DOI:
10.1039/C7RA10461H
(Paper)
RSC Adv., 2017,
7, 51581-51592
Changing the shape of hair with keratin peptides†
Received
20th September 2017
, Accepted 26th October 2017
First published on 6th November 2017
Abstract
Chemical straightening of curly human hair fibres involves the use of strong reducing agents at alkaline pH. Human hair is made of keratin, and the fixation of fibre shape involves the reduction and reformation of new disulphide bonds between keratin molecules. Here, we propose an alternative and green methodology using keratin peptide sequences (10–13 residues) derived from the human genome. In a previous study, we analysed 1235 cysteine-containing peptides encoded by all human genes of hair keratin and keratin-associated proteins. These peptide fragments have been designed by nature to interact with keratin. Here we tested eight peptides, which were select based on their affinity for human hair keratin solution as shown by Matrix-Assisted Laser-Desorption Ionization Time-of-Flight Mass Spectrometry (MALDI-TOF/TOF) and by molecular dynamics simulation. The peptides were characterized in detail regarding their ability to act as hair straightening modulators and to improve the tensile strength and elasticity of hair. Of the eight tested peptides, PepE, PepG and KP showed the highest ability to interact with a keratin peptide model, and to improve hair mechanical properties and straightening efficiency. The proposed solutions presented here will replace harsh reducing agents at alkaline pH by peptide formulations acting at neutral pH to change hair shape through the re-conformation of disulphide bonds. Here, we provide experimental evidence which explains at a molecular level how keratin decapeptides can interact with large keratin molecules in human hair, opening an innovative green approach to changing the shape of hair fibre.
Introduction
The haircare industry has developed a plethora of products to modify hair characteristics, with hair straightening being one of the most popular processes. Many of these products contain toxic or environmentally hazardous chemicals such as strong alkaline agents, thioglycolates, sodium or lithium hydroxide, guanidine or even formaldehyde. These chemicals have high toxicity and the potential to generate poisonous gases, and they have a negative impact on human health.1–7 Table 1 presents the common methods for hair straightening. These treatments damage the hair fibre, reduce the cross-linking density and decrease the hair's physicomechanical properties.8,9 Extremely damaged hair suffers several negative impacts such as the appearance of cracks and lifting of the cuticle, the removal of the hydrophobic top-layer of the hair cuticle and a consequent reduction of surface hydrophobicity.10–12 A further consequence is the alteration of the diffusion rate into keratin fibres, which is dependent on the cross-linking density of the hair. Excessive or repeated hair chemical treatments produce irreversible changes in hair texture, and can result in a fracture of the hair fibre once the cuticle is removed, and cortex exposure leads to breakage.4,8,10,12–18 The use of chemicals also represents an important concern for the health of the consumer and stylist, as well as for the environment.
Table 1 Common methods and agents used in hair straightening
Reducing methods |
Agents |
Methodologies |
References |
Method with reductive agents (thio-relaxers) |
Ammonium thioglycolate, thioglycolic acid, cysteamine hydrochloride, glycerylmonothioglycolate, ammonium sulphite, ammonium bisulphite |
Disruption of disulphide bonds and possible rearrangement through disulphide interchange processes |
9 and 69–72 |
Method with alkaline agents (hydroxides) |
Lye relaxer |
Potassium hydroxide, sodium hydroxide |
Disruption of disulphide bonds and possible rearrangement to disulphide bonds or lanthionine bonds (lye relaxer with pH ≥ 12) |
9, 69 and 72 |
No-lye mix relaxer |
Calcium hydroxide and an activator of the relaxation reaction containing guanidine carbonate |
No-lye no-mix relaxer |
Lithium hydroxide |
Brazilian keratin method |
Formaldehyde |
Cross-links hair keratin with the keratin introduced in the formulation |
9, 72 and 73 |
Biologically, the human hair is a complex fibre with various morphological components. Hair essentially consists of keratin, a fibrous structural protein, which is a member of the superfamily of intermediate filament proteins, containing 18% cysteine as calculated from the overall amino acid composition.19,20 The hair fibre is divided into three main regions: cuticle, cortex, and medulla.21,22 The medulla is located in the core of the hair fibre, and may be partially or entirely absent in fine hair fibres. The cortex is the major bulk of the hair fibre and is constituted of macrofibrils of intermediate filaments, which are in turn composed of α-keratins, found in α-helical form, acting as mechanical support.23 The individual filaments of cortical cells are separated by a cell membrane complex of keratin associated proteins (KAPs), with a high cysteine content and without a well-defined spatial organization.24 Keratin α-helices are coiled by ionic forces, hydrogen bonds, van der Waals forces and disulphide bonds. The cuticle, enclosing the hair cortex, is composed of superimposed layers of cells in a scaled structure. Each cuticle cell contains various sub-lamellar layers (epicuticle, A-layer, exocuticle, and endocuticle) with proteins cross-linked mainly by cysteines. The outer epicuticle layer contributes to the lubricity of the hair and acts as a barrier to the penetration of compounds into the hair.9,21 The free lipids on the surface and the involvement of proteins are critical factors in the overall structure of the hair fibre.
Hair proteins are able to create stronger or weaker interactions.25,26 For example, the presence of disulphide bonds in cysteine residues of the hair keratin protein determine the maintenance of the shape of the hair, and can be altered by perming or relaxing. The disulphide bonds are much stronger when thiol groups are closer together, making these bonds easier to form, and in consequence the hair is much curlier once a curve in the linear protein chain is induced. Hair fibre is sensitive to changes in the pH. Alkaline reducing agents, when in contact with the cortex, break and rearrange the disulphide bonds, stretching the spiral coil of keratin molecules.10 This makes the hair more susceptible to friction, leading to lower hair strength and resistance. However, hair damage caused by the use of chemicals can be minimized, avoided or repaired by the controlled use of proteins or peptides.27 The use of peptides for hair treatments including hair gloss, hair softness and manageability and hair damage repair is well described. Peptides and amino acids have high ability to diffuse into the hair fibre cortex, high substantivity in a practical pH range, and the ability to aid recovery from hair cuticle damage.10,13,16,27–32 Numerous bonds such as ionic, hydrophobic and hydrogen bonds, can be established between the exogenous proteins and the hair keratin. Covalent binding, such as that in disulphide bonds, may also occur.22,27,32,33 Hair products based on synthetic peptides are a reality today, with applied dosages of 0.01%.
Disulphide bond disruption and formation are known to be associated with changes of hair shape.9 Here, we explore the use of peptides as substitutes for alkaline agents and thio-relaxers which are commonly used for hair straightening. Our goal is to present a novel green approach to validate the use of decapeptide sequences as eco-friendly alternatives for the control of hair shape changes. These peptides were selected from a previous broad study which analyzed over 1000 different peptides containing two to five cysteines based on genes for human hair keratin and KAPs.34 It is known that cysteine can be applied as a reducing agent for the substitution of environmentally harmful chemicals.35 The origin of the peptides, their high cysteine content, and their hydrophobicity are intended to improve hair properties, enabling the restructuring and reinforcement of hair fibres. A detailed characterization of the peptides was conducted regarding their ability to act as hair straightening agents and their ability to recover and/or increase hair tensile strength and elasticity. Herein, we explore the application of peptides as innovative ecological and healthy alternatives for hair straightening, without compromising hair integrity, so opening an innovative way to change the shape of the hair fibre.
Experimental
Materials
Human virgin hair samples were provided by International Hair Importers & Products Inc. (New York, USA). All chemicals were purchased from Sigma-Aldrich (Madrid, Spain), except when stated otherwise. A total of eight peptides were designed to interact with hair keratin. These peptides are based on fragments of human hair keratin and KAPs and were selected on the basis of a previous study which used a microarray of peptides.34 Specifically, the peptide sequences are based on keratin associated protein 5–3 (PepA), keratin associated protein 4–8 (PepB), keratin associated protein 9–7 (PepC), keratin type I cuticular Ha4 (PepD), keratin type I cuticular Ha5 (PepE), keratin associated protein 16–1 (PepF), keratin associated protein 5–9 (PepG) and keratin type II cuticular Hb5 (KP). The KP peptide has already been analysed in previous studies.16,28 The peptides were synthesised by JPT Peptide Technologies GmbH (Berlin, Germany). PepA, PepB, PepC, PepD, PepE, PepF and PepG were covalently linked via their N-terminal amines to the fluorescent dye 5(6)-carboxyfluorescein (λex = 492 nm; λem = 517 nm), while KP was covalently linked via its N-terminal amines to the fluorescent dye 5(6)-carboxytetramethyl-rhodaminesuccinimidyl ester, 5(6)-TAMRA (λex = 544 nm and λem = 572 nm), to facilitate the analysis of peptide penetration. The peptides were supplied as lyophilized materials. They were analysed by high performance liquid chromatography (HPLC) and mass spectrometry (MS), and their purities were over 70% (HPLC, 220 nm, C18, linear gradient). The peptides' characteristics are shown in Fig. 2A.
The hair protein-like peptide, KeraPep, (peptide sequence from N-terminal to C-terminal: DDDDDKPCCCSSGCGSSCCQSSCCKPCCSQSSCCVPVCCQCKIDDDDD), has a sequence based on the gene for human hair keratin-associated protein 5–1, and was synthesised by GenScript Inc. (Piscataway, USA). The selected sequence contains a high content of cysteine to model the hair cysteine content and it can be considered to be a good model for the study of the redox behaviour of disulphide bonding of hair proteins and smaller peptides. The penta-aspartate sequences at each extremity were added to induce peptide solubility.
Methods
Matrix-assisted laser-desorption ionization time-of-flight mass spectrometry (MALDI-TOF/TOF) analysis of peptides. Each peptide was processed for MALDI-TOF/TOF analysis. The peptides were desalted, concentrated and spotted onto the MALDI plate using reversed-phase C18 Zip Tips (Millipore, MA, USA), according to the manufacturer's instructions. The matrix used was a solution of 7–8 mg mL−1 α-cyano-4-hydroxycinnamic acid, prepared in 50% (v/v) acetonitrile and 0.1% (v/v) trifluoroacetic acid. Samples were analysed using a 4800 Proteomics Analyzer MALDI-TOF/TOF (AB SCIEX, Framingham, MA, USA). The peptide mass fingerprint data was collected in positive MS reflector mode for the mass-to-charge ratio (m/z) 700–4000 using trypsin autolysis peaks for internal calibration.
Interaction analysis of peptides with a hair protein peptide model by MALDI-TOF/TOF. The peptides were analysed by MALDI-TOF/TOF regarding their binding ability to a peptide hair protein model, a 45-mer peptide based on human hair KAP (abbreviated as KeraPep), to make the identification of peptide binding easier, and to allow for a closer comparison to the simulations. Each peptide was incubated with the KeraPep (1 mg mL−1 in phosphate buffer pH 7), in a ratio of 2
:
1 (w/w) for 1 hour. This incubation was performed at two temperatures: 37 °C and 120 °C. For analysis, a solution of 8–10 mg mL−1 sinapic acid, prepared in 50% (v/v) acetonitrile and 0.1% (v/v) trifluoroacetic acid was used as the matrix. The samples were spotted onto the MALDI plate and analysed using a 4800 Proteomics Analyzer MALDI-TOF/TOF (AB SCIEX, Framingham, MA, USA). The peptide mass fingerprint data was collected in linear mode for the mass-to-charge ratio (m/z) 4000–30
000 using trypsin autolysis peaks for internal calibration. The ratio of the different peaks was determined using the equation:
Qualitative and quantitative analysis of peptide penetration into the hair fibre. The penetration of the peptides into African hair was assessed qualitatively by fluorescence microscopy. The hair fibres treated with the peptides were embedded in an epoxy resin. Transversal cuts of 15 μm width were prepared using a microtome (Microtome Leitz) and the hair cross sections were analysed by fluorescence microscopy (Olympus BX51) using ×40 amplification. The images were collected with the same conditions of brightness, time of exposure and gain, and were examined at the spectral setting of 4′,6-diamidino-2-phenylindole (DAPI – λex = 358 nm; λem = 461 nm) for the peptides PepA, PepB, PepC, PepD, PepE, PepF and PepG, and at the spectral setting of tetramethylrhodamine (TRITC – λex = 557 nm; λem = 576 nm) for the peptide KP. As auto-fluorescence was unavoidable for these samples, the auto-fluorescence of the control sample was measured and subtracted from the fluorescence of the other samples. For homogenization purposes, the KP, labelled with TAMRA and observed with TRITC spectral settings, was depicted as blue. The most representative images were chosen.The quantification of peptide uptake by hair fibres was assessed from the variation in concentration of each peptide after the peptide hair treatments (procedure adapted from Fernandes and Cavaco-Paulo16). Briefly, the hair-peptide solution was monitored by the measurements of the absorbance at 497 nm for peptides PepA, PepB, PepC, PepD, PepE, PepF, and PepG, and at 555 nm for KP, before and after the hair treatment. Calibration curves for each peptide were obtained. Three independent experiments were performed for each peptide condition. The concentration variation (%) or peptide uptake was calculated using the equation:
Molecular dynamics simulations of the interactions between the peptides and the hair protein peptide model. All molecular dynamics simulations were performed with the GROMACS 4.0.7 package using the GROMOS 54A7 united-atom force-field,36–40 with periodic boundary conditions, applying the LINCS41 and the SETTLE42 algorithms to constrain the peptide and water bonds respectively. The simulations ran with a time step of integration of 2 fs, and the single point charge (SPC) model43 was used to represent the water. The temperature was maintained at 300 K with the V-rescale thermostat44,45 and the Parrinello–Rahman barostat46,47 (with isotropic coupling) was used to maintain the pressure at 1 atm. van der Waals and electrostatic interactions were treated by a twin-range method, with short and long range cutoffs (0.8 and 1.4 nm, respectively), and with a reaction field correction for electrostatic interactions using a dielectric constant of 54,48 and the neighbour list was updated every 10 steps. The molecular model of the KeraPep was built through PYMOL software using its amino acid sequence and assigning the KeraPep secondary structure as α-helix (the majority of this specific sequence of keratin-associated protein 5–1 was predicted to be α-helical49,50). The same procedure was used to build the remaining PepC, PepD, PepG and KP computational models, but in these models no secondary structure was defined, due to their small size. The software VMD (Visual Molecular Dynamics)51 was used to create all simulation pictures presented.All simulated systems were initially energy-minimised for around 2000 steps with the steepest descent method. The systems initiated a series of three short equilibration simulations (500 ps in each simulation), with position restraints allowing the slow relaxation of the structures for the final production run (the atoms were restrained with a harmonic force of 103 kJ mol−1 nm−2). The first simulation was performed in the NVT ensemble (number of particles, volume and temperature constant), applying position restraints to all heavy atoms. The second simulation was performed in the NPT ensemble (number of particles, pressure and temperature constant), with position restraints for the same atoms. Lastly, the third simulation was also run in the NPT ensemble, but with position restraints only for the α-carbon atoms of all the amino acids. After the equilibration of the systems by the preparatory simulations, 100 ns of NPT simulations in aqueous systems were performed.
Evaluation of mechanical properties of over-bleached straight hair treated with peptides. The recovery of the mechanical properties of severely chemically damaged straight hair was performed using straight Caucasian hair (curliness type II52). Before the bleaching treatment, hair tresses were washed with a solution of 0.5% sodium dodecyl sulphate (SDS) with constant agitation for 1 hour and dried naturally. Chemically damaged hair underwent eight cycles of bleaching. The bleaching process consisted of the application of 12% (v/v) H2O2 in the presence of 0.1 mol L−1 Na2CO3/NaHCO3 buffer, pH 9.0 at 50 °C for 1 hour, in a bath ratio of 1
:
10. The hair tresses were washed with distilled water after each cycle of bleaching. After that, hair tresses were treated with 0.01% (w/v) of each peptide (PepA, PepB, PepC, PepD, PepE, PepF, PepG, KP) in a formulation of phosphate buffer pH 7, for 1 hour at 37 °C with agitation. After hair treatment, the roughly bound peptides were washed off in distilled water.The mechanical properties of the chemically damaged hair samples were evaluated following guidelines outlined in ASTM D1445-95 for fibre tensile testing. The measurements were performed in an Instron 4505 tensile tester, with a 2.5 N load cell. For each type of hair treatment, 15 single hair fibres with low variability in diameter were randomly taken from the hair tress and individually mounted in the tensile jig, using a paper device with a fixed gauge length of 20 mm. All the hair samples were kept under the same conditions before the measurements. The measurements were performed with a constant rate of 1.5 mm min−1 until breakage. The measurements were performed assuming an average mean fibre diameter of 70 μm (the value that was obtained through previous measurements with light microscopy). The data recorded in the equipment (applied load against extension) was converted to stress (load/unit area) against strain (% extension).
Statistical significances were determined using SPSS, using the one-way ANOVA test, followed by the Tukey's post-hoc test. p-Values ≤ 0.05 were considered statistically significant (expressed in the figures with an asterisk, *) and p-values ≤ 0.01 were considered very significant (**).
Straightening evaluation of single curly hair treated with peptides. The hair straightening was performed using curly African hair (curliness type VII). Hair tresses were washed with a solution of 0.5% SDS, with constant agitation for 1 hour, and dried naturally. The procedure to straighten the curly hair was adapted from the Kirby method .53,54 Single hair fibres of curly hair were forcefully straightened by gluing the root ends on the frame to ensure a straight shape. Hairs were kept evenly straight without any twisting. While straightened, the hair was incubated at 37 °C for 1 hour in a formulation of phosphate buffer pH 7 with 0.01% (w/v) of each peptide (PepA, PepB, PepC, PepD, PepE, PepF, PepG or KP). After treatment, hair was washed with distilled water and allowed to dry naturally, still straightened. In order to evaluate the effect of the peptides, three sequential treatments with the same concentration (0.01% (w/v)) and a separate treatment with a higher peptide concentration (0.1% (w/v)) were performed. Three independent experiments were performed for each peptide treatment. Hair straightened by a chemical relaxing treatment was used as a positive control. Briefly, in the chemical treatment, hair tresses were washed with 0.75 M sodium hydroxide pH 12–14 for 30 minutes, and then combed and washed with water. Then, the hair was washed with 0.1 M acetic acid, as a neutralising agent, and finally the hair was washed with a commercial shampoo. Straightening efficiency was calculated based on the change of the hair twists and length after the treatments, as depicted in Fig. 1, using the equation:
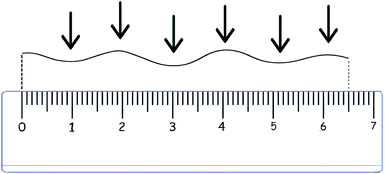 |
| Fig. 1 Scheme of hair measurement and twist counting. | |
Straightening evaluation of curly hair tresses treated with peptides in serum formulation. The efficiency of the peptides at straightening curly hair was evaluated in an alcoholic-based formulation. The effect of the formulation was computationally reproduced in a previous study regarding strong interactions between the hair and alcoholic components.55 The peptides (PepE, PepG and KP) were incorporated into alcoholic formulations at pH 7.5 (1.5% propylene glycol, 10% ethanol, and 0.5% benzyl alcohol). Each serum formulation was applied onto the hair tresses and left in for 15 minutes. The hair straightening process was induced using a flat iron at 200 °C. The method was repeated until each hair tress was dried and straight. Then, the hair tresses were washed with distilled water and dried at 50 °C. The straightening efficiency was calculated using the above-mentioned equation. The hair tresses were then washed and shampooed 20 times.
Results and discussion
Characteristics of chosen peptides
The modification of hair shape was the driving force for the selection of appropriately engineered peptides based on the sequences of keratin and KAPs. These peptides were selected based on a previous study of 1235 cysteine-containing peptides of all genes of human hair keratin and KAPs.34 This study analysed the interaction of the peptides with keratin extracted from human hair, and the peptides with the best characteristics were selected for further analysis. Due to their high cysteine content and hydrophobic properties, these peptides are expected to present higher affinities towards the hair proteins. Furthermore, decapeptides are small enough to be able to penetrate into the hair cortex, but 10 to 13 amino acids residues make these peptides big enough to provide enhancement of strength and restructuring of the hair protein linkage network.10,16,30,32
Firstly, we made a comparative analysis to determine the best peptides, based on their amino acid sequences, isoelectric points, cysteine contents, and hydrophobic and polar amino acids34 (Fig. 2A). The affinity towards the hair fibre is determined by the specific characteristics of each peptide.56,57 The isoelectric point of the peptides influences the interaction profile with the hair keratin. The isoelectric point of human hair has been reported to be around 3.7,58 indicating that hair presents a negative net surface charge under treatments performed at pH 7. At this pH, the peptides PepD, PepE, PepF, PepG and KP also present a negative net charge, which will not favour the adsorption mechanism onto the hair fibre by these peptides. The hydrophobic character of the peptides is another important parameter for their cosmetic effect.10 Hydrophobic and hydrogen interactions contribute to the overall binding force of peptide–hair interactions. In terms of hydrophobic amino acid content, PepE is the most hydrophobic (60%), PepD, PepF and KP present medium hydrophobicity (around 40%), while PepA and PepG are the least hydrophobic (less than 10%). Due to the presence of some reactive groups in the amino acid sequences, other interactions such as polar interactions may be formed, enhancing the capability of some peptides for specific and directional binding.22 The KP peptide presents a high content of polar amino acids (46%). Peptides containing cysteine may also interact covalently with hair keratin where partial recombination or disruption of the disulphide bonds of the hair keratin is involved.59 Cysteine is a standard amino acid with a thiol group which has a strong tendency to reduce disulphide bonds in hair keratin.59 Disulphide bonds can be formed with the cysteine residues when there is an available electron acceptor, such as molecular oxygen in the solution, to oxidise the sulfhydryl groups of the cysteine residues into disulphide bonds. The peptides analysed contain two to five cysteines, Fig. 2A. The relative ratios of species were analysed by MALDI-TOF/TOF (Fig. 2B), revealing that PepA, PepB, PepD, PepF, PepG and KP formed as dimers; PepA, PepB, PepD and KP showed a ratio of approximately 50% dimer configuration; PepG was mainly found as a dimer (around 94%); PepC and PepE were only found in monomeric configurations; while PepF was found as a monomer with a ratio of around 87%.
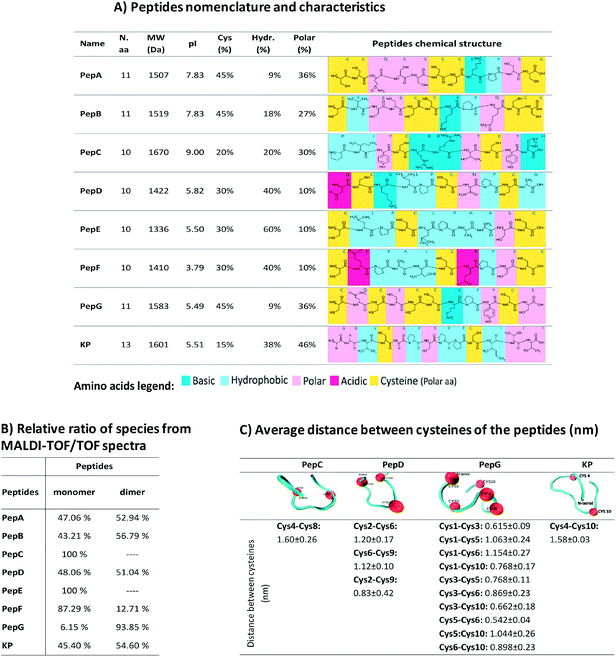 |
| Fig. 2 (A) Peptide nomenclature and characteristics: name, number of amino acids (N.a.a), molecular weight (MW), isoelectric point (pI), percentage of cysteines (Cys), percentage of hydrophobic (Hydr.) and polar amino acids in the peptide sequence, and peptide chemical structures; (B) relative ratio of species determined by MALDI-TOF/TOF; (C) distance between β-carbons of the cysteines of the peptides PepC, PepD, PepG and KP in the last 50 ns of simulation. | |
To further analyse the peptide conformations and their predisposition for the formation of internal disulphide bonds, molecular dynamics simulations were performed for PepC, PepD, PepG and KP. The selection of these peptides was based on the desirability of including a monomeric configuration (PepC), a dimeric configuration (PepG) and two peptides with the ability to form both configurations (PepD and KP). At the end of the simulations with these peptides in water, the GROMACS tool “g_cluster” was used to determine the most frequent spatial conformation of these peptides that occurred in the analysed simulation time. The tool was applied to 100 ns of simulation for each peptide, using a 0.1 nm root mean square cutoff for the calculations. The distances between the β-carbons of the cysteine residues in the peptides were measured in the most common spatial conformation according to the “g_cluster” results. In addition, the same distance was measured along the simulation time using the “g_dist” GROMACS tool and the average distance for the last 10 ns of simulation was calculated. This could provide some insights into the possible propensity of these peptides to form intramolecular disulphide bonds.
Several properties such as the variation of temperature, pressure and potential energy of the systems were calculated using GROMACS tools at the end of the interaction simulations. The parameters evaluated indicated that the systems were well equilibrated during the simulation time.
The average distance between the β-carbons of the cysteine residues of each peptide was measured for the last 50 ns (Fig. 2C); this time allowed the simulation to reach equilibrium. The distances obtained are much longer than the distances occurring in nature; disulphide bond lengthening at a transition state has been described from 0.24 Å to 0.78 Å.60 These distances may indicate peptide conformations with a predisposition for internal bond formation. PepD may have a predisposition for the formation of intramolecular disulphide bonds. PepG has five close cysteines in a total of 11 amino acids which may form several different bonds, such as Cys1–Cys3, Cys1–Cys10, Cys3–Cys5, Cys3–Cys6, Cys3–Cys10, and Cys5–Cys6. The distances between the cysteines of PepC and KP did not indicate a propensity for the formation of bonds between the cysteines. Most peptides alone showed a predisposition to form internal disulphide bonds between the cysteines.
Interaction of peptides with hair fibre
From the previous study with the microarray, the peptides were already known to interact with keratin extracted from human hair.34 Here, the peptides were analysed regarding their ability to interact with the entire hair fibre. Fig. 3 reveals the efficient interaction of the peptides with the human hair fibre, as well as their capability to recover the strength/elasticity of damaged hair and to aid in curl straightening.
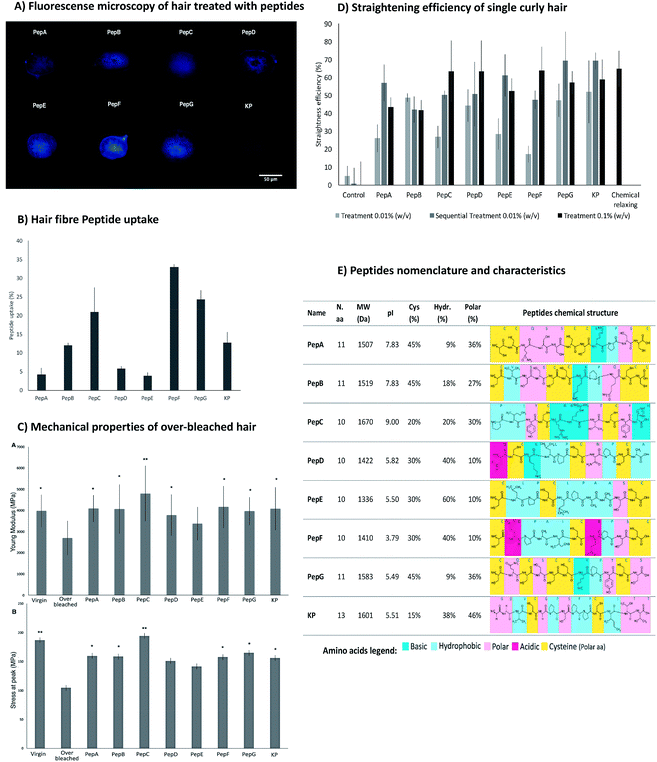 |
| Fig. 3 (A) Fluorescence microscopy of hair treated with peptides at 0.01% (w/v). (B) Peptide uptake by curly African hair measured in terms of absorbance at 497 nm for peptides PepA, PepB, PepC, PepD, PepE, PepF and PepG, and at 555 nm for KP. The hair samples were treated with 0.01% (w/v) of each peptide. (C) Young's modulus (part A) and stress at peak (part B) of Caucasian over-bleached hair (from left to right): virgin hair; over-bleached hair; over-bleached hair treated with 0.01% (w/v): PepA; PepB; PepC; PepD; PepE; PepF; PepG; and KP. p-Values ≤ 0.05 were considered statistically significant (*) and p-values ≤ 0.01, very significant (**), when compared with over-bleached hair. (D) Straightening efficiency of hair treatments for single curly African hair containing 0.01% (w/v) and 0.1% (w/v) of each peptide: control (phosphate buffer); PepA; PepB; PepC; PepD; PepE; PepF; PepG; KP; and with chemical relaxer (from left to the right). Data represent the mean ± standard deviation of independent experiments. (E) Peptide nomenclature and characteristics: name, number of amino acids (N.a.a), molecular weight (MW), isoelectric point (pI), percentage of cysteines (Cys), percentage of hydrophobic (Hydr.) and polar amino acids in the peptide sequence, and peptide chemical structures. | |
The affinity towards human hair fibre was analysed for all peptides using fluorescence microscopy analysis and peptide uptake by the hair fibre. These approaches were performed to confirm the degree of penetration in the binding of peptides along the structure of African hair (Fig. 3A and B). For a broader study, the ability of the peptides to penetrate and bind into straight over-bleached Caucasian hair was also verified (ESI, Fig. S3 and S4†). The peptides had the ability to penetrate into the hair cuticle and cortex for both types of hair as shown by the transversal cuts of hair treated with the peptides (Fig. 3A) and by the peptide uptake as determined by the variation in absorbance of the peptide solutions before and after hair treatments (Fig. 3B).16 The peptide uptake is dependent on both the hair fibre peptide binding ability and the extent of hair damage.
The obtained results were as expected: the damaged hair resulting from the over-bleaching treatment allowed a higher peptide uptake since the access to the hair cortex was easier in a hydrophilic fibre.
The hair's physical structure and the interactions between hair components endow the hair with remarkable mechanical properties. Chemical over-bleaching affects both the hair's structure and its interactions, leading to a significant loss of strength and elasticity.11,61,62 The treatment of the hair fibres with the peptides may re-establish some of the hydrogen and disulphide bonds lost during chemical treatment, improving the hair's mechanical properties. The effect of the peptides on the hair's mechanical properties was assessed through the evaluation of hair elasticity (Young's modulus) and stress at peak (Fig. 3C). The Young's modulus results from α-keratin stretching, which is resisted by disulphide bonds and by hydrogen bonds between turns that stabilize the helical structure of the keratin. After α-keratin stretching, a transition of the keratin from α-helix to β-sheet occurs, in which the keratin chains unfold without a high level of resistance. The measurement of the stress at peak corresponds to the resistance of β-sheets to stretch until reaching the rupture point.63–65
The treatments with the peptides increased the mechanical properties of the hair, as measured by both the Young's modulus and stress at peak. PepA, PepB, PepC, PepD, PepE, PepF, PepG and KP improved the Young's modulus resistance by up to 40% when compared with the over-bleached hair. PepC has high attachment with a positive net charge during the hair treatments, which increases its resistance to washing and its attraction to the hair surface, especially in damaged hair due to the more negative net charge of the hair cuticle. In addition, this peptide did not show a propensity to form intramolecular disulphide bonds but was able to interact with hair proteins. Both properties contribute to the high efficiency of this peptide to improve the mechanical properties of damaged hair. PepD has a close conformation which promotes the formation of intramolecular disulphide bonds, decreasing its propensity to interact with the hair surface, resulting in low fibre uptake and low improvement of hair mechanical properties.
The modification of African hair shape was demonstrated through the ability of the peptides to restructure the disulphide bonds between the peptide and hair fibre. The straightening of African hair was ensured with the binding of the peptides to the hair keratin, which created new bonds, fixed the protein conformation and consequently straightened the hair. The straightening effect of all peptides was clearly demonstrated by quantitative (Fig. 3D) and by visual analysis (for low peptide concentration, 0.01% (w/v), see ESI, Fig. S5†).
The straightening efficiency of the peptides for curly African hair ranged between 17% and 52%, with the best result being obtained for the KP peptide. In contrast, the hair treated with phosphate buffer in the absence of peptides presented a straightening efficiency of around 5%. A typical chemical relaxing treatment using sodium hydroxide as the relaxing agent led to a straightening efficiency of approximately 65%. For peptides PepC, PepD, PepF, PepG and KP, the straightening effect was dependent on concentration; a higher straightening efficiency was obtained for the highest peptide concentration. For 0.1% (w/v) peptide concentrations, the straightening efficiency of these peptides was around 60%, close to the result obtained with the chemical relaxing treatment. The sequential treatment which consisted of a series of three treatments using the same initial concentration (0.01% (w/v)) induced a straightening effect close to that of the chemical treatment for PepE, PepG and KP. The results indicate that the straightening efficiency of the peptides for the African hair fibre was deeply influenced by the peptide interactions, suggesting that the peptides were able to create stable disulphide bonds with the hair keratin.
Peptides can reform disulphide bonds with a large-chain model of hair protein and induce hair shape changes
The binding interaction between the peptides and hair proteins was demonstrated using a 45-mer peptide with high cysteine content, KeraPep, as a hair protein model. This peptide was based on the KAP 5–1 protein of the human hair. This peptide has the sequence: DDDDDKPCCCSSGCGSSCCQSSCCKPCCSQSSCCVPVCCQCKIDDDDD. It has five aspartates to provide improved solubility in aqueous solutions and 15 cysteine residues. It can be considered as a good model of hair protein to study the redox behaviour of disulphide bonding between hair proteins and smaller peptides. MALDI-TOF/TOF analysis revealed that KeraPep exists as a monomer (98.33% as monomer and 1.67% as dimer). The binding of the peptides to this model was analysed using MALDI-TOF/TOF at temperatures of 37 °C and 120 °C. All peptides formed aggregates with the hair protein model by the formation of new disulphide bonds, confirming their ability to interact with hair proteins (ESI, Fig. S2†), as already proven by the microarray research study34 and the previous tests with the human hair fibre. At 37 °C, some aggregates of KeraPep and three units of PepB and PepG were observed (Fig. 4A). An increase of temperature from 37 °C to 120 °C promoted an increase in the size of aggregates between the hair protein model and PepE, PepG and KP. The higher temperature increased the reformation of new disulphide bonds. These results show the ability of these peptides to bind to the hair proteins at higher temperatures, as is required when the hair is subjected to a hair dryer or flat iron, and confirm that a higher temperature favours the formation of the disulphide bonds.66 Hence, the temperature of the reaction is a crucial parameter for an enhanced interaction between the peptides and hair proteins. The re-modulation of the disulphide bonds occurred throughout the conjugation between the small peptides and the KeraPep.
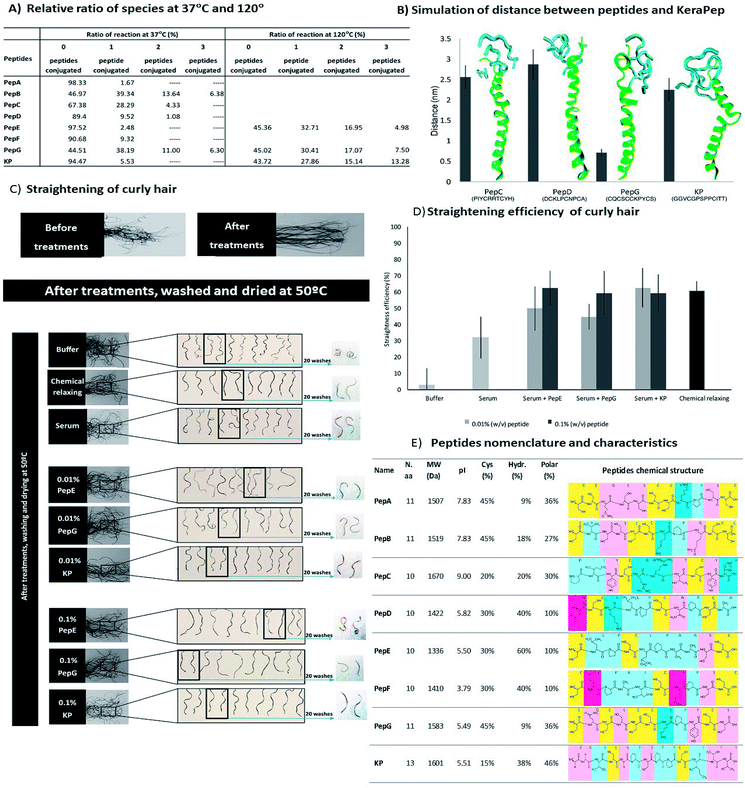 |
| Fig. 4 (A) Relative ratios for the reaction of the peptides and the KeraPep at 37 °C and at 120 °C obtained by MALDI-TOF/TOF. (B) Molecular dynamics simulations of the average distances in the last 10 ns of simulation between dimers of KeraPep and the peptides PepC, PepD, PepG and KP. The images reflect the final state of the simulations. (C) Curly hair tresses before and after peptide treatments; after treatments, the hair tresses were washed and dried at 50 °C. The bottom images present the samples treated with the serum formulations containing peptides (PepE, PepG, KP) at 0.1% (w/v); the images in the middle present the samples treated with the serum formulations containing peptides (PepE, PepG, KP) at 0.01% (w/v); the images at the top show the samples treated without peptides (buffer, chemical and base serum formulation treatments). (D) Straightening efficiency of serum treatments with peptides for curly hair tresses. (E) Peptide nomenclature and characteristics: name, number of amino acids (N.a.a), molecular weight (MW), isoelectric point (pI), percentage of cysteines (Cys), percentage of hydrophobic (Hydr.) and polar amino acids in the peptide sequence, and peptide chemical structures. | |
The KeraPep was further simulated using GROMACS. The peptides used for this simulation were the same as used for the simulations of the distances between β-carbons of the cysteines of each small peptide (PepC, PepD, PepG and KP). For this simulation, several properties were calculated to evaluate the interaction between these peptides and the KeraPep, including the distance, the number of hydrogen bonds, and the radial distribution function (RDF) between the KeraPep and the peptides. It was assumed that a smaller distance between a peptide and the KeraPep predicts a higher affinity between them (Fig. 4B). PepD gave a greater distance to the KeraPep. KP, and in particular PepG, maintained a closer distance to the KeraPep, suggesting their higher affinity for it and consequently for the hair proteins.
The capability to straighten African curly hair was also assessed by the incorporation of peptides into a serum formulation. The formulation containing the peptides was applied to hair tresses. The hair tresses were combed straight and mechanically straightened with a flat iron. The shape of the hair tresses was analysed for each peptide after treatment. The penetration of the peptides into the hair fibre was facilitated through their incorporation into the serum formulation containing benzyl alcohol, propylene glycol and ethanol. The hair tresses were treated with two concentrations (0.01% and 0.1% (w/v)) of the selected peptides: PepE, PepG and KP. The selection of these peptides was based on their straightening efficiencies in the three conditions used previously in the straightening hair treatments (Fig. 3D). Fig. 4 shows the qualitative and quantitative analysis of these treatments. The inclusion of the peptides into a serum formulation was done in order to simulate the effect of the peptides as straightening agents in a commercial formulation. The development of a cosmetic product as a green alternative to the chemical relaxing treatment was the motivation behind the work here presented. Thus, it was essential to evaluate the straightening effect of the peptides when included in a formulation.
The straightening effects of PepE, PepG and KP when applied in a serum formulation were not affected when compared to their effects in phosphate buffer (Fig. 4C and D), with efficiencies of up to 60% for all peptides and for both concentrations. These effects were of the same magnitude as for the chemical treatment. For peptides PepE and PepG, the straightening efficiency was improved by increasing the concentration of peptide in the serum formulation. The base serum formulation itself (without peptide) showed a slight improvement of the straightening efficiency, at around 30%, when compared with the phosphate buffer treatment. This could be attributed to the formation of bonds with the hair keratin. The serum formulation facilitates the penetration of peptides into the hair fibre, which promotes the re-shaping of disulphide bonds, changing the shape of the hair. The re-shaping of the hair was demonstrated qualitatively and quantitatively (Fig. 4C and D, respectively), where PepE, PepG and KP were able to promote a significant change in the hair shape. The effect of these peptide formulations on the African hair lasted for at least 20 washes with shampoo, with the exception of PepE at the concentration of 0.1% (w/v).
Comparing the straightening efficiency of the peptides without the serum formulation (single curly hair) and with the serum formulation (curly hair tresses) it was evident that there was an improvement in the effects of the peptides when they were included in the serum formulation. The serum formulation has previously been used to promote the penetration of peptides into the hair cortex.55 Besides, it may also promote a stabilization and change of peptide conformation aiding the formation of bonds between the peptide and the hair. Peptide interactions are affected by their functional groups, as well as by their involvement in building new bonds or interactions with hair proteins. Peptides have the ability to bind to hair proteins, be absorbed by the hair fibre, improve damaged hair mechanical properties and aid the straightening of curly hair. Each peptide has a specific amino acidic sequence which is crucial for its behaviour towards hair proteins. Due to the conformation of each peptide, the final results are highly dependent on the reactivity of each cysteine and its accessibility; on the residues in the vicinity of the cysteine; on the charge–charge interactions and on the location of the cysteine.67 Basically, the peptide mechanism involves the breakage of the disulphide bonds between hair keratin filaments and then the re-modulation of the hair occurs by the disulphide bond rearrangements between the hair proteins and peptides. The formation of the disulphide bonds between the cysteine thiols presented in the peptides with the hair keratin or KAPs is due to the reduction of the hair protein disulphide bonds and the reformation of these disulphide bonds with the thiol of the peptides and even with themselves. Sulphur atoms of cysteines may also be linked by hydrogen bonds or electrostatic interactions.67 PepE, despite presenting a negative charge during the treatment, improved the hair's mechanical properties. However, its efficiency as a straightening agent was not as evident as that of peptides PepG and KP. This is mainly due to the eventual formation of assemblies based on hydrophobic and polar interactions in PepE. This type of binding and penetration profile is possible in peptides with net negative charge.28,68 PepG and KP present net negative charges; however, their abilities to form disulphide bonds with the hair fibre were more effective. Their conformation and the accessibility of their cysteine residues were imperative for the higher conjugation displayed with hair keratin which led to the effective recovery of chemically damaged hair and efficient straightening of African hair. The effect of the treatments with these peptides was sustained for at least 20 washes with shampoo, confirming the long durability of the treatments.
These results open an innovative way to repair damaged hair fibre from severe and repetitive treatments (e.g. coloration, and perming) and to straighten curly hair, while avoiding conventional chemicals and preserving the integrity of the hair structure.
Conclusions
Although conventional chemical treatments are the most popular methods used to change the style of the hair, they represent a threat to the hair, the user and the environment. The repetitive use of conventional cosmetic chemical treatments can severely affect hair physicochemical properties. Eight engineered small peptides based on fragments of human hair keratin and KAPs were designed to restore hair properties and to straighten curly hair. These peptides, when applied to chemically over-bleached hair and curly African hair, were shown to penetrate the hair fibre into the cortex and to bind to the hair proteins. Due to this binding, they induced a significant recovery in the tensile strength and elasticity of severely damaged hair. Of the eight tested peptides, PepE, PepG and KP showed the highest ability to interact with a keratin peptide model. Based on this result, the three peptides were explored as modulators of hair shape. When applied to curly hair, these peptides were shown to enable a high straightening efficiency without the use of harsh chemicals. These results were supported by molecular dynamics simulations and by MALDI-TOF/TOF, which demonstrated effective conjugation between the small peptides and the peptide hair protein model, based on the re-conformation of disulphide bonds. Peptide treatments at neutral pH involve the formation of intra and intermolecular disulphide bonds between cysteine-based peptides and hair proteins and show great potential for cosmetic use as modulators of hair shape. The new method here proposed, based on peptide formulations, is environmentally friendly and constitutes a real alternative to the conventional chemical treatments, opening a new chapter for a green haircare cosmetic industry.
Conflicts of interest
There are no conflicts to declare.
Acknowledgements
This work was supported by the Portuguese Foundation for Science and Technology (FCT) under the scope of the strategic funding of the UID/BIO/04469/2013 unit and COMPETE 2020 (POCI-01-0145-FEDER-006684) and under the Project RECI/BBB-EBI/0179/2012 (FCOMP-01-0124-FEDER-027462). This study was also supported by BioTecNorte operation (NORTE-01-0145-FEDER-000004) funded by the European Regional Development Fund under the scope of Norte2020 – Programa Operacional Regional do Norte. Célia F. Cruz and Artur Ribeiro thank FCT for SFRH/BD/100927/2014 and SFRH/BPD/98388/2013 grants, respectively.
References
- A. L. Miranda-Vilela, A. J. Botelho and L. A. Muehlmann, Int. J. Cosmet. Sci., 2014, 36, 2–11 CrossRef CAS PubMed.
- M. H. Maneli, P. Smith and N. P. Khumalo, J. Am. Acad. Dermatol., 2014, 70, 276–280 CrossRef CAS PubMed.
- C. L. Galli, F. Bettin, P. Metra, P. Fidente, E. De Dominicis and M. Marinovich, Regul. Toxicol. Pharmacol., 2015, 72, 562–568 CrossRef CAS PubMed.
- O. A. Olsasode and O. A. Olasode, J. Pak. Assoc. Derma., 2009, 203–207 Search PubMed.
- A. J. McMichael, J. Invest. Dermatol. Symp. Proc., 2007, 12, 6–9 CrossRef PubMed.
- B. J. Kaur, H. Singh and A. Lin-Greenberg, J. Natl. Med. Assoc., 2002, 94, 121–213 Search PubMed.
- Encyclopedia of Toxicology, ed. P. Wexler, Elsevier, 3rd edn, 2014 Search PubMed.
- J. M. Dyer, F. Bell, H. Koehn, J. A. Vernon, C. D. Cornellison, S. Clerens and D. P. Harland, Int. J. Cosmet. Sci., 2013, 35, 555–561 CrossRef CAS PubMed.
- C. R. Robbins, Chemical and physical behavior of human hair, Springer, Berlin, Heidelberg, 4th edn, 2002 Search PubMed.
- M. F. Gavazzoni Dias, Int. J. Trichol., 2015, 7, 2 CrossRef PubMed.
- M. T. Korte, S. Akari, H. Kuehn, N. Baghdadli, H. Möhwald and G. S. Luengo, Langmuir, 2014, 30, 12124–12129 CrossRef CAS PubMed.
- C. Barba, S. Scott, A. Roddick-Lanzilotta, R. Kelly, A. M. Manich, J. L. Parra and L. Coderch, Fibers Polym., 2010, 11, 1055–1061 CrossRef CAS.
- J. Gray, Clin. Dermatol., 2001, 19, 227–236 CrossRef CAS PubMed.
- C. Bolduc and J. Shapiro, Clin. Dermatol., 2001, 19, 431–436 CrossRef CAS PubMed.
- R. Dawber, Clin. Dermatol., 1996, 14, 105–112 CrossRef CAS PubMed.
- M. M. Fernandes and A. Cavaco-Paulo, Biocatal. Biotransform., 2012, 30, 10–19 CrossRef CAS.
- C. Barba, M. Martí, A. Roddick-Lanzilotta, A. Manich, J. Carilla, J. L. Parra and L. Coderch, J. Therm. Anal. Calorim., 2010, 102, 43–48 CrossRef CAS.
- B. Bhushan, in Biophysics of Human Hair, Springer Berlin Heidelberg, Berlin, Heidelberg, 2010, pp. 1–19 Search PubMed.
- J. A. Rippon, in Wool dyeing, ed. D. M. Lewis, Bradford: Society of Dyers and Colourists, Bradford, England, 1992, pp. 1–51 Search PubMed.
- C. Popescu and H. Hocker, Chem. Soc. Rev., 2007, 36, 1282–1291 RSC.
- A. Franbourg, P. Hallegot, F. Baltenneck, C. Toutain and F. Leroy, J. Am. Acad. Dermatol., 2003, 48, S115–S119 CrossRef CAS PubMed.
- L. J. Wolfram, J. Am. Acad. Dermatol., 2003, 48, 106–114 CrossRef PubMed.
- M. Er Rafik, J. Doucet and F. Briki, Biophys. J., 2004, 86, 3893–3904 CrossRef CAS PubMed.
- The Science of Hair Car, ed. C. Bouillon and J. Wilkinson, Taylor & Francis, 2nd edn, 2005 Search PubMed.
- In New Cosmetic Science, ed. T. Mitsui, Elsevier, 1st edn, 1998, pp. 12–14 Search PubMed.
- J. Knowlton and S. Pearce, Handbook of Cosmetic Science and Technology, Elsevier Advanced Technology, 3rd edn, 1993 Search PubMed.
- Principles of Polymer Science and Technology in Cosmetics and Personal Care, ed. D. E. Goodard and J. V. Gruber, Marcel Dekker, Inc., 1999, vol. 68 Search PubMed.
- M. M. Fernandes, C. F. Lima, A. Loureiro, A. C. Gomes and A. Cavaco-Paulo, Int. J. Cosmet. Sci., 2012, 34, 1–9 CrossRef PubMed.
- A. Ribeiro, T. Matamá, C. F. Cruz, A. C. Gomes and A. M. Cavaco-Paulo, Int. J. Cosmet. Sci., 2013, 35, 458–466 CrossRef CAS PubMed.
- E. Oshimura, H. Abe and R. Oota, J. Cosmet. Sci., 2007, 58, 347–357 CAS.
- A. L. V. Villa, M. R. S. Aragão, E. P. Dos Santos, A. M. Mazotto, R. B. Zingali, E. P. de Souza and A. B. Vermelho, BMC Biotechnol., 2013, 13, 15 CrossRef CAS PubMed.
- G. Secchi, Clin. Dermatol., 2008, 26, 321–325 CrossRef PubMed.
- R. Schueller and P. Romanowski, in Beginning Cosmetic Chemistry, 2009, pp. 167–169 Search PubMed.
- C. F. Cruz, N. G. Azoia, T. Matamá and A. Cavaco-Paulo, Int. J. Biol. Macromol., 2017, 101, 805–814 CrossRef CAS PubMed.
- K. Wang, R. Li, J. H. Ma, Y. K. Jian and J. N. Che, Green Chem., 2016, 18, 476–481 RSC.
- H. J. C. Berendsen, D. van der Spoel and R. van Drunen, Comput. Phys. Commun., 1995, 91, 43–56 CrossRef CAS.
- E. Lindahl, B. Hess and D. van der Spoel, J. Mol. Model., 2001, 7, 306–317 CrossRef CAS.
- W. R. P. Scott, P. H. Hünenberger, I. G. Tironi, A. E. Mark, S. R. Billeter, J. Fennen, A. E. Torda, T. Huber, P. Krüger and W. F. van Gunsteren, J. Phys. Chem. A, 1999, 103, 3596–3607 CrossRef CAS.
- N. Schmid, A. P. Eichenberger, A. Choutko, S. Riniker, M. Winger, A. E. Mark and W. F. van Gunsteren, Eur. Biophys. J., 2011, 40, 843–856 CrossRef CAS PubMed.
- W. Huang, Z. Lin and W. F. van Gunsteren, J. Chem. Theory Comput., 2011, 7, 1237–1243 CrossRef CAS PubMed.
- B. Hess, H. J. C. Berendsen and J. G. E. M. Fraaije, J. Comput. Chem., 1997, 18, 1463–1472 CrossRef CAS.
- S. Miyamoto and P. A. Kollman, J. Comput. Chem., 1992, 13, 952–962 CrossRef CAS.
- J. Hermans, H. J. C. Berendsen, W. F. Van Gunsteren and J. P. M. Postma, Biopolymers, 1984, 23, 1513–1518 CrossRef CAS.
- H. J. C. Berendsen, W. F. van Gunsteren, A. DiNola and J. R. Haak, J. Chem. Phys., 1984, 3684–3690 CrossRef CAS.
- G. Bussi, D. Donadio and M. Parrinello, J. Chem. Phys., 2007, 126, 14101 CrossRef PubMed.
- M. Parrinello and A. Rahman, J. Appl. Phys., 1981, 52, 7182–7190 CrossRef CAS.
- S. Nosé and M. L. Klein, Mol. Phys., 1983, 50, 1055–1076 CrossRef.
- P. E. Smith and W. F. van Gunsteren, J. Chem. Phys., 1994, 100, 3169 CrossRef CAS.
- G. Yachdav and B. Rost, PredictProtein, http://www.predictprotein.org.
- A. S. Ursula Pieper, B. M. Webb, G. Q. Dong, D. Schneidman-Duhovny, H. Fan, S. J. Kim, N. Khuri, Y. G. Spill, P. Weinkam, M. Hammel, J. A. Tainer and M. Nilges, Nucleic Acids Res., 2014, 42, D336 CrossRef PubMed.
- W. Humphrey, A. Dalke and K. Schulten, J. Mol. Graphics, 1996, 14, 33–38 CrossRef CAS PubMed.
- G. Loussouarn, A.-L. Garcel, I. Lozano, C. Collaudin, C. Porter, S. Panhard, D. Saint-Léger and R. de La Mettrie, Int. J. Dermatol., 2007, 46(suppl. 1), 2–6 CrossRef PubMed.
- D. H. Kirby, Proc. Sci. Sect. Toilet Goods Assoc., 1956, 26, 12-5 Search PubMed.
- A. Barrel, M. Paye and H. Maibach, Handbook of Cosmetic Science and Technology, CRC Press, 4th edn, 2014 Search PubMed.
- E. Antunes, C. F. Cruz, N. G. Azoia and A. Cavaco-Paulo, RSC Adv., 2015, 5, 12365–12371 RSC.
- M. F. Roberto Bessalle, A. Gorea, I. Shalit, J. W. Metzger, C. Dass and D. M. Desiderio, J. Med. Chem., 1993, 6, 1203–1209 CrossRef.
- R. Wimmer, K. K. Andersen, B. Vad, M. Davidsen, S. Mølgaard, L. W. Nesgaard, H. H. Kristensen and D. E. Otzen, Biochemistry, 2006, 45, 481–497 CrossRef CAS PubMed.
- V. A. Wilkerson, J. Biol. Chem., 1935, 329–359 CAS.
- A. Teglia and G. Secchi, in Principles of polymer science and technology in cosmetic and personal care, 1999, pp. 404–477 Search PubMed.
- P. A. Fernandes and M. J. Ramos, Chem.–Eur. J., 2004, 10, 257–266 CrossRef CAS PubMed.
- B. Erik, H. Havitcioglu, S. Aktan and N. Karakus, Skin Res. Technol., 2008, 14, 147–151 CrossRef PubMed.
- B. Bhushan, Prog. Mater. Sci., 2008, 53, 585–710 CrossRef CAS.
- G. Wei, B. Bhushan and P. M. Torgerson, Ultramicroscopy, 2005, 105, 248–266 CrossRef CAS PubMed.
- M. V. R. Velasco, T. C. d S. Dias, A. Z. De Freitas, N. D. V. Júnior, C. Aparecida Sales de Oliveira Pinto, T. M. Kaneko and A. R. Baby, Braz. J. Pharm. Sci., 2009, 45, 153–162 CrossRef.
- C. Popescu and H. Höcker, Int. Rev. Cell Mol. Biol., 2009, 277, 137–156 CAS.
- V. I. Abkevich and E. I. Shakhnovich, J. Mol. Biol., 2000, 300, 975–985 CrossRef CAS PubMed.
- G. Roos, N. Foloppe and J. Messens, Antioxid. Redox Signaling, 2013, 18, 94–127 CrossRef CAS PubMed.
- M. M. Fernandes, A. C. Gomes, A. Vasconcelos, F.-D. Munteanu, T. Tzanov, M. S. T. Gonçalves, N. End, K.-U. Schoening, G. M. Guebitz and A. Cavaco-Paulo, Appl. Microbiol. Biotechnol., 2011, 90, 1311–1321 CrossRef CAS PubMed.
- T. C. de Sá Dias, A. R. Baby, T. M. Kaneko and M. V. Robles Velasco, J. Cosmet. Dermatol., 2007, 6, 2–5 CrossRef PubMed.
- M. A. Manuszak, E. T. Borish, R. R. Wickett, E. T. Borush and R. R. Wickett, J. Soc. Cosmet. Chem., 1996, 47, 213–227 CAS.
- M. A. Manuszak, E. T. Borish and R. R. Wickett, J. Soc. Cosmet. Chem., 1996, 47, 49–58 CAS.
- C. Zviak and A. Sabbagh, in The science of hair care, ed. C. Bouillon and J. Wilkinson, Taylor & Francis, 2nd edn, 2005, pp. 217–244 Search PubMed.
- C. Weathersby and A. McMichael, J. Cosmet. Dermatol., 2013, 12, 144–148 CrossRef PubMed.
Footnotes |
† Electronic supplementary information (ESI) available. See DOI: 10.1039/c7ra10461h |
‡ Both authors contributed equally to this work. |
|
This journal is © The Royal Society of Chemistry 2017 |
Click here to see how this site uses Cookies. View our privacy policy here.