yieldb (%) |
eec (%) |
Unless indicated otherwise, reactions of 1a (0.10 mmol), 2a (0.10 mmol), 3 (0.11 mmol), metal (0.002 mmol), and 4 (0.010 mmol) were carried out in a solvent (1.0 mL), at 25 °C, for 12 h.
Isolated yield.
Determined by HPLC.
0.02 mol% of Rh2(esp)2 was used.
1.0 equivalent of LiCl was used as an additive.
The reaction was conducted at 0 °C. [Ru] = [RuCl2(p-cymene)]2.
|
1 |
[Ru] |
4a
|
CH2Cl2 |
10 |
32 |
2 |
Rh2(OAc)4 |
4a
|
CH2Cl2 |
21 |
32 |
3 |
Rh2(esp)2 |
4a
|
CH2Cl2 |
53 |
32 |
4 |
Rh2(esp)2 |
4b
|
CH2Cl2 |
81 |
16 |
5 |
Rh2(esp)2 |
4c
|
CH2Cl2 |
60 |
58 |
6 |
Rh2(esp)2 |
4d
|
CH2Cl2 |
50 |
77 |
7 |
Rh2(esp)2 |
4e
|
CH2Cl2 |
55 |
58 |
8 |
Rh2(esp)2 |
4f
|
CH2Cl2 |
39 |
46 |
9 |
Rh2(esp)2 |
4d
|
CHCl3 |
52 |
87 |
10d |
Rh2(esp)2 |
4d
|
CHCl3 |
60 |
87 |
11d,e |
Rh
2
(esp)
2
|
4d
|
CHCl
3
|
81
|
91
|
12d,e,f |
Rh2(esp)2 |
4d
|
CHCl3 |
26 |
91 |
With the optimized reaction conditions in hand, we then examined the substrate scope. In general, this Rh(II)/4d combined catalytic system tolerated a wide range of substituents on either the indole or 3-diazooxindole moiety, affording excellent yields and enantioselectivities in most cases (Table 2). The electronic nature of substituents was crucial for the formation of final products by accelerating or slowing down the first C–H functionalization step. For example, the substrates with electron–neutral or electron-donating substituents smoothly underwent a one-pot reaction in the presence of 0.01 mol% of Rh2(esp)2 (e.g., entries 2–4, 6, and 8–10), while the reaction of those with electron-withdrawing substituents required 0.05 mol% of Rh2(esp)2 to ensure the generation of the desired products in good yields (entries 7 and 12). The substituent on either the C6- or C7-position of 3-diazooxindoles 1 had a negative effect on the stereocontrol regardless of the electronic feature (entries 10–12). By converting 5a and a known compound into the same derivative, the absolute configuration of 5 was determined to be S (see Scheme S1 in the ESI† for details).
Table 2 Substrates scope of sequential C–H functionalization/allylation reactiona
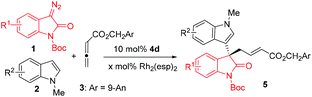
|
Entry |
R1/R2 |
x
|
5
|
Yieldb (%) |
eec (%) |
Unless indicated otherwise, reactions of 3-diazooxindoles 1 (0.10 mmol), 2 (0.10 mmol), and 3 (0.11 mmol) with 4d (0.010 mmol) and x mmol% Rh2(esp)2 were carried out in CHCl3 (1.0 mL), at 25 °C, for 12 h.
Isolated yield.
Determined by HPLC.
20 mol% of 4d was used.
|
1 |
H/5-F |
0.02 |
5b
|
69 |
87 |
2 |
H/5-Me |
0.01 |
5c
|
80 |
90 |
3 |
H/5-OMe |
0.01 |
5d
|
83 |
92 |
4 |
H/6-OMe |
0.01 |
5e
|
81 |
90 |
5 |
H/6-Cl |
0.02 |
5f
|
72 |
90 |
6 |
H/7-Me |
0.01 |
5g
|
64 |
87 |
7d |
5-Br/H |
0.05 |
5h
|
64 |
92 |
8 |
5-Me/H |
0.01 |
5i
|
80 |
86 |
9 |
5-OMe/H |
0.01 |
5j
|
66 |
88 |
10 |
6-OMe/H |
0.01 |
5k
|
77 |
86 |
11 |
6-Br/H |
0.02 |
5l
|
66 |
85 |
12 |
7-Br/H |
0.05 |
5m
|
61 |
72 |
Other electrophiles such as α,β-unsaturated carbonyls could also be adopted in the C–H functionalization/asymmetric Michael addition17f sequentially catalyzed by Rh2(esp)2 and the chiral phosphine 4g (see Fig. S2 in the ESI† for the detailed evaluation of chiral phosphines). Under the optimal conditions (see Table S2 in the ESI† for details), high yields and excellent enantioselectivities were generally provided (Table 3). Non-protected indoles were also permitted to undergo a clean reaction, however with a much diminished enantioselectivity of 56% ee (entry 2), presumably due to the fact that the H-bonding interaction between the catalyst and the substrate was disrupted.17i Again, the presence of electron-donating substituents at the indole moiety gave higher enantioselectivity than those with an electron-deficient substituent (entries 3–8). Moreover, ethyl- and phenyl vinyl ketones underwent a clean reaction to generate 7i and 7j in high yields with excellent or good levels of stereocontrol. By converting 7b and a known compound into a similar derivative, the absolute configuration of 7 was determined to be S (see Scheme S2 in the ESI† for details).
Table 3 Rh(II)/chiral phosphine sequentially catalytic C–H functionalization/Michael addition reactiona
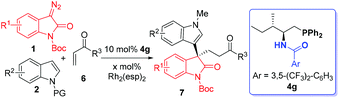
|
Entry |
R1/R2/R3 |
x
|
7
|
Yieldb (%) |
eec (%) |
Unless indicated otherwise, reactions of 3-diazooxindoles 1 (0.10 mmol), 2 (0.10 mmol, PG = Me), and 6 (0.20 mmol) with 4g (0.010 mmol) and x mmol% Rh2(esp)2 were carried out in CHCl3 (1.0 mL), at −60 °C, for 12 h.
Isolated yield.
Determined by HPLC.
PG = H.
10 mol% of 4c was used instead of 4g.
|
1 |
H/H/Me |
0.02 |
7a
|
92 |
91 |
2d |
H/H/Me |
0.02 |
7b
|
82 |
56 |
3 |
H/5-OMe/Me |
0.01 |
7c
|
75 |
92 |
4 |
H/6-OMe/Me |
0.01 |
7d
|
88 |
92 |
5 |
H/6-Cl/Me |
0.02 |
7e
|
88 |
87 |
6 |
H/7-Me/Me |
0.01 |
7f
|
61 |
89 |
7 |
5-Br/H/Me |
0.05 |
7g
|
87 |
92 |
8 |
6-OMe/H/Me |
0.01 |
7h
|
77 |
90 |
9 |
H/H/Et |
0.02 |
7i
|
86 |
90 |
10e |
H/H/Ph |
0.02 |
7j
|
94 |
77 |
Products obtained from these Rh(II)/chiral phosphine sequentially catalytic protocols could be transformed into a structurally more complicated spirocyclic 3,3′-indolyloxindole through classical procedures. For example, in the presence of excess amounts of BF3·Et2O, the Boc group of 7a was first removed to give A, which then underwent an intramolecular Friedel–Crafts/dehydration process to generate a spirocycle 8 (Scheme 3).
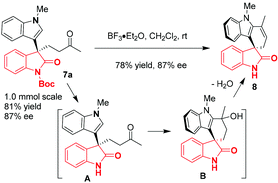 |
| Scheme 3 Synthesis of a spirocycle from 7a. | |
According to previous findings,4d the one-pot reactions might principally encompass two catalytic cycles (Fig. 2), including an insertion reaction of rhodium carbene species to the C–H bond22 to generate a nucleophilic intermediate 9. In the sequential C–H functionalization/allylation reaction, a lithium-stabilized phosphonium enolate 10 would be generated, as suggested by previous LiCl-promoted nucleophilic catalysis,23 which worked as a stronger base to facilitate the enolization of 9, thereby leading to an improved yield (entry 10 vs. 11, Table 1). More importantly, LiCl, in combination with the bifunctional phosphine, constitutes a ternary synergistic activation system (that is, Lewis acid, hydrogen bonds and phosphine synergistic activation), as shown in the transition state TS-I, wherein the oxindole lithium enolate interacted with a chiral thiourea–chloride complex through Li–Cl coordination, as indicated by Jacobsen hypothesis,24 to deliver relatively higher levels of stereocontrol than in the absence of LiCl (entry 10 vs. 11, Table 1). In contrast, the sequential process of C–H functionalization and Michael addition also involves the C–H insertion reaction to generate 9 while the formation of products 7 from 9 is considered to follow the general mechanism reported in the literature.17f
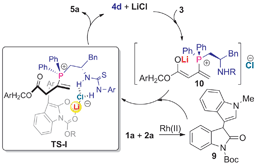 |
| Fig. 2 Proposed mechanism for the Rh(II)/chiral phosphine sequentially catalytic C–H functionalization/asymmetric allylation reaction. | |
Conclusions
We have developed a combined catalyst system comprised of Rh(II) complexes and amino acid-derived chiral phosphines, enabling highly enantioselective sequential C–H functionalization/asymmetric addition reactions of indoles and 3-diazooxindoles with allenoates or vinyl ketones. These reactions afforded enantioenriched 3,3′-indolyloxindoles with unprecedented quaternary stereogenic center-linking patterns, which allowed further transformations into more complex molecules. Moreover, the positive effect of LiCl led to an unusual possibility that the lithium enolate might be able to form a chiral thiourea–chloride complex through Li–Cl coordination to assist the stereochemical control.
Acknowledgements
We are grateful for financial support from NSFC (21232007) and the Ministry of Education.
Notes and references
- A. Steven and L. E. Overman, Angew. Chem., Int. Ed., 2007, 46, 5488 CrossRef CAS PubMed.
-
(a) J. E. DeLorbe, S. Y. Jabri, S. M. Mennen, L. E. Overman and F.-L. Zhang, J. Am. Chem. Soc., 2011, 133, 6549 CrossRef CAS PubMed;
(b) B. M. Trost and M. Osipov, Angew. Chem., Int. Ed., 2013, 52, 9176 CrossRef CAS PubMed.
- For selected examples, see:
(a) C. Guo, J. Song, J.-Z. Huang, P.-H. Chen, S.-W. Luo and L.-Z. Gong, Angew. Chem., Int. Ed., 2012, 51, 1046 CrossRef CAS PubMed;
(b) J.-Z. Huang, X. Wu and L.-Z. Gong, Adv. Synth. Catal., 2013, 355, 2531 CrossRef CAS PubMed;
(c) J. Song, C. Guo, A. Adele, H. Yin and L.-Z. Gong, Chem. – Eur. J., 2013, 19, 3319 CrossRef CAS PubMed;
(d) W. Dai, H. Lu, X. Li, F. Shi and S.-J. Tu, Chem. – Eur. J., 2014, 20, 11382 CrossRef CAS PubMed;
(e) F. Shi, R.-Y. Zhu, W. Dai, C.-S. Wang and S.-J. Tu, Chem. – Eur. J., 2014, 20, 2597 CrossRef CAS PubMed;
(f) F. Shi, H.-H. Zhang, X.-X. Sun, J. Liang, T. Fan and S.-J. Tu, Chem. – Eur. J., 2015, 21, 3465 CrossRef CAS PubMed.
-
(a) B. M. Trost, J. Xie and J. D. Sieber, J. Am. Chem. Soc., 2011, 133, 20611 CrossRef CAS PubMed;
(b) H. Mitsunuma, M. Shibasaki, M. Kanai and S. Matsunaga, Angew. Chem., Int. Ed., 2012, 51, 5217 CrossRef CAS PubMed;
(c) B. M. Trost, J. T. Masters and A. C. Burns, Angew. Chem., Int. Ed., 2013, 52, 2260 CrossRef CAS PubMed;
(d) D.-F. Chen, F. Zhao, Y. Hu and L.-Z. Gong, Angew. Chem., Int. Ed., 2014, 53, 10763 CrossRef CAS PubMed.
- For asymmetric coupling reactions between two indole subunits, see:
(a) T. Arai, Y. Yamamoto, A. Awata, K. Kamiya, M. Ishibashi and M. A. Arai, Angew. Chem., Int. Ed., 2013, 52, 2486 CrossRef CAS PubMed;
(b) R. Liu and J. Zhang, Org. Lett., 2013, 15, 2266 CrossRef CAS PubMed;
(c) H. Zhang, L. Hong, H. Kang and R. Wang, J. Am. Chem. Soc., 2013, 135, 14098 CrossRef CAS PubMed.
-
(a) C. J. Douglas and L. E. Overman, Proc. Nat. Acad. Sci. U. S. A., 2004, 101, 5363 CrossRef CAS PubMed;
(b) K. W. Quasdorf and L. E. Overman, Nature, 2014, 516, 181 CrossRef CAS PubMed.
- For selected reviews, see:
(a) L.-W. Ye, J. Zhou and Y. Tang, Chem. Soc. Rev., 2008, 37, 1140 RSC;
(b) S. X. Wang, X. Y. Han, F. R. Zhong, Y. Q. Wang and Y. X. Lu, Synlett, 2011, 2766 CAS;
(c) Z. Chai and G. Zhao, Catal. Sci. Technol., 2012, 2, 29 RSC;
(d) Y. Wei and M. Shi, Chem. – Asian J., 2014, 9, 2720 CrossRef CAS PubMed.
- For a review, see:
(a) X.-Y. Lu, C.-M. Zhang and Z.-R. Xu, Acc. Chem. Res., 2001, 34, 535 CrossRef CAS PubMed; For the pioneering work, see:
(b) C.-M. Zhang and X.-Y. Lu, J. Org. Chem., 1995, 60, 2906 CrossRef CAS.
- E. Vedejs, O. Daugulis and S. T. Diver, J. Org. Chem., 1996, 61, 430 CrossRef CAS.
- G. Zhu, Z. Chen, Q. Jiang, D. Xiao, P. Cao and X. Zhang, J. Am. Chem. Soc., 1997, 119, 3836 CrossRef CAS.
-
(a) R. P. Wurz and G. C. Fu, J. Am. Chem. Soc., 2005, 127, 12234 CrossRef CAS PubMed;
(b) J. E. Wilson and G. C. Fu, Angew. Chem., Int. Ed., 2006, 45, 1426 CrossRef CAS PubMed;
(c) Y. K. Chung and G. C. Fu, Angew. Chem., Int. Ed., 2009, 48, 2225 CrossRef CAS PubMed;
(d) S. W. Smith and G. C. Fu, J. Am. Chem. Soc., 2009, 131, 14231 CrossRef CAS PubMed;
(e) Y. Fujiwara and G. C. Fu, J. Am. Chem. Soc., 2011, 133, 12293 CrossRef CAS PubMed;
(f) D. T. Ziegler, L. Riesgo, T. Ikeda, Y. Fujiwara and G. C. Fu, Angew. Chem., Int. Ed., 2014, 53, 13183 CrossRef CAS PubMed.
- For a review, see:
(a) Y. Wei and M. Shi, Acc. Chem. Res., 2010, 43, 1005 CrossRef CAS PubMed; For excellent examples, see:
(b) M. Shi and L.-H. Chen, Chem. Commun., 2003, 1310 RSC;
(c) M. Shi, L.-H. Chen and C.-Q. Li, J. Am. Chem. Soc., 2005, 127, 3790 CrossRef CAS PubMed;
(d) Y.-Q. Jiang, Y.-L. Shi and M. Shi, J. Am. Chem. Soc., 2008, 130, 7202 CrossRef CAS PubMed.
- For selected examples, see:
(a) A. Voituriez, A. Panossian, N. Fleury-Brégeot, P. Retailleau and A. Marinetti, J. Am. Chem. Soc., 2008, 130, 14030 CrossRef CAS PubMed;
(b) M. Sampath and T.-P. Loh, Chem. Sci., 2010, 1, 739 RSC;
(c) Z. Shi, P. Yu, T.-P. Loh and G. Zhong, Angew. Chem., Int. Ed., 2012, 51, 7825 CrossRef CAS PubMed;
(d) C. E. Henry, Q. Xu, Y. C. Fan, T. J. Martin, L. Belding, T. Dudding and O. Kwon, J. Am. Chem. Soc., 2014, 136, 11890 CrossRef CAS PubMed.
- B. J. Cowen and S. J. Miller, J. Am. Chem. Soc., 2007, 129, 10988 CrossRef CAS PubMed.
-
(a) Y.-Q. Fang and E. N. Jacobsen, J. Am. Chem. Soc., 2008, 130, 5660 CrossRef CAS PubMed;
(b) Y. Q. Fang, P. M. Tadross and E. N. Jacobsen, J. Am. Chem. Soc., 2014, 136, 17966 CrossRef CAS PubMed.
-
(a) H. Xiao, Z. Chai, C.-W. Zheng, Y.-Q. Yang, W. Liu, J.-K. Zhang and G. Zhao, Angew. Chem., Int. Ed., 2010, 49, 4467 CrossRef CAS PubMed;
(b) H. Xiao, Z. Chai, H.-F. Wang, X.-W. Wang, D.-D. Cao, W. Liu, Y.-P. Lu, Y.-Q. Yang and G. Zhao, Chem. – Eur. J., 2011, 17, 10562 CrossRef CAS PubMed;
(c) J. Chen, Y. Cai and G. Zhao, Adv. Synth. Catal., 2014, 356, 359 CrossRef CAS PubMed;
(d) H.-Y. Wang, K. Zhang, C.-W. Zheng, Z. Chai, D.-D. Cao, J.-X. Zhang and G. Zhao, Angew. Chem., Int. Ed., 2015, 54, 1775 CrossRef CAS PubMed;
(e) Y. P. Lou, C. W. Zheng, R. M. Pan, Q. W. Jin, G. Zhao and Z. Li, Org. Lett., 2015, 17, 688 CrossRef CAS PubMed.
-
(a) X. Han, Y. Wang, F. Zhong and Y. Lu, J. Am. Chem. Soc., 2011, 133, 1726 CrossRef CAS PubMed;
(b) F. Zhong, X. Han, Y. Wang and Y. Lu, Angew. Chem., Int. Ed., 2011, 50, 7837 CrossRef CAS PubMed;
(c) F. Zhong, Y. Wang, X. Han, K.-W. Huang and Y. Lu, Org. Lett., 2011, 13, 1310 CrossRef CAS PubMed;
(d) F. Zhong, X. Han, Y. Wang and Y. Lu, Chem. Sci., 2012, 3, 1231 RSC;
(e) X. Han, F. Zhong, Y. Wang and Y. Lu, Angew. Chem., Int. Ed., 2012, 51, 767 CrossRef CAS PubMed;
(f) F. Zhong, X. Dou, X. Han, W. Yao, Q. Zhu, Y. Meng and Y. Lu, Angew. Chem., Int. Ed., 2013, 52, 943 CrossRef CAS PubMed;
(g) T. Wang, W. Yao, F. Zhong, G. H. Pang and Y. Lu, Angew. Chem., Int. Ed., 2014, 53, 2964 CrossRef CAS PubMed;
(h) X. Han, W. Yao, T. Wang, Y. R. Tan, Z. Yan, J. Kwiatkowski and Y. Lu, Angew. Chem., Int. Ed., 2014, 53, 5643 CrossRef CAS PubMed;
(i) W. Yao, X. Dou and Y. Lu, J. Am. Chem. Soc., 2015, 137, 54 CrossRef CAS PubMed.
- For selected reviews, see:
(a) D.-F. Chen, Z.-Y. Han, X.-L. Zhou and L.-Z. Gong, Acc. Chem. Res., 2014, 47, 2365 CrossRef CAS PubMed;
(b) Z.-H. Shao and H.-B. Zhang, Chem. Soc. Rev., 2009, 38, 2745 RSC;
(c) Z.-T. Du and Z.-H. Shao, Chem. Soc. Rev., 2013, 42, 1337 RSC.
- For early examples, see:
(a) G.-S. Chen, Y.-J. Deng, L.-Z. Gong, A.-Q. Mi, X. Cui, Y.-Z. Jiang, M. C. K. Choi and A. S. C. Chan, Tetrahedron: Asymmetry, 2001, 12, 1567 CrossRef CAS;
(b) M. Nakoji, T. Kanayama, T. Okino and Y. Takemoto, Org. Lett., 2001, 3, 3329 CrossRef CAS PubMed.
- For recent excellent examples, see:
(a) Z.-Y. Han, D.-F. Chen, Y.-Y. Wang, R. Guo, P.-S. Wang, C. Wang and L.-Z. Gong, J. Am. Chem. Soc., 2012, 134, 6532 CrossRef CAS PubMed;
(b) Z.-L. Tao, W.-Q. Zhang, D.-F. Chen, A. Adele and L.-Z. Gong, J. Am. Chem. Soc., 2013, 135, 9255 CrossRef CAS PubMed;
(c) P.-S. Wang, H.-C. Lin, Y.-J. Zhai, Z.-Y. Han and L.-Z. Gong, Angew. Chem., Int. Ed., 2014, 53, 12218 CrossRef CAS PubMed;
(d) S. Mukherjee and B. List, J. Am. Chem. Soc., 2007, 129, 11336 CrossRef CAS PubMed;
(e) K. Sorimachi and M. Terada, J. Am. Chem. Soc., 2008, 130, 14452 CrossRef CAS PubMed;
(f) D. A. Nicewicz and D. W. C. MicMillan, Science, 2008, 322, 77 CrossRef CAS PubMed;
(g) M. E. Muratore, C. A. Holloway, A. W. Pilling, R. I. Storer, G. Trevitt and D. J. Dixon, J. Am. Chem. Soc., 2009, 131, 10796 CrossRef CAS PubMed;
(h) Q. Cai, Z.-A. Zhao and S.-L. You, Angew. Chem., Int. Ed., 2009, 48, 7428 CrossRef CAS PubMed;
(i) Q. Cai, C. Zheng and S.-L. You, Angew. Chem., Int. Ed., 2010, 49, 8666 CrossRef CAS PubMed;
(j) Q.-A. Chen, M.-W. Chen, C.-B. Yu, L. Shi, D.-S. Wang, Y. Yang and Y.-G. Zhou, J. Am. Chem. Soc., 2011, 133, 16432 CrossRef CAS PubMed;
(k) D. A. DiRocco and T. Rovis, J. Am. Chem. Soc., 2012, 134, 8094 CrossRef CAS PubMed;
(l) J. R. Zbieg, E. Yamaguchi, E. L. McInturff and M. J. Krische, Science, 2012, 336, 324 CrossRef CAS PubMed;
(m) S. Krautwald, D. Sarlah, M. A. Schafroth and E. M. Carreira, Science, 2013, 340, 1065 CrossRef CAS PubMed;
(n) S.-Y. Yu, H. Zhang, Y. Gao, L. Mo, S. Wang and Z.-J. Yao, J. Am. Chem. Soc., 2013, 135, 11402 CrossRef CAS PubMed;
(o) T. Miura, N. Nishida, M. Morimoto and M. Murakami, J. Am. Chem. Soc., 2013, 135, 11479 Search PubMed;
(p) Y. Zhang, C. S. Lim, D. S. B. Sim, H.-J. Pan and Y. Zhao, Angew. Chem., Int. Ed., 2014, 53, 1399 CrossRef CAS PubMed;
(q) K. Namitharan, T. Zhu, J. Cheng, P. Zheng, X. Li, S. Yang, B.-A. Song and Y. R. Chi, Nat. Commun., 2014, 5, 3982 CAS;
(r) H. M. Nelson, B. D. Williams, J. Miró and F. D. Toste, J. Am. Chem. Soc., 2015, 137, 3213 CrossRef CAS PubMed.
-
(a) B. G. Jellerichs, J.-R. Kong and M. J. Krische, J. Am. Chem. Soc., 2003, 125, 7758 CrossRef CAS PubMed;
(b) P. Webber and M. J. Krische, J. Org. Chem., 2008, 73, 9379 CrossRef CAS PubMed;
(c) S.-Q. Ye and J. Wu, Tetrahedron Lett., 2009, 50, 6273 CrossRef CAS PubMed.
- S. Muthusamy, C. Gunanathan, S. A. Babu, E. Suresh and P. Dastidar, Chem. Commun., 2002, 824 RSC.
-
(a) C. Zhu, X. Shen and S. G. Nelson, J. Am. Chem. Soc., 2004, 126, 5352 CrossRef CAS PubMed;
(b) C. A. Leverett, V. C. Purohit and D. Romo, Angew. Chem., Int. Ed., 2010, 49, 9479 CrossRef CAS PubMed.
-
(a) M. S. Taylor and E. N. Jacobsen, J. Am. Chem. Soc., 2004, 126, 10558 CrossRef CAS PubMed;
(b) I. T. Raheem, P. S. Thiara, E. A. Peterson and E. N. Jacobsen, J. Am. Chem. Soc., 2007, 129, 13404 CrossRef CAS PubMed;
(c) M. P. Lalonde, M. A. McGowan, N. S. Rajapaksa and E. N. Jacobsen, J. Am. Chem. Soc., 2013, 135, 1891 CrossRef CAS PubMed;
(d) M. Wasa, R. Y. Liu, S. P. Roche and E. N. Jacobsen, J. Am. Chem. Soc., 2014, 136, 12872 CrossRef CAS PubMed;
(e) H. Zhang, S. Lin and E. N. Jacobsen, J. Am. Chem. Soc., 2014, 136, 16485 CrossRef CAS PubMed.
Footnote |
† Electronic supplementary information (ESI) available. See DOI: 10.1039/c5qo00151j |
|
This journal is © the Partner Organisations 2015 |
Click here to see how this site uses Cookies. View our privacy policy here.