DOI:
10.1039/C9RA04488D
(Paper)
RSC Adv., 2019,
9, 29405-29413
One-pot sequential synthesis and antifungal activity of 2-(benzylsulfonyl)benzothiazole derivatives†
Received
14th June 2019
, Accepted 9th September 2019
First published on 17th September 2019
Abstract
New antifungal agrochemicals, derived from 2-(benzylsulfonyl)benzothiazole were synthesized by an environmentally friendly method, using water as reaction medium. These compounds were prepared by a one-pot, two-step synthesis, starting from 2-mercaptobenzothiazole and benzyl halides. The potential fungicides were tested against a panel of phytopathogenic fungi, with many of them showing a significant improvement compared to the non-oxidized analogues and the commercial antifungal Captan. The new derivatives 2-((2-chlorobenzyl)sulfonyl)benzo[d]thiazole (4f) and 2-((4-methylbenzyl)sulfonyl)benzo[d]thiazole (4k) presented remarkable properties, being able to inhibit the growth of two resistant moulds (Aspergillus fumigatus and Aspergillus ustus). Both 4f and 4k could be classified as broad-spectrum fungicides, emerging as possible candidates for the control of these moulds, which have negative impact in food production.
Introduction
Plant and crop protection against plant diseases has been and still is a recurrent issue to ensure food quality.1 The grain crops, such as barley, wheat, oat, soybean, corn and sunflower are the most important feeding source worldwide. Crop rotting and spoilage is mainly caused by fungi, the most important being yeasts and moulds, including Botrytis,2,3 Fusarium,4 Aspergillus,5 Alternaria6 and Cladosporium.3 Botrytis cinerea is a necrotrophic plant pathogenic fungus with a broad host range. It causes the grey mould disease, one of the most important post-harvest diseases of fruits and vegetables worldwide.7 Fusarium oxysporum is one of the most common soil-borne plant pathogenic fungi, causing rot and wilt diseases on many economic plants.8 Aspergillus species are abundant and grow saprotrophically on numerous substrates over a wide range of climatic conditions. They have long been known to be common contaminants of human foods and animal feeds, being able to produce respiratory infections.9,10 Most of these moulds are capable of producing mycotoxins, toxic metabolites produced by fungi, particularly by saprophytic moulds growing on agricultural products.11 These cause not only economic losses but also health hazards to humans and animals.
Chemical protection is one of the most important methods for keeping crops healthy. Recurrent genetic plasticity presented in most mould species, develop resistance to the most common fungicides.1 In addition, results have shown that fungal resistance will accelerate extremely in a few years, causing the appearance of uncontrollable pathogens, which leads to a great challenge to handle this new circumstance.12 This possesses a great concern in community and among professionals, which brings the need for development of safer and more effective agrochemicals with reduced environmental toxicity.13
In the past few decades, various companies have introduced a large number of potent, bioactive fungicides, containing sulfone units, such as oxycarboxin, tazobactam or dapsone (Fig. 1A). A key feature for reaching good bioactivity could be a 5 or 6- membered heterocyclic ring attached to the sulfonyl group.13 Furthermore, benzothiazoles are known for being part of a wide number of bioactive compounds, being many commercial antifungals derived from this heterocycle (Fig. 1B). Benzothiazol-2-thiol and its derivatives are part of an important class of bioactive compounds, having a broad spectrum of bioactivities, such as antimycobacterial.14
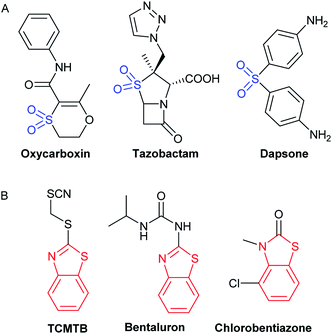 |
| Fig. 1 Commercial bioactive compounds including the structures of interest. (A) Sulfonyl derivatives (blue). (B) Benzothiazole derivatives (red). | |
For example, sulfonylbenzothiazoles with substituents such as Cl, NO2, F, CH3 on the benzothiazole ring have significant antibacterial and antifungal activity.15 In addition, it is known that small molecules, such as benzothiazoles, bind to tubulin, interfering in the mitosis process, and, in consequence leading to apoptosis.16
Organic sulfones are considered useful precursors in organic synthesis due to their ability to attract electrons, stabilizing carbanions and radical intermediates during different chemical reactions.17–19 Additionally, sulfonyl groups can be easily removed, making them very versatile synthetic intermediates.20 On these bases, there has been great interest in the development of methods for the synthesis of sulfonyl derivatives. A widely used method for their preparation is the oxidation of different sulfides or sulfoxides in the presence of a suitable oxidizing agent. Hydrogen peroxide is among the most important oxidants for transforming sulfur groups to sulfones.21–24 Additionally, permanganate salts,13,25–27 m-chloroperoxybenzoic acid,28–31 oxone,32–34 periodate salts35 and orthoperiodic acid,36 can be mentioned as efficient oxidants. However, some synthetic protocols have one or more disadvantages, such as the use of large excess of oxidant,21,23,36,37 or the need for simultaneous application of two oxidants.24,31 Some synthetic pathways imply the use of sophisticated or toxic catalysts,21–23,33–37 in addition to previous in situ preparation of reagents.22–24,27,33,35,37 Furthermore, most of these methods involve the use of problematic or hazardous solvents.21–24,26,28–38 Thus, the oxidation of sulfides to sulfones using water as reaction medium should be advantageous. However, to our knowledge the use of water in such oxidations has not been reported.
We have previously reported the synthesis of 2-(benzylthio)benzothiazoles, including the verification of its bioactivity against different phytopathogenic fungi.39 Thus, the main goal of this work was to obtain several 2-(benzylsulfonyl)benzothiazole derivatives by a simple and eco-friendly methodology, using water as solvent, evaluating their antifungal activity against phytopathogenic fungi, Botrytis cinerea, Fusarium oxysporum and Aspergillus spp. Additionally, we were interested in the evaluation of differences in the antifungal activity of oxidized derivatives prepared in this work versus non-oxidized compounds previously prepared.
Results and discussion
Chemistry
Synthesis. In order to synthesize 2-benzylsulfonyl benzothiazole derivatives, we used a two-step synthetic pathway (Scheme 1). In the first step, 2-mercaptobenzothiazole (1) and different benzyl halides (2a–k) react in a nucleophilic substitution reaction to obtain the respective sulfides (3a–k).39 Those sulfides were oxidized in the second step to obtain the corresponding sulfones (4a–k).
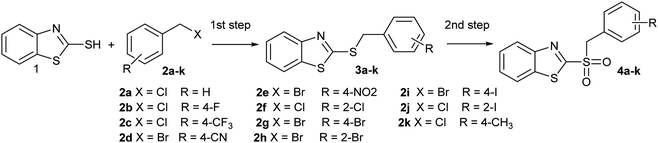 |
| Scheme 1 Two-step pathway proposed for sequential synthesis of 2-benzylsulfonyl benzothiazoles. | |
Compounds 3a–k were prepared according to our previous work,39 obtaining sulfides in very good isolated yields (72–93%), under mild conditions, and employing water as the reaction medium. Water is the preferred solvent, as it is low cost, abundant and safe, so reducing environmental effects. Due to its unique physicochemical properties, it is possible to perform a wide range of reactions with high selectivity and usually reaching good yields and rate increments, compared to organic solvents.40
In order to perform the oxidation reaction on the sulfides previously synthesized, we used reaction conditions previously developed by Lai et al.26 Thus, compounds 3a–k were oxidized by KMnO4, using FeCl3 as catalyst and acetonitrile as solvent. After 90 minutes of reaction at room temperature, sulfones 4a–k were obtained, yielding full substrate conversion, in addition to high selectivity towards sulfide oxidation against other functional groups present in the compounds (Table 1). Compounds 4a–k were obtained pure after solvent extraction, using water and ethyl acetate to remove the KMnO4 remaining. So, we got very good isolated yields (75–86%), being unnecessary further chromatographic purification.
Table 1 Oxidation reactions of sulphurs 3a
In an attempt to obtain 2-benzylsulfonyl benzoxazole derivatives (6a–h), we tried to oxidize the respective sulfides (5a–h) using the same conditions as for benzothiazoles (Scheme 2), but yields were variable (0–86%), after 24 hours of reaction. This could be due to complexation of MnO4− with the oxygen in the heterocycle, instead of interacting with the sulfide, hence the oxidant is deactivated. However, these sulfonyl derivatives were very unstable, and within a few hours to one week they decomposed. More studies are needed to determine the reasons for the variable reactivity, as well as the lack of stability for these compounds.
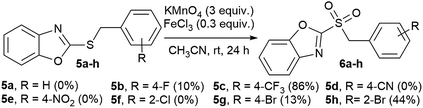 |
| Scheme 2 Oxidation of benzoxazole derivatives. Products detected by GC-MS. Yields obtained by GC are in brackets. | |
In addition, looking to simplify the synthetic process, we designed a one-pot sequential method, starting from 2-mercaptobenzothiazole (1) and benzyl halides (2a–k), to obtain compounds 4a–k, without isolating the respective synthetic intermediate 3a–k. For that reason, it was important to perform the synthesis in a single solvent, saving both solvent and workup time. That is why the sequential synthesis was tried using either water or acetonitrile. Thus, we obtained good conversions of 2 in both solvents (Table 2). Moreover, we did not detect remaining substrate in reactions where water was used as reaction medium, which also facilitated the purification. These facts, in addition to environmentally friendly solvent, makes water the solvent of choice.40 To our knowledge, there are no reports on sulfide oxidation carried out using water as the exclusive reaction medium. There were two cases where 4 derivatives were not fully converted. Thus, 4a and 4k (Table 2, entries 1 and 11), showed the presence of 3a or 3k, which were remaining intermediated in the reaction. This partial conversion was observed even after 24 h reaction to reach the equilibrium. This may be due to electronic influences of the benzyl group on the oxidizable sulfur atom, since they were the only two cases where the ring was not substituted by electron-withdrawing groups.
Table 2 One-pot sequential synthesis of 2-(benzylsulfonyl)benzothiazoles 4a
Comparing the global isolated yields of the final products, using the one-pot reaction pathway (75–89%)or the two independent steps methodology (60–75%), a greater efficiency for the one-pot method can be observed. Thus, the one-pot pathway allowed improving up to 26% for product recovery (Table 2). This is a consequence of the diminution in handling and workup. Furthermore, in the one-pot method, a chromatographic method is not required to isolate the final product, since a simple extraction with water and ethyl acetate is enough to obtain the product with high purity.
Antifungal susceptibility test
Fungal growth was evaluated at six concentrations (3.1–100 mg L−1), using enriched Czapek broth in the presence of compounds 4. Each treatment was carried out in triplicate. The 50% Inhibitory Concentration (IC50) was defined as the minimum concentration required to inhibit the fungal growth by 50%. IC50 values of the compounds, along with the positive control Captan, are summarized in a heat map in Table 3. Significant differences were determined by calculating the t-Student hypothesis test P, at 95% confidence.
Table 3 Antifungal activity of benzothiazole derivatives 4
Heat map scale. Red: most active compounds (low IC50), orange/yellow: compounds with intermediate activity, green: non-active compounds (high IC50). (–): No inhibitory activity detected (IC50 > 100 mg L−1). 60% inhibition at 3.1 mg L−1. 70% inhibition at 3.1 mg L−1. |
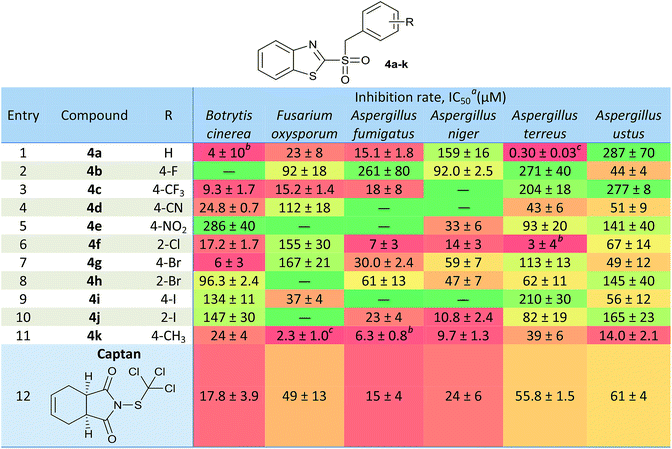 |
In general, a good bioactivity was observed compared to the reference compound, finding candidates with equal or significantly better inhibitory effects than Captan. Also, in many cases, an increment in the bioactivity was observed for derivatives 4a–k, when compared to the results obtained for the unoxidized analogues 3a–k.39 In those cases where IC50 were lower than the minimum concentration assayed (3.1 mg L−1), it was specified the inhibition percentage observed at that concentration as a footnote in Table 3.
For Botrytis cinerea, compounds 4a (R = H, IC50 = 4 μM, Table 3, entry 1), 4f (R = 2-Cl, IC50 = 17.2 μM, Table 3, entry 6) and 4k (R = 4-CH3, IC50 = 24 μM, Table 3, entry 11) showed inhibitory effects significantly equal to Captan (IC50 = 17.8 μM, Table 3, entry 12). Derivatives 4c (R = 4-CF3, IC50 = 9.3 μM, Table 3, entry 3) and 4g (R = 4-Br, IC50 = 6 μM, Table 3, entry 7) achieved significant improvements respect to the control. On the other hand, compared to the activity observed of the sulfides derivatives 3 with the oxidized analogues 4, it could be appreciated that for the analogue 3a (IC50 > 100 mg L−1)39 antifungal activity was substantially improved for 4a (IC50 = 4 μM).
Regarding Fusarium oxysporum, derivatives 4a (R = H, IC50 = 23 μM, Table 3, entry 1), 4c (R = 4-CF3, IC50 = 15.2 μM, Table 3, entry 3) and 4k (R = 4-CH3, IC50 = 2.3 μM, Table 3, entry 11) proved to have a significantly better activity than the control (IC50 = 49 μM, Table 3, entry 12). In addition, the compound 4i (R = 4-I, IC50 = 37 μM, Table 3, entry 9) achieved an inhibition rate comparable to that of Captan. On the other hand, oxidized analogues such as 4c (IC50(3c) = 57 μM), 4i (IC50(3i) = 73 μM) and 4k (IC50(3k) = 79 μM) improved their bioactivity compared to the analogues 3.39 The candidate that stood out against F. oxysporum was 4k, which achieved a growth inhibition of 70% at the lowest concentration tested.
Against A. fumigatus, derivatives 4a (R = H, IC50 = 15.1 μM, Table 3, entry 1), 4c (R = 4-CF3, IC50 = 18 μM, Table 3, entry 3), 4f (R = 2-Cl, IC50 = 7 μM, Table 3, entry 6) and 4j (R = 2-I, IC50 = 23 μM, Table 3, entry 10) showed a similar effect that to Captan (IC50 = 15 μM, Table 3, entry 12). The derivative 4k stands out (R = 4-CH3, IC50 = 6.3 μM, Table 3, entry 11), since it significantly exceeded the inhibition produced by the control. This last compound becomes a very valuable candidate, since it was the only compound showing improvements in the potency against A. fumigatus, respect to the commercial control. Also, oxidized analogues such as 4a (IC50(3a) = 267 μM), 4c (IC50(3c) > 100 mg L−1), 4f (IC50(3f) > 100 mg L−1) and 4k (IC50(3k) = 29 μM) showed improvement respect the sulfur derivatives 3.39
In case of Aspergillus niger, derivatives 4e (R = 4-NO2, IC50 = 33 μM, Table 3, entry 5) and 4f (R = 2-Cl, IC50 = 14 μM, Table 3, entry 6) equaled the reference's potency (IC50 = 24 μM, Table 3, entry 12). On the other hand, 4k (R = 4-CH3, IC50 = 9.7 μM, Table 3, entry 11) was significantly more potent, proving to be the best candidate in the series. Compared to the analogues 3, 4f (IC50(3f) > 100 mg L−1) and 4k (IC50(3k) = 35 μM) were significantly better against A. niger.39
The best candidates selected against Aspergillus terreus were 4a (R = H, IC50 = 0.30 μM, Table 3, entry 1), 4d (R = 4-CN, IC50 = 43 μM, Table 3, entry 4), 4f (R = 2-Cl, IC50 = 3 μM, Table 3, entry 6) and 4k (R = 4-CH3, IC50 = 39 μM, Table 3, entry 11), which proved to be significantly better than Captan (IC50 = 55.8 μM, Table 3, entry 12). Also, derivatives 4h (R = 2-Br, IC50 = 62 μM, Table 3, entry 8) and 4j (R = 2-I, IC50 = 82 μM, Table 3, entry 10) showed inhibition rates comparable to the commercial compound. On the other hand, oxidized analogues such as 4a (IC50(3a) = 13.2 μM), 4f (IC50(3f) = 73 μM), 4h (IC50(3h) > 100 mg L−1), 4j (IC50(3j) > 100 mg L−1) and 4k (IC50(3k) > 100 mg L−1) were better than the unoxidized derivatives.39 Of these compounds, we can highlight 4a and 4f, which have the lowest IC50 of the series against A. terreus.
Against Aspergillus ustus, it was possible to determine that derivatives 4d (R = 4-CN, IC50 = 51 μM, Table 3, entry 4), 4f (R = 2-Cl, IC50 = 67 μM, Table 3, entry 6), 4g (R = 4-Br, IC50 = 49 μM, Table 3, entry 7) and 4i (R = 4-I, IC50 = 56 μM, Table 3, entry 9) did not show significant differences with respect to the IC50 obtained for Captan (IC50 = 61 μM, Table 3, entry 12). Derivatives 4b (R = 4 F, IC50 = 44 μM, Table 3, entry 2) and 4k (R = 4-CH3, IC50 = 14.0 μM, Table 3, entry 11) exceeded the control's inhibition rate. Thus, oxidized analogues such as 4d (IC50(3d) = 92 μM), 4f (IC50(3f) = 229 μM), 4g (IC50(3g) > 100 mg L−1), 4i (IC50(3i) > 100 mg L−1) and 4k (IC50(3k) = 17 mg L−1, 62 μM) improved their effect with respect to the synthetic intermediate.39
When analyzing the potencies of the sulfonyl derivatives 4 with those of their non-oxidized analogues 3, it is observed that the oxidation of the sulfide bridge was beneficial in most cases. This may be due to the increased hydrophilicity of the sulfonyl derivatives, allowing a more efficient membrane permeation. In addition, the SO2 group would provide two new hydrogen bond acceptors, which would increase the interactions in the ligand-target binding.
Considering results obtained in the antifungal susceptibility tests, key candidates can be highlighted, according to their broad action. Among the candidates that showed broad-antifungal activity, the derivative 4f (R = 2-Cl) can be highlighted, since it showed a remarkable inhibitory effect for five out of six microorganisms studied. Furthermore, the derivative 4k (R = 4-CH3), achieved an appreciable inhibition growth with six fungi evaluated, being remarkable the activity observed against F. oxysporum and Aspergillus spp., especially A. fumigatus and A. ustus, which are highly resistant species. The derivative 4c (R = 4-CF3) can be also highlighted, because it allowed a selective growth of the different Aspergillus strains, reaching an adequate inhibition against B. cinerea and F. oxysporum. This may have positive implications in the design of combined therapies of biological and chemical control. Aspergillus is a microorganism widely used in biological control treatments,41 so that a combined treatment could be designed, using Aspergillus with one of these antifungals that allow its development and, thus, improving the efficiency against a problematic pest.
Conclusions
In conclusion, we present a simple, cheap, one-pot methodology to obtain 2-(benzylsulfonyl)benzothiazole derivatives, using a two-step synthetic pathway, affording very good isolated yields, without using a final chromatographic step for product purification. Those compounds were evaluated against six phytopathogenic or problematic fungi, finding a series of candidates which improved their activity with respect to a commercial (Captan), and also compared to their sulfur synthetic intermediates, previously assayed.39 It is worth remarking that some of these compounds were able to inhibit the growth of A. fumigatus and A. ustus, which proved to be resistant to a series of benzothiazole and benzoxazole derivatives. We put a highlight on derivatives 4f (R = 2-Cl) and 4k (R = 4-CH3), since they are new compounds that showed the best inhibition power against these fungi species.
Further studies are ahead, in order to get closer to a mode of action for these compounds. This includes structure–activity relationships, and computational studies. The present work constitutes a contribution to the design, optimization and redesign cycle for bioactive compounds, which will lead us to better and safer agrochemicals in the future.
Experimental
Chemicals
2-Mercaptobenzothiazole, benzyl halides, K2CO3, KMnO4, FeCl3, acetonitrile were commercially available (Sigma, St. Louis, MO) and used as received. Ultrapure water (MilliQ) was used for reactions on water.
Microorganisms
Synthesized compounds were assayed against: (a) Botrytis cinerea isolated from Vitis vinifera cv. Chardonnay, cultivated in Province of San Juan, Argentina; (b) Aspergillus ustus (PN-S4); (c) Aspergillus terreus (M16C); (d) Fusarium oxysporum (M15-Pa) (strains b–d were isolated from soil samples from the Province of San Juan, Argentina); (e) Aspergillus fumigatus (ATTC 26934); (f) Aspergillus niger (ATCC 9029). The microorganisms were grown in Czapek medium (Sigma, St. Louis, MO), enriched with a solution of mineral salts, incubated at 30 °C for Aspergillus spp. and Fusarium oxysporum, and at 22 °C for Botrytis cinerea, during 5–10 days.
General procedure for the oxidation of 2-(benzylthio)benzothiazoles
2-(Benzylthio)benzothiazole (0.2 mmol), KMnO4 (0.6 mmol) and FeCl3 (0.06 mmol) were vigorously stirred in 2 mL of acetonitrile for 90 minutes at room temperature in a sealed vessel. When the reaction was finished, 2 mL of water was added to the crude, and it was extracted with ethyl acetate (three times, 4 mL each), and washed the organic layer with H2O (three times, 4 mL each). The organic layer was dried with anhydrous Na2SO4, and analyzed by Gas Chromatography (GC).
General procedure for the one-pot synthesis of 2-(benzylsulfonyl)benzothiazoles
2-Mercaptobenzothiazole (0.5 mmol), K2CO3 (0.5 mmol) and benzyl halide (0.5 mmol) were vigorously stirred in 2 mL of Ultrapure water for 30 minutes at 50 °C in a sealed vessel. After completion, the reaction media was neutralized by adding a few drops of acetic acid, with further addition of KMnO4 (1.5 mmol), FeCl3 (0.15 mmol) and Ultrapure water (2 mL). The reaction vessel was sealed and let to react for 90 minutes at room temperature. When the reaction was completed, 4 mL of water were added to the crude yield, followed by extraction with ethyl acetate (three times, 8 mL each), with further washing of the organic layer with H2O (three times, 8 mL each). The organic layer was dried with anhydrous Na2SO4 and analyzed by GC.
Antifungal susceptibility test
Bioactivity was determined by microdilution method, in accordance to Clinical and Laboratory Standard Institute (CLSI formerly the National Committee for Clinical Laboratory Standards [NCCLS]) (M27-A3 for yeasts and filamentous fungi) (moulds; the M38-A2 document). Assays were performed in enriched Czapek broth. The inoculum employed was 1–5 × 105 conidia per mL. Stock solutions of tested compounds were prepared in DMSO to give serial two-fold dilutions to final concentrations of 3.1–100 mg L−1 (final DMSO concentration ≤ 2%). Microtiter plates were incubated at 30 °C for Aspergillus and Fusarium spp., and at 22 °C for B. cinerea in a moist and dark chamber. Inhibitory concentrations were recorded after 48 h for Aspergillus and Fusarium spp., and after 72 h for B. cinerea, according to the control fungal growth. The positive control of the commercial antifungal Captan and solvent control, using 2% aqueous DMSO, were included. All tests were run in triplicate. The 50% Inhibitory Concentration (IC50) was defined as the minimum concentration of the compound that resulted in 50% inhibition of the fungal growth. IC50 values were calculated by linear regression plots of % (inhibition) vs. ln(concentration), considering 50% inhibition.
Spectral information
See spectroscopy data of compounds 5a–h.39
2-(Benzylsulfonyl)benzo[d]thiazole, 4a42. Yellow solid. mp: 103.8–106.0 °C. 1H-NMR (400 MHz, CDCl3), δ (ppm): 4.76 (s, 2H), 7.26–7.32 (m, 5H), 7.58 (t, J = 7.3 Hz, 1H), 7.65 (t, J = 7.3 Hz, 1H), 7.94 (d, J = 8.0 Hz, 1H), 8.26 (d, J = 8.2 Hz, 1H). 13C-NMR (100 MHz, CDCl3), δ (ppm): 61.03, 122.30, 125.50, 126.36, 127.66, 128.01, 128.92, 129.24, 131.13, 137.08, 152.59, 165.21. MS (EI+) m/z (%): 289.00 (3), 225.15 (20), 224.05 (52), 91.05 (100), 65.05 (17). HRMS (ESI): (M + Na): C14H11NO2S2Na, calculated: 312.0123, found: 312.0138.
2-((4-Fluorobenzyl)sulfonyl)benzo[d]thiazole, 4b. White solid. mp: 141.8–143.4 °C. 1H-NMR (400 MHz, CDCl3), δ (ppm): 4.72 (s, 2H), 6.96 (t, J = 8.6 Hz, 2H), 7.25 (dd, J = 8.5 Hz, J = 5.2 Hz, 2H), 7.58 (t, J = 7.5 Hz, 1H), 7.64 (t, J = 7.2 Hz, 1H), 7.95 (d, J = 8.1 Hz, 1H), 8.24 (d, J = 8.1 Hz, 1H). 13C-NMR (100 MHz, CDCl3), δ (ppm): 60.13, 116.05 (d, J2F–C = 21.8 Hz), 122.26 (d, J4F–C = 3.4 Hz), 122.34, 125.48, 127.73, 128.11, 132.96 (d, J3F–C = 8.5 Hz), 137.01, 152.56, 163.30 (d, J1F–C = 249.4 Hz), 165.02. MS (EI+) m/z (%): 306.90 (4), 242.95 (9), 241.95 (26), 110.00 (7), 109.00 (100), 108.05 (2), 107.00 (3), 90.00 (4), 89.00 (2), 83.00 (17), 68.95 (2), 63.00 (6). HRMS (ESI): (M + Na): C14H10NO2S2FNa, calculated: 330.0029, found: 330.0039.
2-((4-(Trifluoromethyl)benzyl)sulfonyl)benzo[d]thiazole, 4c29. White solid. mp: 180.1–181.5 °C. 1H-NMR (400 MHz, CDCl3), δ (ppm): 4.81 (s, 2H), 7.43 (d, J = 8.0, 2H), 7.55 (d, J = 8.1, 2H), 7.59 (t, J = 8.0, 1H), 7.66 (t, J = 7.3, 1H), 7.96 (d, J = 7.9, 1H), 8.25 (d, J = 8.2, 1H). 13C-NMR (100 MHz, CDCl3), δ (ppm): 60.35, 122.37, 123.84 (q, J1F–C = 272.7 Hz), 125.50, 125.83 (q, J3F–C = 3.7 Hz), 127.83, 128.24, 131.29 (d, J2F–C = 32.7 Hz), 131.55, 136.96, 152.51, 164.82. MS (EI+) m/z (%): 356.95 (4), 293.10 (34), 292.15 (63), 222.95 (7), 159.10 (100), 133.85 (5), 118.90 (9), 109.15 (30), 107.90 (6), 88.95 (5), 69.00 (4), 63.00 (10). HRMS (ESI): (M + Na): C15H10NO2S2F3Na, calculated: 379.9997, found: 379.9989.
4-((Benzo[d]thiazol-2-ylsulfonyl)methyl)benzonitrile, 4d. White solid. mp: 185.5–186.8 °C. 1H-NMR (400 MHz, CDCl3), δ (ppm): 4.81 (s, 2H), 7.41 (d, J = 8.1 Hz, 2H), 7.57 (d, J = 8.0 Hz, 2H), 7.61 (d, J = 7.7 Hz, 1H), 7.66 (t, J = 7.6 Hz, 1H), 7.96 (d, J = 8.0 Hz, 1H), 8.24 (d, J = 8.1 Hz, 1H). 13C-NMR (100 MHz, CDCl3), δ (ppm): 60.46, 113.35, 118.01, 122.41, 125.52, 127.93, 128.38, 131.73, 131.88, 132.53, 136.90, 152.44, 164.55. MS (EI+) m/z (%): 314.20 (4), 250.25 (31), 249.15 (65), 116.15 (100), 90.15 (13), 89.15 (30), 63.10 (12). HRMS (ESI): (M + Na): C15H10N2O2S2Na, calculated: 337.0076, found: 337.0091.
2-((4-Nitrobenzyl)sulfonyl)benzo[d]thiazole, 4e. White solid. mp: 205.5–207.2 °C. 1H-NMR (400 MHz, DMSO-d6), δ (ppm): 5.35 (s, 2H), 7.61 (d, J = 8.2 Hz, 2H), 7.68–7.77 (m, 2H), 8.21 (d, J = 8.2 Hz, 1H), 8.29–8.32 (m, 2H). 13C-NMR (100 MHz, DMSO-d6), δ (ppm): 59.48, 123.99 (overlap), 125.45, 128.57, 128.80, 133.17, 135.28, 136.92, 148.26, 152.48, 165.79. MS (EI+) m/z (%): 334.90 (3), 333.90 (16), 270.90 (15), 269.95 (66), 223.95 (15), 222.95 (42), 136.00 (35), 135.00 (14), 133.95 (14), 110.00 (1), 109.05 (4), 107.95 (10), 107.00 (13), 106.05 (72), 91.00 (11), 90.00 (74), 89.00 (69), 79.05 (9), 78.00 (100), 77.00 (13), 69.00 (14), 64.00 (33), 63.00 (43). HRMS (ESI): (M + Na): C14H10N2O4S2Na, calculated: 356.9974, found: 356.9985.
2-((2-Chlorobenzyl)sulfonyl)benzo[d]thiazole, 4f. White solid. mp: 123.2–124.3 °C. 1H-NMR (400 MHz, CDCl3), δ (ppm): 4.97 (s, 2H), 7.23 (td, J = 7.5 Hz, J = 1.4 Hz, 1H), 7.28 (td, J = 7.8 Hz, J = 1.6 Hz, 1H), 7.33 (d, J = 7.8 Hz, 1H), 7.42 (dd, J = 7.5 Hz, J = 1.3 Hz, 1H), 7.58 (td, J = 7.1 Hz, J = 0.9 Hz, 1H), 7.64 (td, J = 7.7 Hz, J = 1.2 Hz, 1H), 7.96 (d, J = 8.1 Hz, 1H), 8.24 (d, J = 8.1 Hz, 1H). 13C-NMR (100 MHz, CDCl3), δ (ppm): 58.09, 122.29, 125.06, 125.63, 127.26, 127.70, 128.11, 130.01, 130.76, 133.09, 135.75, 137.31, 152.70, 164.93. MS (EI+) m/z (%): 288.15 (33), 261.20 (2), 260.10 (9), 259.10 (5), 258.10 (22), 224.10 (22), 223.05 (12), 127.10 (32), 125.10 (100), 99.05 (7), 90.10 (11), 89.10 (23), 63.05 (11). HRMS (ESI): (M + Na): C14H10NO2S2ClNa, calculated: 345.9734, found: 345.9742.
2-((4-Bromobenzyl)sulfonyl)benzo[d]thiazole, 4g28. White solid. mp: 151.6–153.6 °C. 1H-NMR (400 MHz, CDCl3), δ (ppm): 4.71 (s, 2H), 7.15 (d, J = 8.4 Hz, 2H), 7.41 (d, J = 8.4 Hz, 2H), 7.60 (td, J = 7.6 Hz, J = 1.1 Hz, 1H), 7.66 (td, J = 7.7 Hz, J = 1.1 Hz, 1H), 7.97 (d, J = 7.9 Hz, 1H), 8.25 (d, J = 8.2 Hz, 1H). 13C-NMR (100 MHz, CDCl3), δ (ppm): 60.26, 122.39, 123.87, 125.42, 125.50, 127.78, 128.17, 132.16, 132.66, 136.99, 152.53, 164.88. MS (EI+) m/z (%): 369.05 (5), 367.05 (5), 305.10 (14), 304.10 (32), 303.10 (13), 302.10 (29), 223.15 (14), 171.05 (76), 169.05 (100), 90.15 (56), 89.10 (35), 63.05 (17). HRMS (ESI): (M + Na): C14H10NO2S2BrNa, calculated: 389.9229, found: 389.9241.
2-((2-Bromobencyl)sulfonil)benzo[d]tiazol, 4h. White solid. mp: 147.2–148.7 °C. 1H-NMR (400 MHz, CDCl3), δ (ppm): 4.99 (s, 2H), 7.21 (t, J = 7.1 Hz, 1H), 7.29 (t, J = 7.3 Hz, 1H), 7.44 (d, J = 7.3 Hz, 1H), 7.52 (d, J = 7.8 Hz, 1H), 7.59 (t, J = 7.3 Hz, 1H), 7.65 (t, J = 7.4 Hz, 1H), 7.97 (d, J = 8.0 Hz, 1H), 8.24 (d, J = 8.1 Hz, 1H). 13C-NMR (100 MHz, CDCl3), δ (ppm): 60.51, 122.29, 125.66, 126.18, 126.91, 127.71, 127.90, 128.11, 130.90, 133.12, 133.39, 137.38, 152.71, 164.86. MS (EI+) m/z (%): 304.85 (5), 303.85 (20), 302.90 (5), 301.90 (19), 288.90 (12), 287.95 (65), 223.95 (35), 222.95 (22), 170.90 (66), 168.90 (67), 91.05 (9), 90.00 (100), 89.00 (64), 64.00 (14), 63.00 (35). HRMS (ESI): (M + Na): C14H10NO2S2BrNa, calculated: 389.9229, found: 389.9226.
2-((4-Iodobenzyl)sulfonyl)benzo[d]thiazole, 4i. White solid. mp: 177.5–179.2 °C. 1H-NMR (400 MHz, CDCl3), δ (ppm): 4.70 (s, 2H), 7.01 (d, J = 8.2 Hz, 2H), 7.79–7.48 (m, 4H), 7.97 (d, J = 8.1 Hz, 1H), 8.25 (d, J = 8.1 Hz, 1H). 13C-NMR (100 MHz, CDCl3), δ (ppm): 60.39, 95.68, 122.39, 125.50, 126.04, 127.77, 128.16, 132.79, 136.99, 138.12, 152.54, 164.90. MS (EI+) m/z (%): 414.85 (8), 351.00 (24), 349.95 (29), 224.15 (18), 223.25 (14), 217.05 (100), 90.15 (66), 89.10 (37), 63.00 (22). HRMS (ESI): (M + Na): C14H10NO2S2INa, calculated: 437.9090, found: 437.9088.
2-((2-Iodobenzyl)sulfonil)benzo[d]tiazol, 4j. White solid. mp: 162.1–163.4 °C. 1H-NMR (400 MHz, CDCl3), δ (ppm): 5.00 (s, 2H), 7.02 (t, J = 7.6 Hz, 1H), 7.32 (t, J = 7.5 Hz, 1H), 7.45 (d, J = 7.7 Hz, 1H), 7.59 (dd, J = 11.6, 4.5 Hz, 1H), 7.64 (dd, J = 11.2, 4.1 Hz, 1H), 7.81 (d, J = 7.9 Hz, 1H), 7.97 (d, J = 8.2 Hz, 1H), 8.25 (d, J = 8.0 Hz, 1H). 13C-NMR (100 MHz, CDCl3), δ (ppm): 64.96, 102.18, 122.28, 125.69, 127.69, 128.10, 128.71, 130.51, 130.82, 132.35, 137.44, 140.22, 152.74, 164.96. MS (EI+) m/z (%): 350.80 (4), 349.80 (18), 287.90 (52), 223.95 (25), 222.95 (16), 216.85 (58), 91.05 (10), 90.00 (100), 89.05 (48), 64.00 (12), 63.05 (24). HRMS (ESI): (M + Na): C14H10NO2S2INa, calculated: 437.9090, found: 437.9076.
2-((4-Methylbenzyl)sulfonyl)benzo[d]thiazole, 4k. White solid. mp: 113.3–115.1 °C. 1H-NMR (400 MHz, CDCl3), δ (ppm): 2.30 (s, 3H), 4.72 (s, 2H), 7.08 (d, J = 7.9 Hz, 2H), 7.15 (d, J = 8.0 Hz, 2H), 7.59 (dd, J = 11.2 Hz, J = 4.0 Hz, 1H), 7.65 (dd, J = 11.3 Hz, J = 4.1 Hz, 1H), 7.95 (d, J = 8.1 Hz, 1H), 8.26 (d, J = 8.2 Hz, 1H). 13C-NMR (100 MHz, CDCl3), δ (ppm): 21.22, 60.74, 122.30, 123.17, 125.49, 127.61, 127.95, 129.65, 130.98, 137.07, 139.27, 152.63, 165.41. MS (EI+) m/z (%): 303.15 (4), 239.25 (7), 238.15 (22), 223.10 (2), 105.15 (100), 90.10 (3), 77.05 (10), 63.00 (3). HRMS (ESI): (M + Na): C15H13NO2S2Na, calculated: 326.0280, found: 326.0284.
2-((4-Fluorobenzyl)sulfonyl)benzo[d]oxazole, 6b. MS (EI+) m/z (%): 291.20 (1), 226.15 (23), 227.15 (7), 110.20 (8), 109.00 (100), 108.05 (2), 107.00 (5), 93.95 (1), 90.95 (1), 90.00 (3), 89.00 (2), 83.00 (11), 68.95 (2), 64.00 (6), 63.00 (7).
2-((4-(Trifluoromethyl)benzyl)sulfonyl)benzo[d]oxazole, 6c. MS (EI+) m/z (%): 341.05 (2), 278.20 (2), 277.15 (20), 276.10 (58), 249.15 (2), 248.10 (7), 208.10 (4), 160.10 (9), 159.05 (100), 120.15 (1), 119.10 (7), 110.10 (2), 109.10 (25), 107.10 (1), 91.10 (2), 90.10 (8), 89.00 (4), 65.05 (1), 64.00 (7), 63.05 (9). HRMS (ESI): (M + Na): C15H10NO3SF3Na, calculated: 364.0226, found: 364.0234.
2-((4-Bromobenzyl)sulfonyl)benzo[d]oxazole, 6g. MS (EI+) m/z (%): 353.95 (1), 353.00 (3), 351.00 (3), 289.95 (1), 289.15 (7), 288.15 (24), 287.15 (7), 286.15 (24), 209.15 (1), 208.15 (6), 207.15 (3), 172.10 (7), 171.05 (77), 170.10 (6), 169.05 (100), 91.10 (6), 90.15 (53), 89.10 (32), 65.05 (1), 64.05 (12), 63.05 (17).
2-((2-Bromobenzyl)sulfonyl)benzo[d]oxazole, 6h. MS (EI+) m/z (%): 289.10 (3), 288.10 (16), 287.10 (3), 286.05 (17), 273.20 (119), 272.15 (75), 209.15 (6), 208.15 (37), 207.05 (10), 172.05 (7), 170.95 (83), 169.00 (100), 91.10 (8), 90.10 (66), 89.05 (45), 87.10 (1), 65.05 (2), 64.05 (14), 63.05 (23), 62.05 (5). HRMS (ESI): (M + Na): C14H10NO3SBrNa, calculated: 373.9457, found: 373.9470.
Conflicts of interest
There are no conflicts of interest to declare.
Acknowledgements
This work was supported in part by CONICET, CICITCA UNSJ, SECYT and ANPCyT. M. S. B. and N. H. C. gratefully acknowledge receipt of a fellowship from CONICET.
Notes and references
- P. E. Russell, J. Agric. Sci., 2005, 143, 11–25 CrossRef CAS.
- Y. Elad, Biol. Rev., 1997, 72, 381–422 CrossRef.
- Y. Elad, Biocontrol Sci. Technol., 2000, 10, 499–507 CrossRef.
- A. D. Omer, J. Granett and R. J. Wakeman, J. Phytopathol., 1999, 147, 433–436 CrossRef.
- C. S. Carranza, C. L. Barberis, S. M. Chiacchiera and C. E. Magnoli, Rev. Argent. Microbiol., 2017, 49, 384–393 Search PubMed.
- Y. Wang, Y. Bao, D. Shen, W. Feng, T. Yu, J. Zhang and X. D. Zheng, Int. J. Food Microbiol., 2008, 123, 234–239 CrossRef PubMed.
- J. P. Munafo and T. J. Gianfagna, J. Agric. Food Chem., 2011, 59, 5945–5954 CrossRef CAS PubMed.
- M. S. M. Mohamed, A. M. Saleh, I. B. Abdel-Farid and S. A. El-Naggar, Pestic. Biochem. Physiol., 2017, 141, 57–64 CrossRef CAS PubMed.
- P.-K. Chang, B. W. Horn, K. Abe and K. Gomi, in Encyclopedia of Food Microbiology, 2014, vol. 1, pp. 77–82 Search PubMed.
- F. Álvarez Lerma, P. Olaechea Astigarraga, M. Palomar Martínez, M. Rodríguez Carvajal, J. F. Machado Casas, M. M. Jiménez Quintana, F. Esteve Urbano, J. C. Ballesteros Herráez and E. Zavala Zegarra, Med. Intensiva, 2015, 39, 149–159 CrossRef PubMed.
- P. Bhatnagar-Mathur, S. Sunkara, M. Bhatnagar-Panwar, F. Waliyar and K. K. Sharma, Plant Sci., 2015, 234, 119–132 CrossRef CAS PubMed.
- X. Pan, S. Xu, J. Wu, J. Luo, Y. Duan, J. Wang, F. Zhang and M. Zhou, Pestic. Biochem. Physiol., 2018, 145, 8–14 CrossRef CAS PubMed.
- W. Xu, J. He, M. He, F. Han, X. Chen, Z. Pan, J. Wang and M. Tong, Molecules, 2011, 16, 9129–9141 CrossRef CAS PubMed.
- J. Kočí, V. Klimešová, K. Waisser, J. Kaustová, H.-M. Dahse and U. Möllmann, Bioorg. Med. Chem. Lett., 2002, 12, 3275–3278 CrossRef.
- R. S. Keri, M. R. Patil, S. a. Patil and S. Budagumpi, Eur. J. Med. Chem., 2015, 89, 207–251 CrossRef CAS PubMed.
- D. E. McNamara, S. Senese, T. O. Yeates and J. Z. Torres, Protein Sci., 2015, 24, 1164–1172 CrossRef CAS PubMed.
- F. Chemla, J. Chem. Soc., Perkin Trans. 1, 2002, 275–299 RSC.
- T. G. Back, Tetrahedron, 2001, 57, 5263–5301 CrossRef CAS.
- L. A. Paquette, Synlett, 2001, 2001, 1–12 CrossRef.
- C. Nájera and M. Yus, Tetrahedron, 1999, 55, 10547–10658 CrossRef.
- C. Zhao, Y. Zhao, H. Chai and P. Gong, Bioorg. Med. Chem., 2006, 14, 2552–2558 CrossRef CAS PubMed.
- M. Rahimizadeh, G. Rajabzadeh, S. M. Khatami, H. Eshghi and A. Shiri, J. Mol. Catal. A: Chem., 2010, 323, 59–64 CrossRef CAS.
- R. Afrasiabi, M. R. Farsani and B. Yadollahi, Tetrahedron Lett., 2014, 55, 3923–3925 CrossRef CAS.
- B. Maleki, S. Hemmati, A. Sedrpoushan, S. S. Ashrafi and H. Veisi, RSC Adv., 2014, 4, 40505–40510 RSC.
- N. Xie, R. A. Binstead, E. Block, W. D. Chandler, D. G. Lee, T. J. Meyer and M. Thiruvazhi, J. Org. Chem., 2000, 65, 1008–1015 CrossRef CAS PubMed.
- S. Lai and D. G. Lee, Tetrahedron, 2002, 58, 9879–9887 CrossRef CAS.
- A. Shaabani, P. Mirzaei, S. Naderi and D. G. Lee, Tetrahedron, 2004, 60, 11415–11420 CrossRef CAS.
- M. T. Makhija, R. T. Kasliwal, V. M. Kulkarni and N. Neamati, Bioorg. Med. Chem., 2004, 12, 2317–2333 CrossRef CAS PubMed.
- N. Allendörfer, M. Es-Sayed, M. Nieger and S. Bräse, Synthesis, Stuttg, 2010, pp. 3439–3448 Search PubMed.
- N. K. Konduru, S. Dey, M. Sajid, M. Owais and N. Ahmed, Eur. J. Med. Chem., 2013, 59, 23–30 CrossRef CAS PubMed.
- A. Dishington, S. Fillery and M. R. V. Finlay, Tetrahedron Lett., 2010, 51, 4211–4213 CrossRef CAS.
- J. S. Yadav, P. V. Reddy and L. Chandraiah, Tetrahedron Lett., 2007, 48, 145–148 CrossRef CAS.
- M. Bagherzadeh, J. Chem. Res., 2003, 765–767 CrossRef CAS.
- A. Ghaemi, S. Rayati, S. Zakavi and N. Safari, Appl. Catal., A, 2009, 353, 154–159 CrossRef CAS.
- V. Mirkhani, S. Tangestaninejad, M. Moghadam, I. Mohammadpoor-Baltork and Z. Saedi, J. Iran. Chem. Soc., 2010, 7, 673–677 CrossRef CAS.
- L. Xu, J. Cheng and M. L. Trudell, J. Org. Chem., 2003, 68, 5388–5391 CrossRef CAS PubMed.
- R. Afrasiabi, F. Jalilian, B. Yadollahi and M. R. Farsani, Inorg. Chem. Commun., 2014, 50, 113–116 CrossRef CAS.
- D. Prat, A. Wells, J. Hayler, H. Sneddon, C. R. McElroy, S. Abou-Shehada and P. J. Dunn, Green Chem., 2016, 18, 288–296 RSC.
- M. S. Ballari, N. Herrera Cano, A. G. Lopez, D. A. Wunderlin, G. E. Feresín and A. N. Santiago, J. Agric. Food Chem., 2017, 65, 10325–10331 CrossRef CAS PubMed.
- A. Chanda and V. V. Fokin, Chem. Rev., 2009, 109, 725–748 CrossRef CAS PubMed.
- H. A. Alwathnani and K. Perveen, Afr. J. Biotechnol., 2012, 11, 1100–1105 Search PubMed.
- A. K. Ghosh and B. Zajc, Org. Lett., 2006, 8, 1553–1556 CrossRef CAS PubMed.
Footnote |
† Electronic supplementary information (ESI) available: More details about antifungal susceptibility tests, spectroscopy data for all compounds. See DOI: 10.1039/c9ra04488d |
|
This journal is © The Royal Society of Chemistry 2019 |
Click here to see how this site uses Cookies. View our privacy policy here.