DOI:
10.1039/C7QM00581D
(Research Article)
Mater. Chem. Front., 2018,
2, 585-590
Rhodium-mediated enantioselective synthesis of a benzopicene-based phospha[9]helicene: the structure–property relationship of triphenylene- and benzopicene-based carbo- and phosphahelicenes†
Received
14th December 2017
, Accepted 11th January 2018
First published on 15th January 2018
Abstract
The enantioselective synthesis of a phospha[9]helicene with a bibenzopicene skeleton has been achieved via the rhodium-mediated intermolecular [2+2+2] cycloaddition of a binaphthyl-linked tetrayne with a phosphorus-linked diyne. The photophysical and chiroptical properties of the thus obtained phospha[9]helicene were compared with our previously synthesized benzopicene-based carbo[9]helicene and triphenylene-based carbo- and phospha[7]helicenes. Significantly red shifted absorption and emission maxima were observed in the benzopicene-based phospha[9]helicene as a result of extended π-conjugation, but its fluorescence quantum yield and circularly polarized luminescence activity (glum) were lower than those of the other three helicenes.
Introduction
The asymmetric synthesis of higher-order helicenes with unique extended π-conjugation attracts much attention from organic and physical organic chemists, because there is a good chance that their unique structures cause enhanced photophysical/chiroptical properties.1 For example, our research group achieved the asymmetric synthesis of carbo- and phospha[7]helicenes with a bitriphenylene skeleton, such as (+)-1 and (+)-2 (Fig. 1), via the rhodium-mediated intermolecular [2+2+2] cycloaddition2 of biphenyl-linked tetraynes with carbonyl- or phosphorus-linked diynes.3–7 Pleasingly, the thus obtained triphenylene-based carbo[7]helicene (+)-1 showed significantly higher circularly polarized luminescence (CPL) activity8–10 and a higher fluorescence quantum yield11 compared with unsubstituted carbohelicenes, although the CPL activity of triphenylene-based phospha[7]helicene (+)-2 was not investigated.3d Recently, our research group reported the asymmetric synthesis of more π-extended carbohelicenes, carbo[9]helicenes with a bibenzopicene skeleton, such as (+)-3 (Fig. 1), via the rhodium-mediated intermolecular [2+2+2] cycloaddition of a binaphthyl-linked tetrayne with carbonyl-linked diynes.3h Unfortunately, the CPL spectra of (+)-3 showed two opposite peaks due to the presence of two types of chiral fluorophores, and thus its CPL activity was significantly smaller than that of triphenylene-based carbohelicene (+)-1. In this paper, we synthesized phospha[9]helicene with a bibenzopicene skeleton (−)-4 (Fig. 1) via the rhodium-mediated enantioselective intermolecular [2+2+2] cycloaddition of the binaphthyl-linked tetrayne with the phosphorus-linked diyne. The photophysical and chiroptical properties of the thus obtained benzopicene-based phospha[9]helicene (−)-4 were compared with the previously synthesized benzopicene-based carbo[9]helicene (+)-33h and triphenylene-based carbo- and phospha[7]helicenes (+)-1 and (+)-2.3d
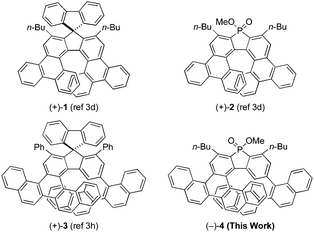 |
| Fig. 1 Structures of triphenylene-based carbo- and phospha[7]helicenes (+)-1 and (+)-2, and benzopicene-based carbo- and phospha[9]helicenes (+)-3 and (−)-4. Their absolute configurations were not determined. | |
Results and discussion
The reaction of binaphthyl-linked tetrayne 5 with phosphorus-linked diyne 6 was examined in the presence of a cationic rhodium(I)/chiral bisphosphine complex at room temperature. After screening chiral ligands and reaction conditions, we found that the desired enantioselective intermolecular [2+2+2] cycloaddition proceeded to give the corresponding benzopicene-based phospha[9]helicene (−)-4 with a high ee value by employing (S)-tol-BINAP as a chiral ligand and slow addition of 6 under diluted conditions, although the product yield was low (Fig. 2).
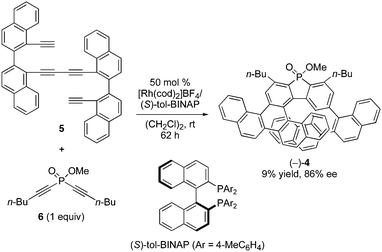 |
| Fig. 2 Rhodium-mediated enantioselective synthesis of phosphahelicene (−)-4 from tetrayne 5 and diyne 6. | |
The photophysical properties of this benzopicene-based phospha[9]helicene 4 were compared with the previously synthesized triphenylene-based carbo- and phospha[7]helicenes 1 and 2,3d and benzopicene-based carbo[9]helicene 33h (Table 1 and Fig. 3). Phospha[9]helicene 4 showed red shifts of absorption and emission maxima compared with [7]helicenes 1 and 2 and carbo[9]helicene 3 as a result of extended π-conjugation. The shape of the emission spectra of 4 did not depend on the concentration despite the possible intermolecular interactions of benzopicene moieties. This feature is the same as our previously reported carbo[9]helicene 3. The fluorescence quantum yield of 4 in CHCl3 solution was lower than those of 1–3, but significantly higher than that of unsubstituted [9]helicene (ϕF = 0.01).12
Table 1 Comparison of the photophysical properties of triphenylene- and benzopicene-based carbo- and phospha-helicenesa
Compound |
UV-absorption λmax/nm |
Fluorescence λmax/nm (excitation wavelength/nm) |
ϕ
F (excitation wavelength/nm) |
Measured in CHCl3 at 25 °C.
Data of ref. 3d.
Data of ref. 3h.
1.0 × 10−5 M.
|
1
|
262, 370, 400 |
449 (350) |
0.30 (350) |
2
|
273, 370, 388 |
487 (350) |
0.22 (350) |
3
|
268, 289, 312 |
488 (350) |
0.18 (400) |
4
|
284, 315d |
502 (340)d |
0.085 (400)d |
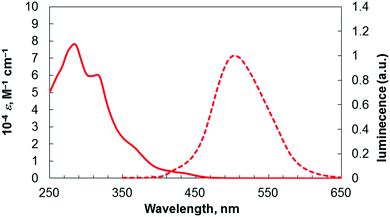 |
| Fig. 3 UV/Vis absorption (solid line) and emission (dashed line) spectra of 4 in CHCl3 (1.0 × 10−5 M). | |
Thus, the introduction of the phosphorus atom and additional benzene rings results in red shifts of absorption and emission maxima, and a decrease in the quantum yield.
The electronic properties of helicenes 1–4 were examined by DFT and time-dependent DFT (TD-DFT) calculations using Gaussian 09 at the wB97XD/6-31G(d) level. Frontier molecular orbitals (MOs) are depicted in Fig. 4 and Fig. S1–S4 (ESI†). In 1–3, the HOMOs are delocalized and spread over the whole molecules [Fig. 4(a)–(c)]. In 4, the HOMO and HOMO−1 energies are very close. The HOMO is localized on the central aromatic rings, but the HOMO−1 is delocalized and spread over the whole molecule [Fig. 4(d)]. Interestingly, interaction between the benzopicene moieties of the HOMO−1 in 4 is larger than that of the HOMO in 3 as seen in their side views, presumably as a result of the extensive overlap of the aromatic rings due to the longer length of the C–P bond than the C–C bond. On the other hand, the LUMOs are mainly localized on the central aromatic rings in all helicenes 1–4 [Fig. 4(a)–(d)].
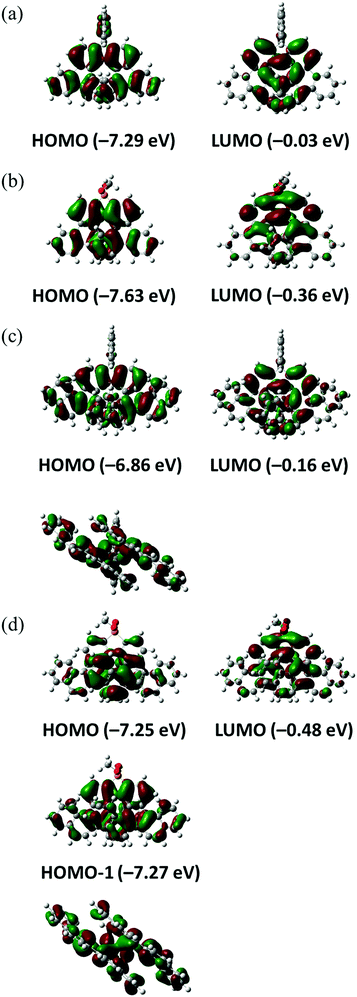 |
| Fig. 4 Pictorial representation of the frontier molecular orbitals of 1 (a), 2 (b), 3 (c) and 4 (d). Side views of the HOMO of (c) and the HOMO−1 of (d) are also shown. | |
The energy diagrams of helicenes 1–4 are shown in Fig. 5. The energy levels of 1–4 revealed that benzopicene-based carbo[9]helicene 3 shows the highest HOMO level (−6.86 eV) and benzopicene-based phospha[9]helicene 4 shows the lowest LUMO level (−0.48 eV). With respect to the HOMO–LUMO energy gap, the general trend is that the HOMO–LUMO energy gaps are significantly decreased by the introduction of additional benzene rings (1 and 2vs.3 and 4), but are slightly increased by the introduction of the phosphorus atom (1 and 3vs.2 and 4).
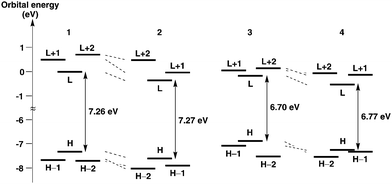 |
| Fig. 5 Energy diagrams for MOs of helicenes 1–4. Two-way arrows represent HOMO–LUMO energy gaps. | |
Finally, we examined the chiroptical properties of triphenylene-based phospha[7]helicene 2 and benzopicene-based phospha[9]helicene 4. The optical rotation value of 4 is larger than that of benzopicene-based carbo[9]helicene 33h but smaller than those of triphenylene-based carbo- and phospha[7]helicenes 13d and 23d (Table 2). The corresponding electronic circular dichroism (ECD) spectra of (+)-2 and (−)-2 and (+)-4 and (−)-4 in CHCl3 are shown in Fig. 6. It was found that these enantiomers show strong Cotton effects, which are mirror images. Furthermore, we attempted to measure the CPL of (+)-2 and (−)-2 and (+)-4 and (−)-4 (Fig. 6). The degree of CPL is given by the luminescence anisotropy factor, which is defined as glum = 2(IL − IR)/(IL + IR), where IL and IR are the luminescence intensities of left and right circularly polarized light, respectively.13 The average CPL value of 2 was glum = +8.1 × 10−4 at 487 nm, which is markedly smaller than that of triphenylene-based carbo[7]helicene (+)-1 (Table 2). The average CPL value of 4 was glum = +4.8 × 10−4 at 502 nm,14 which is the smallest value among these four helicenes (Table 2). As same as the previously reported benzopicene-based carbo[9]helicene 3, the CPL spectra of (−)-4 showed two opposite peaks presumably due to the presence of two types of chiral fluorophores, which lowers the CPL value. Thus, the combination of two triphenylene skeletons and one fluorene skeleton is most effective in increasing the CPL value while retaining its good fluorescence quantum yield.
Table 2 Comparison of the chiroptical properties of carba- and phosphahelicenes with bitriphenylene or bibenzopicene skeletonsa
Compound |
[α]25Db |
g
lum = 2(IL − IR)/(IL + IR)b (excitation wavelength/nm) |
Measured in CHCl3 at 25 °C.
Values are corrected to 100% ee.
Data of ref. 3d.
Data of ref. 3g.
At 1.0 × 10−5 M.
At 1.0 × 10−5 M.
|
1
|
684 |
3.2 × 10−2 (449) |
2
|
1230 |
8.1 × 10−4 (487)e |
3
|
322 |
7 × 10−4 (544) |
4
|
352 |
4.8 × 10−4 (502)f |
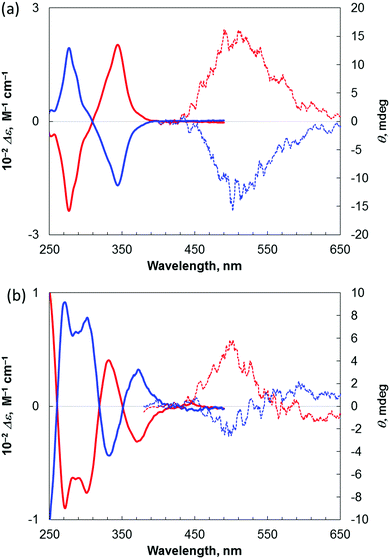 |
| Fig. 6 (a) ECD and CPL spectra of (−)-2 (blue solid line and blue dashed line, respectively) and (+)-2 (red solid line and red dashed line, respectively) in CHCl3 (1.0 × 10−5 M). (b) ECD and CPL spectra of (+)-4 (blue solid line and blue dashed line, respectively) and (−)-4 (red solid line and red dashed line, respectively) in CHCl3 (ECD: 1.0 × 10−5 M, CPL: 5.0 × 10−5 M). Values are corrected to 100% ee. | |
Conclusions
In conclusion, we have achieved the enantioselective synthesis of a phospha[9]helicene with a bibenzopicene skeleton via the rhodium-mediated intermolecular [2+2+2] cycloaddition of a binaphthyl-linked tetrayne with a phosphorus-linked diyne. The photophysical and chiroptical properties of the thus obtained phospha[9]helicene were compared with our previously synthesized benzopicene-based carbo[9]helicene and triphenylene-based carbo- and phospha[7]helicenes. Significantly red shifted absorption and emission maxima were observed in the benzopicene-based phospha[9]helicene as a result of extended π-conjugation, but its fluorescence quantum yield and circularly polarized luminescence activity (glum) were lower than those of the other three helicenes. Thus, the present study clearly indicates that the combination of two triphenylene skeletons and one fluorene skeleton is most effective in increasing the CPL value while retaining its good fluorescence quantum yield.
Experiments
Instrumentation and materials
1H NMR (400 MHz), 13C NMR (100 MHz), and 31P NMR (162 MHz) spectra were recorded on a Bruker AVANCE III HD 400 spectrometer. Chemical shifts were reported on the delta scale in ppm relative to CDCl3 (d = 7.26 ppm) for 1H NMR and CDCl3 (d = 77.16 ppm) for 13C NMR. UV/vis absorption spectra were recorded on a JASCO V-630 spectrometer. Fluorescence spectra were recorded on a JASCO FP-6200 spectrophotometer. Fluorescence quantum yields were obtained on a Hamamatsu Photonics, Absolute PL Quantum Yield Measurement System, C11347-01. Solution samples in CHCl3 for the measurement of fluorescence quantum yields were degassed by nitrogen gas bubbling for 30 minutes. Optical rotation values were measured on a JASCO P-2200. Circular dichroism (CD) spectra were obtained on a JASCO J-820 spectrometer. Circularly polarized luminescence (CPL) spectra were obtained on a JASCO CPL-200 at the Natural Science Center for Basic Research and Development (N-BARD), Hiroshima University. Mass spectra were recorded on a Bruker micrOTOF Focus II using the ESI-TOF method for acetonitrile solutions. Anhydrous CH2Cl2 (No. 27099-7) and (CH2Cl)2 (No. 28450-5) were obtained from Aldrich and used as received. Solvents for the synthesis of substrates were dried over Molecular Sieves 4 Å (Wako) prior to use. (S)-Tol-BINAP was obtained from Takasago International Corporation. Tetrayne 53h and diyne 63d were prepared according to the literature. Bitriphenylene-based phospha[7]helicene (−)-2 for ECD and CPL measurements was prepared via our previously reported procedure using (R)-Segphos instead of (S)-Segphos as a ligand.3d All other reagents were obtained from commercial sources and used as received. All reactions were carried out under an argon or nitrogen atmosphere in oven-dried glassware with magnetic stirring.
General procedures
(−)-4.
(S)-tol-BINAP (8.5 mg, 0.013 mmol) and [Rh(cod)2]BF4 (5.1 mg, 0.013 mmol) were dissolved in CH2Cl2 (2.0 mL) and the mixture was stirred at room temperature for 30 min. H2 was introduced into the resulting solution in a Schlenk tube. After stirring at room temperature for 30 min, the resulting mixture was concentrated. To a solution of the residue in (CH2Cl)2 (1.0 mL) a solution of 6 (6.0 mg, 0.025 mmol) in (CH2Cl)2 (2.0 mL) was added, and the mixture was stirred for 1 min. To the resulting mixture a solution of 5 (15.1 mg, 0.025 mmol) in (CH2Cl)2 (2.0 mL) was added, and the mixture was stirred for 3 min. The mixture was stirred at room temperature for 62 h. The resulting solution was concentrated and purified by preparative thin-layer chromatography (TLC) (eluent: CHCl3/toluene/AcOEt = 20
:
20
:
1) to give (−)-4 (1.9 mg, 0.0022 mmol, 9% yield, 86% ee). Orange solid, m.p. 158 °C (dec.); [α]25D −352° (c 0.00060, CHCl3, 86% ee); 1H NMR (CDCl3, 400 MHz): δ 8.71 (dd, J = 8.4, 4.1 Hz, 2H), 8.61 (d, J = 4.6 Hz, 2H), 8.26 (d, J = 9.0 Hz, 2H), 8.04–7.94 (m, 6H), 7.69–7.57 (m, 6H), 7.36 (d, J = 8.3 Hz, 1H), 7.30 (d, J = 8.0 Hz, 1H), 7.15 (d, J = 7.9 Hz, 2H), 6.40–6.28 (m, 4H), 4.05 (d, J = 12.3 Hz, 3H), 3.49–3.32 (m, 4H), 2.27–2.02 (m, 4H), 1.81–1.67 (m, 4H), 1.18–1.12 (m, 6H); 13C NMR (CDCl3, 100 MHz): δ 145.1, 144.87, 144.85, 144.6, 142.1, 142.0, 141.9, 133.92, 133.90, 133.8, 133.7, 133.55, 133.48, 131.31, 131.25, 129.8, 129.7, 129.6, 128.64, 128.62, 128.5, 128.33, 128.28, 128.2, 128.12, 128.07, 128.0, 127.9, 127.65, 127.61, 127.59, 127.54, 127.49, 127.2, 126.04, 126.02, 125.88, 125.86, 125.7, 125.64, 125.58, 125.4, 125.1, 124.73, 124.67, 124.4, 124.3, 124.0, 123.8, 123.3, 123.2, 123.0, 122.8, 122.7, 121.6, 121.4, 120.9, 52.82, 52.76, 34.43, 34.39, 34.2, 34.0, 33.9, 33.8, 22.90, 22.87, 14.2 (31P–13C couplings were not determined); 31P NMR (CDCl3, 162 MHz): 46.5; HRMS (ESI) calcd for C61H47NaO2P [M + Na]+ 865.3206, found 865.3193; CHIRALPAK ID-3, n-hexane/2-propanol = 80
:
20, 1.0 mL min−1, retention times: 15.5 min (major isomer) and 26.4 min (minor isomer).
(+)-4.
This compound was prepared via the same procedure for the synthesis of (−)-4 using (R)-tol-BINAP instead of (S)-tol-BINAP as a ligand.
Conflicts of interest
There are no conflicts to declare.
Acknowledgements
This work was supported partly by a Grant-in-Aid for Scientific Research on Innovative Areas “π-System Figuration: Control of Electron and Structural Dynamism for Innovative Functions” (JP26102004) from the Japan Society for the Promotion of Science (JSPS, Japan) and ACT-C (No. JPMJCR1122YR) from the Japan Science and Technology Agency (JST, Japan). We thank the Natural Science Center for Basic Research and Development (N-BARD), Hiroshima University, for the measurement of CPL using a JASCO CPL-200, and Prof. Toshikazu Takata and Dr Kazuko Nakazono (Tokyo Institute of Technology) for the measurement of ECD. We also thank Takasago International Corporation for the gifts of tol-BINAP and Segphos, Umicore for generous support in supplying the rhodium and palladium complexes, and Central Glass Co., Ltd, for the gift of trifluoromethanesulfonic anhydride.
Notes and references
- For reviews on the helicene synthesis, see:
(a) K. Tanaka, Y. Kimura and K. Murayama, Bull. Chem. Soc. Jpn., 2015, 88, 375 CrossRef CAS;
(b)
K. Tanaka, in Transition-Met.-Mediated Aromat. Ring Constr., ed. K. Tanaka, Wiley, Hoboken, 2013, ch. 10 Search PubMed;
(c) Z. Peng and N. Takenaka, Chem. Rec., 2013, 13, 28 CrossRef CAS PubMed;
(d) Y. Shen and C.-F. Chen, Chem. Rev., 2012, 112, 1463 CrossRef CAS PubMed;
(e) R. Amemiya and M. Yamaguchi, Chem. Rec., 2008, 8, 116 CrossRef CAS PubMed;
(f)
A. Rajca and M. Miyasaka, in Funct. Org. Mater., ed. T. T. J. Müller and U. H. F. Bunz, Wiley-VCH, Weinheim, 2007, p. 543 Search PubMed;
(g) C. Nuckolls, R. Shao, W.-G. Jang, N. A. Clark, D. M. Walba and T. J. Katz, Chem. Mater., 2002, 14, 773 CrossRef CAS;
(h) T. J. Katz, Angew. Chem., Int. Ed., 2000, 39, 1921 CrossRef CAS.
- For recent reviews on the rhodium(I)-catalyzed [2+2+2] cycloaddition, see:
(a)
K. Tanaka and Y. Shibata, in Transition-Met.-Mediated Aromat. Ring Constr., ed. K. Tanaka, Wiley, Hoboken, 2013, ch. 4 Search PubMed;
(b) Y. Shibata and K. Tanaka, Synthesis, 2012, 323 CAS;
(c) K. Tanaka, Heterocycles, 2012, 85, 1017 CrossRef CAS;
(d) K. Tanaka, Synlett, 2007, 1977 CrossRef CAS See also ref. 1a.
- For examples of the enantioselective helicene synthesis via the rhodium(I)-mediated [2+2+2] cycloaddition, see:
(a) K. Tanaka, A. Kamisawa, T. Suda, K. Noguchi and M. Hirano, J. Am. Chem. Soc., 2007, 129, 12078 CrossRef CAS PubMed;
(b) K. Tanaka, N. Fukawa, T. Suda and K. Noguchi, Angew. Chem., Int. Ed., 2009, 48, 5470 CrossRef CAS PubMed;
(c) N. Fukawa, T. Osaka, K. Noguchi and K. Tanaka, Org. Lett., 2010, 12, 1324 CrossRef CAS PubMed;
(d) Y. Sawada, S. Furumi, A. Takai, M. Takeuchi, K. Noguchi and K. Tanaka, J. Am. Chem. Soc., 2012, 134, 4080 CrossRef CAS PubMed;
(e) Y. Kimura, N. Fukawa, Y. Miyauchi, K. Noguchi and K. Tanaka, Angew. Chem., Int. Ed., 2014, 53, 8480 CrossRef CAS PubMed;
(f) K. Murayama, Y. Oike, S. Furumi, M. Takeuchi, K. Noguchi and K. Tanaka, Eur. J. Org. Chem., 2015, 1409 CrossRef CAS;
(g) R. Yamano, J. Hara, K. Murayama, H. Sugiyama, H. Uekusa, S. Kawauchi, Y. Shibata and K. Tanaka, Org. Lett., 2017, 19, 42 CrossRef CAS PubMed;
(h) K. Murayama, Y. Shibata, H. Sugiyama, H. Uekusa and K. Tanaka, J. Org. Chem., 2017, 82, 1136 CrossRef CAS PubMed.
- For examples of the enantioselective helicene synthesis via the nickel(0)-mediated [2+2+2] cycloaddition, see:
(a) I. G. Stará, I. Starý, A. Kollárovič, F. Teplý, Š. Vyskočil and D. Šaman, Tetrahedron Lett., 1999, 40, 1993 CrossRef;
(b) F. Teplý, I. G. Stará, I. Starý, A. Kollárovič, D. Šaman, Š. Vyskočil and P. Fiedler, J. Org. Chem., 2003, 68, 5193 CrossRef PubMed;
(c) A. Jančařik, J. Rybáček, K. Cocq, J. V. Chocholoušová, J. Vacek, R. Pohl, L. Bednárová, P. Fiedler, I. Cisařová, I. G. Stará and I. Starý, Angew. Chem., Int. Ed., 2013, 52, 9970 CrossRef PubMed;
(d) M. Buchta, J. Rybácek, A. Jančařík, A. A. Kudale, M. Buděšínský, J. V. Chocholoušová, J. Vacek, L. Bednárová, I. Císařová, G. J. Bodwell, I. Starý and I. G. Stará, Chem. – Eur. J., 2015, 21, 8910 CrossRef CAS PubMed.
- For examples of the enantioselective helicene synthesis via the iridium(I)-mediated [2+2+2] cycloaddition, see: T. Shibata, T. Uchiyama, Y. Yoshinami, S. Takayasu, K. Tsuchikama and K. Endo, Chem. Commun., 2012, 48, 1311 RSC.
- For examples of the enantioselective helicene synthesis via the cobalt(I)-mediated [2+2+2] cycloaddition, see:
(a) B. Heller, M. Hapke, C. Fischer, A. Andronova, I. Starý and I. G. Stará, J. Organomet. Chem., 2013, 723, 98 CrossRef CAS;
(b) J. Klívar, A. Jančařik, D. Šaman, R. Pohl, P. Fiedler, L. Bednárová, I. Starý and I. G. Stará, Chem. – Eur. J., 2016, 22, 14401 CrossRef PubMed;
(c) I. G. Sánchez, M. Šámal, J. Nejedlý, M. Karras, J. Klívar, J. Rybáček, M. Buděšínský, L. Bednárová, B. Seidlerová, I. G. Stará and I. Starý, Chem. Commun., 2017, 53, 4370 RSC.
- For other recent examples of the enantioselective helicene synthesis, see:
(a) E. González-Fernández, L. D. M. Nicolls, L. D. Schaaf, C. Farés, C. W. Lehmann and M. Alcarazo, J. Am. Chem. Soc., 2017, 139, 1428 CrossRef PubMed;
(b) M. Sako, Y. Takeuchi, T. Tsujihara, J. Kodera, T. Kawano, S. Takizawa and H. Sasai, J. Am. Chem. Soc., 2016, 138, 11481 CrossRef CAS PubMed;
(c) M. Tanaka, Y. Shibata, K. Nakamura, K. Teraoka, H. Uekusa, K. Nakazono, T. Takata and K. Tanaka, Chem. – Eur. J., 2016, 22, 14190 CrossRef PubMed;
(d) J. Doulcet and G. R. Stephenson, Chem. – Eur. J., 2015, 21, 18677 CrossRef CAS PubMed;
(e) J. Doulcet and G. R. Stephenson, Chem. – Eur. J., 2015, 21, 13431 CrossRef CAS PubMed;
(f) K. Nakamura, S. Furumi, M. Takeuchi, T. Shibuya and K. Tanaka, J. Am. Chem. Soc., 2014, 136, 5555 CrossRef CAS PubMed;
(g) L. Kötzner, M. J. Webber, A. Martínez, C. DeFusco and B. List, Angew. Chem., Int. Ed., 2014, 53, 5202 Search PubMed;
(h) A. Grandbois and S. K. Collins, Chem. – Eur. J., 2008, 14, 9323 CrossRef CAS PubMed;
(i) J. Caeiro, D. Peña, A. Cobas, D. Pérez and E. Guitián, Adv. Synth. Catal., 2006, 348, 2466 CrossRef CAS;
(j) M. Miyasaka, A. Rajca, M. Pink and S. Rajca, J. Am. Chem. Soc., 2005, 127, 13806 CrossRef CAS PubMed;
(k) M. C. Carreño, M. González-López and A. Urbano, Chem. Commun., 2005, 611 RSC;
(l) A. Rajca, M. Miyasaka, M. Pink, H. Wang and S. Rajca, J. Am. Chem. Soc., 2004, 126, 15211 CrossRef CAS PubMed;
(m) M. C. Carreño, S. García-Cerrada and A. Urbano, Chem. – Eur. J., 2003, 9, 4118 CrossRef PubMed.
- For selected recent examples of the CPL of small organic molecules, see:
(a) S. Sato, A. Yoshii, S. Takahashi, S. Furumi, M. Takeuchi and H. Isobe, Proc. Natl. Acad. Sci. U. S. A., 2017, 114, 13097 CrossRef CAS PubMed;
(b) T. Otani, A. Tsuyuki, T. Iwachi, S. Someya, K. Tateno, H. Kawai, T. Saito, K. S. Kanyiva and T. Shibata, Angew. Chem., Int. Ed., 2017, 56, 3906 CrossRef CAS PubMed;
(c) M. Gon, Y. Morisaki, R. Sawada and Y. Chujo, Chem. – Eur. J., 2016, 22, 2291 CrossRef CAS PubMed;
(d) R. B. Alnoman, S. Rihn, D. C. O’Connor, F. A. Black, B. Costello, P. G. Waddell, W. Clegg, R. D. Peacock, W. Herrebout, J. G. Knight and M. J. Hall, Chem. – Eur. J., 2016, 22, 93 CrossRef CAS PubMed;
(e) H. Sakai, T. Kubota, J. Yuasa, Y. Araki, T. Sakanoue, T. Takenobu, T. Wada, T. Kawai and T. Hasobe, Org. Biomol. Chem., 2016, 14, 6738 RSC;
(f) H. Sakai, T. Kubota, J. Yuasa, Y. Araki, T. Sakanoue, T. Takenobu, T. Wada, T. Kawai and T. Hasobe, J. Phys. Chem. C, 2016, 120, 7860 CrossRef CAS;
(g) K. Goto, R. Yamaguchi, S. Hiroto, H. Ueno, T. Kawai and H. Shinokubo, Angew. Chem., Int. Ed., 2012, 51, 10333 CrossRef CAS PubMed;
(h) H. Maeda, Y. Bando, K. Shimomura, I. Yamada, M. Naito, K. Nobusawa, H. Tsumatori and T. Kawai, J. Am. Chem. Soc., 2011, 133, 9266 CrossRef CAS PubMed;
(i) T. Kaseyama, S. Furumi, X. Zhang, K. Tanaka and M. Takeuchi, Angew. Chem., Int. Ed., 2011, 50, 3684 CrossRef CAS PubMed;
(j) H. Tsumatori, T. Nakashima and T. Kawai, Org. Lett., 2010, 12, 2362 CrossRef CAS PubMed . See also ref. 3d, f–h, and 7e.
- For a selected recent review on CPL, see: E. M. Sánchez-Carnerero, A. R. Agarrabeitia, F. Moreno, B. L. Marato, G. Muller, M. J. Ortiz and S. de la Moya, Chem. – Eur. J., 2015, 21, 13488 CrossRef PubMed.
- For recent typical examples of the envisaged application of the class of helical molecules in chiroptical sensing and nanoscale assemblies, see:
(a) M. Agnes, A. Nitti, D. A. V. Griend, D. Dondi, D. Merli and D. Pasini, Chem. Commun., 2016, 52, 11492 RSC;
(b) M. Caricato, A. K. Sharma, C. Coluccini and D. Pasini, Nanoscale, 2014, 6, 7165 RSC.
- For selected recent examples of highly fluorescent helicenes, see:
(a) H. Sakai, T. Kubota, J. Yuasa, Y. Araki, T. Sakanoue, T. Takenobu, T. Wada, T. Kawai and T. Hasobe, J. Phys. Chem. C, 2016, 120, 7860 CrossRef CAS;
(b) Y. Yamamoto, H. Sakai, J. Yuasa, Y. Araki, T. Wada, T. Sakanoue, T. Takenobu, T. Kawai and T. Hasobe, J. Phys. Chem. C, 2016, 120, 7421 CrossRef CAS;
(c) Y. Yamamoto, H. Sakai, J. Yuasa, Y. Araki, T. Wada, T. Sakanoue, T. Takenobu, T. Kawai and T. Hasobe, Chem. – Eur. J., 2016, 22, 4263 CrossRef CAS PubMed;
(d) H. Sakai, S. Shinto, J. Kumar, Y. Araki, T. Sakanoue, T. Takenobu, T. Wada, T. Kawai and T. Hasobe, J. Phys. Chem. C, 2015, 119, 13937 CrossRef CAS . See also ref. 3d, 7e and 8b.
- R. H. Martin and M. J. Marchant, Tetrahedron, 1974, 30, 343 CrossRef CAS.
-
(a)
J. P. Riehl and F. Muller, Compr. Chiropt. Spectrosc., Wiley, New York, 2012 Search PubMed;
(b)
Circ. Dichroism, ed N. Berova, K. Nakanishi and R. W. Woody, Wiley-VCH, New York, 2nd edn, 2000 Search PubMed;
(c) M. Grell and D. D. C. Bradley, Adv. Mater., 1999, 11, 895 CrossRef CAS;
(d) J. P. Riehl and F. S. Richardson, Chem. Rev., 1986, 86, 1 CrossRef CAS.
- The different intensities of the CPL spectra of (−)-4 and (+)-4 may arise from the large error in measurement due to the very small values of the CPL. This phenomenon was observed in the CPL spectra of 2-methylhexahelicene. See: J. Phys. Chem. C, 2014, 118, 1682.
Footnote |
† Electronic supplementary information (ESI) available. See DOI: 10.1039/c7qm00581d |
|
This journal is © the Partner Organisations 2018 |
Click here to see how this site uses Cookies. View our privacy policy here.