DOI:
10.1039/D1QO00085C
(Research Article)
Org. Chem. Front., 2021,
8, 1976-1982
Visible-light-promoted cross-coupling reaction of hypervalent bis-catecholato silicon compounds with selenosulfonates or thiosulfonates†
Received
17th January 2021
, Accepted 18th February 2021
First published on 19th February 2021
Abstract
Under mild metal-free conditions, we successfully developed a visible-light-promoted free radical cross coupling of hypervalent bis-catecholato silicon compounds with selenosulfonates or thiosulfonates and efficiently constructed unsymmetrical 1°-alkyl–alkyl selenide compounds which are difficult to prepare. This protocol uses mild reaction conditions and easily available substrates, has a wide range substrate scope, high yields, and simple operation and no need of expensive photosensitive catalysts. It provides a green and simple method for the preparation of unsymmetrical alkyl–alkyl selenide compounds. At the same time, this strategy is also applicable for the efficient construction of aryl–alkyl selenides, heteroayl–alkyl selenides and aryl–alkyl sulfides.
Introduction
Selenium is an essential trace element for the human body, and it mainly exists in internal organs and blood in the form of selenoamino acids and peptides in organisms.1 Selenium is the basic component of some enzyme active parts, and affects the activity and function of enzymes. In the human body, it participates in the formation of thyroxine deiodinase, glutathione peroxidase and other proteins in the form of selenocysteine.2 In recent years, many commercial selenium supplement products have appeared on the market. Relevant studies have shown that adequate selenium supplementation is beneficial to health. In addition, organic selenium compounds are widely used in organic chemistry,3 selenium-containing biologically active molecules,4 pesticides5 and materials.6 It can be seen that the synthesis of organic selenium compounds has important practical significance. In the past few years, selenosulfonates have been widely used in the construction of organoselenide compounds as an important class of reagents and intermediates. Common strategies include transition metal-catalyzed C–Se bond construction7 (Scheme 1a, left), C–Se bond construction under metal-free catalysis8 and C–Se bond construction under photocatalysis9 (Scheme 1a, right). However, these strategies need to use expensive transition metal catalysts or additives. On the other hand, low atom economy and confined secondary alkyl–alkyl selenides scope limit their further applications. Therefore, it is of great significance to develop a simple, mild and efficient method to prepare organoselenide compounds with wide substrate scope.
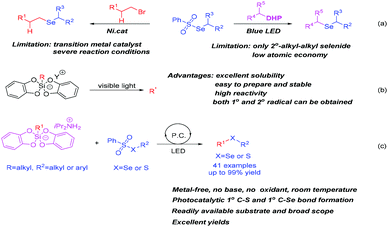 |
| Scheme 1 (a) Ni-catalyzed C–Se bond construction and C–Se bond construction under photocatalysis. (b) Hypervalent silicon compounds as a source of C-radicals. (c) Visible-light-promoted free radical cross coupling of hypervalent bis-catecholato silicon compounds with selenosulfonates or thiosulfonates. | |
In recent years, visible light-mediated photoredox catalysis has received more and more attention. Due to its special reaction mode, many transformations that could not be achieved through the transition metal catalytic process before are possible. However, finding free radical precursors with sufficient stability and functional group compatibility is a big challenge. For instance, organoborates (especially alkyl trifluoroborate),10 4-alkyl-1,4-dihydropyridines (DHPs)11 have been widely applied in the synthesis of various compounds. However, the main drawbacks are the formation of polluting boron fluoride side products and the continuing difficulty in generating primary radicals under photocatalytic conditions. Therefore, it is particularly important to find a free radical precursor that is more environmentally friendly and has high substrate universality. In recent years, hypervalent silicon compounds12 have been widely used in various free radical reaction strategies as a kind of highly active free radical precursor. Kumada et al.13 first reported the free radical reaction of organic pentafluorosilicate under copper catalysis conditions, however its poor solubility severely limited its application in organic synthesis. The hypervalent silicon derivative of bis-catechol reported by Frye's group has received widespread attention due to its more soluble properties (Scheme 1b).14 These ideal radical precursors can generate various functionalized alkyl radicals especially the primary alkyl radicals smoothly under low-energy visible light using ready-made photocatalysts, which has attracted wide attention from chemists. Prof. Molander's group15 and other groups16 have done a lot of excellent work in this area. Herein, we developed a free radical cross-coupling reaction between bis-catechol hypervalent silicon derivatives and alkylselenosulfonates under visible light mediation, and successfully constructed primary unsymmetrical alkyl–alkyl selenide compounds. This strategy is also applicable to the construction of aryl–alkyl selenides, heteroayl–alkyl selenides and aryl-alkyl sulfides (Scheme 1c). More importantly, we used this strategy to successfully construct the selenocarbamate compound 3r. This shows that the strategy has broad application prospects in the biological field.
Results and discussion
Initially, we tried the reaction of propylsilicate 1a, Se-(2-phenoxyethyl) benzenesulfonoselenoate 2d, and organometallic photocatalyst Ru(bpy)3(PF6)2 in (dry) DMF at room temperature for 14 h under a N2 atmosphere. Gratifyingly, the reaction proceeded smoothly to give the target product (2-phenoxyethyl)(propyl)selane 3d. With this promising result in hand, we tried to optimize the reaction conditions. First we investigated the ratio effects of 1a and 2a. It was found that when the ratio of 1a to 2a is 1.67
:
1.0, 3d could be obtained in 64% GC-yield (Table 1, entries 1–4). Then, we tried the reactions in several different dry solvents such as DMA, MeCN, and DMSO. It was found that when anhydrous DMSO was used, the target product 3d could be obtained in 73% GC-yield (Table 1, entries 5–7). We also investigated other different photosensitive catalysts. To our delight, 3d was obtained in 85% GC-yield when the reaction was carried out utilizing acriflavin II as the catalyst (Table 1, entries 8–12). It should be noted that the reaction could not proceed under an air atmosphere. (Table 1, entry 13). 3d was obtained in 99% isolated yield when the reaction time was prolonged to 24 h (Table 1, entry 14).
Table 1 Optimization of the reaction conditions for the preparation of unsymmetrical alkyl–alkyl selenide compoundsa,b

|
Entry |
1a (equiv.) |
2a (equiv.) |
Cat |
Solvent |
GC-yieldb |
Reaction conditions: propylsilicate 1a, Se-(2-phenoxyethyl) benzenesulfonoselenoate 2d; photosensitive catalyst (2.5 mol%), solvent(dry) (1 ml), room temperature, 14 h.
Yields were determined by GC-MS analysis using biphenyl as an internal standard, GC-yield.
Under an air atmosphere.
24 h.
Isolated yield.
|
1 |
1.00 |
2.0 |
1 |
DMF |
45% |
2 |
1.50 |
1.0 |
1 |
DMF |
56% |
3 |
1.67 |
1.0 |
1 |
DMF |
64% |
4 |
2.50 |
1.0 |
1 |
DMF |
58% |
5 |
2.00 |
1.0 |
1 |
DMA |
29% |
6 |
2.00 |
1.0 |
1 |
DMSO |
73% |
7 |
2.00 |
1.0 |
1 |
MeCN |
48% |
8 |
2.00 |
1.0 |
2 |
DMSO |
85% |
9 |
2.00 |
1.0 |
3 |
DMSO |
75% |
10 |
2.00 |
1.0 |
4 |
DMSO |
56% |
11 |
2.00 |
1.0 |
5 |
DMSO |
69% |
12 |
2.00 |
1.0 |
6 |
DMSO |
81% |
13c |
2.00 |
1.0 |
2 |
DMSO |
N.R |
14d |
2.00 |
1.0 |
2 |
DMSO |
99%e |
|
With the optimized reaction conditions in hand, we set about assessing the generality of this protocol. First, we examined the reactivity of different substituted selenosulfonates (Scheme 2). The reaction of propylsilicate 1a with Se-phenethyl benzenesulfonoselenoate 2a gave the target product 3a in 78% yield. The reaction of propylsilicate 1a with Se-(3-phenylpropyl) benzenesulfonoselenoate 2b afforded the desired product 3b in 77% yield. The reaction of propylsilicate 1a with Se-(4-phenylbutyl) benzenesulfonoselenoate 2c furnished 3c in 85% yield. To our delight, when ethyl 4-((phenylsulfonyl)selanyl)butanoate 2e was applied to the reaction, the target product 3e was obtained in 93% yield successfully, which demonstrates excellent functional group tolerance. Next, we investigated the universality of different substituted benzylselenosulfonates. It should be noted that whether it is a methyl-substituted benzylselenosulfonate 2f or an electron-withdrawing group functionalized benzylselenosulfonate 2g, or a halogen-substituted benzylselenosulfonate 2h were applied to the reactions with 1a, the desired products 3f–h could be obtained in good to excellent yields, respectively.
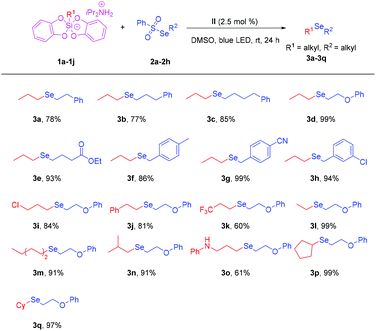 |
| Scheme 2 Substrate scope for unsymmetrical alkyl–alkyl selenide compounds. Reaction conditions: substituted silicate 1 (0.34 mmol), substituted selenosulfonates 2 (0.2 mmol), II (2.5 mol%), (dry) DMSO (1 mL), room temperature, blue LED, N2, 24 h. Isolated yields. | |
Then, we further investigated the universality of different substituted alkylsilicates (Scheme 2). The reaction showed a broad functional group compatibility, in which both halogen substituted alkylsilicate, phenyl substituted alkylsilicate and trifluoromethyl substituted alkylsilicate were well tolerated. The target products 3i–k were uniformly obtained in excellent yields. It was found that the length of the chain has no effect on the yields. The target products 3l–n were uniformly obtained in excellent yields too. Surprisingly, the secondary amine substituted alkylsilicate substrate was also well tolerated. The product 3o could be obtained in 61% yield. When cyclopentylsilicate and cyclohexylsilicate were applied to the reaction, the target products 3p and 3q were isolated in excellent yields, respectively.
Encouraged by the above achievements, we turned attention to extending the method to arylselenosulfonates. However, under the previous conditions, the reaction of propylsilicate 1a with Se-phenyl benzenesulfonoselenoate 4a failed to afford product 5a. To our satisfaction, when the reaction temperature was increased to 80 °C, it smoothly led to the target product 5a in 92% yield. With the optimal modified reaction conditions in hand, we explored the scope of different substituted alkylsilicates (Scheme 3). Similarly, the reaction showed a broad functional group compatibility. When hexylsilicate was applied to the reaction, the target product 5b could be obtained in 93% yield. When isobutylsilicate was applied to the reaction, the target product 5c could also be isolated in 58% yield. When phenethylsilicate and chloropropylsilicate were applied to the reaction. The target products 5d and 5e could be obtained in 88% and 93% yields, respectively. The secondary amine substituted alkylsilicate substrate was well tolerated and the target product 5f could be obtained in 58% yield. Subsequently, we examined the substrate scope of arylselenosulfonates (Scheme 3). It was found that when Se-phenyl arylsulfonates bore electron donating groups (4-OMe, 3-Me), the target products 5i and 5j could be obtained in 94% and 83% yields, respectively. The reaction of 1a with Se-(naphthalen-1-yl) benzenesulfonoselenoate 4c led to the desired product 5k in 94% yield. When Se-(2-chlorophenyl) benzenesulfonoselenoate 4c and Se-(pyridin-3-yl) benzenesulfonoselenoate 4d were applied to the reaction, the target products 5l and 5m could also be obtained in 67% and 58% yields, respectively. This study provides a new method for the construction of heteroaryl alkyl selenide compounds.
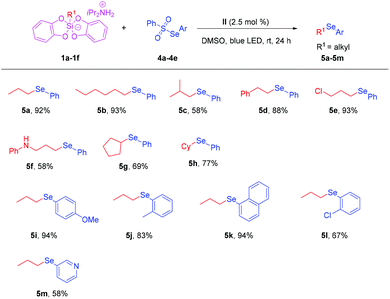 |
| Scheme 3 Substrate scope for aryl–alkyl selenides compounds. Reaction conditions: substituted silicate 1 (0.34 mmol), substituted selenosulfonates 4 (0.2 mmol), II (2.5 mol%), (dry)DMSO (1 mL), 80 °C, Blue LED, N2, 24 h. Isolated yields. | |
In order to investigate the applicability of this free radical cross-coupling strategy, we tried the reaction of alkylsilicates with thiosulfonates under similar reaction conditions (Scheme 4). It was found that the reactivity of thiosulfonates is lower than that of selenosulfonates. There are limited four substituted alkylsilicates, which were successfully applied to the reaction to afford the desired products. The target products 7a–d were obtained in 41%–78% yields, respectively. Next, we examined the reactivity of different substituted thiosulfonates. It is worth pointing out that the reaction showed a broad functional group compatibility, in which both electron-donating group Me and electron-donating withdrawing groups such as Cl and NO2, were well tolerated. The target products 7e–7f could be observed in excellent yields. To our delight, when S-(4-hydroxyphenyl) benzenesulfonothioate and S-(4-aminophenyl) benzenesulfonothioate were applied to the reaction, the target products 7h and 7i were obtained in moderate yields, respectively. The reaction of S-(benzo[d]thiazol-2-yl) benzenesulfonothioate with cyclohexylsilicate led to 7j in 86% yield. Unfortunately, alkyl thiosulfonates failed to afford the desired products under the identical reaction conditions.
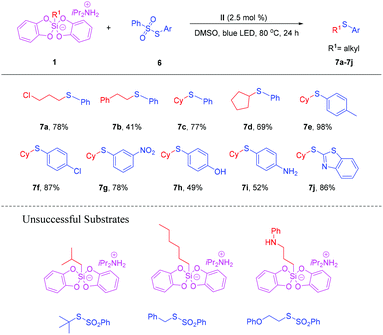 |
| Scheme 4 Substrate scope for aryl-alkyl sulfides compounds. Reaction conditions: substituted silicate 1 (0.34 mmol), substituted thiosulfonates 6 (0.2 mmol), II (2.5 mol%), (dry)DMSO (1 mL), 80 °C, blue LED, N2, 24 h. Isolated yields. | |
In order to improve the atom economy of the reaction and explore the further application of this reaction, we successfully recovered catechol with good yield (Scheme 5a). The successful recovery and reuse of catechol shows that this protocol has high atom economy. In order to explore the further application of this reaction, we tried the scale-up reaction of products 3q, 5h and 7c. To our delight, the target products 3q, 5h and 7c could be obtained in excellent yields, respectively (Scheme 5b). In addition, according to the relevant literature,16 the treatment of compound 7c with different oxidizing agents successfully led to sulfoxide 8a and sulfone 8b in 88% and 78% yields, respectively (Scheme 5c). The above experiments show that this strategy has huge advantages in the potential industrial applications. What is exciting is that the reaction of cyclohexylsilicate with methyl (2S)-2-((tert-butoxycarbonyl)amino)-3-(phenoxyselenonothioyl)propanoate 2i under the optimized conditions successfully constructed the target product methyl (S)-2-((tert-butoxycarbonyl)amino)-3-(cyclohexylselanyl)propanoate 3r in 55% yields (Scheme 5d). This shows that the strategy has broad application prospects in the biological field.
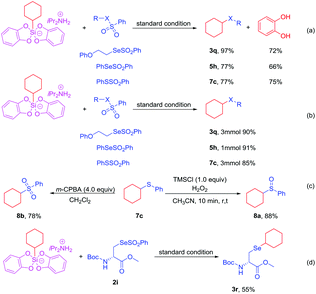 |
| Scheme 5 Related derivative reactions. (a) Recovery and reuse of catechol. (b) The scale-up reaction of products 3q, 5h and 7c. (c) Under suitable oxidation conditions, the sulfide could be selectively oxidized to sulfoxide or sulfone. (d) Successfully constructed the target product methyl (S)-2-((tert-butoxycarbonyl)amino)-3-(cyclohexylselanyl)propanoate. | |
Further mechanistic studies were conducted to gain deeper insight into the reaction pathway. Adding stoichiometric amounts of radical scavenger TEMPO or BHT and 1,1-diphenylethylene to the reaction significantly suppressed the conversion, which revealed that a radical mechanism might be involved in this transformation. The reaction of cyclohexylsilicate with diselenide compound 9 instead of selenosulfonategave 3q in only <10% yield. This result indicates that diselenide compound 9 was not the main reaction intermediate of this reaction (Scheme 6).
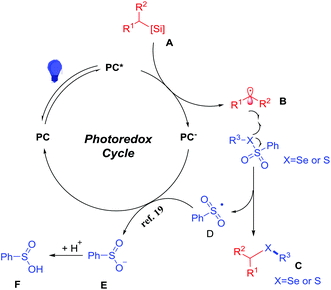 |
| Scheme 6 Mechanistic studies. (a) Radical scavengers are added under standard conditions, the target product could only be obtained in trace yields. (b) Treatment of cyclohexyl silicon and diselenide 9 under standard reaction conditions could not lead the target product. | |
Based on the above experimental results and previous reports,17,18 we proposed a plausible mechanism in Scheme 7. The photocatalyst assists the single electron transfer of the alkylsilicate to generate an alkyl radical B. Subsequently, alkyl radical reacts B with selenosulfonates or sulfonothioates to afford the desired products and a sulfone radical intermediate.19 Then the sulfone radical is reduced to a sulfone anionic intermediate E. Subsequently, protonation of intermediate E affords the benzosulfinic acid.20
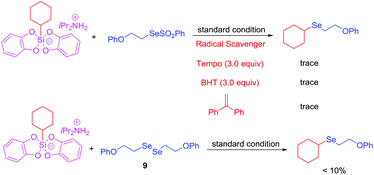 |
| Scheme 7 A plausible mechanism. | |
Conclusion
In summary, we have successfully developed a light-mediated two-component free radical cross-coupling reaction between alkylsilicate compounds and selenosulfonates under metal-free catalysis conditions. At the same time, this strategy is also applicable to the efficient construction of aryl-alkyl selenides, heteroayl-alkyl selenides and aryl-alkyl sulfides. A new method for efficiently constructing Csp3–Se and Csp3–S bonds without any additives was developed. Successful construction of the unsymmetrical primary alkyl–alkyl selenide compounds, which are difficult to prepare, is the highlight of this work. It provides a green and simple method for the preparation of alkyl–alkyl selenide and aryl–alkyl selenide compounds. The recovery and reuse of catechol and the scale-up experiments show the broad prospects of this strategy in industrial applications. Successful construction of the selenocarbamate compound 3r shows that the strategy has broad application prospects in the biological field.
Experimental section
General procedure
Synthesis of 3d.
In a glovebox, an oven-dried screw-capped 8 mL vial equipped with a magnetic stir bar was charged with diisopropylammonium bis(catecholato)propylsilicate 1a (129.6 mg, 0.34 mmol) and Se-(2-phenoxyethyl) benzenesulfonoselenoate 2d (68.4 mg 0.2 mmol), cat (1.4 mg, 2.5 mol%), and DMSO (1.0 mL) were added via a syringe. The reaction mixture was stirred at r.t for 24 h under a 40 W LED lamp (40 W; λ = 450–460 nm; 5 cm away; made in TanLu. Ltd; borosilicate glass). After 24 h, the crude reaction mixture was diluted with ethyl acetate (20 mL) and washed with water (20 mL × 3). The organic layer was dried over Na2SO4, filtered, and concentrated. The residue was purified by flash chromatography to afford the pure product 3d.
Synthesis of 5a.
In a glovebox, an oven-dried screw-capped 8 mL vial equipped with a magnetic stir bar was charged with diisopropylammonium bis(catecholato)propylsilicate 1a (129.6 mg, 0.34 mmol) and Se-phenyl benzenesulfonoselenoate 4a (59.6 mg 0.2 mmol), cat (1.4 mg, 2.5 mol%), and DMSO (1.0 mL) were added via a syringe. The reaction mixture was stirred at 80 °C for 24 h under a 40 W LED lamp (40 W; λ = 450–460 nm; 5 cm away; made in TanLu. Ltd; borosilicate glass). After 24 h, the crude reaction mixture was diluted with ethyl acetate (20 mL) and washed with water (20 mL × 3). The organic layer was dried over Na2SO4, filtered, and concentrated. The residue was purified by flash chromatography to afford the pure product 5a.
Synthesis of 7c.
In a glovebox, an oven-dried screw-capped 8 mL vial equipped with a magnetic stir bar was charged with bis(catechol)diisopropyl ammonium cyclohexyl silicate 1f (129.6 mg, 0.34 mmol) and S-phenyl benzenesulfonothioate 6a (50.0 mg 0.2 mmol), cat (1.4 mg, 2.5 mol%), and DMSO (1.0 mL) were added via a syringe. The reaction mixture was stirred at 80 °C for 24 h under a 40 W LED lamp (40 W; λ = 450–460 nm; 5 cm away; made in TanLu. Ltd; borosilicate glass). After 24 h, the crude reaction mixture was diluted with ethyl acetate (20 mL) and washed with water (20 mL × 3). The organic layer was dried over Na2SO4, filtered, and concentrated. The residue was purified by flash chromatography to afford the pure product 7c.
Conflicts of interest
There are no conflicts to declare.
Acknowledgements
We gratefully acknowledge the National Natural Science Foundation of China (No. 21971174, 21772137), PAPD, Cyrus Tang (Zhongying) scholar and Soochow University for financial support.
Notes and references
- T. Maseko, D. L. Callahan, F. R. Dunshea, A. Doronila, S. D. Kolev and K. Ng, Chemical characterisation and speciation of organic selenium in cultivated selenium-enriched Agaricus bisporus, Food Chem., 2013, 141, 3681 CrossRef CAS PubMed.
- E. Dumont, F. Vanhaecke and R. Cornelis, Selenium speciation from food source to metabolites: a critical review, Anal. Bioanal. Chem., 2006, 385, 1304 CrossRef CAS PubMed.
-
(a) J. Luo, Z.-C. Zhu, Y.-N. Liu and X.-D. Zhao, Diaryl Selenide Catalyzed Vicinal Trifluoromethylthioamination of Alkenes, Org. Lett., 2015, 17, 3620 CrossRef CAS PubMed;
(b) L.-H. Liao, R.-Z. Guo and X.-D. Zhao, Organoselenium-Catalyzed Regioselective C-H Pyridination of 1,3-Dienes and Alkenes, Angew. Chem., Int. Ed., 2017, 56, 3201 CrossRef CAS PubMed.
- P. C. Srivastava and R. K. Robins, Synthesis and antitumor activity of 2-.beta.-D-ribofuranosylselenazole-4-carboxamide and related derivatives, J. Med. Chem., 1983, 26, 445 CrossRef CAS PubMed.
- L. L. Miller, J. B. Rasmussen, V. P. Palace and A. Hontela, Physiological stress response in white suckers from agricultural drain waters containing pesticides and selenium, Ecotoxicol. Environ. Saf., 2009, 72, 1249 CrossRef CAS.
- E. C. Mägi, L. B. Fu, H. C. Nguyen, M. R. E. Lamont, D. I. Yeom and B. J. Eggleton, Enhanced Kerr nonlinearity in sub-wavelength diameter As2Se3 chalcogenide fiber tapers, Opt. Express, 2007, 15, 10324 CrossRef PubMed.
- Y. Fang, T. Rogge, L. Ackermann, S.-Y. Wang and S.-J. Ji, Nickel-catalyzed reductive thiolation and selenylation of unactivated alkyl bromides, Nat. Commun., 2018, 9, 2240 CrossRef PubMed.
-
(a) S.-L. Huang, Z.-L. Chen, H. Mao, F.-L. Hu, D.-M. Li, Y. Tan, F.-Q. Yang and W.-L. Qin, Metal-free difunctionalization of alkynes to access tetrasubstituted olefins through spontaneous selenosulfonylation of vinylidene ortho-quinone methide (VQM), Org. Biomol. Chem., 2019, 17, 1121 RSC;
(b) Z.-L. Chen, F.-L. Hu, S.-L. Huang, Z.-G. Zhao, H. Mao and W.-L. Qin, Organocatalytic Enantioselective Selenosulfonylation of a C–C Double Bond To Form Two Stereogenic Centers in an Aqueous Medium, J. Org. Chem., 2019, 84, 8100 CrossRef CAS PubMed;
(c) M. J. Davies and C. H. Schiesser, 1,4-Anhydro-4-seleno-D-talitol (SeTal): a remarkable selenium-containing therapeutic molecule, New J. Chem., 2019, 43, 9759 RSC;
(d) N. Al-Maharik, L. Engman, J. Malmstrom and C. H. Schiesser, Intramolecular Homolytic Substitution at Selenium: Synthesis of Novel Selenium-Containing Vitamin E Analogues, J. Org. Chem., 2001, 66, 6286 CrossRef CAS PubMed;
(e) M. W. Carland, R. L. Martin and C. H. Schiesser, Preparation of novel selenapenams and selenacephems by nucleophilic and radical chemistry involving benzyl selenides, Org. Biomol. Chem., 2004, 2, 2612 RSC;
(f) K. M. Aumann, P. J. Scammells, J. M. White and C. H. Chiesser, On the stability of 2-aminoselenophene-3-carboxylates: potential dual-acting selenium-containing allosteric enhancers of A1 adenosine receptor binding, Org. Biomol. Chem., 2007, 5, 1276 RSC.
-
(a) C. Ghiazza, L. Khrouz, C. Monnereau, T. Billard and A. Tlili, A. Visible-light promoted fluoroalkylselenolation: toward the reactivity of unsaturated compounds, Chem. Commun., 2018, 54, 9909 RSC;
(b) M. R. Mutra, V. S. Kudale, J. Li, W.-H. Tsaia and J.-J. Wang, Alkene versus alkyne reactivity in unactivated 1,6-enynes: regio- and chemoselective radical cyclization with chalcogens under metal- and oxidant-free conditions, Green Chem., 2020, 22, 2288 RSC;
(c) R. Zhang, P. Xu, S.-Y. Wang and S.-J. Ji, Visible Light-Induced Co- or Cu-Catalyzed Selenosulfonylation of Alkynes: Synthesis of β-(Seleno)vinyl Sulfones, J. Org. Chem., 2019, 84, 12324 CrossRef CAS PubMed;
(d) J. Li, X.-E. Yang, S.-L. Wang, L.-L. Zhang, X.-Z. Zhou, S.-Y. Wang and S.-J. Ji, Visible-Light-Promoted Cross-Coupling Reactions of 4-Alkyl-1,4-dihydropyridines with Thiosulfonate or Selenium Sulfonate: A Unified Approach to Sulfides, Selenides, and Sulfoxides, Org. Lett., 2020, 22, 4908 CrossRef CAS PubMed.
- For representative works, see:
(a) Y. Nishigaichi, T. Orimi and A. Takuwa, Photo-allylation and photo-benzylation of carbonyl compounds using organotrifluoroborate reagents, J. Organomet. Chem., 2009, 694, 3837 CrossRef CAS;
(b) G. Sorin, R. M. Mallorquin, Y. Contie, A. Baralle, M. Malacria, J.-P. Goddard and L. Fensterbank, Oxidation of Alkyl Trifluoroborates: An Opportunity for Tin-Free Radical Chemistry, Angew. Chem., Int. Ed., 2010, 49, 8721 CrossRef CAS PubMed;
(c) G. A. Molander, V. Colombel and V. A. Braz, Direct Alkylation of Heteroaryls Using Potassium Alkyl- and Alkoxymethyltrifluoroborates, Org. Lett., 2011, 13, 1852 CrossRef CAS PubMed;
(d) J. W. Lockner, D. D. Dixon, R. Risgaard and P. S. Baran, Practical Radical Cyclizations with Arylboronic Acids and Trifluoroborates, Org. Lett., 2011, 13, 5628 CrossRef CAS PubMed;
(e) S. R. Neufeldt, C. K. Seigerman and M. S. Sanford, Mild Palladium-Catalyzed C–H Alkylation Using Potassium Alkyltrifluoroborates in Combination with MnF3, Org. Lett., 2013, 15, 2302 CrossRef CAS PubMed.
- For representative works, see:
(a) K. Nakajima, X.-F. Guo and Y. Nishibayashi, Cross-Coupling Reactions of Alkenyl Halides with 4-Benzyl-1,4- Dihydropyridines Associated with E to Z Isomerization under Nickel and Photoredox Catalysis, Chem. – Asian J., 2018, 13, 3653 CrossRef CAS PubMed;
(b) W.-W. Chen, Z. Liu, J.-Q. Tian, J. Li, J. Ma, X. Cheng and G.-G. Li, Building Congested Ketone: Substituted Hantzsch Ester and Nitrile as Alkylation Reagents in Photoredox Catalysis, J. Am. Chem. Soc., 2016, 138, 12312 CrossRef CAS PubMed;
(c) X. Liu, R.-Y. Liu, J. Dai, X. Cheng and G.-G. Li, Application of Hantzsch Ester and Meyer Nitrile in Radical Alkynylation Reactions, Org. Lett., 2018, 20, 6906 CrossRef CAS PubMed;
(d) K. Nakajima, Y.-L. Zhang and Y. Nishibayashi, Alkylation Reactions of Azodicarboxylate Esters with 4-Alkyl-1,4-Dihydropyridines under Catalyst-Free Conditions, Org. Lett., 2019, 21, 4642 CrossRef CAS PubMed;
(e) S.-Z. Liang, T. Kumon, R. A. Angnes, M. Sanchez, B. Xu and G. B. Hammond, Synthesis of Alkyl Halides from Aldehydes via Deformylative Halogenation, Org. Lett., 2019, 21, 3848 CrossRef CAS PubMed.
-
(a) R. R. Holmes, The stereochemistry of nucleophilic substitution of tetracoordinate silicon, Chem. Rev., 1990, 90, 17 CrossRef CAS;
(b) C. Chuit, R. J. P. Corriu, C. Reye and J. C. Young, Reactivity of penta- and hexacoordinate silicon compounds and their role as reaction intermediates, Chem. Rev., 1993, 93, 1371 CrossRef CAS.
- J. Yoshida, K. Tamao, T. Kakui, A. Kurita, M. Murata, K. Yamada and M. Kumada, Organofluorosilicates in organic synthesis. 13. Copper(II) oxidation of organopentafluorosilicates, Organometallics, 1982, 1, 369 CrossRef CAS.
- C. L. Frye, Pentacoordinate Silicon Derivatives. II.1 Salts of Bis(o-arylenedioxy) organosiliconic Acids, J. Am. Chem. Soc., 1964, 86, 3170 CrossRef CAS.
- For representative works, see:
(a) N. R. Patel, C. B. Kelly, M. Jouffroy and G. A. Molander, Engaging Alkenyl Halides with Alkylsilicates via Photoredox Dual Catalysis, Org. Lett., 2016, 18, 764 CrossRef CAS PubMed;
(b) M. Jouffroy, D. N. Primer and G. A. Molander, Base-Free Photoredox/Nickel Dual-Catalytic Cross-Coupling of Ammonium Alkylsilicates, J. Am. Chem. Soc., 2016, 138, 475 CrossRef CAS PubMed;
(c) S. Zheng, D. N. Primer and G. A. Molander, Nickel/Photoredox-Catalyzed Amidation via Alkylsilicates and Isocyanates, ACS Catal., 2017, 7, 7957 CrossRef CAS PubMed;
(d) A. G. Domínguez, R. Mondal and C. Nevado, Dual Photoredox/Nickel-Catalyzed Three-Component Carbofunctionalization of Alkenes, Angew. Chem., 2019, 131, 12414 CrossRef;
(e) J. K. Matsui, S. B. Lang, D. R. Heitz and G. A. Molander, Photoredox-Mediated Routes to Radicals: The Value of Catalytic Radical Generation in Synthetic Methods Development, ACS Catal., 2017, 7, 2563 CrossRef CAS PubMed For representative works, see:
(f) N. R. Patel, C. B. Kelly, A. P. Siegenfeld and G. A. Molander, Mild, Redox-Neutral Alkylation of Imines Enabled by an Organic Photocatalyst, ACS Catal., 2017, 7, 1766 CrossRef CAS PubMed;
(g) S. B. Lang, R. J. Wiles, C. B. Kelly and G. A. Molander, Photoredox Generation of Carbon-Centered Radicals Enables the Construction of 1,1−Difluoroalkene Carbonyl Mimics, Angew. Chem., Int. Ed., 2017, 56, 15073 CrossRef CAS PubMed;
(h) S. T. J. Cullen and G. K. Friestad, Alkyl Radical Addition to Aliphatic and Aromatic N-Acylhydrazones Using an Organic Photoredox Catalyst, Org. Lett., 2019, 21, 8290 CrossRef CAS PubMed.
- For representative works, see:
(a) V. Corcé, L. M. Chamoreau, E. Derat, J. P. Goddard, C. Ollivier and L. Fensterbank, Silicates as Latent Alkyl Radical Precursors: Visible-Light Photocatalytic Oxidation of Hypervalent Bis-Catecholato Silicon Compounds, Angew. Chem., Int. Ed., 2015, 54, 11414 CrossRef PubMed;
(b) L. Chenneberg, C. Lévêque, V. Corcé, A. Baralle, J. P. Goddard, C. Ollivier and L. Fensterbank, Single-Electron-Transfer Oxidation of Trifluoroborates and Silicates with Organic Reagents: A Comparative Study, Synlett, 2016, 731 CAS;
(c) C. Lévêque, L. Chenneberg, J. P. Goddard, C. Ollivier and L. Fensterbank, Primary alkyl bis-catecholato silicates in dual photoredox/nickel catalysis: aryl- and heteroarylalkyl cross coupling reactions, Org. Chem. Front., 2016, 3, 462 RSC;
(d) C. Lévêque, L. Chenneberg, J. P. Goddard, C. Ollivier and L. Fensterbank, Organic photoredox catalysis for the oxidation of silicates: applications in radical synthesis and dual catalysis, Chem. Commun., 2016, 52, 9877 RSC;
(e) A. Cartier, E. Levernier, A. L. Dhimane, T. Fukuyama, C. Ollivier, I. Ryu and L. Fensterbank, Synthesis of Aliphatic Amides through a Photoredox Catalyzed Radical Carbonylation Involving Organosilicates as Alkyl Radical Precursors, Adv. Synth. Catal., 2020, 362, 2254 CrossRef CAS;
(f) T. Guo, L. Zhang, X.-B. Liu, Y.-W. Fang, X.-P. Jin, Y. Yang, Y. Li, B. Chen and M.-H. Ouyang, Visible-Light-Promoted Redox-Neutral Cyclopropanation Reactions of α-Substituted Vinylphosphonates and Other Michael Acceptors with Chloromethyl Silicate as Methylene Transfer Reagent, Adv. Synth. Catal., 2018, 360, 4459 CrossRef CAS;
(g) Y.-J. Liu, W.-P. Luo, J.-L. Wu, Y.-W. Fang, Y. Li, X.-P. Jin, L. Zhang, Z.-Y. Zhang, F.-F. Xu and C. Du, Radical addition-polar termination cascade: efficient strategy for photoredox-neutralcatalysed cyclopropanation and Giese-type reactions of alkenyl N-methyliminodiacetyl boronates, Org. Chem. Front., 2020, 7, 1588 RSC;
(h) K. D. Raynor, G. D. May, U. K. Bandarage and M. J. Boyd, Generation of Diversity Sets with High sp3 Fraction Using the Photoredox Coupling of Organotrifluoroborates and Organosilicates with Heteroaryl/Aryl Bromides in Continuous Flow, J. Org. Chem., 2018, 83, 1551 CrossRef CAS PubMed;
(i) A. Cartier, E. Levernier, V. Corcé, T. Fukuyama, A. L. Dhimane, C. Ollivier, I. Ryu and L. Fensterbank, Carbonylation of Alkyl Radicals Derived from Organosilicates through Visible-Light Photoredox Catalysis, Angew. Chem., Int. Ed., 2019, 58, 1789 CrossRef CAS PubMed.
-
(a) K. Bahrami, M. M. Khodaei, B. H. Yousefi and M. S. Arabi, TMSCl-promoted selective oxidation of sulfides to sulfoxides with hydrogen peroxide, Tetrahedron Lett., 2010, 51, 6939 CrossRef CAS;
(b) Y. Fang, T. Rogge, L. Ackermann, S.-Y. Wang and S.-J. Ji, Nickel-catalyzed reductive thiolation and selenylation of unactivated
alkyl bromides, Nat. Commun., 2018, 9, 2240 CrossRef PubMed.
-
(a) N. R. Patel, C. B. Kelly, A. P. Siegenfeld and G. A. Molander, Mild, Redox-Neutral Alkylation of Imines Enabled by an Organic Photocatalyst, ACS Catal., 2017, 7, 1766 CrossRef CAS PubMed;
(b) J. Li, X.-E. Yang, S.-L. Wang, L.-L. Zhang, X.-Z. Zhou, S.-Y. Wang and S.-J. Ji, Visible-Light-Promoted Cross-Coupling Reactions of 4-Alkyl-1,4-dihydropyridines with Thiosulfonate or Selenium Sulfonate: A Unified Approach to Sulfides, Selenides, and Sulfoxides, Org. Lett., 2020, 22, 4908 CrossRef CAS PubMed.
- P. Carta, N. Puljic, C. Robert, A. L. Dhimane, C. Ollivier, L. Fensterbank, E. Lacôte and M. Malacria, Intramolecular homolytic substitution at the sulfur atom: an alternative way to generate phosphorus- and sulfur-centered radicals, Tetrahedron, 2008, 64, 11865 CrossRef CAS.
-
(a) P.-Z. Xie, J.-Y. Wang, Y.-N. Liu, J. Fan, X.-Y. Wo, W.-S. Fu, Z.-L. Sun and T. P. Loh, Water-promoted C-S bond formation reactions, Nat. Commun., 2018, 9, 1321 CrossRef PubMed;
(b) J. Li, X.-E. Yang, S.-L. Wang, L.-L. Zhang, X.-Z. Zhou, S.-Y. Wang and S.-J. Ji, Visible-Light-Promoted Cross-Coupling Reactions of 4-Alkyl-1,4-dihydropyridines with Thiosulfonate or Selenium Sulfonate: A Unified Approach to Sulfides, Selenides, and Sulfoxides, Org. Lett., 2020, 22, 4908 CrossRef CAS PubMed.
Footnote |
† Electronic supplementary information (ESI) available. See DOI: 10.1039/d1qo00085c |
|
This journal is © the Partner Organisations 2021 |