DOI:
10.1039/D2VA00324D
(Critical Review)
Environ. Sci.: Adv., 2023,
2, 1042-1059
Biochar implications in cleaner agricultural production and environmental sustainability
Received
21st December 2022
, Accepted 1st June 2023
First published on 1st June 2023
Abstract
Achieving food security while mitigating climate change is the foremost challenge for researchers and policy planners globally. Thus, dual objective approaches/techniques need to be developed, which can potentially increase food production with zero/negative greenhouse gas (GHG) emissions. The global agricultural production system generates a huge amount of bio-waste, which threatens agricultural and environmental sustainability. However, conversion of agricultural waste into biochar can potentially address the food insecurity and climate change challenges concurrently. Biochar production and utilization is proposed as an innovative solution for achieving the Sustainable Development Goals (SDGs), such as zero hunger, poverty, and climate change mitigation, by enhancing farm productivity and reducing/offsetting anthropogenic CO2 emission. Globally, biochar has the potential to increase crop productivity by 11% and reduces 12% human-induced GHG emissions annually. Biochar can potentially sequester ∼0.7–1.8 Gt CO2 (C eq.) y−1 in the soil system. Furthermore, biochar application improves soil health, which facilitates the plant growth and crop productivity. Biochar application can alters the plant physiology and makes the plant system more tolerant against biotic and abiotic stresses. Biochar is also an excellent in situ-sorbent for soil contaminants. However, some inconsistent reports about the utility of biochar are also available. Hence, an in-depth understanding about the uses and impact of biochar on the food production, soil health, and climate change mitigation is highly warranted, for framing the research priorities and policies for developing cleaner and sustainable agricultural production systems.
Environmental significance
Biochar production and utilization is proposed as an innovative solution for climate change mitigation by reducing/offsetting anthropogenic CO2 emissions. Biochar is an excellent carbon neutral material; one tonne biochar production can permanently remove ∼2.68 Mg CO2eq. from the atmosphere. Biochar curtails CO2 emissions through fossil fuel replacement by the production of syngas and bio-oil, in addition to its application and long-term C storage in the soil. Furthermore, biochar application decreases soil bulk density (ρb) and improves soil productive capacity. Carbon sequestration via biochar production is technically feasible and can be economically viable, approach to address the climate change, and land degredation issues especially with the current development of the carbon sink economy.
|
1. Introduction
The United Nations has setup 17 Sustainable Development Goals (SDGs) and 269 targets for 2030, out of which 10 SDGs are directly linked with agriculture. SDGs 1 and 2 are related to “no poverty ” and “zero hunger” as crucial for sustainable agriculture. Globally, ∼811 million people suffer from hunger, and one in ten people suffers from chronic hunger.1 This indicates that the world population is at a critical juncture, and needs technological attention and legislative support to ensure food security. Hence, to meet global food security while harmonizing the dwindling resources and fluctuating climate, agricultural production systems must be more productive and adaptable.2
Globally, anthropogenic activities added ∼16 Pg C (1 Pg = 1 billion metric tonnes) to the environment annually, which corresponds to 24% net primary productivity.3,4 Human-induced CO2 emission increases by >3% per annum, which puts Earth on an irreversible climate change track.5 Agricultural production systems contribute about 10–14% of the total GHG emissions,6 which is likely to be increased to 50% by 2030.7 Hence, the implementation of an ambitious climate mitigation program is highly desirable to achieve environmental sustainability. To control the rising temperature and total GHG emission, future agricultural production systems must be carbon neutral or negative.8 The initiative of “4 per 1000” was launched by the United Nations Framework Convention on Climate Change9 with the focus on soils for food security and the environment to combat the global GHG emission issues (https://www.4p1000.org). It aims to increase the annual soil organic carbon (SOC) sequestration of global agricultural lands to 2–3 Gt (Giga tonnes) carbon (C) in the upper 100 cm soil, which can effectively offset 20–35% of the global C emission. Hence, carbon negative high food-producing technologies must be adopted to address the multiple challenges.
Agricultural-derived biomass is a readily available renewable energy and nutrient source. However, transportation cost increases the price of biomass energy. Hence, for energy generation from biomass, cost effective and robust technologies are highly warranted.10 Unscientific biomass management practices like field burning and open dumping causes environmental and human health issues.11 Field fire impairs the air quality, posing health hazards, like skin/eyes irritation, asthma, bronchitis, emphysema, and cancer.12 Modern technology-lead biomass valorization can potentially increase the farmer's income, energy security and environmental and soil quality.13,14 Biomass energy can be cost-effective energy sources in rural areas. Hence, adequate incentive facilities are warranted to encourage the use of low-cost biomass energy.15,16 Biochar applications in agricultural production systems are attaining immense importance due to its economic and environmental benefits.17 Biochar can potentially be considered an innovative tool to address the food insecurity and environmental issues by improving soil health,18 crop productivity,19 curtailing GHG emissions20 and water pollution.21 The biochar production by thermal decomposition at elevated temperature in the absence of oxygen (pyrolysis) has proved to be an alternative strategy for agri-waste management.22,23 Additionally, modern technology-lead agri-waste-pyrolysis yielded syngas, bio-oil, and biochar.24 Traditional biochar production methods emit more methane (CH4), N2O, soot, or volatile organic compounds, which causes excess C payback time and is also hazardous to health.25,26 Utilizing biomasses as biochar via pyrolysis and hydrothermal carbonation reported superiority in terms of GHG reduction over traditional methods.21 Biochar is a solid C-rich pyrolyzed (250 and 900 °C) biomass material produced under oxygen-free condition.17 It is a superior C carbon source and has better nutrient retention capacity over other C sources.27
Biochar helps in two ways to reduce the atmospheric CO2: (1) by its production and (2) by sequestering atmospheric CO2 in soil.28–30 It is possible to offset up to 1.8 Pg CO2eq. (Penta gram carbon dioxide equivalent) per annum through biochar, compared to 15.4 Pg CO2eq. emitted annually.4 However, inconsistent reports are also available on the GHG emission mitigation potential of biochar. For instance, biochar-amended soil had higher CO2 and N2O emissions due to the carbonaceous nature and quality of biochar feedstock.31 Another study suggested that biochar application did not have any significant effect on CO2 emission from paddy and chestnut soils in China. This was mainly due to the soil and feed stock attributes.32 However, biochar application at the rate of 30 Mg ha−1 reduces CO2 emission by 31.5% and 7.2% in forest and agricultural soils, respectively.33 Long-term biochar application decreases CH4 emission.34 However, short-term applications induced CH4.35 Biochar application induced CO2 emission by 22.14% and decreased N2O emission by 30–38%, and did not influence the CH4 emission.36,37 The variable effect of biochar on GHG emission from the soil might be attributed to variations in the soil, feedstock, climate types, and measurement methods of GHG emission, application rate, and pyrolysis temperature.38,39 Overall, soil-applied biochar improves agricultural and environmental sustainability.40
Biochar application decreases soil bulk density (ρb), and enhances soil productive capacity and plant growth.41,42 Biochar application modulates the soil health, resulting in higher crop yields.43–46 Liu et al. (2013)47 screened the 116 published studies from 21 countries, and summarized that biochar application increased crop productivity by 11%. But biochar application rate must be optimized as at higher doses of biochar application had the deterimental effect on plant growth.48 Abukari et al. (2021) showed that application of biochar at the rate of 30 Mg ha−1 enhanced legume productivity by 30%, vegetable yield by 29%, and cereal yield by 7–8% over no biochar.49 The addition of recalcitrant C in the soil enhances the soil-water-nutrient holding capacity, which promotes crop growth.50 An increased level of non-labile C in the total SOC is important to mitigate climate change and restore the fertility of the degraded soil.50–52 Continuous biochar application with the rate of 11.25 Mg ha−1 in both crops under a maize-wheat system for five years fixes 182.3 Mg ha−1 CO2 over the control plot.53 Short-term biochar application at the rate of 7.5 Mg ha−1 also improves SOC by 39% in maize-wheat systems after two cropping cycles. Owing to the priming effect, short-term biochar application increases the labile C pool. However, long-term biochar application suppressed the SOC mineralization.54,55 Still, the biochar interaction with SOC changes over time, and the negative priming effect is nullified after 3–5 years of application.28,53 One tonne SOC increment in cropland increases crop productivity by 50% and curtails atmospheric CO2 by 5–15%.28,53 Besides that, biochar also has the potential to suppress the plant pathogen by altering the soil microbiome structure.46,56 Biochar reduces runoff losses, nutrients erosion, and soil pollutant loads,52 and improves carbon sequestration. Overall, biochar is a potential solution to address the global food, energy, and environmental challenges.57–59 However, this information needs to be brought together in a comprehensive and analytical way, which may be more useful to the researchers and policy planners. The present review is an effort to provide a comprehensive assessment and insight of the different mechanisms involved in biochar production, its application and effect on food production, and environmental sustainability, which may be helpful to formulate the biochar-based policy for sustainable and clean agricultural production.
2. Agricultural waste and biochar production
Conversion of forest land to biomass producing crops or forest felling for feedstock is not a sustainable practice as land-use changes lose ∼22 Mg C ha−1, and will result in a carbon payback time of ∼10 years. Hence, forest trees must not be cut for the cultivation of biomass-producing crops. Furthermore, productive land should not be brought under the cultivation of biomass crops, as it will compromise food security. On the other hand, crop residue management is challenging to farmers as it is a labor-consuming and costly affair. The absence of site-specific residue management technologies further makes it tedious.11 Crop residue, poultry litter, dairy manure, sugarcane trash, cotton trash green wastage of various crops, animal manures, and other forests' unused wood material are potential substrates for biochar production.60,61 Weed biomass can be pyrolyzed in an oxygen-free condition to produce biochar. Lignin-rich agro-waste is more efficient for biochar production, as it yielded more char as compared to cellulose and hemicellulose-rich materials. Pyrolysis temperature also plays a key role in biochar production. Lignin-rich biomass pyrolyzed at a wider temperature range (160–900 °C) while hemicellulose and cellulose-rich residues were pyrolyzed at a temperature of 220–315 °C and 315–400 °C, respectively. Low-temperature/high-pressure (hydrothermal carbonization) and slow pyrolysis are two effective methods of biochar production from different feedstock. Biochar properties mainly depend on the type of feedstock and pyrolysis temperatures. Biochar produced at a higher temperature (>550 °C) has more surface area and sorption capacity, as compared to those produced at a lower temperature. Pyrolysis completely avoided the immediate decay of residue inputs, and halted the CO2 and CH4 emissions.
Pyrolysis converts cellulosic carbons into more stable aromatic carbons, which can subsequently be customized for diverse agricultural uses.62 Biochar production from crop biomass is performed by the dry pyrolysis process in the absence of oxygen at high temperatures.41 Thermochemical conversion techniques, including gasification, pyrolysis, hydrothermal carbonization, and torrefaction, are the most commonly used practices for biochar production.47,63 Pyrolysis is the most commonly used technique for biochar production.64 The process of conversion of crop residue into final biochar production depends on the nature of the crop, stage of crop residue, size, shape, and composition concerning organic material, viz., cellulose, hemicellulose, lignin, etc.65,66 Biochar production at higher temperatures is reported to have a higher surface area and pore volume than those produced at a lower temperature.67 The wet pyrolysis process of biochar production is associated with the hydrothermal carbonization (HTC) process, in which biochar is produced at low temperature and pressure in an aqueous solution. This process is more useful in residues containing more lignin, cellulose, and hemicellulose.68,69 The temperature requirement in this process for converting biomass into biochar is comparatively lower than pyrolysis.70 Biochar produced through HTC has less surface area and is not ideal for agricultural use as compared to biochar produced by pyrolysis.71 However, the HTC is an energy-efficient process and operates at lower temperatures with higher biochar recovery.72 Biochar produced through pyrolysis has higher surface areas than that produced through hydrothermal carbonation. Liquid biofuels and syngas, such as CH4, C2H6, CO, CH4, and H2, are the co-biochar products resulting from pyrolysis and hydrothermal carbonation.71,73–75
3. Effect of biochar on crop productivity
Biochar application in agriculture improves crop yield by altering soil properties and enhancing the SOC content.76,77 Improvement in soil quality and tolerance in plants against biotic and abiotic stresses due to biochar application is responsible for improvements in agricultural production.78 The increased crop yields from biochar application are linked to improved soil quality.79 Biochar improves carbon sequestration, soil quality, and crop growth significantly.80 Biochar application in nutrient-deficient and degraded soils increases agricultural productivity.81 Application of eucalyptus-based biochar at the rate of 50 Mg ha−1 increases maize productivity by 48–50% in acidic soils of humid tropical condition.82 Yield improvements in different crops due to biochar application under various ecologies were reported by several researchers (Table 1). Biochar improves soil structure and carbon content, as well as nutrient availability, and microbial population, which improve crop yields.83 The delayed production of an organic layer on the biochar surface during aging helps to retain nutrients, and may account for the higher yield.84,85 Biochar increases soil structure, fertility, nutrients use efficiency (NUE), and crop yields.86,87 Biochar application enhances agricultural productivity via improvement soil health, decrease in crop biotic and abiotic stresses, and modulation of plant physiological processes.
Table 1 Effect of biochar on the productivity of different crops under various ecologies
Location |
Crop |
The material used and dose |
Yield enhancement |
Reference |
Cereals
|
Hunan province, China |
Rice |
Rice husk biochar (20 t ha−1) |
6% economic yield enhancement over control |
167
|
São Paulo, Brazil |
Wheat |
Sugarcane straw biochar (1.9%) |
27% and 16%, improvement in grain and total biomass yields, respectively over control |
168
|
Nanjing, China |
Straw biochar (20 Mg ha−1) |
The grain yield was 2.91–19.4% higher over urea application at 200 kg ha−1 |
33
|
Sumatra, Indonesia |
Maize |
Cacao shell biochar (15 Mg ha−1) |
Cacao shell biochar recorded 8.6 times higher grain yield compared to the same dose of rice husk biochar |
169
|
Northern and Upper East Ghana |
Rice husk and sorghum (2 Mg ha−1) |
27% and 16%, increment in grain and shoot biomass yield over control, respectively |
170
|
![[thin space (1/6-em)]](https://www.rsc.org/images/entities/char_2009.gif) |
Pulses
|
Kumasi, Ghana |
Cowpea |
Corn cob feedstock (5 Mg ha−1) |
Increased seed yield by 36% over control |
78
|
Lake Victoria basin, Kenya |
Soybean |
Accasia spp. wood biochar (100 Mg ha−1) |
Increased seed yield by 0.43 t ha−1 over control |
171
|
Bule wereda, Southern Ethiopia |
Garden pea |
Lantana camara biochar (12 Mg ha−1) |
54% higher yield compared to corn cob biochar of the same rate of application |
172
|
Montes Claros, Brazil |
French bean |
Biochar from sawdust filter (7.12%) |
448% higher per plant seed yield (18.6 g) compared to control (3.39 g) |
173
|
Karnal, India |
Green gram |
Sugarcane bagasse biochar (SBB) (4.5 g kg−1 soil) |
Enhanced pod yield by 15% over 50% RDF alone |
116
|
![[thin space (1/6-em)]](https://www.rsc.org/images/entities/char_2009.gif) |
Oilseeds
|
Rasuwa, Nepal |
Mustard |
Eupatorium adenophorum shrub biochar (40 Mg ha−1) |
Increased grain yield by 134% compared to control |
174
|
Riyadh, Saudi Arabia |
Sunflower |
Rice straw biochar + foliar silicon |
Increased seed yield by 27% over control |
175
|
North Queensland, Australia |
Groundnut |
Willow wood biochar (10 Mg ha−1) |
Increased seed yield by 21% over fertilizer alone |
94
|
Dhaka, Bangladesh |
Sesamum |
Biochar (6 Mg ha−1) |
18.8% higher yield over control |
176
|
![[thin space (1/6-em)]](https://www.rsc.org/images/entities/char_2009.gif) |
Vegetables
|
Geraldton, Western Australia |
Cucumber |
Poultry litter biochar (PLB) (13 Mg ha−1) |
Application of PLB + poultry manure (9 Mg ha−1) + nitrophos (5 Mg ha−1) increased cucumber yield by 300% over PLB (1 Mg ha−1) + poultry manure (2 Mg ha−1) + nitrophos (13 Mg ha−1) |
177
|
Dhading, Nepal |
Pumpkin |
Eupatorium adenophorum biomass loaded with urine |
300% higher pumpkin yield over urine application alone |
178
|
Hohai, China |
Tomato |
Wheat straw biochar (8%) |
Increased ∼50% fruit yield over control |
179
|
Ondo State, Nigeria |
Cocoyam |
Hardwood biochar (20 t ha−1) |
Increased economic yield by 9% over hardwood biochar (10 Mg ha−1) |
180
|
4. Effect of biochar on stress tolerance in crops
Biochar has various benefits in agriculture, including the alleviation of various kinds of abiotic stress, such as salt and drought.88,89 Biochar application at a rate of 20 Mg ha−1 increased nutrient uptake and chlorophyll concentrations in pumpkin plants.90 Biochar improved tomato leaf water usage efficiency, stomatal conductance, chlorophyll concentration, photosynthesis, and relative water content in dry conditions.91 The high adsorption capacity of salt makes biochar an excellent ameliorant for the reclamation of the degraded salt-affected region.92 Biochar has a high salt adsorption capability due to its vast surface area and cation exchange capacity. As a result, biochar can be used to reduce plant sodium intake, and thereby mitigate the negative effects of salt stress. Biochar application increases agricultural output in acidic soils by allowing plants to tolerate salt.82 Biochar facilitates the absorption of an array of soil contaminants owing to its high surface area, porosity, and adsorption ability.93 The plants' physiological and biochemical properties were altered due to biochar application, which helps to combat the multiple stresses.94 Biochar modulates the different plant physiological and soil properties, which helps the plants develop the resistance mechanism against biotic and abiotic stresses (Fig. 1). Biochar application increases plant photosynthesis, chlorophyll content, and transpiration rate in different crops under various growing media.87,94 It also enhances the gas exchange ability of crop leaves and reduces oxidative stress.95 Differential crop responses to various kinds of biochar are reported by various research across the eco-regions of the world (Table 2). Biochar has been shown to boost plant performance, and lessen the severity of both air and soil-borne pathogen when used as a soil amendment. Biochar has the potential to have both direct and indirect antagonistic effects on pathogens, such as generating systemic resistance in plants, as pathogens and biochar both reside in the soil.96 The biochar application geared the acquired and induced systemic resistance pathways, giving the soil a broad-spectrum disease-controlling potential.97 Biochar generated from green waste and wood waste was used to reduce early blight growth in tomatoes.91 Biochar has a positive impact on favorable microorganisms that actively defend against diseases causing soil pathogens by producing complex chemicals. Some plant-based products with insecticidal qualities, such as neem cake, can be used to make biochar, and their effect on insect-pest tolerance in plants can be studied. The nature of raw material and the application rate of biochar influence the management of insect pests and nematodes.98 Moreover, plant tolerance to biotic and abiotic stresses due to biochar is linked with improvements in beneficial soil microorganisms in the soil, soil amelioration, modulation in plant biochemical constituents, and morpho-physiological characteristics.
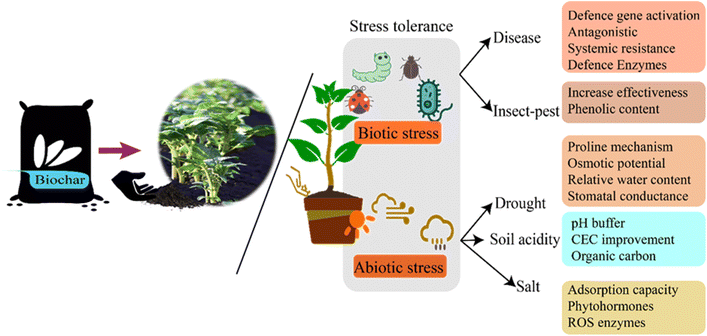 |
| Fig. 1 Biochar modulates soil condition and plant physiology. | |
Table 2 The effects of biochar on crop stress tolerance
Location |
Crop |
Biochar application |
Type of stress tolerance |
References |
Cereals
|
Changsha, China |
Rice |
Rapeseed stover biochar 40 g kg−1 soil |
Reduced heat stress by altering root zone soil and regulating heat shock protein in roots and leaves |
167
|
Bahawalpur, Pakistan |
Wheat |
Wheat straw biochar (37.18 g kg−1 soil) |
Drought stress resistance by increasing water use efficiency by 19.30% over control |
181
|
Rawalpindi, Pakistan |
Biochar (2%) |
Mitigating salt stress by decreasing proline and soluble sugar contents by 51% and 27%, respectively, and increasing leaf water by 16% and osmotic potentials by 10% |
182
|
Matatirtha, Nepal |
Maize |
Eupatorium adenophorum biochar (2%) |
Increased nutrient availability in acidic soils in nutrient-stressed condition |
174
|
El-Gharbia, Egypt |
Barley |
Biochar (20 Mg ha−1) |
Reduced electrolyte leakage, lipid peroxidation, and proline levels reduce drought stress |
183
|
![[thin space (1/6-em)]](https://www.rsc.org/images/entities/char_2009.gif) |
Pulses
|
Henan, China |
Soybean |
Wheat straw biochar (10 g kg−1 soil) |
Significantly increased water use efficiency by 27.5% over control |
41
|
Gazipur, Bangladesh |
Poultry litter biochar (50 Mg ha−1) |
Enhanced drought resistance due to increase in water content in plants and reduction in chlorophyll breakdown in leaves |
184
|
Gazipur, Bangladesh |
Pea |
Sawdust and rice husk biochar |
Reduces oxidative and osmotic stress |
95
|
Germany |
Lupin |
Biochar char from maize and wood |
Inoculated bacteria Pseudomonas putida and Stenotrophomonas pavanii survived better in the rhizosphere |
185
|
![[thin space (1/6-em)]](https://www.rsc.org/images/entities/char_2009.gif) |
Vegetables
|
Rishon Lezion, Israel |
Tomato |
Pepper plant waste biochar (3%) |
Enhanced resistance against Fusarium oxysporum f. sp. radicis lycopersici |
96
|
Guangdong, China |
Peanut shell and wheat straw biochar (2%) of each |
The bacterial wilt disease index was reduced by 28.6% and 65.7%, respectively |
47
|
Lubbock, United States |
Brinjal |
Oak and pine biochar (12.5 kg) |
Increased stomatal conductance and photosynthetic rate, as well as reduced leaf temperature and electrolyte leakage, to reduce salinity stress in plants |
186
|
New Delhi, India |
Spinach |
Wood biochar |
Increased chlorophyll, carotenoid, and relative water of the leaf |
187
|
![[thin space (1/6-em)]](https://www.rsc.org/images/entities/char_2009.gif) |
Forage
|
Yangzhou, China |
Forage sorghum |
Wheat straw biochar (2.5%) |
Mitigate the negative effects of salinity on antioxidant enzymes |
188
|
Amhara Region, Ethiopia |
Teff |
Eucalyptus biochar (12 Mg ha−1) |
Lowering exchangeable soil acidity reduced the negative effects of soil acidity on plants |
189
|
![[thin space (1/6-em)]](https://www.rsc.org/images/entities/char_2009.gif) |
Others
|
Multan, Pakistan |
Mint |
Fruit and vegetable waste and compost biochar (1 : 1) |
The leaves' lead uptake was reduced by 13.5% over non-treated plants |
190
|
Merelbeke, Belgium |
Strawberry |
Biochar (3%) |
On both leaves and fruits, there is a decreased sensitivity to the fungal disease Botrytis cinerea |
191
|
5. Effect of biochar on soil health
Most tropical and subtropical soils suffer from severe soil fertility depletion due to excess nutrient mining and soil organic matter reduction, resulting in poor agricultural productivity.99,100 Hence, the application of an innovative product like biochar is highly warranted to address the complex issue of soil quality and agricultural productivity.2,101 Biochar addition modulates the soil biology, chemistry, and physical properties.10 During the last two decades, even though biochar as an amendment has been widely researched, the impact of its application was mixed due to the complex nature of biochar, soils, and crops.2,102 The effects of the biochar is variable; it works efficiently in acidic, but deteriorated coarse textures soils.103 The optimum application of biochar has the potential to improve soil conditions and reduce GHG emissions, and also immobilize toxic metals and other organic pollutants.86,101 However, contrary to these, some adverse effects of biochar at higher doses have also been documented.104
5.1 Effect of biochar on soil physical properties
Biochar application has shown a differential effect on soil physical properties like bulk density (ρb), porosity, hydraulic conductivity, and soil color under diverse soil and climate types.104,105 The ρb is an important physical soil parameter that controls nutrient availability, porosity, and compactness of the soil. Biochar is a porous material having less ρb (0.3 to 0.6 Mg m−3) than the common agricultural soils. The temperature during pyrolysis and the nature of the feedstock used for biochar production influence the ρb of the biochar. Hence, the reduction in ρb is entirely driven by soil condition, the dose, and the properties of biochar applied. Application of woodchip biochar decreases the ρb of planosol by 5.1% over the control.2 The response of the biochar on ρb is more effective in light soil than in heavy soils.106,107 Biochar addition promotes soil fungal growth, as well as microbial activities, resulting in soil agglomeration, along with profuse root development, subsequently reducing ρb.108 The positive effect of biochar on soil porosity and hydraulic conductivity have also been reported by many researchers.66,109 The aggregate formation and stability index of silty loam, sandy loam, silty clay, and clay soils had been improved with biochar application,110 while no change was reported in sandy loam soil.34 The biochar application can change the size and distribution of soil pores to a relatively smaller pore size, which positively impacts crop growth and development.111 Biochar application reduces the ρb, which alters soil porosity and nutrient availability, resulting in higher above and below-ground biomass.112 Hydraulic conductivity of the soil is crucial for available soil water movement within the soil, and also for the water holding capacity. Biochar application had more impact on sandy soil as compared to clay soil.2,113 The feedstock used for biochar preparations also had a varied response for WHC; woody feedstock had higher WHC than lignocellulosic and herbaceous feedstock due to the greater pore space.114 In sandy soil, the application of biochar significantly reduced the saturated hydraulic conductivity due to more water retention in a single layer.115
5.2 Effect of biochar on soil chemical properties
The soil chemical properties are very crucial sustainability indicators of crop production, which is entirely dependent on soil nutrient status and crop acquisition pattern.116 The post-green revolution era created a multi-nutrient deficiency in soil due to the global continuous overuse of straight fertilizers.117 A healthy soil environment plays a pivotal role not only in good plant growth, but also in higher productivity.6 Hence, innovative nutrient management options, viz., application of biochar produced from different feedstocks over a long period to the soil can increase the soil nutrient effectiveness and their use efficiency compared to the control.105 Over a long term (>5 years), biochar additions enhanced the soil organic matter (46%), which is the basis for the nutrients mineralization process.118 The necessary mineral nutrients contained in the biochar keep helping the plants by providing continuous flow from soil to the plants.105 Furthermore, biochar application increases the cation exchange capacity, which resulted in improved K, Ca, Mg, Zn, Mn, and Cu availability. All such positive effects of biochar have been reported to enhance plant growth and crop yield.119 All these plant nutrients are pH-dependent, which is very much affected by the application of biochar as its nature is alkaline (pH 6–12).100 Biochar application reduces the concentration of Al3+ and H+ in the ions in acidic soils.120
A large variation in biochar applications ranging from 10 to 150 Mg ha−1 in soil has been reported in many studies. However, the economic feasibility was mostly observed in the range between 20 and 30 Mg ha−1.32 In many studies, the biochar application increased the C, N, and P content in the soils, and promoted soil aggregation and stimulation of microorganism activities.18,121,122 The improvement of soil chemical properties was witnessed with the increase in organic matter. Furthermore, similar positive results of improvement of electrical conductivity and pH were observed in contaminated soil with the application of biochar.123 The availability of essential plant nutrients is largely dependent on the soil pH. The nutrient supplying ability of biochar applied to the soil is mainly dependent on the feedstock used for its preparation. The increased availability of the nutrient with the addition of biochar is mainly due to changes in circulation, retention, and conversion to plant-available form.124 The higher CEC of biochar adsorbed NH4+ on the surface and was reused. Therefore, N utilization and its loss were minimized by the adsorption of NH3.125 The soil microbial nitrogen content increased with increasing biochar addition into the soil as microorganisms absorbed NO3 N in R-NH2, which is easily adsorbed by biochar and soil minerals. Biochar can detoxify heavy metals in contaminated soil,126 as well as other sources of toxic effluents.101 The biochar with a large specific surface area and diverse negatively charged anions suitably immobilized the inorganic pollutants in soil.127 The mobility of heavy metals in soil is reduced due to adsorption, ion exchange, and stabilization with the formation of organometallic compounds.128 The combined application of biochar along with compost help in the immobilization of toxic materials in heavy metal polluted soils.123,129 Rodríguez-Vila et al. (2016)124 reported a reduced concentration of Al, Cu, Fe, Mn, Ni, Zn, Co, and Mg in soil water and increased potential immobilization of toxic elements, thereby reducing the contamination risk with oak wood biochar. The combined application of biochar with compost is reported to reduce potentially toxic metals, improve soil fertility, and thus have a synergistic effect on soil remediation, as well as higher crop productivity.18,130 Maize biochar-based fertilizer can be used as a remedial measure for cadmium (Cd)-polluted soil, as its presence caused rigorous health issues in humans via induction into the food chain.123 Furthermore, due to the nutrients chelating properties, biochar application reduces the nutrient leaching in the soil system.10
5.3 Effect of biochar on soil biological health
The soil biological properties play a crucial role in nutrients mineralization, which is the basis for plant nutrients availability.129 Soil microbial diversity is directly related to the soil's physicochemical properties.33 The biochar itself, having a porous and aromatic structure with stretched surface, becomes a harbor for soil microbial organisms and provides nutrients for their multiplication.18,131 Biochar is helpful in bacterial decomposition, as it contained decomposed C and N on its surface. The enhanced microbial biomass carbon (MBC) and microbial biomass nitrogen (MBN) contents have accompanied the corresponding decrease in dissolved organic C concentration, and have a stronger microbial diversity.132 The biochar surface adsorbed the bacteria, making them less susceptible to leaching, and thus increased the population of bacteria in the soil. The biochar application increased the nitrogen fixation capacity by increasing the number of N-fixing bacteria.133 Biochar application significantly enhanced the soil MBC and MBN.126 Biochar alters the soil enzymatic reaction; however, the intensity of the soil enzymatic alterations depends upon the feedstock nature from which biochar has been developed.134 The addition of biochar developed from pinewoods and grass as feedstock has decreased the β-glucosidase and phosphate activities in soil.135 In contrast, Oladele et al. (2019)19 reported that the activities of alkaline phosphatase, urease, invertase, and catalase have been increased with the addition of rice husk biochar (12 Mg ha−1).
6. Biochar for climate change mitigation
6.1 Carbon sequestration potential of biochar
The global carbon cycle is largely linked to climate change and CO2 emission from fossil fuel usage and land-use change.99 Soil carbon sequestration is the trapping of CO2 from the atmosphere, and storing it in the soil in more stable forms.136,137 As a climate change extenuation approach, carbon sequestration is a simple and robust technique to track national carbon accounting.138,139 Furthermore, carbon sequestration facilitates the conversion of biomass into the recalcitrant soil C pool and lowers the CO2 levels in the atmosphere. Thus, the production of biochar from crop residue and its application into the soil have demonstrated a significant potential for carbon sequestration and extenuation of the harmful impacts of climate change.29,136 Biochar is an excellent carbon neutral material. A total of 2.879 Mg CO2eq. is embodied in a tonne of biochar (dry basis), and one tonne biochar production can permanently remove ∼2.68 Mg CO2eq. from the atmosphere.140 However, the carbon removal capacity of the biochar depends on the quality of the raw material and biochar production temperature. Conversion of one tonne agricultural residues into biochar removes ∼0.92 Mg CO2eq. from the atmosphere,141 while conversion of one tonne forestry residue sequesters 2.74 Mg CO2eq.142 Furthermore, it has been estimated that by 2050, the carbon removal potential of biochar will be about 0.3–2 Gt CO2 y−1.143 It has been predicted that the use of biochar can offset anthropogenic CO2 emissions by up to 12%.4 Some studies reported that biochar can sequester carbon by up to 2.27 Pg C y−1 in the soil at the global level. Due to the inherently fixed carbon in the biomass materials, which would otherwise break down into GHG and remain buried in the soil for a longer time, biochar has the potential to avert climate change.
Biochar has been recognized as an important approach for climate change mitigation by sequestering CO2 in soils, and thereby reducing excess CO2 from the atmosphere.27 As a result, it may be a viable alternative strategy for sequestering more CO2 from crop wastes than traditional agricultural practices, which result in rapid carbon mineralization and increased CO2 release into the atmosphere.144 The carbon sequestration potential of biochar lies between 0.7 and 1.8 Gt CO2eq. y−1.51,145 The environmental stability of biochar-C is extremely high as compared to other C sources, which facilitate the changes of the short-term atmospheric C cycle to the long-term geological C cycle when applied to soil.146 However, the C content of biochar mainly depends on the types of processing (heating temperature of pyrolysis) and types of feedstock used.145,147 The C content of biochar tends to rise as the production temperature rises. However, the biochar produced at 500 °C had maximum C sequestration ability, even though the biochar produced at higher temperature contains more C in the recalcitrant form than the biochar produced at a lower temperature.148 Biochar made from wheat straw at 500 °C had a higher carbon content (662 g kg−1) than biochar made at 300 and 400 °C.149 With an increase in product temperature from 350 to 500 °C, there was a greater organic C content of 13.98% to 20.57% and 16.45% to 26.91% in coffee husk biochar and corn cob biochar, respectively.150 Biochar's carbon sequestration capacity can be potentially increased by increasing the proportion of stable carbon content. This is usually performed by the pyrolysis process, which reduces the solids production, while increasing the C release as a gas, leading to the increased CO2 emissions when burned.146,151
The carbon sequestration mechanism through biochar in soil is complicated and not thoroughly understood. However, the C sequestration mechanism of biochar mainly depends on feedstock types, pyrolysis temperature, and soil texture. Biochar put into the soil interacts with the environment's precipitation and temperature to determine how long biochar carbon is kept in the soil. The trapping of the labile pool in biochar pores and the protection of soil C in organo-mineral fractions physically might lead to a negative priming effect.150 However, the organo-mineral complex formation is the main mechanism of C stabilization in the soil after biochar application.53 The size of the biochar particles, stability, and interaction with soil particles and environments determine the C residence time in the soil.8,151 Biochar adds more carbon to the passive pool of stable or inert carbon, which is less prone to degradation.152 The CO2 collected by biochar may bond due to interactions between clay minerals and functional groups on the surface (Fig. 2). As a result of its interactions with soil particles, biochar becomes stable in the soil. Owing to the larger surface area, clay particles stabilize biochar more effectively than the sand particles.99 Biochar sequester more carbon in C-starved soil than in soils with higher carbon content. The C content in the soil and rate of soil organic carbon (SOC) breakdown are inversely related; thus, biochar application reduces the C degradation and hence improves the SOC status. Biochar additions in soil restricted the gasses' losses of nitrogen by arresting/reducing the nitrification and denitrification processes, in addition to increasing the soil CH4 uptake.133,153 Irrespective of the feedstock types used in biochar preparation, biochar loses the C content over the period due to the aging effect. Biochar prepared from maize stover had less carbon mineralization ability as compared to the biochar prepared from pulses and other cereal residue.154,155 Likewise, Eucalyptus saligna pyrolysis at 550 °C resulted in lower SOC mineralization by 5.5% over the control.156 Application of biochar at the rate of 4.2 Mg ha−1 y−1 increases the soil C storage of 2.35 ± 0.4 Mg C ha−1 y−1 in sugarcane fields across the different regions of Brazil.157 In maize crops, biochar produced from corn residue increased the SOC sequestration by 12–37% in the furrow slice (15 cm soil depth).158
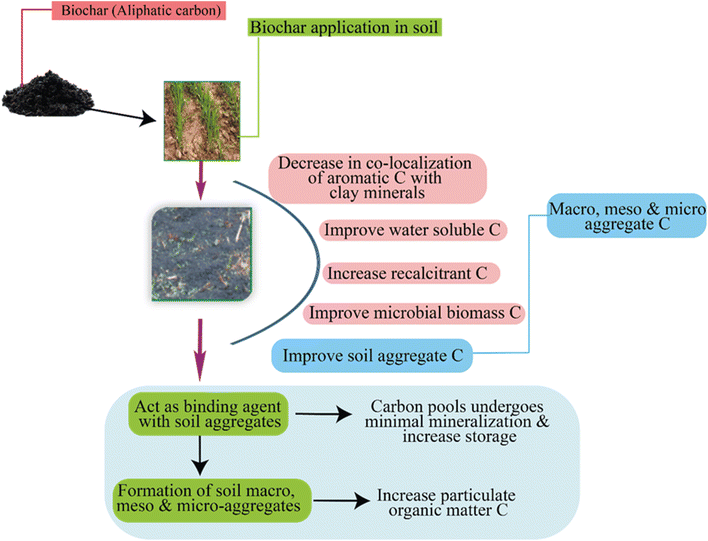 |
| Fig. 2 Possible mechanism of carbon sequestration by biochar inside the soil. | |
7. Biochar and GHG emission mitigation
Crop residue burning generates a huge amount of greenhouse gases (GHG), especially CO2, CO, and CH4. The burning of one tonne rice straw produces ∼3 kg of particulate organic matter (POM), 1460 kg of CO2, 60 kg of CO, 199 kg of ash, and 2 kg of SO2.152 The composition of the atmosphere is altered due to crop residue burning, resulting in an imbalance of radiation. Due to increased GHG emissions, the stratospheric ozone layer has been depleted. The long-term application of recalcitrant C-rich biochar has been shown to be an effective technique for mitigating climate change,159 but the effect of biochar on GHG emission mitigation is variable (Table 3). Priyadarshani and Prabhune (2009)160 suggested that biochar could help to reduce greenhouse gas emissions by 2–4% out of the 1900 million tonnes of CO2 in India. Biochar curtails CO2 emissions through fossil fuel replacement by the production of syngas and bio-oil, in addition to its application and long-term C storage in the soil.161 The beneficial impacts of biochar application in terms of GHG emission reduction were previously reported by several researchers.137,152 In contrast, He et al. (2018)36 found that incorporation of biochar enhanced soil CO2 fluxes by 22.14%, but lowered N2O fluxes by 30.92% and had no effect on CH4 fluxes. Biochar reduces the capacity of the soil to produce N2O by causing microbial immobilization of the available nitrogen in the soil.162 Biochar reduced the cumulative N2O by 52–84% and NO by 47–67% emissions, as compared to those of chemical fertilization.163 Biochar can thus be used in conjunction with mineral fertilizers to reduce N2O emissions, while not interfering with mineralization or nitrification processes. Furthermore, at high pyrolysis temperatures, N2O emissions were shown to be lower than at low pyrolysis temperatures. Under the controlled condition, the conjoint application of Miscanthus biochar at the rate of 30 Mg ha−1 (pyrolyzed at 600 °C) and nitrogen-rich litter reduces N2O emissions by 42% over the control.164 Biochar application increases soil pH, restrains nitrate reductase activity, and reduces N2O emission.32 According to Yanai et al. (2007),165 biochar application reduces N2O emissions by 85% over the control. Application of biochar at the rate of 20 and 40 Mg ha−1 in rice-wheat systems reduced N2O emissions by 19.55% and 26.35%, respectively, over the control.126 However, a lower dose of biochar with the rate of 20 Mg ha−1 resulted in an 8.89% higher N2O emission than the control, whereas increasing the biochar dose from 20 Mg ha−1 to 40 Mg ha−1 led to 26.06% lower N2O emission.158 The amount of biochar incorporated also has a tenacious impact on soil N2O emissions. Biochar promotes N2O emission at lower rates of application, while it lowers N2O emission at larger dosage.158 Soil supplemented with biochar as an organic amendment is reported to reduce CH4 emissions.115,158 Biochar improves soil aeration, reduces denitrification, and increases CH4 sink capacity,152 thereby retarding the CH4 emission. The near-complete suppression of CH4 emissions was noticed in soybean crops supplemented with biochar in acidic soil of Eastern Colombian Plains.166 Biochar application at the rate of 20 and 40 Mg ha−1 in rice-wheat systems reduced the CH4 emission in rice by 11.19–17.45% over the control.126 Moreover, biochar can potentially reduce the GHG emissions. However, the GHG emission mitigation potential of biochar is controlled by several factors like the biochar substrate, application dose, preparation methods, soil and crop management techniques, and the reaction of biochar with soil constitutes and other applied inputs, like manure and fertilizers, etc.158
Table 3 Impact of biochar application on greenhouse gases emissiona
Biochar material |
Tested crops |
Pyrolysis temperature (°C) |
Rate of biochar application (Mg ha−1) |
Impact on greenhouse gases (percent) reduction (−) or increase (+) over control |
Reference |
N2O |
CO2 |
CH4 |
*AWD-alternate wetting and drying; **CF-continuous flooding.
|
Wheat straw |
Maize |
450 |
20 |
+5.60 |
−2.43 |
+21.67 |
115
|
40 |
+4.76 |
−12.57 |
+23.33 |
Maize straw |
Maize |
450–500 |
15 |
+58.15 |
+22.08 |
−87.27 |
158
|
30 |
+79.15 |
+21.53 |
−103 |
45 |
+76.49 |
+30.97 |
−74.54 |
Wheat straw |
Wheat |
500 |
20 |
+19.55 |
— |
+11.19 |
126
|
40 |
+26.35 |
— |
+17.45 |
Wheat straw |
Wheat |
— |
3.75 |
+0.99 |
— |
+32.65 |
21
|
7.50 |
+6.40 |
— |
+25.85 |
Rice straw |
Rice |
600 |
20 |
−8.89 |
— |
+23.83 |
158
|
40 |
+26.06 |
— |
+12.54 |
Rice straw |
Rice |
500 |
AWD* + 6.65 |
+30.93 |
— |
+56.19 |
192
|
CF** + 6.65 |
+38.64 |
— |
+60.34 |
8. Biochar certification for marketability
In general, carbon sequestration via biochar production is technically feasible and can be economically viable, especially with the current development of the carbon sink economy. Carbon removal services via biochar offered through marketplaces require appropriate certification and monitoring for building the credential and authenticity. Biochar eligibility is highly dependent on the type of feedstock utilized and processing conditions employed. Biochar includes technical feasibility, scalability potential, costs, carbon permanence, verification and monitoring, as well as application benefits concerning various potential carbon reservoirs.140 Process optimization is imperative to produce an end product that meets the basic requirement for achieving the stability in carbon sequestration. Certification is envisioned for sustainable biochar production. Presently, the European Biochar Certificate (EBC) is a well-recognized voluntary industry standard in Europe. In Switzerland, the EBC certificate is mandatory for selling biochar. Based on the uses, the EBC promoted four types of biochar, viz., EBC-Feed, EBC-AgroBio, EBC-Agro and EBC-Material.143 The EBC certification encompasses a complete assessment of feedstocks and production process eligibilities, health and safety norms, sampling procedures, labeling and quality management protocols, and biochar properties. EBC has given the standard analytic procedures for the estimation of biochar properties. Hence, properties must meet the threshold criteria of the prescribed classes. Fawzy et al. (2021)143 summarized a declaration requirement and threshold of specified biochar properties for the particular classes. The EBC is the first system to provide the methodology for biochar carbon sink potential certification. Hence, the biochar incorporated in the feedstock, manures, slurry, or any other substrate in the carbon sink potential may be converted into the carbon sink certificates. The carbon sink potential is the summation of all form of emission arising from the production of biochar (factory gate to production site). Carbon sink potential certificates enable the produce to sell the carbon sink potential on the online market place. Only carbon-neutral feedstocks like residual biomass and crops that do not reduce the total C stock are allowed to be used for the certification of biochar-based carbon sinks. Biomass obtained via the destruction of forests and other natural carbon sinks are not permitted. International biochar initiative (IBI) is also a volunteer biochar certification agency in the USA. Like EBC, the IBI also set its standard for biochar certification, but IBI does not prescribe the biochar production process for certification. Hence, EBC is the only organization that provides the biochar production certifications.143 Currently, puro and carbon future are two marketplaces that provide carbon removal through biochar. Production process certification from the EBC or any other similar organization is mandatory for trading the biochar via these marketplaces. However, each marketplace has its own C removal quantification process based on the certain parameters.143
9. Conclusions and future perspectives
The available literature indicates that biochar production and application in cropland not only reduces GHG emission, but also improves soil health, crop productivity, and economic returns. Hence, it can be summarized that the sustainable production and utilization of biochar can help in achieving several SDGs. Although a lot of literature is available on biochar production and application in agriculture, there are major issues that remain unsolved, like biomass characterization for biochar production and its interaction with soil environments. Hence, a comprehensive understanding of the carbon dynamic controlling and GHG regulating mechanism of biochar under different ecosystems need to be properly examined. Furthermore, there is a need of a robust study about the impact assessment of biochar on the microbial structure in soil systems. Furthermore, the biochar impact on the non-targeted organism and its interaction with other components of ecosystems must be evaluated for a proper understanding about the biochar interaction with the soil-plant-human-animal health continuum. Despite the various claimed benefits of biochar in the agricultural and environmental sustainability, its field level uses are very low. Hence, the availability of feedstock, permanency of biochar application, economic feasibility, and demand and supply of biochar need to be evaluated for large-scale adoption. The farm-level economic and social feasibility of the production and application of biochar must be systematically studied, which may give a clear-cut idea in the future propagation of biochar technology. Field level extension functionaries need to be trained about the biochar production. Similarly, there must be awareness among the ultimate clients. In this regard, the government can play a crucial role by providing financial assistance and subsidies to the farmers for the development of community-level low energy requiring preferably renewable energy-based biochar production units, especially in south Asia and other resource-poor countries.
Data availability
All the data supported the statements given in the manuscript.
Author contributions
Subhash Babu: conceptualization; methodology; writing original draft; Raghavendra Singh: formal analysis; writing – original draft; Sanjeev Kumar: writing – review & editing; Sanjay Singh Rathore: resources; supervision; writing – review & editing; Devideen Yadav: writing – review & editing; Sanjay Kumar Yadav: writing – review & editing; Vivek Yadav: writing – review & editing; Meraj Alam Ansari: writing reviewing & editing; Anup Das: software; writing – review & editing; Gandhamanagenahalli Adireddy Rajanna: resources; writing – review & editing; Owais Ali Wani: software; writing – review & editing; Rishi Raj: writing – original draft; Dinesh Kumar Yadav: writing – original draft; Vinod Kumar Singh: writing – review & editing.
Conflicts of interest
The authors declare that they have no known competing financial interests or personal relationships that could have appeared to influence the work reported in this paper.
Acknowledgements
The authors greatly acknowledge the immense contribution of Dr Gulab Singh Yadav (Research Team Member), who conceptualized the idea and reviewed the first draft. Unfortunately, he is no longer with us, as he succumbed to COVID-19 on 19.05.2021.
References
-
Food and Agriculture Organization (FAO) of the United Nations, International Fund for Agricultural Development, United Nations Children's Fund, World Food Programme, and World Health Organization, The State of Food Security and Nutrition in the World 2017: Building Resilience for Peace and Food Security, 2017 Search PubMed.
- L. D. Burrell, F. Zehetner, N. Rampazzo, B. Wimmer and G. Soja, Long-term effects of biochar on soil physical properties, Geoderma, 2016, 282, 96–102 CrossRef CAS.
- H. Haberl, K. H. Erb, F. Krausmann, V. Gaube, A. Bondeau, C. Plutzar, S. Gingrich, W. Lucht and M. Fischer-Kowalski, Quantifying and mapping the human appropriation of net primary production in earth's terrestrial ecosystems, Proc. Natl. Acad. Sci. U. S. A., 2007, 104, 12942–12947 CrossRef CAS PubMed.
- D. Woolf, J. E. Amonette, F. A. Street-Perrott, J. Lehmann and S. Joseph, Sustainable biochar to mitigate global climate change, Nat. Commun., 2010, 1, 1–9 Search PubMed.
- S. Solomon, G. K. Plattner, R. Knutti and P. Friedlingstein, Irreversible climate change due to carbon dioxide emissions, Proc. Natl. Acad. Sci. U. S. A., 2009, 106, 1704–1709 CrossRef CAS PubMed.
- S. Babu, R. Singh, R. Avasthe, G. S. Yadav, K. Mohapatra, T. Selvan, A. Das, V. K. Singh, D. Valente and I. Petrosillo, Soil carbon dynamics in Indian Himalayan intensified organic rice-based cropping sequences, Ecol. Indic., 2020, 114, 106292 CrossRef CAS.
-
M. Boko, I. Niang, A. Nyong, A. Vogel, A. Githeko, M. Medany, B. Osman-Elasha, R. Tabo and P. Z. Yanda, Africa Climate Change 2007: Impacts, Adaptation and Vulnerability: Contribution of Working Group II to the Fourth Assessment Report of the Intergovernmental Panel on Climate Change, 2007 Search PubMed.
- A. N. Yadav, Beneficial plant-microbe interactions for agricultural sustainability, J. Appl. Biol. Biotechnol., 2021, 9, 1–4 CAS.
- D. Roberts, A global roadmap for climate change action: From COP17 in Durban to COP21 in Paris, S. Afr. J. Sci., 2016, 112, 1–3 Search PubMed.
- A. I. Osman, S. Fawzy, M. Farghali, M. El-Azazy, A. M. Elgarah, R. A. Fahim, M. A. Maksoud, A. A. Ajlan, M. Yousry, Y. Saleem and D. W. Rooney, Biochar for agronomy, animal farming, anaerobic digestion, composting, water treatment, soil remediation, construction, energy storage, and carbon sequestration: a review, Environ. Chem. Lett., 2022, 20, 2385–2485 CrossRef CAS PubMed.
- P. Chawala and H. Sandhu, Stubble burn area estimation and its impact on ambient air quality of Patiala & Ludhiana district, Punjab, India, Heliyon, 2020, 6, e03095 CrossRef PubMed.
- M. I. Abdurrahman, S. Chaki and G. Saini, Stubble burning: Effects on health & environment, regulations and management practices, Environ. Adv., 2020, 2, 100011 CrossRef.
- S. Babu, S. S. Rathore, R. Singh, S. Kumar, V. K. Singh, S. K. Yadav, V. Yadav, R. Raj, D. D. Yadav, K. Shekhawat and O. A. Wani, Exploring agricultural waste biomass for energy, food and feed production and pollution mitigation: A review, Bioresour. Technol., 2022, 360, 127566 CrossRef CAS PubMed.
- G. S. Yadav, M. Datta, S. Babu, C. Debnath and P. K. Sarkar, Growth and productivity of lowland rice (Oryza sativa) as influenced by substitution of nitrogen fertilizer by organic sources, Indian J. Agric. Sci., 2013, 83, 1–038 Search PubMed.
- S. Babu, A. Das, R. Singh, K. P. Mohapatra, S. Kumar, S. S. Rathore, S. K. Yadav, P. Yadav, M. A. Ansari, A. S. Panwar and O. A. Wani, Designing an energy efficient, economically feasible, and environmentally robust integrated farming system model for sustainable food production in the Indian Himalayas, Sustain, Food Technol., 2023, 1, 126–142 Search PubMed.
- A. I. Osman, L. Chen, M. Yang, G. Msigwa, M. Farghali, S. Fawzy, D. W. Rooney and P. S. Yap, Cost, environmental impact, and resilience of renewable energy under a changing climate: a review, Environ. Chem. Lett., 2023, 21, 741–764 CrossRef CAS.
- S. Khan, C. Chao, M. Waqas, H. P. H. Arp and Y.-G. Zhu, Sewage sludge biochar influence upon rice (Oryza sativa L) yield, metal bioaccumulation and greenhouse gas emissions from acidic paddy soil, Environ. Sci. Technol., 2013, 47, 8624–8632 CrossRef CAS PubMed.
- S. Ye, G. Zeng, H. Wu, J. Liang, C. Zhang, J. Dai, W. Xiong, B. Song, S. Wu and J. Yu, The effects of activated biochar addition on remediation efficiency of co-composting with contaminated wetland soil, Resour., Conserv. Recycl., 2019, 140, 278–285 CrossRef.
- S. Oladele, A. Adeyemo and M. Awodun, Influence of rice husk biochar and inorganic fertilizer on soil nutrients availability and rain-fed rice yield in two contrasting soils, Geoderma, 2019, 336, 1–11 CrossRef CAS.
-
C. M. Roberts, The Dissertation Journey: A Practical and Comprehensive Guide to Planning, Writing, and Defending Your Dissertation, Corwin. Press., 2010 Search PubMed.
- L. Xiang-Hong, H. Feng-Peng and Z. Xing-Chang, Effect of biochar on soil aggregates in the Loess Plateau: results from incubation experiments, Int. J. Agric. Biol., 2012, 14 Search PubMed.
- S. Das, P. Ngene, P. Norby, T. Vegge, P. E. De Jongh and D. Blanchard, All-solid-state lithium-sulfur battery based on a nanoconfined LiBH4 electrolyte, J. Electrochem. Soc., 2016, 163, A2029 CrossRef CAS.
- K. Zazai, O. Wani, A. Ali and M. Devi, Phytoremediation and carbon sequestration potential of agroforestry systems: A review, Int. J. Curr. Microbiol. Appl. Sci., 2018, 7, 2447–2457 CrossRef.
- S. Mau, I. Pletikosa and J. Wagner, Forecasting the next likely purchase events of insurance customers: A case study on the value of data-rich multichannel environments, Int. J. Bank Mark., 2018, 36, 1125–1144 CrossRef.
- L. Dunnigan, B. J. Morton, P. J. Ashman, X. Zhang and C. W. Kwong, Emission characteristics of a pyrolysis-combustion system for the co-production of biochar and bioenergy from agricultural wastes, Waste Manage., 2018, 77, 59–66 CrossRef CAS PubMed.
- Q. Yang, O. Masek, L. Zhao, H. Nan, S. Yu, J. Yin, Z. Li and X. Cao, Country-level potential of carbon sequestration and environmental benefits by utilizing crop residues for biochar implementation, Appl. Energy, 2021, 282, 116275 CrossRef CAS.
- J. Lehmann, M. C. Rillig, J. Thies, C. A. Masiello, W. C. Hockaday and D. Crowley, Biochar effects on soil biota–a review, Soil Biol. Biochem., 2011, 43, 1812–1836 CrossRef CAS.
- R. Lal, Soil carbon sequestration impacts on global climate change and food security, Sci, 2004, 304, 1623–1627 CrossRef CAS PubMed.
- R. Lal, Managing soils and ecosystems for mitigating anthropogenic carbon emissions and advancing global food security, Bio. Sci., 2010, 60, 708–721 CrossRef.
- J. Lehmann, A handful of carbon, Nature, 2007, 447, 143–144 CrossRef CAS PubMed.
- I. Hawthorne, M. S. Johnson, R. S. Jassal, T. A. Black, N. J. Grant and S. M. Smukler, Application of biochar and nitrogen influences fluxes of CO2, CH4 and N2O in a forest soil, J. Environ. Manage., 2017, 192, 203–214 CrossRef CAS PubMed.
- Z. Wang, Y. Li, S. X. Chang, J. Zhang, P. Jiang, G. Zhou and Z. Shen, Contrasting effects of bamboo leaf and its biochar on soil CO2 efflux and labile organic carbon in an intensively managed Chinese chestnut plantation, Biol. Fertil. Soils, 2014, 50, 1109–1119 CrossRef.
- H. Sun, W. Shi, M. Zhou, X. Ma and H. Zhang, Effect of biochar on nitrogen use efficiency, grain yield and amino acid content of wheat cultivated on saline soil, Plant, Soil Environ., 2019, 65, 83–89 CrossRef CAS.
- Y. Liu, M. Yang, Y. Wu, H. Wang, Y. Chen and W. Wu, Reducing CH4 and CO2 emissions from waterlogged paddy soil with biochar, J. Soils Sediments, 2011, 11, 930–939 CrossRef CAS.
- K. Karhu, T. Mattila, I. Bergström and K. Regina, Biochar addition to agricultural soil increased CH4 uptake and water holding capacity–Results from a short-term pilot field study, Agric., Ecosyst. Environ., 2011, 140, 309–313 CrossRef CAS.
- S. He, L. Ding, X. Wang, Y. Pan, H. Hu, K. Li and H. Ren, Biochar carrier application for nitrogen removal of domestic WWTPs in winter: challenges and opportunities, Appl. Microbiol. Biotechnol., 2018, 102, 9411–9418 CrossRef CAS PubMed.
- N. Borchard, M. Schirrmann, M. L. Cayuela, C. Kammann, N. Wrage-Mönnig, J. M. Estavillo, T. Fuertes-Mendizábal, G. Sigua, K. Spokas and J. A. Ippolito, Biochar, soil and land-use interactions that reduce nitrate leaching and N2O emissions: a meta-analysis, Sci. Total Environ., 2019, 651, 2354–2364 CrossRef CAS PubMed.
- G. Zhou, X. Zhou, T. Zhang, Z. Du, Y. He, X. Wang, J. Shao, Y. Cao, S. Xue and H. Wang, Biochar increased soil respiration in temperate forests but had no effects in subtropical forests, For. Ecol. Manage., 2017, 405, 339–349 CrossRef.
- C. Ji, Y. Jin, C. Li, J. Chen, D. Kong, K. Yu, S. Liu and J. Zou, Variation in soil methane release or uptake responses to biochar amendment: a separate meta-analysis, Ecosystems, 2018, 21, 1692–1705 CrossRef CAS.
- B. Hu, Y. Ai, J. Jin, T. Hayat, A. Alsaedi, L. Zhuang and X. Wang, Efficient elimination of organic and inorganic pollutants by biochar and biochar-based materials, Biochar, 2020, 2, 47–64 CrossRef.
- Y. Zhang, J. Ding, H. Wang, L. Su and C. Zhao, Biochar addition alleviate the negative effects of drought and salinity stress on soybean productivity and water use efficiency, BMC Plant Biol., 2020, 20, 1–11 CrossRef PubMed.
- H. Liao, C. Zheng, J. Long and I. Guzmán, Effects of biochar amendment on tomato rhizosphere bacterial communities and their utilization of plant-derived carbon in a calcareous soil, Geoderma, 2021, 396, 115082 CrossRef CAS.
- S.-H. Jien and C.-S. Wang, Effects of biochar on soil properties and erosion potential in a highly weathered soil, Catena, 2013, 110, 225–233 CrossRef CAS.
- T. Purakayastha, S. Kumari and H. Pathak, Characterisation, stability, and microbial effects of four biochars produced from crop residues, Geoderma, 2015, 239, 293–303 CrossRef.
- B. Dume, D. Ayele, A. Regassa and G. Barecha, Interactive effects of biochar in soil related to feedstock and pyrolysis temperature, American-Eurasian, J. Agric. Environ. Sci., 2016, 16, 442–448 CAS.
- M. Zhang, G. Cheng, H. Feng, B. Sun, Y. Zhao, H. Chen, J. Chen, M. Dyck, X. Wang and J. Zhang, Effects of straw and biochar amendments on aggregate stability, soil organic carbon, and enzyme activities in the Loess Plateau, China, Environ, Sci. Pollut. Res., 2017, 24, 10108–10120 CrossRef CAS PubMed.
- X. Liu, A. Zhang, C. Ji, S. Joseph, R. Bian, L. Li, G. Pan and J Paz-Ferreio, Biochar’s effect on crop productivity and the dependence on experimental conditions—a meta-analysis of literature data, Plant and Soil, 2013, 373(1/2), 583–594 CrossRef CAS.
- L. A. Biederman and W. S. Harpole, Biochar and its effects on plant productivity and nutrient cycling: a meta-analysis, GCB Bioenergy, 2013, 5, 202–214 CrossRef CAS.
-
A. Abukari, Z. A. Imoro, A. Z. Imoro and A. B. Duwiejuah, Sustainable use of biochar in environmental management, ed. T. Otsuki, IntechOpen, 2021, vol. 8, DOI:10.5772/intechopen.96510.
- J. Lehmann, J. Gaunt and M. Rondon, Biochar sequestration in terrestrial ecosystems–a review, Mitig. Adapt. Strateg. Glob. Chang, 2006, 11, 403–427 CrossRef.
- K. Paustian, J. Lehmann, S. Ogle, D. Reay, G. P. Robertson and P. Smith, Climate-smart soils, Nature, 2016, 532, 49–57 CrossRef CAS PubMed.
- A. Saini, N. K. Aggarwal, A. Sharma, M. Kaur and A. Yadav, Utility potential of Parthenium hysterophorus for its strategic management, Adv. Agric., 2014, 2014, 381859 Search PubMed.
- Y. Liu, Y. Bi, Y. Xie, X. Zhao, D. He, S. Wang, C. Wang, T. Guo and G. Xing, Successive straw biochar amendments reduce nitrous oxide emissions but do not improve the net ecosystem economic benefit in an alkaline sandy loam under a wheat–maize cropping system, Land Degrad. Dev., 2020, 31, 868–883 CrossRef.
- Y. Luo, Q. Lin, M. Durenkamp, A. Dungait and P. Brookes, Soil priming effects following substrates addition to biochar-treated soils after 431 days of pre-incubation, Biol. Fertil. Soils, 2017, 53, 315–326 CrossRef CAS.
- M. Ventura, G. Alberti, P. Panzacchi, G. Delle Vedove, F. Miglietta and G. Tonon, Biochar mineralization and priming effect in a poplar short rotation coppice from a 3-year field experiment, Biol. Fertil. Soils, 2019, 55, 67–78 CrossRef CAS.
- M. Kolton, E. R. Graber, L. Tsehansky, Y. Elad and E. Cytryn, Biochar-stimulated plant performance is strongly linked to microbial diversity and metabolic potential in the rhizosphere, New Phytol., 2017, 213, 1393–1404 CrossRef CAS PubMed.
- M. K. Awasthi, Y. Duan, S. K. Awasthi, T. Liu, H. Chen, A. Pandey, Z. Zhang and M. J. Taherzadeh, Emerging applications of biochar: Improving pig manure composting and attenuation of heavy metal mobility in mature compost, J. Hazard. Mater., 2020, 389, 122116 CrossRef CAS PubMed.
- S. Shao, Y. Zhao, W. Zhang, G. Hu, H. Xie, J. Yan, S. Han, H. He and X. Zhang, Linkage of microbial residue dynamics with soil organic carbon accumulation during subtropical forest succession, Soil Biol. Biochem., 2017, 114, 114–120 CrossRef CAS.
- D. A. Laird, The charcoal vision: a win–win–win scenario for simultaneously producing bioenergy, permanently sequestering carbon, while improving soil and water quality, Agron. J., 2008, 100, 178–181 CrossRef.
- S. Varjani, G. Kumar and E. R. Rene, Developments in biochar application for pesticide remediation: current knowledge and future research directions, J. Environ. Manage., 2019, 232, 505–513 CrossRef CAS PubMed.
- D. Datta and S. Saxena, Efficient Recycling of Crop Residue through biochar for better agriculture and environment, Indian Farming, 2017, 67(4), 17–19 Search PubMed.
- R. Gurav, S. K. Bhatia, T.-R. Choi, Y. L. Park, J. Y. Park, Y. H. Han, G. Vyavahare, J. Jadhav, H. S. Song and P. Yang, Treatment of furazolidone contaminated water using banana pseudostem biochar engineered with facile synthesized magnetic nanocomposites, Bioresour. Technol., 2020, 297, 122472 CrossRef CAS PubMed.
- S. Pang, Advances in thermochemical conversion of woody biomass to energy, fuels and chemicals, Biotechnol. Adv., 2019, 37, 589–597 CrossRef CAS PubMed.
- J. I. Osayi, S. Iyuke and S. E. Ogbeide, Biocrude production through pyrolysis of used tyres, J. Catal., 2014, 2014, 386371 Search PubMed.
- J. A. Libra, K. S. Ro, C. Kammann, A. Funke, N. D. Berge, Y. Neubauer, M. M. Titirici, C. Fühner, O. Bens and J. Kern, Hydrothermal carbonization of biomass residuals: a comparative review of the chemistry, processes and applications of wet and dry pyrolysis, Biofuels, 2011, 2, 71–106 CrossRef CAS.
- S. Joseph, C. Peacocke, J. Lehmann and P. Munroe, Developing a biochar classification and test methods, Biochar for environmental management, Sci. Technol., 2009, 1, 107–126 Search PubMed.
- M. Ahmad, S. S. Lee, X. Dou, D. Mohan, J.-K. Sung, J. E. Yang and Y. S. Ok, Effects of pyrolysis temperature on soybean stover-and peanut shell-derived biochar properties and TCE adsorption in water, Bioresour. Technol., 2012, 118, 536–544 CrossRef CAS PubMed.
- J. Mumme, L. Eckervogt, J. Pielert, M. Diakité, F. Rupp and J. Kern, Hydrothermal carbonization of anaerobically digested maize silage, Bioresour. Technol., 2011, 102, 9255–9260 CrossRef CAS PubMed.
- M. Buttmann, Climate friendly coal from hydrothermal carbonization of biomass, Chem. Ing. Tech., 2011, 83, 1890–1896 CrossRef CAS.
- J. Lee, K. Lee, D. Sohn, Y. M. Kim and K. Y. Park, Hydrothermal carbonization of lipid extracted algae for hydrochar production and feasibility of using hydrochar as a solid fuel, Energy, 2018, 153, 913–920 CrossRef CAS.
- G. Gascó, J. Paz-Ferreiro, M. L. Álvarez, A. Saa and A. Méndez, Biochars and hydrochars prepared by pyrolysis and hydrothermal carbonisation of pig manure, Waste Manage., 2018, 79, 395–403 CrossRef PubMed.
- B. M. Ghanim, D. S. Pandey, W. Kwapinski and J. J. Leahy, Hydrothermal carbonisation of poultry litter: effects of treatment temperature and residence time on yields and chemical properties of hydrochars, Bioresour. Technol., 2016, 216, 373–380 CrossRef CAS PubMed.
- M. Lucian, M. Volpe, L. Gao, G. Piro, J. L. Goldfarb and L. Fiori, Impact of hydrothermal carbonization conditions on the formation of hydrochars and secondary chars from the organic fraction of municipal solid waste, Fuel, 2018, 233, 257–268 CrossRef CAS.
- M. Volpe, J. L. Goldfarb and L. Fiori, Hydrothermal carbonization of Opuntia ficus-indica cladodes: Role of process parameters on hydrochar properties, Bioresour. Technol., 2018, 247, 310–318 CrossRef CAS PubMed.
- C. Gopu, L. Gao, M. Volpe, L. Fiori and J. L. Goldfarb, Valorizing municipal solid waste: Waste to energy and activated carbons for water treatment via pyrolysis, J. Anal. Appl. Pyrolysis, 2018, 133, 48–58 CrossRef CAS.
- B. A. Oni, O. Oziegbe and O. O. Olawole, Significance of biochar application to the environment and economy, Ann. Agric. Sci., 2019, 64, 222–236 CrossRef.
- A. Tomczyk, Z. Sokołowska and P. Boguta, Biochar physicochemical properties: pyrolysis temperature and feedstock kind effects, Rev. Environ, Sci. Biotechnol., 2020, 19, 191–215 CrossRef CAS.
- E. Yeboah, G. Asamoah, P. Ofori, B. Amoah and K. O. A. Agyeman, Method of biochar application affects growth, yield and nutrient uptake of cowpea, Open Agric., 2020, 5, 352–360 CrossRef.
- S. Jeffery, D. Abalos, M. Prodana, A. C. Bastos, J. W. Van Groenigen, B. A. Hungate and F. Verheijen, Biochar boosts tropical but not temperate crop yields, Environ. Res. Lett., 2017, 12, 053001 CrossRef.
- S. Sahota, V. K. Vijay, P. Subbarao, R. Chandra, P. Ghosh, G. Shah, R. Kapoor, V. Vijay, V. Koutu and I. S. Thakur, Characterization of leaf waste-based biochar for cost effective hydrogen sulphide removal from biogas, Bioresour. Technol., 2018, 250, 635–641 CrossRef CAS PubMed.
- M. Hussain, M. Farooq, A. Nawaz, A. M. Al-Sadi, Z. M. Solaiman, S. S. Alghamdi, U. Ammara, Y. S. Ok and K. H. Siddique, Biochar for crop production: potential benefits and risks, J. Soils Sediments, 2017, 17, 685–716 CrossRef CAS.
- L.-M. Raboin, A. H. D. Razafimahafaly, M. B. Rabenjarisoa, B. Rabary, J. Dusserre and T. Becquer, Improving the fertility of tropical acid soils: Liming versus biochar application? A long term comparison in the highlands of Madagascar, Field Crops Res., 2016, 199, 99–108 CrossRef.
- V. Vijay, S. Shreedhar, K. Adlak, S. Payyanad, V. Sreedharan, G. Gopi, T. Sophia van der Voort, P. Malarvizhi, S. Yi and J. Gebert, Review of large-scale biochar field-trials for soil amendment and the observed influences on crop yield variations, Front. Energy Res., 2021, 499 Search PubMed.
- N. Hagemann, S. Joseph, H.-P. Schmidt, C. I. Kammann, J. Harter, T. Borch, R. B. Young, K. Varga, S. Taherymoosavi and K. W. Elliott, Organic coating on biochar explains its nutrient retention and stimulation of soil fertility, Nat. Commun., 2017, 8, 1–11 CrossRef CAS PubMed.
- G. Bonanomi, F. Ippolito, G. Cesarano, B. Nanni, N. Lombardi, A. Rita, A. Saracino and F. Scala, Biochar as plant growth promoter: better off alone or mixed with organic amendments?, Front. Plant Sci., 2017, 8, 1570 CrossRef PubMed.
- G. Agegnehu, A. M. Bass, P. N. Nelson and M. I. Bird, Benefits of biochar, compost and biochar-compost for soil quality, maize yield and greenhouse gas emissions in a tropical agricultural soil, Sci. Total Environ., 2016, 543, 295–306 CrossRef CAS PubMed.
- D. Trupiano, C. Cocozza, S. Baronti, C. Amendola, F. P. Vaccari, G. Lustrato, S. Di Lonardo, F. Fantasma, R. Tognetti and G. S. Scippa, The effects of biochar and its combination with compost on lettuce (Lactuca sativa L.) growth, soil properties, and soil microbial activity and abundance, Int. J. Agron., 2017, 3158207 Search PubMed.
- S. Ali, M. Rizwan, M. F. Qayyum, Y. S. Ok, M. Ibrahim, M. Riaz, M. S. Arif, F. Hafeez, M. I. Al-Wabel and A. N. Shahzad, Biochar soil amendment on alleviation of drought and salt stress in plants: a critical review, Environ. Sci. Pollut. Res., 2017, 24, 12700–12712 CrossRef CAS PubMed.
- S. Mansoor, N. Kour, S. Manhas, S. Zahid, O. A. Wani, V. Sharma, L. Wijaya, M. N. Alyemeni, A. A. Alsahli and H. A. El-Serehy, Biochar as a tool for effective management of drought and heavy metal toxicity, Chemosphere, 2020, 129458 Search PubMed.
- A. R. S. Langeroodi, E. Campiglia, R. Mancinelli and E. Radicetti, Can biochar improve pumpkin productivity and its physiological characteristics under reduced irrigation regimes?, Sci. Hortic., 2019, 247, 195–204 CrossRef.
- M. Rasool, A. Akhter, G. Soja and M. S. Haider, Role of biochar, compost and plant growth promoting rhizobacteria in the management of tomato early blight disease, Sci. Rep., 2021, 11, 1–16 CrossRef PubMed.
- S. C. Thomas, S. Frye, N. Gale, M. Garmon, R. Launchbury, N. Machado, S. Melamed, J. Murray, A. Petroff and C. Winsborough, Biochar mitigates negative effects of salt additions on two herbaceous plant species, J. Environ. Manage., 2013, 129, 62–68 CrossRef CAS PubMed.
- M. Rizwan, S. Ali, M. F. Qayyum, M. Ibrahim, M. Zia-ur-Rehman, T. Abbas and Y. S. Ok, Mechanisms of biochar-mediated alleviation of toxicity of trace elements in plants: a critical review, Environ. Sci. Pollut. Res., 2016, 23, 2230–2248 CrossRef CAS PubMed.
- G. Agegnehu, A. M. Bass, P. N. Nelson, B. Muirhead, G. Wright and M. I. Bird, Biochar and biochar-compost as soil amendments: effects on peanut yield, soil properties and greenhouse gas emissions in tropical North Queensland, Australia, Agric., Ecosyst. Environ., 2015, 213, 72–85 CrossRef CAS.
- M. Z. Alam, L. Carpenter-Boggs, M. A. Hoque and G. J. Ahammed, Effect of soil amendments on antioxidant activity and photosynthetic pigments in pea crops grown in arsenic contaminated soil, Heliyon, 2020, 6, e05475 CrossRef CAS PubMed.
- A. K. Jaiswal, Y. Elad, I. Paudel, E. R. Graber, E. Cytryn and O. Frenkel, Linking the belowground microbial composition, diversity and activity to soilborne disease suppression and growth promotion of tomato amended with biochar, Sci. Rep., 2017, 7, 1–17 CrossRef PubMed.
- Y. Elad, E. Cytryn, Y. M. Harel, B. Lew and E. R. Graber, The biochar effect: plant resistance to biotic stresses, Phytopathol. Mediterr., 2011, 50, 335–349 Search PubMed.
- J. Poveda, Á. M. Gómez, C. Fenoll and C. Escobar, The use of biochar for plant-pathogen control, Phytopathology, 2021, 111, 1490–1499 CrossRef CAS PubMed.
- R. Lal, Sequestering carbon and increasing productivity by conservation agriculture, J. Soil Water Conserv., 2015, 70, 55A–62A CrossRef.
- G. Agegnehu, A. Srivastava and M. I. Bird, The role of biochar and biochar-compost in improving soil quality and crop performance: A review, Appl. Soil Ecol., 2017, 119, 156–170 CrossRef.
- J. A. Antonangelo, X. Sun and H. Zhang, The roles of co-composted biochar (COMBI) in improving soil quality, crop productivity, and toxic metal amelioration, J. Environ. Manage., 2021, 277, 111443 CrossRef CAS PubMed.
- T. E. Lychuk, R. C. Izaurralde, R. L. Hill, W. B. McGill and J. R. Williams, Biochar as a global change adaptation: predicting biochar impacts on crop productivity and soil quality for a tropical soil with the Environmental Policy Integrated Climate (EPIC) model, Mitig. Adapt. Strateg. Glob. Change, 2015, 20, 1437–1458 CrossRef.
- A. Crane-Droesch, S. Abiven, S. Jeffery and M. S. Torn, Heterogeneous global crop yield response to biochar: a meta-regression analysis, Environ. Res. Lett., 2013, 8, 044049 CrossRef.
- M. Brtnicky, R. Datta, J. Holatko, L. Bielska, Z. M. Gusiatin, J. Kucerik, T. Hammerschmiedt, S. Danish, M. Radziemska and L. Mravcova, A critical review of the possible adverse effects of biochar in the soil environment, Sci. Total Environ., 2021, 148756 CrossRef CAS PubMed.
- Y. Zhang, J. Wang and Y. Feng, The effects of biochar addition on soil physicochemical properties: A review, Catena, 2021, 202, 105284 CrossRef CAS.
- P. R. Quin, A. Cowie, R. Flavel, B. Keen, L. Macdonald, S. Morris, B. P. Singh, I. Young and L. Van Zwieten, Oil mallee biochar improves soil structural properties—A study with x-ray micro-CT, Agric. Ecosyst. Environ., 2014, 191, 142–149 CrossRef CAS.
- J. Ulyett, R. Sakrabani, M. Kibblewhite and M. Hann, Impact of biochar addition on water retention, nitrification and carbon dioxide evolution from two sandy loam soils, Eur. J. Soil Sci., 2014, 65, 96–104 CrossRef CAS.
- C. Steiner, W. G. Teixeira, J. Lehmann, T. Nehls, J. L. V. de Macêdo, W. E. Blum and W. Zech, Long term effects of manure, charcoal and mineral fertilization on crop production and fertility on a highly weathered Central Amazonian upland soil, Plant Soil, 2007, 291, 275–290 CrossRef CAS.
- P. G. Oguntunde, B. J. Abiodun, A. E. Ajayi and N. van de Giesen, Effects of charcoal production on soil physical properties in Ghana, J. Plant Nutr. Soil Sci., 2008, 171, 591–596 CrossRef CAS.
- L. Ouyang, F. Wang, J. Tang, L. Yu and R. Zhang, Effects of biochar amendment on soil aggregates and hydraulic properties, J. Soil Sci. Plant Nutr., 2013, 13, 991–1002 Search PubMed.
- H. Dokoohaki, F. E. Miguez, D. Laird, R. Horton and A. S. Basso, Assessing the biochar effects on selected physical properties of a sandy soil: an analytical approach, comm, Soil Sci. Plant Anal., 2017, 48, 1387–1398 CrossRef CAS.
- K. Rasa, J. Heikkinen, M. Hannula, K. Arstila, S. Kulju and J. Hyväluoma, How and why does willow biochar increase a clay soil water retention capacity?, Biomass Bioenergy, 2018, 119, 346–353 CrossRef CAS.
- F. G. Verheijen, A. Zhuravel, F. C. Silva, A. Amaro, M. Ben-Hur and J. J. Keizer, The influence of biochar particle size and concentration on bulk density and maximum water holding capacity of sandy vs. sandy loam soil in a column experiment, Geoderma, 2019, 347, 194–202 CrossRef.
-
C. A. Masiello, B. Dugan, C. E. Brewer, K. A. Spokas, J. M. Novak, Z. Liu and G. Sorrenti, in Biochar for Environmental Management, Routledge, 2015, pp. 575–594 Search PubMed.
- J. Zhang, C. Qun and Y. Changfu, Biochar effect on water evaporation and hydraulic conductivity in sandy soil, Pedosphere, 2016, 26, 265–272 CrossRef.
- A. Singh, A. Singh and T. Purakayastha, Characterization of biochar and their influence on microbial activities and potassium availability in an acid soil, Arch. Agron. Soil Sci., 2019, 65, 1302–1315 CrossRef CAS.
- S. Babu, K. Mohapatra, A. Das, G. S. Yadav, M. Tahasildar, R. Singh, A. Panwar, V. Yadav and P. Chandra, Designing energy-efficient, economically sustainable and environmentally safe cropping system for the rainfed maize–fallow land of the Eastern Himalayas, Sci. Total Environ., 2020, 722, 137874 CrossRef CAS PubMed.
- K. Zygourakis, Biochar soil amendments for increased crop yields: how to design a “designer” biochar, AIChE J., 2017, 63, 5425–5437 CrossRef CAS.
- F. Liang, G.-t. LI, Q.-m. LIN and X.-r. ZHAO, Crop yield and soil properties in the first 3 years after biochar application to a calcareous soil, J. Integr. Agric., 2014, 13, 525–532 CrossRef CAS.
- R. Chintala, T. E. Schumacher, L. M. McDonald, D. E. Clay, D. D. Malo, S. K. Papiernik, S. A. Clay and J. L. Julson, Phosphorus Sorption and Availability from Biochars and Soil/Biochar Mixtures, Clean: Soil, Air, Water, 2014, 42, 626–634 CAS.
- J. M. Novak, J. A. Ippolito, D. W. Watts, G. C. Sigua, T. F. Ducey and M. G. Johnson, Biochar compost blends facilitate switchgrass growth in mine soils by reducing Cd and Zn bioavailability, Biochar, 2019, 1, 97–114 CrossRef PubMed.
- R. Radin, R. A. Bakar, C. F. Ishak, S. H. Ahmad and L. C. Tsong, Biochar-compost mixture as amendment for improvement of polybag-growing media and oil palm seedlings at main nursery stage, Int. J. Recycl. Org. Waste Agric., 2018, 7, 11–23 CrossRef.
- H. Chen, S. K. Awasthi, T. Liu, Y. Duan, Z. Zhang and M. K. Awasthi, Compost biochar application to contaminated soil reduces the (im) mobilization and phytoavailability of lead and copper, J. Chem. Technol. Biotechnol., 2020, 95, 408–417 CrossRef CAS.
- A. Rodríguez-Vila, R. Forján, R. S. Guedes and E. F. Covelo, Changes on the phytoavailability of nutrients in a mine soil reclaimed with compost and biochar, Water, Air, Soil Pollut., 2016, 227, 1–12 CrossRef.
- A. Taghizadeh-Toosi, T. J. Clough, R. R. Sherlock and L. M. Condron, Biochar adsorbed ammonia is bioavailable, Plant Soil, 2012, 350, 57–69 CrossRef CAS.
- B. Wu, G. Cheng, K. Jiao, W. Shi, C. Wang and H. Xu, Mycoextraction by Clitocybe maxima combined with metal immobilization by biochar and activated carbon in an aged soil, Sci. Total Environ., 2016, 562, 732–739 CrossRef CAS PubMed.
- C. Nzediegwu, S. Prasher, E. Elsayed, J. Dhiman, A. Mawof and R. Patel, Effect of biochar on heavy metal accumulation in potatoes from wastewater irrigation, J. Environ. Manage., 2019, 232, 153–164 CrossRef CAS PubMed.
- Z. D. Nejad, M. C. Jung and K.-H. Kim, Remediation of soils contaminated with heavy metals with an emphasis on immobilization technology, Environ. Geochem. Health, 2018, 40, 927–953 CrossRef PubMed.
- H. Liu, S. Guo, K. Jiao, J. Hou, H. Xie and H. Xu, Bioremediation of soils co-contaminated with heavy metals and 2, 4, 5-trichlorophenol by fruiting body of Clitocybe maxima, J. Hazard. Mater., 2015, 294, 121–127 CrossRef CAS PubMed.
- X. Zhang, H. Wang, L. He, K. Lu, A. Sarmah, J. Li, N. S. Bolan, J. Pei and H. Huang, Using biochar for remediation of soils contaminated with heavy metals and organic pollutants, Environ. Sci. Pollut. Res., 2013, 20, 8472–8483 CrossRef CAS PubMed.
- K. Mackie, S. Marhan, F. Ditterich, H. Schmidt and E. Kandeler, The effects of biochar and compost amendments on copper immobilization and soil microorganisms in a temperate vineyard, Agric., Ecosyst. Environ., 2015, 201, 58–69 CrossRef CAS.
- X. Song, M. Liu, D. Wu, B. S. Griffiths, J. Jiao, H. Li and F. Hu, Interaction matters: Synergy between vermicompost and PGPR agents improves soil quality, crop quality and crop yield in the field, Appl. Soil Ecol., 2015, 89, 25–34 CrossRef.
- M. A. Rondon, J. Lehmann, J. Ramírez and M. Hurtado, Biological nitrogen fixation by common beans (Phaseolus vulgaris L.) increases with bio-char additions, Biol. Fertil. Soils, 2007, 43, 699–708 CrossRef.
- C. Amoah-Antwi, J. Kwiatkowska-Malina, S. F. Thornton, O. Fenton, G. Malina and E. Szara, Restoration of soil quality using biochar and brown coal waste: A review, Sci. Total Environ., 2020, 722, 137852 CrossRef CAS PubMed.
- E. J. Foster, N. Hansen, M. Wallenstein and M. F. Cotrufo, Biochar and manure amendments impact soil nutrients and microbial enzymatic activities in a semi-arid irrigated maize cropping system, Agric., Ecosyst. Environ., 2016, 233, 404–414 CrossRef.
- R. Sedjo and B. Sohngen, Carbon sequestration in forests and soils, Annu. Rev. Resour. Econ., 2012, 4, 127–144 CrossRef.
-
G. Venkatesh, K. Gopinath, K. S. Reddy, B. S. Reddy, J. Prasad, G. R. Rao, G. Pratibha, C. Srinivasarao, G. R. Chary and M. Prabhakar, Biochar Production and its Use in Rainfed Agriculture: Experiences from CRIDA, 2018 Search PubMed.
- B. Glaser, J. Lehmann and W. Zech, Ameliorating physical and chemical properties of highly weathered soils in the tropics with charcoal–a review, Biol. Fertil. Soils, 2002, 35, 219–230 CrossRef CAS.
- E. Yeboah, P. Ofori, G. Quansah and S. Sohi, Improving soil productivity through biochar amendments to soils, Afr. J. Environ. Sci., 2009, 3, 34–41 CAS.
- S. Fawzy, A. I. Osman, N. Mehta, D. Moran, H. Ala'a and D. W. Rooney, Atmospheric carbon removal via industrial biochar systems: A techno-economic-environmental study, J. Cleaner Prod., 2022, 371, 133660 CrossRef CAS.
- Q. Yang, O. Mašek, L. Zhao, H. Nan, S. Yu, J. Yin, Z. Li and X. Cao, Country-level potential of carbon sequestration and environmental benefits by utilizing crop residues for biochar implementation, Appl. Energy, 2021, 282, 116275 CrossRef CAS.
- E. Muñoz, G. Curaqueo, M. Cea, L. Vera and R. Navia, Environmental hotspots in the life cycle of a biochar-soil system, J. Cleaner Prod., 2017, 158, 1–7 CrossRef.
- S. Fawzy, A. I. Osman, H. Yang, J. Doran and D. W. Rooney, Industrial biochar systems for atmospheric carbon removal: a review, Environ. Chem. Lett., 2021, 19, 3023–3055 CrossRef CAS.
- E. Bruun, D. Müller-Stöver, P. Ambus and H. Hauggaard-Nielsen, Application of biochar to soil and N2O emissions: potential effects of blending fast-pyrolysis biochar with anaerobically digested slurry, Eur. J. Soil Sci., 2011, 62, 581–589 CrossRef CAS.
- O. Mašek, W. Buss, P. Brownsort, M. Rovere, A. Tagliaferro, L. Zhao, X. Cao and G. Xu, Potassium doping increases biochar carbon sequestration potential by 45%, facilitating decoupling of carbon sequestration from soil improvement, Sci. Rep., 2019, 9, 1–8 CrossRef PubMed.
-
Biochar for Environmental Management: Science, Technology and Implementation, ed. J. Lehmann and S. Joseph, Routledge, 2015 Search PubMed.
- N. Ameloot, E. R. Graber, F. G. Verheijen and S. De Neve, Interactions between biochar stability and soil organisms: review and research needs, Eur. J. Soil Sci., 2013, 64, 379–390 CrossRef CAS.
- V. Yadav, T. Karak, S. Singh, A. K. Singh and P. Khare, Benefits of biochar over other organic amendments: Responses for plant productivity (Pelargonium graveolens L.) and nitrogen and phosphorus losses, Ind. Crops Prod., 2019, 131, 96–105 CrossRef CAS.
- M. A. Naeem, M. Khalid, M. Arshad and R. Ahmad, Yield and nutrient composition of biochar produced from different feedstocks at varying pyrolytic temperatures, Pak. J. Agric. Sci., 2014, 51 Search PubMed.
- B. Dume, G. Berecha and S. Tulu, Characterization of biochar produced at different temperatures and its effect on acidic nitosol of Jimma, Southwest Ethiopia, Int. J. Soil Sci., 2015, 10, 63 CrossRef.
- K. Crombie, O. Mašek, S. P. Sohi, P. Brownsort and A. Cross, The effect of pyrolysis conditions on biochar stability as determined by three methods, GCB Bioenergy, 2013, 5, 122–131 CrossRef CAS.
-
C. Srinivasarao, K. Gopinath, G. Venkatesh, A. Dubey, H. Wakudkar, T. Purakayastha, H. Pathak, P. Jha, B. Lakaria and D. Rajkhowa, Use of Biochar for Soil Health Enhancement and Greenhouse Gas Mitigation in India, Potential Constraints, 2013 Search PubMed.
- L. Van Zwieten, S. Kimber, S. Morris, K. Chan, A. Downie, J. Rust, S. Joseph and A. Cowie, Effects of biochar from slow pyrolysis of papermill waste on agronomic performance and soil fertility, Plant Soil, 2010, 327, 235–246 CrossRef CAS.
- A. Anand, V. Kumar and P. Kaushal, Biochar and its twin benefits: Crop residue management and climate change mitigation in India, Renewable Sustainable Energy Rev., 2022, 156, 111959 CrossRef CAS.
-
D. K. Gupta, C. K. Gupta, R. Dubey, R. K. Fagodiya, G. Sharma, N. Mohamed, R. Dev and A. Shukla, in Biochar Applications in Agriculture and Environment Management, Springer, 2020, pp. 141–165 Search PubMed.
- Z. H. Weng, L. Van Zwieten, B. P. Singh, E. Tavakkoli, S. Joseph, L. M. Macdonald, T. J. Rose, M. T. Rose, S. W. Kimber and S. Morris, Biochar built soil carbon over a decade by stabilizing rhizodeposits, Nat. Clim. Change, 2017, 7, 371–376 CrossRef CAS.
- D. Lefebvre, A. Williams, J. Meersmans, G. J. Kirk, S. Sohi, P. Goglio and P. Smith, Modelling the potential for soil carbon sequestration using biochar from sugarcane residues in Brazil, Sci. Rep., 2020, 10, 1–11 CrossRef PubMed.
- W. Yang, G. Feng, D. Miles, L. Gao, Y. Jia, C. Li and Z. Qu, Impact of biochar on greenhouse gas emissions and soil carbon sequestration in corn grown under drip irrigation with mulching, Sci. Total Environ., 2020, 729, 138752 CrossRef CAS PubMed.
- J. Layek, R. Narzari, S. Hazarika, A. Das, K. Rangappa, S. Devi, A. Balusamy, S. Saha, S. Mandal, R. G. Idapuganti, S. Babu, B. U. Choudhary and V. K. Mishra, Prospects of biochar for sustainable agriculture and carbon sequestration: an overview for Eastern Himalayas., Sustainability, 2022, 14(11), 6684–1822 CrossRef CAS.
-
K. Priyadarshani and R. Prabhune, Biochar for Carbon Reduction, Sustainable Agriculture and Soil Management (BiocharM), Final Report APN Project. J., 2009 Search PubMed.
- A. Cross and S. P. Sohi, The priming potential of biochar products in relation to labile carbon contents and soil organic matter status, Soil Biol. Biochem., 2011, 43, 2127–2134 CrossRef CAS.
- T. DeLuca, M. MacKenzie, M. Gundale and W. Holben, Wildfire-produced charcoal directly influences nitrogen cycling in ponderosa pine forests, Soil Sci. Soc. Am. J., 2006, 70, 448–453 CrossRef CAS.
- V. Nelissen, B. K. Saha, G. Ruysschaert and P. Boeckx, Effect of different biochar and fertilizer types on N2O and NO emissions, Soil Biol. Biochem., 2014, 70, 244–255 CrossRef CAS.
- C. Bamminger, N. Zaiser, P. Zinsser, M. Lamers, C. Kammann and S. Marhan, Effects of biochar, earthworms, and litter addition on soil microbial activity and abundance in a temperate agricultural soil, Biol. Fertil. Soils, 2014, 50, 1189–1200 CrossRef CAS.
- Y. Yanai, K. Toyota and M. Okazaki, Effects of charcoal addition on N2O emissions from soil resulting from rewetting air-dried soil in short-term laboratory experiments, Soil Sci. Plant Nutr., 2007, 53, 181–188 CrossRef CAS.
-
M. Rondon, J. Ramirez and J. Lehmann, Charcoal additions reduce net emissions of greenhouse gases to the atmosphere, Proceedings of the 3rd USDA Symposium on Greenhouse Gases and Carbon Sequestration in Agriculture and Forestry, USDA Baltimore, 2005, pp. 21–24 Search PubMed.
- M. Huang, X. Yin, J. Chen and F. Cao, Biochar Application Mitigates the Effect of Heat Stress on Rice (Oryza sativa L.) by Regulating the Root-Zone Environment, Front. Plant Sci., 2021, 12, 711725 CrossRef PubMed.
- T. F. Abbruzzini, C. A. Davies, F. H. Toledo and C. E. Cerri, Dynamic biochar effects on nitrogen use efficiency, crop yield and soil nitrous oxide emissions during a tropical wheat-growing season, J. Environ. Manage., 2019, 252, 109638 CrossRef CAS PubMed.
- G. Cornelissen, N. L. Nurida, S. E. Hale, V. Martinsen, L. Silvani and J. Mulder, Fading positive effect of biochar on crop yield and soil acidity during five growth seasons in an Indonesian Ultisol, Sci. Total Environ., 2018, 634, 561–568 CrossRef CAS PubMed.
- E. Calys-Tagoe, A. Sadick, E. Yeboah and B. Amoah, Biochar effect on maize yield in selected farmers fields in the northern and upper east regions of Ghana, J. Exp. Agric. Int., 2019, 30, 1–9 CrossRef.
- T. Kätterer, D. Roobroeck, O. Andrén, G. Kimutai, E. Karltun, H. Kirchmann, G. Nyberg, B. Vanlauwe and K. R. de Nowina, Biochar addition persistently increased soil fertility and yields in maize-soybean rotations over 10 years in sub-humid regions of Kenya, Field Crops Res., 2019, 235, 18–26 CrossRef.
- T. Berihun, S. Tolosa, M. Tadele and F. Kebede, Effect of biochar application on growth of garden pea (Pisum sativum L.) in acidic soils of Bule Woreda Gedeo Zone Southern Ethiopia, Int. J. Agron., 2017, 2017, 6827323 Search PubMed.
- I. C. Silva, L. A. Fernandes, F. Colen and R. A. Sampaio, Growth and production of common bean fertilized with biochar, Cienc. Rural, 2017, 47(11) DOI:10.1590/0103-8478cr20170220.
- N. R. Pandit, J. Mulder, S. E. Hale, V. Martinsen, H. P. Schmidt and G. Cornelissen, Biochar improves maize growth by alleviation of nutrient stress in a moderately acidic low-input Nepalese soil, Sci. Total Environ., 2018, 625, 1380–1389 CrossRef CAS PubMed.
- M. F. Seleiman, Y. Refay, N. Al-Suhaibani, I. Al-Ashkar, S. El-Hendaw and E. M. Hafez, Integrative effects of rice-straw biochar and silicon on oil and seed quality, yield and physiological traits of Helianthus annuus L. grown under water deficit stress, Agron, 2019, 9, 637 CrossRef CAS.
- T. S. Roy, M. T. Rahaman, R. Chakraborty, M. Mostofa and M. S. Rahaman, Effect of Biochar Application as a Soil Amendment on Growth and Yield of Sesame (Sesamum indicum l.), Bangladesh Agron. J., 2019, 22, 113–127 CrossRef.
- Z. M. Solaiman, M. I. Shafi, E. Beamont and H. M. Anawar, Poultry litter biochar increases mycorrhizal colonisation, soil fertility and cucumber yield in a fertigation system on sandy soil, Agriculture, 2020, 10, 480 CrossRef CAS.
- H. P. Schmidt, B. H. Pandit, V. Martinsen, G. Cornelissen, P. Conte and C. I. Kammann, Fourfold increase in pumpkin yield in response to low-dosage root zone application of urine-enhanced biochar to a fertile tropical soil, Agriculture, 2015, 7, 723–741 CrossRef.
- D. She, X. Sun, A. H. Gamareldawla, E. A. Nazar, W. Hu, K. Edith and S. E. Yu, Benefits of soil biochar amendments to tomato growth under saline water irrigation, Sci. Rep., 2018, 8, 14743 CrossRef PubMed.
- A. O. Adekiya, T. M. Agbede, A. Olayanju, W. S. Ejue, T. A. Adekanye, T. T. Adenusi and J. F. Ayeni, Effect of biochar on soil properties, soil loss, and cocoyam yield on a tropical sandy loam Alfisol, Sci. World J., 2020, 2020 Search PubMed.
- I. Haider, M. A. Raza, R. Iqbal, M. U. Aslam, M. Habib-ur-Rahman, S. Raja, M. T. Khan, M. M. Aslam, M. Waqas and S. Ahmad, Potential effects of biochar application on mitigating the drought stress implications on wheat (Triticum aestivum L.) under various growth stages, J. Saudi Chem. Soc., 2020, 24, 974–981 CrossRef CAS.
- S. Kanwal, N. Ilyas, S. Shabir, M. Saeed, R. Gul, M. Zahoor, N. Batool and R. Mazhar, Application of biochar in mitigation of negative effects of salinity stress in wheat (Triticum aestivum L.), J. Plant Nutr., 2018, 41, 526–538 CrossRef CAS.
- Y. Hafez, K. Attia, S. Alamery, A. Ghazy, A. Al-Doss, E. Ibrahim, E. Rashwan, L. El-Maghraby, A. Awad and K. Abdelaal, Beneficial effects of biochar and chitosan on antioxidative capacity, osmolytes accumulation, and anatomical characters of water-stressed barley plants, Agronomy, 2020, 10, 630 CrossRef CAS.
- M. A. Mannan, E. Halder, M. A. Karim and J. U. Ahmed, Alleviation of adverse effect of drought stress on soybean (Glycine max. L.) by using poultry litter biochar, Bangladesh Agron. J., 2016, 19, 61–69 CrossRef.
- D. Egamberdieva, V. Shurigin, B. Alaylar, H. Ma, M. E. Müller, S. Wirth, M. Reckling and S. D. Bellingrath-Kimura, The effect of biochars and endophytic bacteria on growth and root rot disease incidence of Fusarium infested narrow-leafed lupin (Lupinus angustifolius L.), Microorganisms, 2020, 8, 496 CrossRef CAS PubMed.
- V. Parkash and S. Singh, Potential of biochar application to mitigate salinity stress in eggplant, Hortic. Sci., 2020, 55, 1946–1955 CAS.
- D. Jabborova, K. Annapurna, S. Paul, S. Kumar, H. A. Saad, S. Desouky, M. F. Ibrahim and A. Elkelish, Beneficial features of biochar and arbuscular mycorrhiza for improving spinach plant growth, root morphological traits, physiological properties, and soil enzymatic activities, J. Fungi, 2021, 7, 571 CrossRef CAS PubMed.
- M. E. Hussien Ibrahim, A. Y. Adam Ali, G. Zhou, A. M. Ibrahim Elsiddig, G. Zhu, N. E. Ahmed Nimir and I. Ahmad, Biochar application affects forage sorghum under salinity stress, Chil. J. Agric. Res., 2020, 80, 317–325 CrossRef.
- A. Abewa, B. Yitaferu, Y. G. Selassie and T. Amare, The role of biochar on acid soil reclamation and yield of Teff (Eragrostis tef [Zucc] Trotter) in North western Ethiopia, J. Agric. Sci., 2014, 6, 1 Search PubMed.
- M. Zafar-ul-Hye, M. Tahzeeb-ul-Hassan, A. Wahid, S. Danish, M. J. Khan, S. Fahad, M. Brtnicky, G. S. Hussain, M. L. Battaglia and R. Datta, Compost mixed fruits and vegetable waste biochar with ACC deaminase rhizobacteria can minimize lead stress in mint plants, Sci. Rep., 2021, 11, 1–20 CrossRef PubMed.
- A. Caroline, J. Debode, B. Vandecasteele, T. D'Hose, P. Cremelie, A. Haegeman, T. Ruttink T, P. Dawyndt and M. Maes, Biological, physicochemical and plant health responses in lettuce and strawberry in soil or peat amended with biochar, Appl. Soil Ecol., 2016, 107, 1–2 CrossRef.
- D. Pandey, A. Daverey and K. Arunachalam, Biochar: Production, properties and emerging role as a support for enzyme immobilization, J. Cleaner Prod., 2020, 255, 120267 CrossRef CAS.
|
This journal is © The Royal Society of Chemistry 2023 |
Click here to see how this site uses Cookies. View our privacy policy here.