DOI:
10.1039/C9SC04073K
(Edge Article)
Chem. Sci., 2019,
10, 10510-10515
Design, synthesis and application of a new type of bifunctional Le-Phos in highly enantioselective γ-addition reactions of N-centered nucleophiles to allenoates†
Received
14th August 2019
, Accepted 1st September 2019
First published on 3rd September 2019
Abstract
A novel class of cyclic phosphine derived bifunctional catalysts (Le-Phos) is reported, which can be readily prepared from inexpensive and commercially available starting materials and exhibit good performances in enantioselective γ-addition reactions of N-centered nucleophiles and allenoates under mild conditions. The salient features of this reaction include high product yields, good enantioselectivity, mild reaction conditions, and broad substrate scope and gram-scale scalability.
Introduction
Over the past few years, asymmetric phosphine-catalyzed reactions have emerged as powerful and versatile tools for the construction of C–C and C–X bonds,1 which relies very much on the evolution of various new chiral phosphine catalysts.2 There are mainly two types of chiral phosphine catalysts developed: highly nucleophilic monofunctional phosphine catalysts such as cyclic phosphines P1–P5 (Fig. 1, Type 1) and diphenylphosphine-derived bifunctional catalysts bearing a hydrogen donor such as P6–P9 (Fig. 1, Type 2). Both displayed good catalytic activities and were effective in enantiomeric control in asymmetric phosphine catalysis.1a,g,3 Recently, we developed several novel diphenylphosphine-derived bifunctional phosphines from commercially available chiral sulfinamide.4 To further advance a new catalyst design, we aimed to combine the advantages of the aforementioned two types of phosphine catalysts, thus developing a novel bifunctional cyclic phosphine catalyst. We report herein the design and synthesis of Le-Phos, and its application in highly enantioselective phosphine catalyzed γ-addition of N-centered nucleophiles to allenoates.
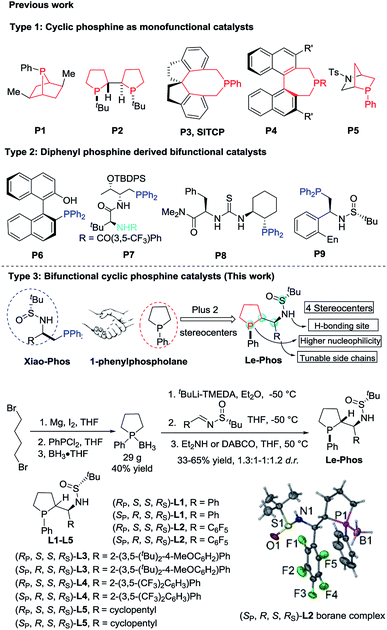 |
| Fig. 1 Different types of chiral phosphine catalysts. | |
Results and discussion
Fortunately, we found that Le-Phos could be easily prepared from commercially available inexpensive tert-butylsulfinamide, aldehyde and 1-phenylphospholane borane complexes in simple steps. Treatment of 1-phenylphospholane borane complexes5 with tBuLi in the presence of TMEDA at −50 °C for 4 h gave the lithium intermediate, which added to chiral (RS)-sulfinimines, furnishing a pair of major diastereomers of Le-PhosL1–L5 in 33–65% total yields after removal of borane.6 To our delight, these two major diastereoisomers could be separated by flash column chromatography on silica gel. The absolute configurations of (RP,S,S,RS)-L2 and (SP,R,S,RS)-L2 were established by single crystal X-ray diffraction analysis.7
Asymmetric phosphine-catalyzed γ-addition reactions of various nucleophiles to allenoates have attracted much attention in the past few years.8–10 In 1998, Zhang and co-workers reported the catalyzed asymmetric γ-addition of 1,3-dicarbonyl compounds to terminal allenoates using bicyclic phosphine P2 for the first time.9 Furthermore, Fu, Jacobsen, Lu and our groups have successfully expanded the scope of nucleophiles such as alcohols, thiols, carbon, amides and ketimines by the employment of different types of phosphine catalysts.10 The asymmetric γ-addition8–11 of N-centered nucleophiles with pKa values between 8 and 10 (in H2O) to γ-substituted allenoates has been only partially realized by the group of Jacobsen, in which P8 was used as the catalyst.10n Very recently, Guo and coworkers successfully extended N-centered nucleophiles to pyrazoles and imidazoles with the use of (S)-SITCP and (S)-BINOL as cocatalysts.13 However, there still lacks a robust catalyst system for the asymmetric γ-addition of various N-centered nucleophiles to allenoates. For example, (S)-SITCP, P8 and our developed Xiao-Phos P9 could not yield satisfactory results for the asymmetric γ-addition of 2-oxazolidone 1a to allenoate 2a (Table 1, entries 1–3). Interestingly, (SP,R,S,RS)-L1–L4 showed much higher catalytic activity and much better enantioselectivity than their diastereoisomers (RP,S,S,RS)-L1–L4 (Table 1, entries 4–11). To our delight, 54% yield of 3aa with 97% ee and E/Z > 20
:
1 could be achieved with the use of (SP,R,S,RS)-L4 (Table 1, entry 11). Due to the competitive isomerization and partial kinetic resolution,10l increasing allenoate 2a to two equivalents could improve the 68% yield (Table 1, entry 13). Changing the solvent from toluene to PhCF3, DCM and DCE led to around 90% yield with 96–97% ees (Table 1, entries 14–17).
Table 1 Screening reaction conditionsa

|
Entry |
Catalyst |
Solvent |
E/Zb |
Yieldb (%) |
eec (%) |
Reaction conditions: 1a (0.10 mmol), 2a (0.12 mmol), and the catalyst (0.01 mmol) in toluene (1.5 mL) at room temperature.
NMR yield with the use of CH2Br2 as the internal standard.
Determined by HPLC analysis on a chiral stationary phase.
Performed with 2a (0.15 mmol).
Performed with 2a (0.20 mmol). DCM = dichloromethane, DCE = 1,2-dichloroethane.
|
1 |
(S)-SITCP |
Toluene |
5 : 1 |
39 |
87 |
2 |
P8
|
Toluene |
4 : 1 |
11 |
72 |
3 |
P9
|
Toluene |
2 : 1 |
21 |
57 |
4 |
(RP,S,S,RS)-L1 |
Toluene |
3 : 1 |
7 |
19 |
5 |
(RP,S,S,RS)-L2 |
Toluene |
— |
NR |
— |
6 |
(RP,S,S,RS)-L3 |
Toluene |
2 : 1 |
5 |
46 |
7 |
(RP,S,S,RS)-L4 |
Toluene |
2 : 1 |
9 |
11 |
8 |
(SP,R,S,RS)-L1 |
Toluene |
>20 : 1 |
40 |
86 |
9 |
(SP,R,S,RS)-L2 |
Toluene |
>20 : 1 |
10 |
69 |
10 |
(SP,R,S,RS)-L3 |
Toluene |
>20 : 1 |
46 |
97 |
11 |
(SP,R,S,RS)-L4 |
Toluene |
>20 : 1 |
54 |
97 |
12d |
(SP,R,S,RS)-L4 |
Toluene |
>20 : 1 |
60 |
97 |
13e |
(SP,R,S,RS)-L4 |
Toluene |
>20 : 1 |
68 |
97 |
14e |
(SP,R,S,RS)-L4 |
Et2O |
>20 : 1 |
60 |
97 |
15e |
(SP,R,S,RS)-L4 |
PhCF3 |
>20 : 1 |
90 |
97 |
16e |
(SP,R,S,RS)-L4 |
DCM |
>20 : 1 |
89 |
96 |
17e |
(SP,R,S,RS)-L4 |
DCE |
>20 : 1 |
90 |
97 |
Having identified the optimal reaction conditions, the substrate scope was then examined and it proved to be quite general (Scheme 1). Linear alkyl (3ab–3ad), branched alkyl (3ae), and various alkyl groups bearing functional groups such as phenyl (3af), esters (3ag and 3ak), terminal alkenes and alkynyl (3ah–3ai), and halogen (3aj) were well tolerated and provided high levels of yields and enantioselectivities (94–98% ees). Cyclic alkyl groups such as cyclopentyl (3al), cyclohexyl (3am), and NPhth groups (3an) could also be well compatible, delivering the corresponding adducts in high yields with 95–96% ees. It seems that the ester moiety did not affect the reaction much, furnishing 3ao–3aq in high yields with 93–97% ees and E/Z > 20
:
1.
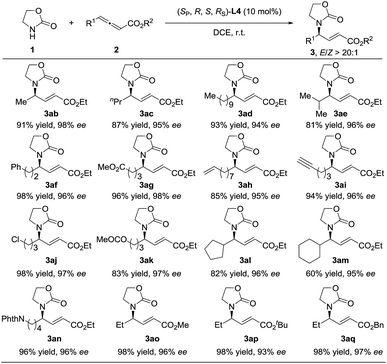 |
| Scheme 1 Investigation of the scope by variation of the allenoate component. | |
The reactions of chiral 2-oxazolidones also proceeded well, delivering 3ca–3ea in satisfactory yields with high des and E/Z > 20
:
1 (Scheme 2). The addition of racemic 2-oxazolidone 1f did not show good diastereoselectivity but still delivered high enantioselectivity. Then, the reactions of thiazolidin-2-one (pKa ∼ 12.8) with various allenoates also proceeded smoothly, furnishing products 3ga and 3gc–3gg in 85–99% yields with 95–96% ees. It should be pointed out that these products share the same skeleton with patented 11β-HSD1 inhibitors (11β-hydroxysteroid dehydrogenase type 1 inhibitors).12
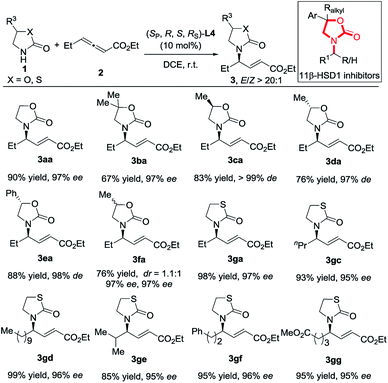 |
| Scheme 2 Investigation of the scope by variation of 2-oxazolidone. | |
The scope of N-centered nucleophiles was then extended to much weak nucleophilic pyrrolidine-2,5-diones (Scheme 3). In this case, (SP,R,S,RS)-L2 was found to be the most efficient catalyst, indicating that the reaction is quite sensitive to the structure of N-centered nucleophiles, which further supports that the development of new catalysts with structural diversity is quite important. The reactions of various substituted pyrrolidine-2,5-diones with 5a delivered the desired γ-addition adducts in 68–91% yields with 87–94% ees. The absolute configuration of 6ba was established by single crystal X-ray diffraction analysis.7 It is interesting to find that the absolute configuration of 6ba is different from that of compound 3; despite this, the catalysts have the same absolute configuration.
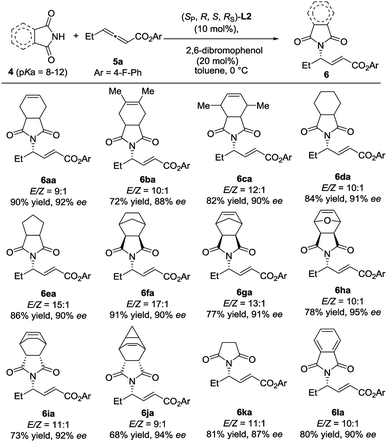 |
| Scheme 3 Investigation of the scope of pyrrolidine-2,5-diones. | |
We next examined the reaction scope with respect to the allenoate component (Scheme 4). A variety of γ-substituted allenoates (R1) were applicable to this asymmetric γ-addition. In general, both linear and branched cycloalkyl groups at the γ-position were well tolerated. For example, allenoates 5b–5g with various acyclic and cyclic alkyl groups at the γ-position could be well compatible, and the desired adducts were obtained in high yields with up to 93% ee. Satisfactorily, various functional groups such as halogens (5h and 5i), ester (5j), phenyl (5k), and terminal and internal alkenes (5l–5n) were well tolerated and the desired adducts were obtained in moderate to good yields with up to 92% ee and >20
:
1 E/Z selectivity.
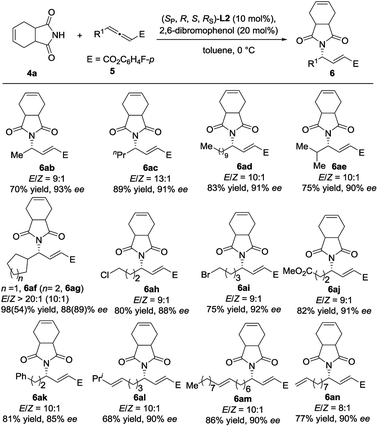 |
| Scheme 4 Investigation of the scope by variation of the allenoate component. | |
Additionally, the additions of TsNH2 (pKa ∼ 10.2), PhSO2NH2 (pKa ∼ 10.1), (BocNH)2 (pKa ∼ 8.7) and pyrazole (pKa ∼ 2.5)13 also proceeded smoothly under the catalysis of Le-Phos with different R groups (eqn (1)–(3)).
We were pleased to find that the desired product 3ga could be obtained in 96% yield, 94% ee and E/Z > 20
:
1 with only 2.5 mol% catalyst loading on a 10 mmol scale (Scheme 5). The synthetic utilities of the representative product 3ga were then showcased. The hydrolysis of the ester moiety was realized with NaOH/H2O2
14 to give acid 12 in 73% yield without loss of enantioselectivity. The corresponding amide 137 could be further delivered in 94% yield with 95% ee. The copper-catalyzed conjugate borylation of 3ga proceeded smoothly at room temperature, furnishing the desired product 14 in 94% yield with 98% ee and 5
:
1 d.r.15 Reduction of the double bond furnished the product 15 in 98% yield with 95% ee. Moreover, we could obtain an amino alcohol derivative 16 through reductive ring-opening of 15, which afforded the diester 17 after further esterification. Furthermore, with the use of mCPBA,16 the C–C double bond of 6aa would undergo epoxidation to deliver the corresponding product 18 in good yield without loss of the enantioselectivity. The amidation reaction of 6aa with BnNH2/AcOH17 proceeded smoothly at room temperature, delivering the corresponding amide 19 in 85% yield with 89% ee. The reduction of the double bond of 6lo was achieved via the Pd/C-catalyzed hydrogenation, furnishing product 20 in 96% yield without loss of the ee. The corresponding γ-aminoacid 21 was obtained in 78% yield by acidic deprotection.18 Then, 21 was reacted with benzoyl chloride to deliver an amino acid derivative 22 in 63% yield with 87% ee.19
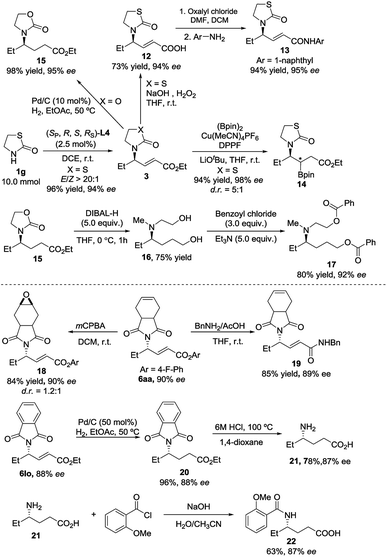 |
| Scheme 5 Elaboration of γ-addition adducts. | |
Based on the above experimental results and previous relevant studies, a possible transition state (TS-1) for (SP,R,S,RS)-L4 and possible transition state (TS-1′) for (RP,S,S,RS)-L4 to control stereoselectivity are proposed in Scheme 6. For the reaction using (SP,R,S,RS)-L4 as the catalyst, the nucleophile and the double bond are located on the same side (transition state TS-1) via the hydrogen-bonding between nucleophiles and the NH moiety, which favors the formation of the R-enantiomer of 3. In contrast, when (RP,S,S,RS)-L4 was used as the catalyst, another transition state TS-1′ was proposed, in which there may exist a steric repulsion between the phenyl linked to P and the nucleophile. Additionally, the nucleophile is located on different sides of the double bond and thus hindered the addition reaction to give the product in low yield and ee.
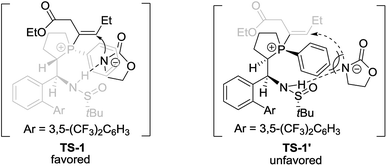 |
| Scheme 6 Comparison of two transition states. | |
Conclusions
In summary, we have developed a novel type of bifunctional chiral sulfinamide cyclic phosphine catalyst Le-Phos, which can be easily prepared on a gram scale from inexpensive commercially available starting materials in short steps. (SP,R,S,RS)-Le-Phos has shown excellent performance in the enantioselective γ-addition reactions of various N-centered nucleophiles to γ-substituted allenoates, acquiring a series of γ-addition adducts in high yields with up to 98% ees and excellent regioselectivity and diastereoselectivity under mild conditions. Its prominent characteristics are general substrate scope, mild reaction conditions, good yields, high enantioselectivities, ease of scale-up to gram scale, and further synthetic transformations of products. Further explorations of Le-Phos as the organocatalyst and chiral ligand of transition metals in asymmetric catalysis are currently underway in our group and will be reported in due course.
Conflicts of interest
There are no conflicts to declare.
Acknowledgements
We are grateful to 973 Programs (2015CB856600), the National Natural Science Foundation of China (21425205), and Changjiang Scholars and Innovative Research Team in University (PCSIRT) for financial support.
Notes and references
- For reviews containing the construction of C–C and C–X bonds by phosphines, see:
(a) Y. Xiao, Z. Sun, H. Guo and O. Kwon, Beilstein J. Org. Chem., 2014, 10, 2089 CrossRef PubMed;
(b) W. Li and J. Zhang, Chem. Soc. Rev., 2016, 45, 1657 RSC;
(c) T. Wang, X. Han, F. Zhong, W. Yao and Y. Lu, Acc. Chem. Res., 2016, 49, 1369 CrossRef CAS PubMed;
(d) H. Li and Y. Lu, Asian J. Org. Chem., 2017, 6, 1130 CrossRef CAS;
(e) Y. Wei and M. Shi, Org. Chem. Front., 2017, 4, 1876 RSC;
(f) H. Guo, Y. Fan, Z. Sun, Y. Wu and O. Kwon, Chem. Rev., 2018, 118, 10049 CrossRef CAS PubMed;
(g) H. Ni, W.-L. Chan and Y. Lu, Chem. Rev., 2018, 118, 9344 CrossRef CAS PubMed ; for some selected examples on phosphine-catalyzed reactions, see:;
(h) S. Takizawa, T. M.-N. Nguyen, A. Grossmann, D. Enders and H. Sasai, Angew. Chem., Int. Ed., 2012, 51, 5423 (
Angew. Chem.
, 2012
, 124
, 5519
) CrossRef CAS PubMed;
(i) X. Han, W.-L. Chan, W. Yao, Y. Wang and Y. Lu, Angew. Chem., Int. Ed., 2016, 55, 6492 (
Angew. Chem.
, 2016
, 128
, 6602
) CrossRef CAS PubMed;
(j) J.-J. Xing, Y.-N. Gao and M. Shi, Adv. Synth. Catal., 2018, 360, 2552 CrossRef CAS;
(k) Y. Gu, P. Hu, C. Ni and X. Tong, J. Am. Chem. Soc., 2015, 137, 6400 CrossRef CAS PubMed;
(l) D. Wang, W. Liu, Y. Hong and X. Tong, Org. Lett., 2018, 20, 5002 CrossRef CAS PubMed;
(m) E. Li, H. Jin, P. Jia, X. Dong and Y. Huang, Angew. Chem., Int. Ed., 2016, 55, 11591 (
Angew. Chem.
, 2016
, 128
, 11763
) CrossRef CAS PubMed;
(n) J. Chen and Y. Huang, Org. Lett., 2017, 19, 5609 CrossRef CAS PubMed;
(o) B. Mao, W. Shi, J. Liao, H. Liu, C. Zhang and H. Guo, Org. Lett., 2017, 19, 6340 CrossRef CAS PubMed;
(p) C. Qin, Y. Liu, Y. Yu, Y. Fu, H. Li and W. Wang, Org. Lett., 2018, 20, 1304 CrossRef CAS PubMed;
(q) M. Shi, L.-H. Chen and C.-Q. Li, J. Am. Chem. Soc., 2005, 127, 3790 CrossRef CAS PubMed;
(r) Y.-Q. Jiang, Y.-L. Shi and M. Shi, J. Am. Chem. Soc., 2008, 130, 7202 CrossRef CAS PubMed.
-
(a) E. Vedejs, O. Daugulis and S. T. Diver, J. Org. Chem., 1996, 61, 430 CrossRef CAS PubMed;
(b) E. Vedejs and O. Daugulis, J. Am. Chem. Soc., 1999, 121, 5813 CrossRef CAS.
- For some selected examples based on application of two types of chiral phosphines, see:
(a) Q.-G. Wang, S.-F. Zhu, L.-W. Ye, C.-Y. Zhou, X.-L. Sun, Y. Tang and Q.-L. Zhou, Adv. Synth. Catal., 2010, 352, 1914 CrossRef CAS;
(b) F. Zhong, X. Han, Y. Wang and Y. Lu, Angew. Chem., Int. Ed., 2011, 50, 7837 (
Angew. Chem.
, 2011
, 123
, 7983
) CrossRef CAS PubMed;
(c) B. Tan, N. R. Candeias and C. F. Barbas III, J. Am. Chem. Soc., 2011, 133, 4672 CrossRef CAS PubMed;
(d) Y. Fujiwara and G. C. Fu, J. Am. Chem. Soc., 2011, 133, 12293 CrossRef CAS PubMed;
(e) N. Pinto, P. Retailleau, A. Voituriez and A. Marinetti, Chem. Commun., 2011, 47, 1015 RSC;
(f) I. P. Andrews and O. Kwon, Chem. Sci., 2012, 3, 2510 RSC;
(g) Z. Shi, P. Yu, T.-P. Loh and G. Zhong, Angew. Chem., Int. Ed., 2012, 51, 7825 (
Angew. Chem.
, 2012
, 124
, 7945
) CrossRef CAS PubMed;
(h) Z. Jin, R. Yang, Y. Du, B. Tiwari, R. Ganguly and Y. R. Chi, Org. Lett., 2012, 14, 3226 CrossRef CAS PubMed;
(i) F. Zhong, X. Dou, X. Han, W. Yao, Q. Zhu, Y. Meng and Y. Lu, Angew. Chem., Int. Ed., 2013, 52, 943 (
Angew. Chem.
, 2013
, 125
, 977
) CrossRef CAS PubMed;
(j) C. E. Henry, Q. Xu, Y. C. Fan, T. J. Martin, L. Belding, T. Dudding and O. Kwon, J. Am. Chem. Soc., 2014, 136, 11890 CrossRef CAS PubMed;
(k) L. Cai, K. Zhang and O. Kwon, J. Am. Chem. Soc., 2016, 138, 3298 CrossRef CAS PubMed.
-
(a) X. Su, W. Zhou, Y. Li and J. Zhang, Angew. Chem., Int. Ed., 2015, 54, 6874 (
Angew. Chem.
, 2015
, 127
, 6978
) CrossRef CAS PubMed;
(b) W. Zhou, X. Su, M. Tao, C. Zhu, Q. Zhao and J. Zhang, Angew. Chem., Int. Ed., 2015, 54, 14853 (
Angew. Chem.
, 2015
, 127
, 15066
) CrossRef CAS PubMed;
(c) W. Zhou, P. Chen, M. Tao, X. Su, Q. Zhao and J. Zhang, Chem. Commun., 2016, 52, 7612 RSC.
- X.-M. Sun, K. Manabe, W. W.-L. Lam, N. Shiraishi, J. Kobayashi, M. Shiro, H. Utsumi and S. Kobayashi, Chem.–Eur. J., 2005, 11, 361 CrossRef PubMed.
- Other tiny diastereomers exist as a mixture and it is difficult to get clean NMR..
- CCDC 1819863 ((RP,S,S,RS)-L2 with borane), 1819864 ((SP,R,S,RS)-L2 with borane), 181986 (6ba), and 1860469 (13) contain the supplementary crystallographic data for this paper.†.
-
(a) B. M. Trost and C.-J. Li, J. Am. Chem. Soc., 1994, 116, 3167 CrossRef CAS;
(b) B. M. Trost and C.-J. Li, J. Am. Chem. Soc., 1994, 116, 10819 CrossRef CAS;
(c) B. M. Trost and G. R. Drake, J. Org. Chem., 1997, 62, 5670 CrossRef CAS;
(d) C. Alvarez-Ibarra, A. G. Csákÿ and C. Gómez de la Oliva, Tetrahedron Lett., 1999, 40, 8465 CrossRef CAS;
(e) C. Alvarez-Ibarra, A. G. Csákÿ and C. Gómez de la Oliva, J. Org. Chem., 2000, 65, 3544 CrossRef CAS PubMed;
(f) D. Virieux, A.-F. Guillouzic and H.-J. Cristau, Tetrahedron, 2006, 62, 3710 CrossRef CAS;
(g) Q.-F. Zhou, K. Zhang and O. Kwon, Tetrahedron, 2015, 56, 3273 CrossRef CAS PubMed;
(h) Z. Huang, X. Yang, F. Yang, T. Lu and Q. Zhou, Org. Lett., 2017, 19, 3524 CrossRef CAS PubMed.
- Z. Chen, G. Zhu, Q. Jiang, D. Xiao, P. Cao and X. Zhang, J. Org. Chem., 1998, 63, 5631 CrossRef CAS.
-
(a) T. Wang, W. Yao, F. Zhong, G.-H. Pang and Y. Lu, Angew. Chem., Int. Ed., 2014, 53, 2964 CrossRef CAS PubMed;
(b) T. Wang, D. L. Hoon and Y. Lu, Chem. Commun., 2015, 51, 10186 RSC;
(c) T. Wang, Z. Yu, D. L. Hoon, K.-W. Huang, Y. Lan and Y. Lu, Chem. Sci., 2015, 6, 4912 RSC;
(d) T. Wang, Z. Yu, D. L. Hoon, C. Phee, Y. Lan and Y. Lu, J. Am. Chem. Soc., 2016, 138, 265 CrossRef CAS PubMed;
(e) P. Chen and J. Zhang, Org. Lett., 2017, 19, 6550 CrossRef CAS PubMed;
(f) Y. K. Chung and G. C. Fu, Angew. Chem., Int. Ed., 2009, 48, 2225 (
Angew. Chem.
, 2009
, 121
, 2259
) CrossRef CAS PubMed;
(g) S. W. Smith and G. C. Fu, J. Am. Chem. Soc., 2009, 131, 14231 CrossRef CAS PubMed;
(h) R. Sinisi, J. Sun and G. C. Fu, Proc. Natl. Acad. Sci. U. S. A., 2010, 107, 20652 CrossRef CAS PubMed;
(i) J. Sun and G. C. Fu, J. Am. Chem. Soc., 2010, 132, 4568 CrossRef CAS PubMed;
(j) Y. Fujiwara, J. Sun and G. C. Fu, Chem. Sci., 2011, 2, 2196 RSC;
(k) R. J. Lundgren, A. Wilsily, N. Marion, C. Ma, Y. K. Chung and G. C. Fu, Angew. Chem., Int. Ed., 2013, 52, 2525 (
Angew. Chem.
, 2013
, 125
, 2585
) CrossRef CAS PubMed;
(l) M. Kalek and G. C. Fu, J. Am. Chem. Soc., 2015, 137, 9438 CrossRef CAS PubMed;
(m) D. T. Ziegler and G. C. Fu, J. Am. Chem. Soc., 2016, 138, 12069 CrossRef CAS PubMed;
(n) Y.-Q. Fang, P. M. Tadross and E. N. Jacobsen, J. Am. Chem. Soc., 2014, 136, 17966 CrossRef CAS PubMed.
- For metal-catalyzed symmetric γ-addition:
(a) R. E. Kinder, Z. Zhang and R. A. Widenhoefer, Org. Lett., 2008, 10, 3157 CrossRef CAS PubMed;
(b) C. Michon, F. Medina, M.-A. Abadie and F. Agbossou-Niedercorn, Organometallics, 2013, 32, 5589 CrossRef CAS;
(c) B. Alcaide, P. Almendros, I. Fernández, R. Martin-Montero, F. MartÍnez-Peña, M. P. Ruiz and M. R. Torres, ACS Catal., 2015, 5, 4842 CrossRef CAS;
(d) R. J. Harris, R. G. Carden, A. N. Duncan and R. A. Widenhoefer, ACS Catal., 2018, 8, 8941 CrossRef CAS.
-
(a)
D. A. Claremon, L. Zhuang, Y. Ye, S. B. Singh, C. M. Tice and G. Mcgeehan, Inhibitors of 11β-Hydroxysteroid Dehydrogenase Type 1, WO/2009/117109, Sep 24, 2009;
(b) L. Sun, J.-H. Ye, W.-J. Zhou, X. Zeng and D.-G. Yu, Org. Lett., 2018, 20, 3049 CrossRef CAS PubMed.
- H. Wang and C. Guo, Angew. Chem., Int. Ed., 2019, 58, 2854 (
Angew. Chem.
, 2019
, 131
, 2880
) CrossRef CAS PubMed.
- S. L. Wiskur and G. C. Fu, J. Am. Chem. Soc., 2005, 127, 6176 CrossRef CAS PubMed.
-
(a) H. E. Burks, S. Liu and J. P. Morken, J. Am. Chem. Soc., 2007, 129, 8766 CrossRef CAS PubMed;
(b) Z. Liu, H.-Q. Ni, T. Zeng and K. M. Engle, J. Am. Chem. Soc., 2018, 140, 3223 CrossRef CAS PubMed.
- K. N. Houk, Y. Lin and F. K. Brown, J. Am. Chem. Soc., 1986, 108, 554 CrossRef CAS PubMed.
- J. Li, W. Chang, W. Ren, J. Dai and Y. Shi, Org. Lett., 2016, 18, 5456 CrossRef CAS PubMed.
- M. Nasopoulou, D. Georgiadis, M. Matziari, V. Dive and A. Yiotakis, J. Org. Chem., 2007, 72, 7222 CrossRef CAS PubMed.
- B. M. Trost and C. Lee, J. Am. Chem. Soc., 2001, 123, 12191 CrossRef CAS PubMed.
Footnotes |
† Electronic supplementary information (ESI) available. CCDC 1819863, 1819864, 1819865 and 1860469. For ESI and crystallographic data in CIF or other electronic format see DOI: 10.1039/c9sc04073k |
‡ These authors contributed equally to this study. |
|
This journal is © The Royal Society of Chemistry 2019 |