DOI:
10.1039/D1RA05090G
(Review Article)
RSC Adv., 2021,
11, 31235-31259
A review on the phytochemistry and pharmacology of the herb Scoparia dulcis L. for the potential treatment of metabolic syndrome
Received
1st July 2021
, Accepted 11th September 2021
First published on 22nd September 2021
Abstract
This review discusses the chemical constituents and pharmacological effects of Scoparia dulcis L. (S. dulcis) plants. So far, approximately 160 compounds have been identified from S. dulcis, among which 115 compounds may be related to the treatment of metabolic syndrome. Extracts of S. dulcis have effects of reducing fasting blood glucose level, increasing the plasma insulin level, and stimulating insulin secretion to treat diabetes. They also produce antihyperlipidemic effects by increasing serum high-density lipoprotein levels, the anti-atherogenic index of plasma, and HMG-CoA reductase activity. The chemical composition of glutinol and glutinone, isolated from S. dulcis, provide potential anti-inflammatory effects. These compounds can also reduce total cholesterol, triacylglycerol, and low-density lipoprotein (LDL)-cholesterol and increase high-density lipoprotein (HDL)-cholesterol to provide the anti-atherosclerotic effect. S. dulcis exerts anti-arthritic properties through its effect on cytokine levels, significantly reducing IFN-γ and IL-6 levels and elevating IL-10 levels. The extracts carry out hepatoprotective effect by preventing the descent of the antioxidative enzymes of superoxide dismutase (SOD), glutathione peroxidase (GPx), glutathione reductase (GRd), and glutathione-S-transferase (GST). Therefore, S. dulcis provides new potential for medicine given its numerous therapeutic properties and can be promoted as a complementary or alternative therapy for patients with chronic conditions.
Introduction
Scoparia dulcis L. (S. dulcis) is a medicinal botanical herb that has been widely used for generations in southern China, India, Brazil, Paraguay, and Nigeria. Traditional Chinese medicine believes that S. dulcis has stomachic, diuretic, antitussive, heat clearing, and toxin absorbing effects. It is applied in traditional Chinese herbal medicine to treat colds, fever, coughs with lung heat, sore throats, enteritis, abnormal urination, hunger swelling, eczema, and miliaria since ancient times. S. dulcis is also commonly used in herbal tea for health benefits in southern China. In other ethnomedicine communities, S. dulcis is also used to treat gastric problems, edema, liver diseases, and respiratory diseases.1 Chemical analysis of S. dulcis identified various components including nitrogen-containing compounds, flavonoids, diterpenoids, triterpenoids, steroids, phenolics, and aliphatics, which can demonstrate its definite pharmacological effects. As a result, a large number of researchers started to study the chemical components and pharmacological activities through different extract methods from S. dulcis and confirmed that S. dulcis provides various pharmacological effects, related to the treatment of metabolic syndrome. These include antidiabetic, anti-hyperlipidaemia, anti-inflammatory, anti-atherosclerotic, anti-arthritic, hepatoprotective, anti-oxidative, and anti-urolithiasis94 activities. The incidence of metabolic syndrome in the world has been gradually increasing, especially in some developing countries, is even higher than some developed countries.2 Thus, the chemical substance basis of and current pharmacological research on S. dulcis will be comprehensively reviewed to explain its potential role in the treatment of metabolic syndrome. In addition to complementing the use of conventional drugs, some common herbs can guide new drug discoveries, which are also important developments in this field. Therefore, the updated and comprehensive review of the phytochemical and pharmacological properties of S. dulcis will provide the foundation for the potential development of new medicine in the treatment of metabolic syndrome.
Methodology and aim of the review
This literature collection on S. dulcis was conducted mainly with the SciFinder covering the period from 2000 to 2020. The other electronic sources used were from scientific databases, including PubMed, ScienceDirect, Web of Science, Google Scholar, Baidu Scholar, Bing academic, ResearchGate, and CNKI. The local literature of traditional Chinese medicine such as Fujian folk herbal medicine, Records of traditional Chinese medicine in Guangxi, Folk herbal medicine in Southern Fujian, Chinese herbal medicine in Guangxi, Manual of commonly used Chinese herbal medicines by Guangzhou Army, Chinese Materia Medica, National collection of Chinese herbal medicines, and Chinese herbal medicine in Fujian, were referenced as well.
To date, there is little literature reviewing the phytochemical and pharmacological basis of S. dulcis for the treatment of metabolic syndrome. Previous review article of Mishra et al.3 had briefly introduced some chemical constituents and pharmacological effects of S. dulcis. However, the article does not describe the correlation between the various pharmacological actions in detail. In the literature review of Pamunuwa et al.,4 the authors mainly introduced the therapeutic effect of S. dulcis on diabetes and only briefly mentioned the other pharmacological effects of S. dulcis.
This review article was mainly focused on the treatment of metabolic syndrome to investigate the relevant medical chemistry and pharmacological mechanisms of S. dulcis. At the same time, the related pharmacological effects and mechanisms confirmed in vivo and in vitro experiments can also provide inspiration for the pharmaceutical industry and food supplement to develop the potential medicine or food to treat the metabolic syndrome.
Chemical constituents
There are 115 compounds in S. dulcis that have therapeutic potential for the treatment of metabolic syndrome. The chemical structures of compounds in S. dulcis are listed in the following section. These chemical substances can be roughly divided into the following categories: nitrogen-containing compounds, flavonoids, diterpenoids, triterpenoids, steroids, phenolics, and other aliphatics. So far, the flavonoids, diterpenoids, and alkaloids contained in S. dulcis are the most diverse. Each of the compounds has been marked with a number (1–115), and some of them have defined unique biological activities. The antidiabetic, hypolipidemic, anti-inflammatory, and antioxidative effects of these compounds are the molecular basis for the use of S. dulcis in the treatment of metabolic syndrome. The compounds are extracted from different parts of S. dulcis, including the whole plant, aerial parts, leaves, and roots. Most of the compounds are isolated from the leaves of S. dulcis. The pharmacological activities of some compounds need to be further studied, and some compounds are expected to be further researched and developed as medicines for the treatment of metabolic syndrome.
Nitrogen-containing compounds
Nitrogen containing compounds, of which alkaloids are the most important, are very commonly found in plants. In recent years, studies have found that some benzoxazin-3-one derivatives can act as mineralocorticoid receptor antagonists to treat hypertension, thereby treating some cardiovascular diseases.5 2-hydroxy-2H-1,4-benzoxazol-3-one in S. dulcis was discovered by Kamperdick et al.6 Benzoxazine and benzoxazolinone were reported by Babincova et al.7 and Mishra et al.3 Coixol, 1-hydroxy-6-methoxy-2-benzoxazolinon, 3,6-dimethoxy-benzoxazolin-2(3H)-one, (2R)-2-(β-D-glucopyranosyloxy)-7-methoxy-2H-1,4-benzoxazin-3(4H)-one, (2R)-2-(β-D-glucopyranosyloxy)-4,7-dimethoxy-2H-1,4-benzoxazin-3(4H)-one, and (2R)-7-methoxy-2H-1,4-benzoxazin-3(4H)-one 2-O-β-galactopyranoside were isolated from the water extract of the dried aerial parts of S. dulcis by Wu et al.8 Dextromoramide and 2-heptadecyl-2-imidazoline were identified in the methanol extract and 1-methyl-2-pyrrolidinone, N1-acetylspermine, cyclohexylamine, and procaine were identified in the water extract of S. dulcis by Wankhar et al.9 Tin et al.10 investigated the methanol fraction of whole plants of S. dulcis and isolated 2-hydroxy-7-methoxy-1,4(2H)-benzoxazin-3-one 2-O-β-D-glucopyranoside. In addition, two catecholamines, epinephrine and norepinephrine, were identified in the aqueous extract of S. dulcis by Freire et al.11 Epinephrine and norepinephrine act on α and β receptors, to accelerate heart rate and raise blood pressure. The chemical structures of the nitrogen-containing compounds are shown in Table 1.
Table 1 The chemical structures of nitrogen-containing compounds, extracted from various part of S. dulcis
No. |
Compound |
Part of plant |
Chemical structure |
CAS number |
Chemical formula |
Ref. |
1 |
2-Hydroxy-2H-1,4-benzoxazol-3-one |
Whole plant |
 |
|
C8H7NO3 |
6 |
2 |
Benzoxazine |
Whole plant |
 |
254-18-2 |
C8H7NO |
7 |
3 |
Benzoxazolinone |
Whole plant |
 |
59-49-4 |
C7H5NO2 |
7 |
4 |
Coixol |
Aerial |
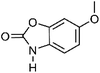 |
532-91-2 |
C8H7NO3 |
8 |
5 |
1-Hydroxy-6-methoxy-2-benzoxazolinon |
Aerial |
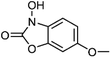 |
1402088-77-0 |
C8H7NO4 |
8 |
6 |
3,6-Dimethoxy-benzoxazolin-2(3H)-one |
Aerial |
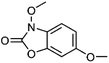 |
1402088-76-9 |
C9H9NO4 |
8 |
7 |
(2R)-2-(β-D-Glucopyranosyloxy)-7-methoxy-2H-1,4-benzoxazin-3(4H)-one |
Aerial |
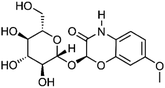 |
113565-31-4 |
C15H19NO9 |
8 |
8 |
(2R)-2-(β-D-Glucopyranosyloxy)-4,7-dimethoxy-2H-1,4-benzoxazin-3(4H)-one |
Aerial |
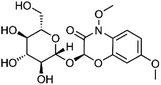 |
113565-33-6 |
C16H21NO10 |
8 |
9 |
(2R)-7-Methoxy-2H-1,4-benzoxazin-3(4H)-one 2-O-β-galactopyranoside |
Aerial |
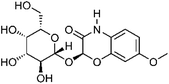 |
1402088-75-8 |
C15H19NO9 |
8 |
10 |
7-Methoxy-2,4-hydroxy-1,4-benzoxazin-3(2H)-one |
Whole plant |
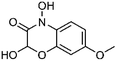 |
15893-52-4 |
C9 H9 NO5 |
3 |
11 |
Dextromoramide |
Leaves |
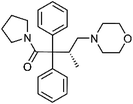 |
357-56-2 |
C25H32N2O2 |
9 |
12 |
2-Heptadecyl-2-imidazoline |
Leaves |
 |
105-28-2 |
C20H40N2 |
9 |
13 |
1-Methyl-2-pyrrolidinone |
Leaves |
 |
872-50-4 |
C5H9NO |
9 |
14 |
N1-Acetylspermine |
Leaves |
 |
25593-72-0 |
C12H28N4O |
9 |
15 |
Cyclohexylamine |
Leaves |
 |
108-91-8 |
C6H13N |
9 |
16 |
Procaine |
Leaves |
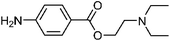 |
59-46-1 |
C13H20N2O2 |
9 |
17 |
Epinephrine |
Aerial |
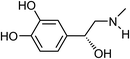 |
51-43-4 |
C9H13NO3 |
11 |
18 |
Norepinephrine |
Aerial |
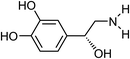 |
51-41-2 |
C8H11NO3 |
11 |
Flavonoids
Flavonoids are one of the most important compounds in S. dulcis. Flavonoids generally refer to a series of compounds composed of two benzene rings (A ring and B ring) with phenolic hydroxyl groups connected to each other through three central carbon atoms, creating a compound composed of C6–C3–C6 units. So far, the flavonoids in S. dulcis can be roughly divided into flavones, flavonols, flavan-3-ols, and flavanones.
Flavones
According to the current literature research, most of the flavonoids found in S. dulcis are flavones. Scutellarin methylester, cynaroside, 5,7,8,3′,4′,5′-hexahydroxy-flavone glucuronide, and 5-hydroxy-6,7-dimethoxyflavone-4′-O-β-glucose were isolated from S. dulcis by Kawasaki et al.12 5,7,8,3′,4′,5′-hexahydroxy-flavone glucuronide and iso-vitexin showed β-glucuronidase inhibitory activity in the experiments.12 In the same year, Hayashi et al.13 used 70% ethanol to extract the above-ground parts of S. dulcis, and isolated 5,7-dihydroxy-3′4′,6,8-tetrametoxyflavone from it. This compound exhibited significant cytotoxic activity against HeLa 299 and S3 cell lines with ID50 values of 0.097 and 0.140, respectively. Three flavone glycosides, 5,6,4′-trihydroxyflavone 7-O-α-L-2,3-di-O-acetylrhamnopyranosyl-(1→6)-β-D-glucopyranoside, apigenin 7-O-α-L-2,3-di-O-acetylrhamnopyranosyl-(1→6)-β-D-glucopyranoside, and apigenin 7-O-α-L-3-O-acetylrhamnopyranosyl-(1→6)-β-D-glucopyranoside were isolated from methanol extract of S. dulcis for the first time.14 The latter two compounds have been experimentally confirmed to have the effect of promoting NGF-induced neurite outgrowth in PC12D cells. Acacetin, cirsimarin, cynaroside, linarin, vicenin-2, vitexin, and iso-vitexin were reported by Mishra et al.3 and some of them have antioxidative and hypotensive activities. Liu et al.15 determined apigenin, luteolin, homoplantaginin, acerosin, pectolinarin, scutellarein, hispidulin, and apigenin-8-C-α-L-arabinopyranoside from the 70% aqueous acetone extract of the dry aerial part of S. dulcis. Among them, scutellarein, hispidulin, apigenin, and luteolin have been tested to have PPAR-γ (peroxisome proliferator-activated receptor gamma) agonistic effects with EC50 values of about 0.9–24.9 μM. Apigenin-7-O-glucuronide, hispidulin-7-O-glucuronide, nevadensin, cirsimaritin, cirsiliol, and salvigenin were identified by HPLC-MS/MS analysis of methanol extract from leaves of S. dulcis.16 Further experiments showed that the ethyl acetate extracts of S. dulcis have potential α-glucosidase and tyrosinase inhibitory activity. Scutellarin, isorhoifolin, and scutellarein 7-O-α-glucuronamide were also reported in S. dulcis.7,8,10 The chemical structures of flavones compounds are shown in Table 2.
Table 2 The chemical structures of flavones compounds, extracted from various part of S. dulcis
No. |
Compound |
Part of plant |
Chemical structure |
CAS number |
Chemical formula |
Ref. |
19 |
Scutellarin methylester |
Whole plant |
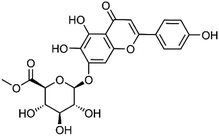 |
|
C22H20O12 |
12 |
20 |
Scutellarin |
Whole plant |
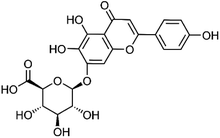 |
27740-01-8 |
C21H18O12 |
7 |
21 |
5,7,8,3′,4′,5′-Hexahydroxy-flavone glucuronide |
Whole plant |
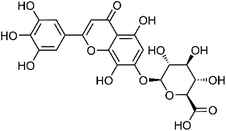 |
|
C21H18O14 |
12 |
22 |
5-Hydroxy-6,7-dimethoxyflavone-4′-O-β-glucose |
Whole plant |
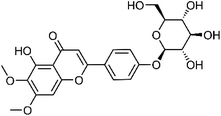 |
|
C23H24O11 |
12 |
23 |
iso-Vitexin |
Leaves |
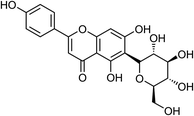 |
38953-85-4 |
C21H20O10 |
3 |
24 |
5,7-Dihydroxy-3′4′,6,8-tetrametoxyflavone |
Whole plant |
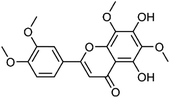 |
56003-01-1 |
C19H18O8 |
13 |
25 |
Acerosin |
Aerial |
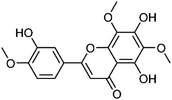 |
15835-74-2 |
C18H16O8 |
15 |
26 |
Nevadensin |
Leaves |
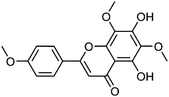 |
10176-66-6 |
C18H16O7 |
16 |
27 |
5,6,4′-Trihydroxyflavone 7-O-α-L-2,3-di-O-acetylrhamnopyranosyl-(1→6)-β-D-glucopyranoside |
Whole plant |
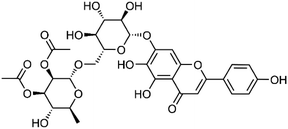 |
682354-22-9 |
C31H34O17 |
14 |
28 |
Apigenin 7-O-α-L-2,3-di-O-acetylrhamnopyranosyl-(1→6)-β-D-glucopyranoside |
Whole plant |
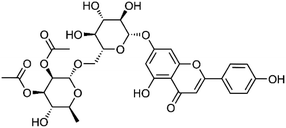 |
682354-24-1 |
C31H34O16 |
14 |
29 |
Apigenin 7-O-α-L-3-O-acetylrhamnopyranosyl-(1→6)-β-D-glucopyranoside |
Whole plant |
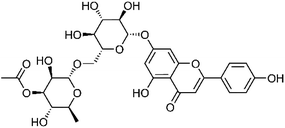 |
682354-23-0 |
C29H32O15 |
14 |
30 |
Acacetin |
Whole plant |
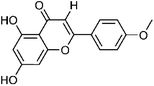 |
480-44-4 |
C16H12O5 |
3 |
31 |
Apigenin |
Whole plant |
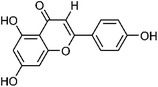 |
520-36-5 |
C15H10O5 |
15 |
32 |
Cirsimarin |
Whole plant |
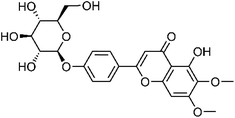 |
13020-19-4 |
C23H24O11 |
3 |
33 |
Cynaroside |
Whole plant |
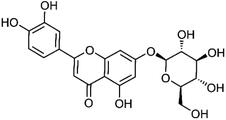 |
5373-11-5 |
C21H20O11 |
3 |
34 |
Homoplantaginin |
Aerial |
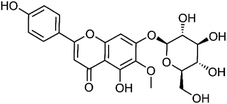 |
17680-84-1 |
C22H22O11 |
15 |
35 |
Linarin |
Whole plant |
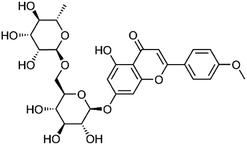 |
480-36-4 |
C28H32O14 |
3 |
36 |
Pectolinarin |
Aerial |
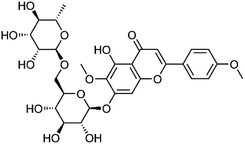 |
28978-02-1 |
C29H34O15 |
15 |
37 |
Isorhoifolin |
Aerial |
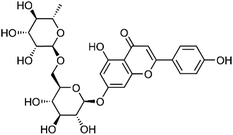 |
552-57-8 |
C27H30O14 |
7 |
38 |
Vicenin-2 |
Whole plant |
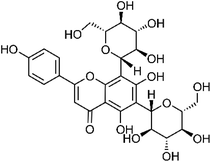 |
23666-13-9 |
C27H30O15 |
3 |
39 |
Vitexin |
Leaves |
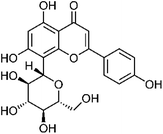 |
3681-93-4 |
C21H20O10 |
3 |
40 |
Luteolin |
Whole plant |
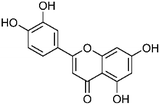 |
491-70-3 |
C15H10O6 |
15 |
41 |
Scutellarein |
Whole plant |
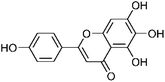 |
529-53-3 |
C15H10O6 |
15 |
42 |
Hispidulin |
Aerial |
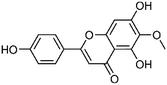 |
1447-88-7 |
C16H12O6 |
15 |
43 |
Apigenin-8-C-α-L-arabinopyranoside |
Aerial |
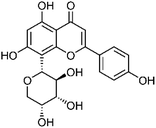 |
38642-55-6 |
C20H18O9 |
15 |
44 |
Apigenin-7-O-glucuronide |
Leaves |
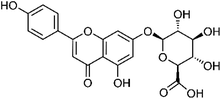 |
29741-09-1 |
C21H18O11 |
16 |
45 |
Hispidulin-7-O-glucuronide |
Leaves |
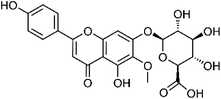 |
31105-76-7 |
C22H20O12 |
16 |
46 |
Cirsimaritin |
Leaves |
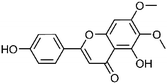 |
6601-62-3 |
C17H14O6 |
16 |
47 |
Cirsiliol |
Leaves |
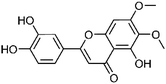 |
34334-69-5 |
C17H14O7 |
16 |
48 |
Salvigenin |
Leaves |
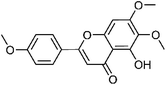 |
19103-54-9 |
C18H16O6 |
16 |
49 |
Scutellarein 7-O-α-glucuronamide |
Aerial |
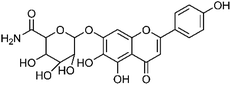 |
1402088-78-1 |
C21H19NO11 |
8 |
Flavonols
Flavonols are the second most abundant flavonoids in S. dulcis. Three flavonols, morin, dihydroxy-dimethoxyflavone, and hydroxy-tetramethoxyflavone, were identified by HPLC-MS/MS analysis of methanol extract from leaves of S. dulcis.16 Dillenetin 3-O-(6′′-O-p-coumaroyl)-β-D-glucopyranoside was isolated from the 70% aqueous acetone extract of the dry aerial part of S. dulcis. Experiments showed that this compound had low agonistic activity on PPAR-γ. The 70% ethanol extract of S. dulcis showed the presence of rutin, quercetin, and kaempferol. It has been found that kaempferol can alleviate hyperglycemia by inhibiting liver gluconeogenesis, improving liver sensitivity to insulin, and inhibiting inflammatory response and oxidative stress.17,18 Rutin and quercetin are also known to have antihyperglycemic effects. The glucose lowering mechanisms may include reducing the absorption of carbohydrates in the small intestine, inhibiting tissue gluconeogenesis, increasing tissue uptake and utilization of glucose, stimulating beta cells to secrete insulin, and protecting Langerhans islets from degeneration.19,20 The chemical structures of flavonols compounds are shown in Table 3.
Table 3 The chemical structures of flavonols compounds, extracted from various part of S. dulcis
No. |
Compound |
Part of plant |
Chemical structure |
CAS number |
Chemical formula |
Ref. |
50 |
Morin |
Leaves |
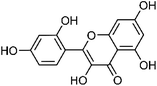 |
480-16-0 |
C15H10O7 |
16 |
51 |
Dihydroxy-dimethoxyflavone |
Leaves |
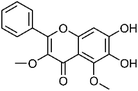 |
PubChem CID: 123885531 |
C17H14O6 |
16 |
52 |
Hydroxy-tetramethoxyflavone |
Leaves |
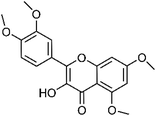 |
1244-78-6 |
C19H18O7 |
16 |
53 |
Dillenetin 3-O-(6′′-O-p-coumaroyl)-β-D-glucopyranoside |
Aerial |
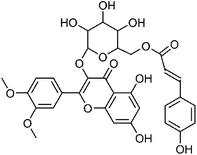 |
1613545-05-3 |
C32H30O14 |
15 |
54 |
Rutin |
Whole plant |
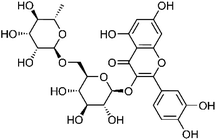 |
153-18-4 |
C27H30O16 |
40 |
55 |
Quercetin |
Leaves |
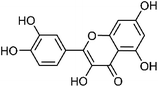 |
117-39-5 |
C15H10O7 |
45 |
56 |
Kaempferol |
Whole plant |
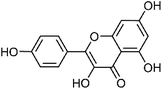 |
520-18-3 |
C15H10O6 |
40 |
Other flavonoids
Catechin and naringin were present in the 70% aqueous ethanol extract from aerial parts of S. dulcis. They belong to flavan-3-ols and isoflavones respectively. Both catechin and naringin have antihyperglycemic and antihyperlipidemic effects. They are both antioxidants, and naringin seems to alleviate metabolic syndrome by preventing oxidative damage and pro-inflammatory cytokine release.21,22 The chemical structures of other flavonoids compounds are shown in Table 4.
Table 4 The chemical structures of other flavonoids compounds, extracted from various part of S. dulcis
No. |
Compound |
Part of plant |
Chemical structure |
CAS number |
Chemical formula |
Ref. |
57 |
Catechin |
Whole plant |
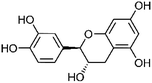 |
7295-85-4 |
C15H14O6 |
40 |
58 |
Naringin |
Whole plant |
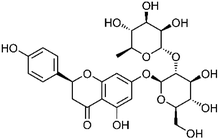 |
10236-47-2 |
C27H32O14 |
40 |
Diterpenoids
Diterpenoids refer to a group of compounds whose molecular skeleton consists of 4 isoprene units and contains 20 carbon atoms. Giang et al.23 isolated scopadulcic acid A, scopadulcic acid B, scopadulciol, 4-epi-scopadulcic acid B, iso-dulcinol, scopadulcic acid C, and dulcidiol from the aerial parts of S. dulcis. In MTT (3-[4,5-dimethylthiazol-2-yl]-2,5-diphenyl-tetrazolium bromide) cytotoxic assay, 4-epi-scopadulcic acid B, iso-dulcinol, and scopadulcic acid C exhibited cytotoxic activity against KB cells with IC50 values ranging from 2–50 μg mL−1. Scopadulcic acid B and scopadulciol were also reported to exhibit gastric proton pump inhibitory activity, specifically scopadulcic acid B was a reversible inhibitor.24 Dulcinodal, dulcinodiol, and scopadiol decanoate were isolated from petroleum ether extract of aerial parts of S. dulcis. They can be classified as labdane-derived diterpenes. 4-epi-7α-O-Acetylscoparic acid A, 7α-hydroxyscopadiol, (7S)-4-epi-7-hydroxyscoparic acid A, 7α-O-acetyl-8,17β-epoxyscoparic acid A, neo-dulcinol, dulcinodal-13-one, and 4-epi-7α-hydroxydulcinodal-13-one were determined by Liu et al.15 from the 70% aqueous acetone extract of the dry aerial part of S. dulcis by analysing their NOESY spectra. The inhibitory effect of 4-epi-scopadulcic acid B on α-glucosidase (IC50: 14.6 ± 1.5 μM) is more potent than that of acarbose in the positive control group (IC50: 3760 ± 157.2 μM). Scoparic acid A, scoparic acid B, and scoparic acid C were isolated from the whole plant of S. dulcis, and their cytotoxic activity was verified by Hayashi et al.13 Scoparic acid E was isolated from the CH2Cl2 extract of the dried whole plant of S. dulcis by Zhang et al.25 Sun et al.26 isolated scoparicol A and scoparicol B from the aerial parts of S. dulcis and various spectroscopic techniques were used to determine their chemical structures. It is worth mentioning that scoparic acid E, scoparicol A, and scoparicol B were all tested for their ability to attenuate plamitate-induced viability at 25 and 50 μM. The result showed that they could all attenuate palmitate-induced viability in MIN6 cells.25,26 Scoparic acid D, isolated from S. dulcis, was compared with miglitol and voglibose, and found to have similar α-glucosidase enzyme inhibitory activity.27 Hayashi et al.28 isolated scopadulin from the CHCl3-soluble part of S. dulcis. It is a tetracyclic diterpene and has antiviral activity. Scopanolal and scopadiol were isolated from the petroleum ether extract of aerial parts of S. dulcis by Ahsan et al.29 The two compounds have been confirmed to have anti-gastric cancer activity in vitro. In addition, phytol, which is commonly found in plants, also has anti-inflammatory, anti-oxidative, antidiabetic, and antihyperlipidemic effects.30 The chemical structures of diterpenoids compounds are shown in Table 5.
Table 5 The chemical structures of diterpenoids compounds, extracted from various part of S. dulcis
No. |
Compound |
Part of plant |
Chemical structure |
CAS number |
Chemical formula |
Ref. |
59 |
Scopadulcic acid A |
Aerial |
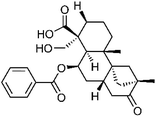 |
114804-64-7 |
C27H34O6 |
23 |
60 |
4-epi-Scopadulcic acid B |
Aerial |
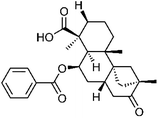 |
577992-08-6 |
C27H34O5 |
23 |
61 |
Scopadulcic acid B |
Aerial |
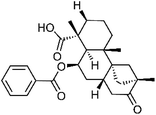 |
114804-65-8 |
C27H34O5 |
23 |
62 |
Scopadulciol |
Aerial |
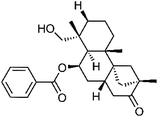 |
136565-26-9 |
C27H36O4 |
23 |
63 |
iso-Dulcinol |
Aerial |
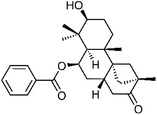 |
578714-90-6 |
C27H36O4 |
23 |
64 |
Scopadulcic acid C |
Aerial |
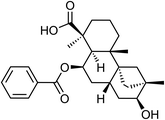 |
|
C27H34O5 |
23 |
65 |
Dulcidiol |
Aerial |
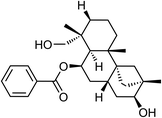 |
578714-91-7 |
C27H38O4 |
23 |
66 |
Dulcinodal |
Aerial |
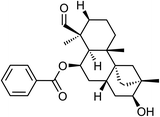 |
1443044-14-1 |
C27H36O4 |
29 |
67 |
Dulcinodiol |
Aerial |
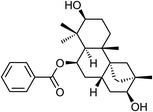 |
1443044-15-2 |
C27H38O4 |
29 |
68 |
Scopadiol decanoate |
Aerial |
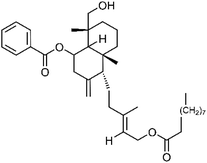 |
1443044-16-3 |
C37H56O5 |
29 |
69 |
4-epi-7α-O-Acetylscoparic acid A |
Aerial |
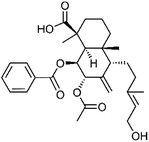 |
1613544-96-9 |
C29H38O7 |
15 |
70 |
7α-Hydroxyscopadiol |
Aerial |
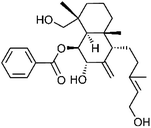 |
1613544-98-1 |
C27H38O5 |
15 |
71 |
(7S)-4-epi-7-Hydroxyscoparic acid A |
Aerial |
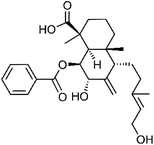 |
1613545-04-2 |
C27H36O6 |
15 |
72 |
7α-O-Acetyl-8,17β-epoxyscoparic acid A |
Aerial |
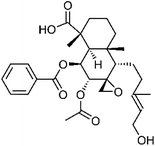 |
1613545-00-8 |
C29H38O8 |
15 |
73 |
neo-Dulcinol |
Aerial |
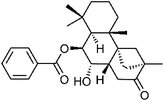 |
1613692-96-8 |
C27H36O4 |
15 |
74 |
Dulcinodal-13-one |
Aerial |
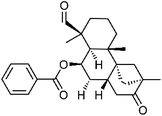 |
1613545-02-0 |
C27H34O4 |
15 |
75 |
4-epi-7α-Hydroxydulcinodal-13-one |
Aerial |
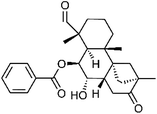 |
1613545-03-1 |
C27H34O5 |
15 |
76 |
Scoparic acid A |
Aerial |
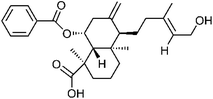 |
116425-30-0 |
C27H36O5 |
13 |
77 |
Scoparic acid B |
Aerial |
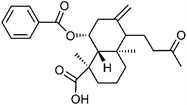 |
116425-29-7 |
C25H32O5 |
13 |
78 |
Scoparic acid C |
Whole plant |
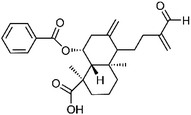 |
116425-28-6 |
C26H32O5 |
13 |
79 |
Scoparic acid E |
Whole plant |
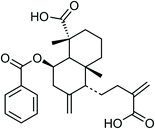 |
|
C26H32O6 |
25 |
80 |
Scoparicol A |
Aerial |
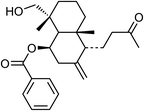 |
|
C25H34O4 |
26 |
81 |
Scoparicol B |
Aerial |
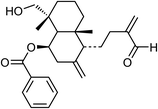 |
|
C26H34O4 |
26 |
82 |
Scoparic acid D |
Whole plant |
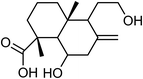 |
1256659-95-6 |
C16H26O4 |
27 |
83 |
Scopadulin |
Whole plant |
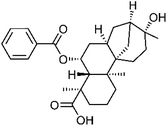 |
129058-58-8 |
C27H36O5 |
28 |
84 |
Scopanolal |
Aerial |
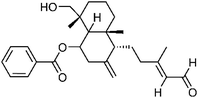 |
578714-92-8 |
C27H36O4 |
29 |
85 |
Scopadiol |
Whole plant |
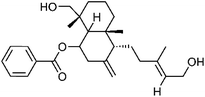 |
130838-00-5 |
C27H38O4 |
29 |
86 |
Phytol |
Leaves |
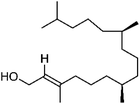 |
150-86-7 |
C20H40O |
49 |
Triterpenoids
Most triterpenoids are considered to be formed by the condensation of 6 isoprene, so skeleton of most triterpenoids contain 30 carbon atoms. 6 triterpenoids, friedelin, glutinol, α-amyrin, dulcioic acid, betulinic acid, and ifflaionic acid were identified from petrol extract of S. dulcis by Mahato et al.31 Glutinol was confirmed to promote insulin secretion.32 Betulinic acid has been found to have antidiabetic, anti-inflammatory, antihyperlipidemic, anti-viral, and anti-tumor activities, which provides a pharmacological basis for its use in the treatment of metabolic syndrome.33 Lupeol and glutenol were reported by Wan et al.34 Glutinone was isolated from the methanol extract of S. dulcis by Sharma Khaga et al.32 Glutinone can exert anti-inflammatory activity by inhibiting cyclooxygenase-1 (COX-1).35 Taraxerol was reported by Babincova et al.7 It exerts anti-inflammatory activity by inhibiting the NF-κB singling pathway.36 Taraxerol also has antidiabetic activity and was found to attenuate diabetic neuropathy in rats with type 2 diabetes.37 The chemical structures of triterpenoids compounds are shown in Table 6.
Table 6 The chemical structures of triterpenoids compounds, extracted from various part of S. dulcis
No. |
Compound |
Part of plant |
Chemical structure |
CAS number |
Chemical formula |
Ref. |
87 |
Friedelin |
Whole plant |
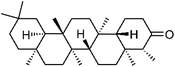 |
559-74-0 |
C30H50O |
31 |
88 |
Glutinol |
Whole plant |
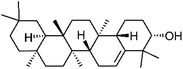 |
545-24-4 |
C30H50O |
31 |
89 |
α-Amyrin |
Whole plant |
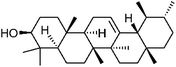 |
638-95-9 |
C30H50O |
31 |
90 |
Dulcioic acid |
Whole plant |
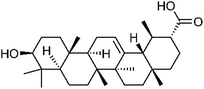 |
78516-69-5 |
C30H48O3 |
31 |
91 |
Betulinic acid |
Roots |
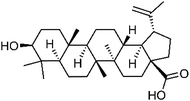 |
472-15-1 |
C30H48O3 |
31 |
92 |
Lupeol |
Whole plant |
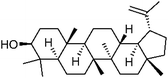 |
545-47-1 |
C30H50O |
34 |
93 |
Ifflaionic acid |
Whole plant |
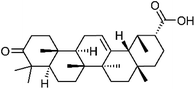 |
6805-19-2 |
C30H46O3 |
31 |
94 |
Glutenol |
Whole plant |
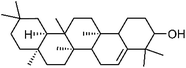 |
137397-38-7 |
C30H50O |
34 |
95 |
Glutinone |
Whole plant |
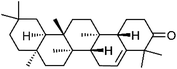 |
508-09-8 |
C30H48O |
32 |
96 |
Taraxerol |
Whole plant |
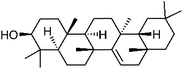 |
127-22-0 |
C30H50O |
7 |
Steroids
So far, there are still relatively few studies on steroids in S. dulcis. Steroids may be involved in the metabolism of calcium and phosphorus in the human body. Babincova et al.7 reported three steroids found in S. dulcis. They are daucosterol, stigmasterol, and β-sitosterol. It has been reported that stigmasterol has anti-inflammatory and antioxidative effects and can improve the tissue damage caused by the inflammatory response induced by ischemia-reperfusion injury.38 Daucosterol can also improve cerebral ischemia-reperfusion injury and protect neurons.25 β-Sitosterol has many pharmacological properties, including anti-inflammatory, antidiabetic, and antihyperlipidemic. Experiments showed that the compound is a promising supplement for the treatment of metabolic syndrome.39 The chemical structures of steroids compounds are shown in Table 7.
Table 7 The chemical structures of Steroids compounds, extracted from various part of S. dulcis
No. |
Compound |
Part of plant |
Chemical structure |
CAS number |
Chemical formula |
Ref. |
97 |
Daucosterol |
Whole plant |
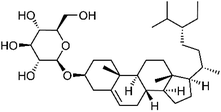 |
474-58-8 |
C35H60O6 |
7 |
98 |
Stigmasterol |
Whole plant |
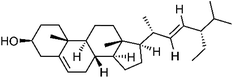 |
83-48-7 |
C29H48O |
7 |
99 |
β-Sitosterol |
Whole plant |
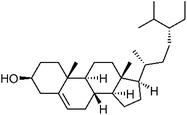 |
83-46-5 |
C29H50O |
7 |
Phenolics
Phenolic compounds widely exist in plants and have aromatic odor. The phenolic compounds sorted out below do not include flavonoids. Datta et al.40 determined chlorogenic acid, caffeic acid, ferulic acid, and sinapic acid from the 70% ethanol extract of S. dulcis. Chlorogenic acid can improve blood glucose and lipid levels by regulating gene expression. Additionally, it can lower blood pressure, and has many pharmacological activities, including liver protection, anti-inflammation, and cardiovascular protection.41 A study showed that the combination of caffeic acid and ferulic acid in the treatment of mice with metabolic syndrome resulted in significant reductions in their blood sugar, cholesterol, and triglycerides levels, and improvement in liver cell steatosis by increasing liver intake of cholesterol and reducing triglyceride synthesis.42 Sinapic acid has also been confirmed to have antidiabetic, hepatoprotective, anti-inflammatory, antioxidative, and cardioprotective effects.43 Forsythoside G were determined by HPLC-MS/MS analysis of methanol extract from leaves of S. dulcis.16 Acteoside was isolated from the water extract of the dried aerial parts of S. dulcis by Wu et al.8 and was confirmed to exert anti-osteoarthritic activity via the JAK/STAT signaling pathway.44 Tin et al.10 investigated the methanol fraction of whole plants of S. dulcis and isolated ferruginoside C. p-Coumaric acid was reported by Beh et al.45 to have the ability to reverse diabetic nephropathy in their experiments.46 Gentisic acid reported by Babincova et al.7 also has anti-inflammatory, hepatoprotective, antioxidative, and neuro-protective activities.47 The chemical structures of phenolic compounds are shown in Table 8.
Table 8 The chemical structures of phenolic compounds, extracted from various part of S. dulcis
No. |
Compound |
Part of plant |
Chemical structure |
CAS number |
Chemical formula |
Ref. |
100 |
Chlorogenic acid |
Whole plant |
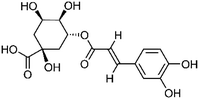 |
327-97-9 |
C16H18O9 |
40 |
101 |
Caffeic acid |
Whole plant |
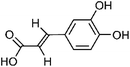 |
331-39-5 |
C9H8O4 |
40 |
102 |
Ferulic acid |
Whole plant |
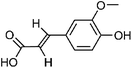 |
1135-24-6 |
C10H10O4 |
40 |
103 |
Sinapic acid |
Whole plant |
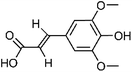 |
530-59-6 |
C11H12O5 |
40 |
104 |
p-Coumaric acid |
Leaves |
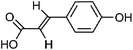 |
7400-08-0 |
C9H8O3 |
45 |
105 |
Forsythoside G |
Leaves |
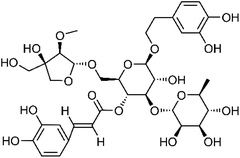 |
129802-19-3 |
C35H46O19 |
16 |
106 |
Acteoside |
Aerial |
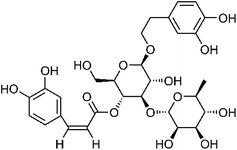 |
61276-17-3 |
C29H36O15 |
8 |
107 |
Ferruginoside C |
Aerial |
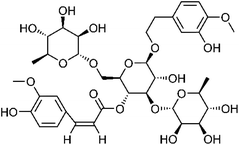 |
213991-03-8 |
C37H50O19 |
10 |
108 |
Gentisic acid |
Whole plant |
 |
490-79-9 |
C7H6O4 |
7 |
Other aliphatics
It is reported that mannitol is found in S. dulcis.7 This compound has osmotic diuretic effect, but its oral absorption is low. Hexalure, 2-hexyldecanoic acid, 5Z-eicosenoic acid, and methyl arachidate were identified in the aqueous extract of S. dulcis by Wankhar et al.9 Wangsa et al.48 also found that stearic acid and methyl stearate in S. dulcis have estrogen agonistic effects by using molecular docking technology. The chemical structures of other aliphatics compounds are shown in Table 9.
Table 9 The chemical structures of other aliphatics compounds, extracted from various part of S. dulcis
No. |
Compound |
Part of plant |
Chemical structure |
CAS number |
Chemical formula |
Ref. |
109 |
Mannitol |
Whole plant |
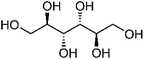 |
87-78-5 |
C6H14O6 |
7 |
110 |
Hexalure |
Leaves |
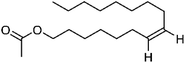 |
23192-42-9 |
C18H34O2 |
9 |
111 |
2-Hexyldecanoic acid |
Leaves |
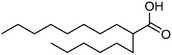 |
25354-97-6 |
C16H32O2 |
9 |
112 |
5Z-Eicosenoic acid |
Leaves |
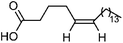 |
7050-07-9 |
C20H38O2 |
9 |
113 |
Methyl arachidate |
Leaves |
 |
1120-28-1 |
C21H42O2 |
9 |
114 |
Stearic acid |
Whole plant |
 |
57-11-4 |
C18H36O2 |
48 |
115 |
Methyl stearate |
Whole plant |
 |
112-61-8 |
C19H38O2 |
48 |
Pharmacological basis to treat metabolic syndrome
In recent years, researchers have studied the chemical components and pharmacological activities of the different extracts from S. dulcis and confirmed that S. dulcis provides various therapeutic effects related to the treatment of chronic conditions. These include antidiabetic, antihyperlipidemic, anti-atherosclerotic, anti-inflammatory, anti-arthritic, and hepatoprotective properties. This paper aims to investigate the pharmacological effects of S. dulcis as they pertain to the treatment of metabolic syndrome and its complications through a review of the existing literature.
Antidiabetic effect
The scoparic acid D (SAD) aqueous extract of S. dulcis showed significant antidiabetic activity by decreasing fasting blood glucose levels, increasing the plasma insulin levels and also stimulating insulin secretion from islets to increase plasma insulin levels on streptozotocin (STZ)-induced hyperglycemic rats. An in vitro study showed that 20 μg mL−1 SAD increased insulin secretion best compared to 16.7 mM glucose (positive control) and 40–80 μg SAD.50 The insulin-secretagogue activity of the aqueous extract of S. dulcis exhibited a significant stimulatory effect on insulin secretion from isolated Balb/c splenic pancreatic islets in vitro.51 The aqueous extract of S. dulcis (200 mg kg−1) showed effects on the polyol pathway and lipid peroxidation in the livers of STZ induced diabetic Wistar rats.52 SAD also exhibited binding affinity to the active site of human α-glucosidase enzyme, comparable to commercial drugs Miglitol and Voglibose.53 The diabetic rats treated with 200 mg kg−1 of the S. dulcis plant extract exhibited significant reduction in blood glucose level.54 Another 15 days experiment also showed a significant anti-hyperglycemic effect of the aqueous S. dulcis plant extract.50,55 When rats with STZ-induced diabetes were administered the S. dulcis plant extract, they exhibited a significant blood glucose reduction and an increase in plasma insulin as compared to rats administered with glibenclamide, the standard drug.56 Additionally, as compared to the standard drug tolbutamide, glutinol from S. dulcis caused a moderate insulin secretory activity increase, and coixol from S. dulcis showed a significant activity increase.57,58 The flavonoid extract of S. dulcis (400 mg kg−1) was found to decrease the serum glucose level to near normal in a glucose tolerance test and normoglycemic study.59 Coixol from S. dulcis exhibited significant antidiabetic activity by improving the glucose tolerance and fasting blood glucose levels in diabetic animals.57 The α-glucosidase inhibitors with flavonoids such as scutellarein, hispidulin, apigenin, luteolin, and the triterpenoid betulinic acid have been found in S. dulcis. 4-epi-scopadulcic acid B from S. dulcis exhibited significant inhibitory activity against α-glucosidase. Additionally, an in vitro study of 4-epi-7α-O-acetylscoparic acid A, scutellarein, hispidulin, apigenin, luteolin, and acerosin from S. dulcis showed PPAR-γ agonistic activity.15,60 A flavonoid fraction, containing quercetin, p-coumaric acid, luteolin, and apigenin in the ratio of 8
:
26
:
1
:
3, extracted from S. dulcis by thin layer chromatography fraction-7 (SDF7), possesses significant glucose uptake activity by upregulating glucose transporter-4 (Glut 4) expression and trans-location. Additionally, it showed better glucose transport activity than insulin even in the insulin resistance model induced by free fatty acids. TC 10 was confirmed to promote Glut 4 translocation in immortalized rat skeletal (L6) myotubes by remodelling cellular actin and it can be induced by SDF7.61 SDF7 was capable of inducing the differentiation of 3T3-F442a adipocytes, stimulating the secretion of adiponectin, and upregulating peroxisome proliferator-activated receptor-γ (PPAR-γ mRNA) on the cells as well as other mechanisms that have already been elucidated.45 The range of antiglycation potential of S. dulcis decoction was 13–213 μg mL−1.62 Important experiments about the antidiabetic activities of different extracts from S. dulcis are listed in Table 10.
Table 10 The important experiments of antidiabetic activities of different extract/isolate from different parts of S. dulcisa
Extract/isolate |
Plant parts |
In vitro/in vivo |
Method |
Effects |
Control used |
Ref. |
PC, positive control; NC, negative control; b.w., body weight; SPEt, S. dulcis plant water extract. |
H2O |
Whole plant |
In vivo |
Blood glucose and plasma insulin determination |
The 200 mg kg−1 b.w. extract exhibited significant activity in decreasing the fasting blood glucose level to 98 ± 3 mg dL−1 compared to the glibenclamide of 114 ± 9 mg dL−1. The plasma insulin level was increased to about 11 μU mL−1 when treated with the extract as compared to glibenclamide of 9 μU mL−1 |
600 μg kg−1 glibenclamide (PC), STZ induced diabetic rats (NC), and normal group |
92 |
EtOH |
Whole plant |
In vivo |
Streptozotocin induced diabetic rats |
Scoparic acid D administered to STZ-induced hyperglycemic rats showed significant plasma insulin increasing activity at the dose of 20 mg kg−1 SAD |
Compared with the normal group |
93 |
MeOH |
Whole plant |
In vitro |
Islet isolation, MIN-6 cell culture and insulin secretion assay |
Coixol showed a significant insulin secretory activity (230.35 ± 11.12%) at the dosage of 200 μM, which was more than tolbutamide (212.01 ± 16.76%) |
16.7 mM glucose, tolbutamide (PC) |
58 |
H2O |
Whole plant |
In vivo |
Streptozotocin induced diabetic rats |
Exhibited significant antihyperglycemic effect (p < 0.01). At first glucose was administered orally and then SPEt was treated. After 120 min, the blood glucose level of rats was close to normal (98.8 ± 5.7 mg dL−1) with a dosage of 200 mg kg−1 SPEt |
Diabetic control (NC) and normal group |
51 |
H2O |
Whole plant |
In vitro |
Islet isolation and incubation |
Islets incubated with 10 μg mL−1 of SPEt increased insulin secretion to 7 μIU mL−1 compared with about 4 μIU mL−1 of PC and 1 μIU mL−1 of NC |
16.7 mM glucose (PC), and 5 mM STZ (NC) |
51 and 54 |
H2O |
Whole plant |
In vivo |
Oral glucose tolerance test |
All groups were treated with 2 g kg−1 mL−1 glucose in advance. Normal groups treated with SPEt showed almost the same blood glucose level compared to untreated groups after 120 min. Diabetic groups treated with 200 mg kg−1 SPEt showed significant antihyperglycemic effect (98.96 ± 5.70 mg dL−1) compared to the control groups (326.91 ± 4.40 mg dL−1) and 600 μg kg−1 glibenclamide (117.70 ± 6.76 mg dL−1) |
Glibenclamide (PC), normal and diabetic control groups |
55 |
Antihyperlipidemic effect
Hyperlipidemia is a well-known complication of diabetes. In diabetic rats treated with 200 mg kg−1 of aqueous extract of S. dulcis, their serum cholesterol, low-density lipoprotein (LDL), very low-density lipoprotein (VLDL), triglycerides, free fatty acids, and phospholipids were significantly reduced, while their high-density lipoprotein (HDL) levels were elevated. The activity of rat hepatic hydroxymethylglutaryl-CoA (HMG-CoA) reductase was also significantly reversed towards normalization after treating with an aqueous extracts of S. dulics.63 An aqueous extract of S. dulcis plant can increase serum high-density lipoprotein cholesterol, anti-atherogenic index, and HMG-CoA reductase activity, resulting in an antihyperlipidemic effect.63 The methanolic extract of S. dulcis can increase uptake of glucose at tissue level and increase pancreatic β-cell function or due to inhibition of intestinal glucose absorption of glucose, producing significant antihyperglycemic activity.59 Porridge with fresh S. dulcis leaf extract decreased fasting blood glucose (FBG) and glycated hemoglobin (HbA1c) of type 2 diabetic patients. This porridge had no effect on cholesterol measurements and no toxicity; therefore, this porridge is a suitable meal for diabetic patients.64
Anti-inflammatory effect
The anti-inflammatory activity of S. dulcis was confirmed on rat paw edema induced by carrageenan.65 Oral treatment with ethanol extract of the S. dulcis inhibited the carrageenan-induced edema.66 The anti-inflammatory property of S. dulcis was investigated on the model of carrageenin-induced pleurisy, suggesting that ethanol extract played a crucial role in inhibiting the production of exudate and leucocyte migration. Water extract of S. dulcis was endowed with anti-inflammatory potential in rat paw edema induced by carrageenan or dextran.65,66 The 70% ethanol extract of S. dulcis and betulinic acid, one of the triterpenoids extracted from S. dulcis, exhibited a significant anti-inflammatory effect by mitigating the development of λ-carrageenan induced edema.66
The anti-inflammatory activity of S. dulcis of both 70% ethanol extract of S. dulcis and betulinic acid not only significantly inhibited the cyclooxygenase-2 (COX-2) activity in the edema tissue, but also decreased the tissue concentration of nitric oxide (NO), tumor necrosis factor alpha (TNF-α), interleukin-1β (1L-1β), and malondialdehyde (MDA).67
In vitro experiments utilizing the lipopolysaccharide (LPS)-stimulated in RAW 264.7 macrophages,10 treatment with both ethanol and ethyl acetate extracts of its aerial part showed a significant nitric oxide (NO) production inhibitory potency at 30 and 100 μg mL−1, whilst 100 μg mL−1 hexane extract of its aerial part was observed to exert the same effect. Glutinone, an isolation from hexane fraction of methanol extract of S. dulcis, was observed to exert a moderate effect in inhibition of TNF-α and a weak effect in inhibiting IL-1β and NO production.32 Ethanol extract of S. dulcis also exerted its anti-inflammatory effect on ovalbumin-induced rat paw edema.68
As for components isolated from S. dulcis, glutinol and glutinone are two potential anti-inflammatory agents involved in the inflammation. It is noteworthy that both glutinol and ethanol extract only exert an active anti-inflammatory effect at the beginning of inflammatory process, approximately 2 hours after administration. This result suggests that glutinol and ethanolic extract of S. dulcis may not inhibit the activity of COX. It was observed that extract of S. dulcis did not interfere with prostaglandins that induced swelling and vasodilatation.66 Both indomethacin and ethanol extract barely affected the chronic inflammation models because they were stimulated by other agents which acted in a way different from that of nonsteroidal anti-inflammatory substances.
Anti-atherosclerotic effect
When the hyperglycaemia of diabetic patients makes blood vessels throughout the body fragile and easily injured, so that the walls of blood vessels become inflamed by damage, causing platelets to concentrate on the damaged areas to repair the condition, it will lead to “atherosclerosis” causing cardiovascular diseases. Atherosclerosis affects many organs of the body, leading to strokes, ischemic heart disease, and gangrene in the lower extremities. Dyslipidemia, hypertension, diabetes as well as genetic factors are the main risk factors of atherosclerosis. As for the dyslipidemia, it's clear that LDL accumulates in the endothelium after passing through the arterial wall, in which LDL is likely to convert into oxidized low-density lipoprotein (oxLDL). oxLDL is the key factor leading to atherosclerosis. oxLDL can stimulate inflammation signals and cause macrophages or smooth muscle cells to take up cholesterol to form foam cells. Going a step further, foam cells form fatty streaks that mark the condition of atherosclerosis.69 Therefore, detecting the antioxidant and anti-inflammatory activities of the extract of S. dulcis is helpful for the further study of its anti-atherosclerotic mechanism.
In vivo animal test results showed that an aqueous suspension in clean drinking water of pulverized S. dulcis reduced total cholesterol, trriacylglycerol, and LDL-cholesterol and HDL-cholesterol was also significantly reduced.70 The foliar methanol extract of S. dulcis inhibited LDL and its oxidation to oxLDL to prevent foam cell formation, provided with strong anti-atherogenic potential.71,72
Hepatoprotective effect
Metabolic diseases are prone to the non-alcoholic fatty liver disease (NAFLD). S. dulcis has been used to treat this disease.34,73 Therefore, some research achievements verified this herbal hepatoprotective activity by using various extracted ingredients of the herb, such as whole plants, roots,and foliar, using a variety of organic solvents. The ethanol extract of S. dulcis increased serum alanine aminotransferase (ALT) and aspartate amino-transferase (AST) and elevated the content of reduced glutathione (GSH), while decreasing the malondialdehyde (MDA) level. The hepatoprotective effect was achieved by preventing descending the antioxidative enzymes of superoxide dismutase (SOD), glutathione peroxidase (GPx), glutathione reductase (GRd), and glutathione-S-transferase (GST) to decrease the damage of lipid peroxidation.74 The hepatoprotective effect of herbal extracts, decreasing the increased ALT, AST, bilirubin, and cholesterol, was compared with that of suitable silymarin to verify its hepatoprotective effect.75,76 The other in vivo test also displayed that the herbal extracts decrease the levels of ALT and AST and increase the level of albumin in carbon tetrachloride (CCl4)-induced liver injury in mice. Less vacuole form, neutrophil infiltration, and hepatocyte necrosis were also present in histopathological analysis in CCl4-induced liver injury in mice.77 Histological and serum biochemistry analysis are necessary as parameters to reflect the improvement of liver function status, containing GSH, MDA and so on.77 Data comparison confirms the antihepatotoxic activity and hepato-curative activity of S. dulcis extract.
Anti-oxidative effect
Oxidative stress is the core pathogenesis of diabetes complications, due to excessive oxygen ions. There are four substances for the removal of free radicals, including SOD, methionine sulfoxide reductase (MSR), catalase (CAT), and GPx.
The aqueous extract of S. dulcis showed significant activity in reducing the plasma lipid peroxidation. The parameters of 2-thiobarbituric acid reacting substances test and thiobarbituric acid reactive substances (TBARS) assay, hydroperoxides, and ceruloplasmin were reduced and the activities of plasma insulin, superoxide dismutase, catalase, glutathione peroxidase, glutathione-S-transferase, and reduced glutathione were increased.50 These extracts can significantly reduce the degree of lipid peroxidation in STZ induced diabetic rats and also found that the values and effects of CAT, SOD, GPx, and GSH were slightly better than those of gelibenclamide.50 Otherwise, these extracts significantly reduced the lipid peroxidation and intracellular reactive oxygen species (ROS) level.51 The activities of both CAT and MDA were increased by cadmium while the SOD activity was attenuated in the in vivo test of rats' liver, kidney, and heart. Coadministration of aqueous extract of S. dulcis at dose of 1000 mg kg−1 and CdCl2 showed restored activities of both SOD and CAT.78
The presence of phenol, flavonoids, and total antioxidants in the methanol and aqueous extract of S. duicis displayed that the methanol extract method has effective extraction solvents, which provided higher reduction content than that of aqueous solvents.9
The antioxidant activity of 70% ethanol extract of S. dulcis was also assessed in the in vivo antioxidant enzymes assay and found that the levels of antioxidant enzyme (SOD, GPx, and GRd) activities were significantly increased.67 The aqueous leaf extract of S. dulcis for treatment of brain in the noise environment showed that the levels of H2O2, NO, and lipid peroxide in the brain of rats induced by noise could be significantly reduced and protein thiols could be increased. Immunohistochemical study of brain tissue showed that these extracts could down-regulate the expression of neuronal nitric oxide synthases (nNOS) and calcium-insensitive NOS (iNOS) in rat brain. The levels of SOD, CAT, GPX, and GST in the brain of rats treated with the extract were significantly decreased, while the levels of GR, GSH, vitamin C, and vitamin E were significantly increased. In addition, histopathological study showed that the extract of S. dulcis could reduce the changes of cerebral cortex caused by noise.79
Besides, the aqueous extract of S. duicis had the excellent antioxidant and dose-dependent antioxidant index. Both methanol and aqueous extract were provided with the potential free radical scavenging activity at 2,2-diphenyl-1-pyridyl hydrazine (DPPH) model.80 The anti-oxidant activity of various extract method were compared by their inhibition of xanthine oxidase and lipoxygenase. The hexane, methanol and chloroform extracts of the whole plant produced weak to moderate activity in inhibition of xanthine oxidase and exhibited significant inhibitory activity in lipoxygenase inhibition. All extracts (hexane, chloroform, and methanol) of S. dulcis exhibited more than 50% inhibition on lipid peroxidation. Additionally, the results indicated that methanol extract of S. dulcis performed better in the inhibition of lipid peroxidation, compared with ascorbic acid extract.81 The hydroalcoholic extract of S. dulcis also exhibited significant antioxidant activity in NO radical and DPPH free radical scavenging activity test.82 The methanol extract of S. dulcis can significantly reduce H2O2-induced oxidative stress in Sf9 cells.83 There are some important experiments about the anti-oxidant and antiatherogenic activities of different extract/isolate from different parts of S. dulcis, listed in Table 11.
Table 11 The important anti-oxidant and anti-atherogenic activities of different extract/isolate from different parts of S. dulcisa
Extract/isolate |
Plant parts |
In vitro/in vivo |
Method |
Effects |
Control used |
Ref. |
PC, positive control; NC, negative control; BHA, butyl hydroxyl anisole. |
EtOH |
Whole plant |
In vitro |
TBARS analysis |
The increased MDA was restored to normal level by 200 mg kg−1 silymarin and 0.5 g kg−1 EtOH extract. Besides, the extract at the dose of 1.0 mg kg−1 could continuously decrease the level of MDA |
Silymarin (PC), control group, and CCl4 group (NC) |
74 |
70% aqueous ethanol and MeOH |
Whole plant |
In vitro |
Thiocyanate method and spectrophotometry |
The percentage of preventing lipid peroxidation was 42.006 ± 0.797% using 70% aqueous ethanol as extractant and 23.836 ± 0.273% using methanol as extractant |
Groups without the extract (NC) |
40 |
MeOH |
Aerial |
In vitro |
MTT survival assay |
The extract increased viability of Sf9 cells exposed to 750 μM and 1 mM H2O2 |
750 μM and 1 mM H2O2 (NC) and normal group |
83 |
70% EtOH/betulinic acid |
Whole plant |
In vivo |
SOD, GPx, and GRd assay |
Ethanolic extracts (0.5 and 1 g kg−1), betulinic acid (40 mg kg−1) and indomethacin (20 mg kg−1) significantly increased the levels of SOD, GPx, and GRd activities |
Indomethacin (PC) |
67 |
Hexane, CCl3, and MeOH |
Whole plant |
In vitro |
Liver lipid peroxidation assay |
All extracts showed significant inhibition effect on lipid peroxidation. Methanol extract showed the highest percentage of inhibition on lipid peroxidation among tested extracts and positive control |
Ascorbic acid (PC) |
81 |
MeOH |
Leaves |
In vitro |
Spectrophotometry |
The methanol extract had the most efficient lipid peroxidation inhibition activity. Besides, the IC50 value of methanol extract was slightly higher than the BHA |
BHA (PC) |
71 |
MeOH, EtOAc, acetone, and hexane |
Leaves |
In vitro |
Oil red O stain |
There was evident reduction of oxLDL-treated cells or no foam cells after adding 30 μg mL−1 methanol extract |
Cells treated with oxidized LDL (NC) |
71 |
Hyper/hypotensive effect
The aqueous fraction of ethanol extracts of S. dulcis can contract the muscle of vas deferens of rats similar to norepinephrine dose dependently. The left atria incubated with the aqueous fraction of the S. dulcis extract showed enhanced contractile force. Histamine induced tension of tracheal rings could be inhibited by aqueous fraction of the extract and epinephrine.66 The polar extract of the aerial parts of S. dulcis, containing noradrenaline and adrenaline substances, displayed the hypertensive effect in vivo, relaxing the tracheal smooth musculature and increasing inotropism in heart muscle.11 Nevertheless, the experimental results of Esume et al. 201188 showed that higher dose of the water and methanol extracts of S. dulcis significantly increased blood pressure from baseline values of animal tests. Although S. dulcis plant has been widely used in the treatment of hypertension, the effect of treating hypertension needs more scientific research to demonstrate its therapeutic effect.
Clinical studies
Clinical study is the third stage of drugs development at the aim of providing basis for administration methods, evaluating the safety, measuring the efficacy, and further test. A randomized crossover clinical trial was carried out by Subhashinie et al.64 to show the antihyperglycemic effects of herbal porridge with S. dulcis leaves extract in diabetics in Sri Lanka by confirming the antihyperglycemic effects of herbal porridge made of S. dulcis leaves extract in diabetics in Sri Lank. The total porridge used for treatment is 40 g, and the porridge contains about 13–15 g of fresh S. dulcis leaves. As a result, this porridge has the characteristic of decreasing the Fasting Blood Glucose and HbA1c with no effect on cholesterol measurements. At the dose test, no toxicity was observed. Consequently, the porridge made with S. dulcis leaves extract can be widely used in commercial products and sold to type 2 diabetic patients.
35 mild to moderate type 2 diabetes patients complete more than six months of trial. Their age ranged between 35–70 years old. The fasting blood glucose is between 127 mg dL−1 and 302 mg dL−1. The extruded meals and porridge were prepared with tender leaves extract of S. dulcis. 35 participations are divided into two groups taking different meals at the same stage. The clinical trial period consists of three phases. At the first stage, group 1 as the test group eat specific porridge once a day, 3 days per week, for three months; group 2 as the control group eat normal breakfast without S. dulcis leaves extract maintaining the same time period and the same frequency. The second period is a wash-out period to prepare for the next crossover trial, lasting for a week. At the last period, group 1 as the control group eat normal breakfast once a day, 3 days per week, for three months; group 2 as the test group eat this porridge.
At the beginning and end of the first and third stages, experimenter measure the following data of 35 participants: glucose measurements (including fasting blood glucose and HbA1c), lipid measurements (including total cholesterol, HDL-C, LDL-C, triglycerides, and cholesterol ratios), and the toxicity paraments (including liver enzymes, creatinine, CRP, and eGFR). Fasting blood glucose at the test trial are all lower than the measurement results at the control trial. Comparing the test results before and after first and third stages, HbA1c shows a downward trend in the test group instead of upward trend in the control group. Lipid measurements showed slight but irregular changes between test and control group. The result of toxicity parameters is normal with no significant difference within or between two groups in first and third stages.
In summary, herbal porridge made of S. dulcis leaves extract has antihyperglycemic effect with little to no effect on the cholesterol and toxicity measurements. This randomized crossover clinical trial reveals the feasibility of commercial production of this porridge for type 2 diabetic patients.
Toxicological studies
The toxicity of coixol was evaluated in the in vitro MTT assay and showed nontoxic in both MIN-6 and 3T3 cell lines (IC50 > 200 μM). Acute and subacute toxicity evaluated in mice showed no significant changes in serum creatine, ALT, and AST, no other abnormal manifestation and no death at 100 mg kg−1 body weight, suggesting that coixol was nontoxic in kidney and liver.32 Coixol administered intravenously showed no toxic effects.64 Mice treated with 20, 100, and 500 mg kg−1 of coixol also exhibited no toxic manifestations.32 Ethanolic extract of whole plants of S. dulcis (EESD) exhibited no toxic effect after the observation period of 72 h when administered orally at doses of 1000, 2000, and 3000 mg kg−1.84 The aqueous extract of S. dulcis at a dosage of 8 g kg−1 administered orally exhibited no mortality, no changes in posture, motor activity, and behavior in rats compared to the control group. The 30 days' administration of the extract did not show any gross poisoning symptoms or deaths, but histopathological examination showed mild portal, vascular, stroma, and interstitial congestion in liver, heart, testis, and lung respectively.85
An acute toxicity study showed that administration of both aqueous and methanol extract of S. dulcis at doses up to 5 g kg−1 in rats produce no mortality,86 morphological, and behavioral toxic effects. A 28 days sub-chronic toxicity study using aqueous and methanol extracts of S. dulcis administration in rats (500, 1000, and 1500 mg kg−1) exhibited no obvious difference (p > 0.05) in hematological parameters such as red blood cells (RBC), hemoglobin (HGB), hematocrit (HCT), mean corpuscular volume (MCV), mean cell hemoglobin (MCH), mean corpuscular hemoglobin concentration (MCHC), and platelets (PLT) when compared with the control group treated with distilled water (1 mL kg−1) only. At the same time, the liver toxicity test of aqueous and methanol leaf extracts were also carried out and some biochemical parameters were measured. The methanol extract (500, 1000, and 1500 mg kg−1) treated rats showed no significant difference (p > 0.05) of ALT and AST level compared to the normal groups. The aqueous extract at doses of 500 and 1000 mg kg−1 treated rats exhibited a slight increase in AST. However, the 1500 mg kg−1 aqueous extract treated rats showed no significant difference of ALT and AST level. The aqueous and methanol extract of S. dulcis at doses of 500, 1000, and 1500 mg kg−1 exhibited no significant difference (p > 0.05) in parameters of total protein (TP), alkaline phosphatase (ALP), and serum albumin (ALB). The kidney toxicity of S. dulcis was also tested by measuring the parameters of urea and creatinine in urine. Data showed that the aqueous extract of S. dulcis administered orally at doses of 500, 1000, and 1500 showed no significant difference of urea and creatinine level compared to the control group. But when treated with 1000 mg kg−1 of methanol extract, the urea level significantly increased to 118.98 ± 8.20 μmol L−1 compared to the normal group of 53.45 ± 13.93 μmol L−1. The methanol treated groups at other doses exhibited no significant difference compared to the control group. Some tissue injuries were observed in histopathological studies. The kidney section of rats treated with 500 mg kg−1 of aqueous and methanol leaf extracts showed slight lymphocyte hyperplasia and tubular necrosis; the 1000 mg kg−1 treated groups showed moderate lymphocyte hyperplasia and mild tubular adhesion; 1500 mg kg−1 treated groups showed mild lymphocyte hyperplasia. The liver section of rats treated with 500 mg kg−1 of aqueous leaf extracts showed mild necrosis and cytoplasmic vacuolation; 1000 mg kg−1 treated groups showed moderate Kupfer cell hyperplasia and hepatocellular necrosis; 1500 mg kg−1 treated groups exhibited normal features, but showed mild hepatocellular necrosis in the methanol extract treated groups.87
The rats treated with 0.01–2 g kg−1 ethanolic-aqueous extracts orally exhibited no behavioral change and toxicological signs. When the extract at doses higher than 0.1 g kg−1 administered to rats intraperitoneally, significant piloerection and spontaneous motor activity were observed. After that, the animals died six hours later.11 Rats injected intraperitoneally with 100 mg kg−1 and 200 mg kg−1 of water and methanol extract did not die. The LD50 values of aqueous and methanol extract of S. dulcis administered intraperitoneally using arithmetic method of karber were measured, which were 535 mg kg−1 and 390 mg kg−1 respectively, indicating the methanol extract was more toxic.88
Discussion and conclusion
A considerable number of chemical constituents were isolated and identified from S. dulcis and evaluated by their different pharmacological activities. However, there is ample scope to explore many more bioactive compounds based on this plant's varied geographical locations. Further studying their biological properties and mechanism of action will greatly benefit the development of new therapeutic agents.
Research has shown that α-glucosidase inhibitors (AGIs), extracted from S. dulcis, demonstrated definitive benefits on glycemic control and postload insulin levels for patients with type 2 diabetes.89 Flavonoids, terpenoids, and fatty acids extracted from S. dulcis can increase insulin sensitivity in patients with diabetes. Peroxisome proliferator-activated receptors (PPARs) agonists found in S. dulcis also have reproducible renoprotective effects on diabetic kidney disease through PPAR-independent pathways.90 Furthermore, the aqueous extract of S. dulcis (SAD) showed significant antidiabetic activity in decreasing fasting blood glucose level, increasing the plasma insulin level, and stimulating insulin secretion. SAD also exhibited fine affinity to the active site of human α-glucosidase enzyme, comparable to commercial drugs Miglitol and Voglibose. Glutinol from S. dulcis showed moderate insulin secretory activity while coixol showed significant activity as compared to the standard drug tolbutamide. The flavonoid extract of S. dulcis decreased the serum glucose level to near normal in glucose tolerance test and normoglycemic study, demonstrating antihyperglycemic activity. Coixol from S. dulcis exhibited significant antidiabetic activity in improving the glucose tolerance and fasting blood glucose levels in diabetic animals. A flavonoid fraction extracted from S. dulcis showed higher glucose transport activity than insulin even in the insulin resistance model induced by free fatty acids. However, this study does not discuss the potential toxicity of ultra-high doses of S. dulcis extracts which may cause liver or kidney damage. More toxicological studies on humans should also be further carried out in the future.
Review of Freire et al. in 1993, Chow et al. in 1974 and Esume et al. 2011 found that dose dependent hypertension produced by the ethanolic extract of S. dulcis and its aqueous fraction is useful for treatment of hypertension.66,88,91 However, low-dose norepinephrine and epinephrine are easily degraded in the digestive tract after oral administration and are not absorbed into the blood in significant amounts. Nevertheless, the test results of Esume et al. 2011
88 also display that the higher doses of the aqueous and methanol extract of S. dulcis leaves significantly increased blood pressure, heart rate and mean arterial pressure. Therefore, therapeutic effect of S. dulcis on blood pressure needs to be tested clinically to substantiate claims of producing medicinal value.
The aspect of this review can assist the future study to clarify the compatibility of compound prescription in the treatment of metabolic diseases based on the ethnomedicines. 115 active chemical compounds in S. dulcis and their pharmacological activities have been elucidated in phytochemical studies. Particularly, scoparic acid is a unique compound of S. dulcis that may have extensive pharmacological activity and is the material basis for its treatment of metabolic syndrome. Therefore, the research of the pharmacological interactions of S. dulcis with the other medicinal herbs should be further conducted, which not only promotes the therapeutic activity for metabolic diseases but also develops new medicine for treating chronic conditions. For example, parts of pharmaceutical utility of S. dulcis with other medicinal herbs have not been deeply investigated, such as the treatment for kidney stones, diuresis, cardiovascular protection, and anticancer effects.
At present, only a fraction of chemical profile has been identified for S. dulcis. Further research may isolate additional chemical substances through other extraction methods on various part of this plant. It may be more meaningful from the perspective of new medicine development to guide the separation and extraction of new compounds in S. dulcis based on the pharmacological effects of the corresponding extracts of S. dulcis. In addition, compounds in S. dulcis that have clear antihyperglycemic and anti-hyperlipidaemia effects can be identified. The structure–activity relationships may be further explored based on their common chemical composition and pharmacological effects. Once the functional group is identified, substituent can potentially be modified to achieve higher pharmacological effectiveness. Furthermore, pharmacologists can continue to explore the specific targets of certain compounds in S. dulcis. For example, the targets of hypoglycemic effect may not be only those mentioned in this review, and new targets need to be further studied. Finally, if certain compounds in S. dulcis or their modified compounds with higher pharmacological activity are to be put into mass production in the pharmaceutical industry, the future researchers need to design a reasonable synthetic route. In short, S. dulcis is a well-used traditional herbal medicine, and perhaps new targets for the treatment of metabolic syndrome can be explored from the chemicals in it in the future.
Author contributions
Zikang Jiang and Lei Wen: concept development idea generation, literature collection and evaluation, manuscript preparation and editing, conduction of pharmacological effects, and analyzing their chemicals substances of S. dulcis.
Jinghui Sung: conduction of pharmacological effects analyses, associated with their effect on metabolic therapy, analysis results discussion, draft manuscript preparation, and editing.
Xuyun Wang, Yangyang Zhang, Yaomiao Wang, and Haifeng Zhou: pharmacological effects and their chemicals substances of S. dulcis analyses.
Conflicts of interest
There is no conflict to declare.
Notes and references
- K. Masaru, H. Toshimitsu, A. Munehisa and M. H. Naokata, Phytochemistry, 1988, 27(11), 3709–3711 CrossRef.
- M. Silink, Endocrinology, 2011, 2007(1), 12–14 Search PubMed.
- M. R. Mishra, R. K. Behera, S. Jha, A. K. Panda, A. Mishra, D. K. Pradhan and P. R. Choudary, Int. J. Phytomed., 2011, 3, 422–438 CAS.
- G. Pamunuwa, D. N. Karunaratne and Y. Waisundara Viduranga, Evid.-Based Complementary Altern. Med, 2016, 8243215 Search PubMed.
- K. Hasui, J. Wang, X. Jia, M. Tanaka, T. Nagai, T. Matsuyama and Y. Eizuru, Acta Histochem. Cytoc., 2011, 44(3), 119–131 CrossRef PubMed.
- C. Kamperdick, T. P. Lien, T. V. Sung and G. Adam, Pharmazie, 1997, 52(12), 965–966 CAS.
- M. Babincova, K. Schronerova and P. Sourivong, Fitoterapia, 2008, 79(7–8), 587–588 CrossRef PubMed.
- W. H. Wu, T. Y. Chen, R. W. Lu, S. T. Chen and C. C. Chang, Phytochemistry, 2012, 83, 110–115 CrossRef CAS PubMed.
- W. Wankhar, S. Srinivasan, R. Rajan and S. Rathinasamy, J. Appl. Pharmaceut. Sci., 2015, 5(7), 029–034 CrossRef CAS.
- N. N. T. Tin, N. D. T. Truc, H. T. T. Hang, P. T. N. Trinh, T. D. Lam, L. T. Dung, 2nd International Conference on Mechanical Engineering and Applied Composite Materials, Iop Publishing Ltd, Bristol, 2019 Search PubMed.
- S. M. D. Freire, L. M. B Torres, C. Souccar and A. J. Lapa, J. Pharm. Pharmacol., 1996, 48(6), 624–628 CrossRef CAS PubMed.
- M. Kawasaki, T. Hayashi, M. Arisawa, N. Morita and L. H. Berganza, Phytochemistry, 1988, 27(11), 3709–3711 CrossRef CAS.
- T. Hayashi, M. Kishi, M. Kawasaki, M. Arisawa, N. Morita and L. H. Berganza, J. Nat. Prod., 1988, 51(2), 360–363 CrossRef CAS.
- Y. Li and Y. Ohizumi, Yakugaku Zasshi, 2004, 124(7), 417–424 CrossRef CAS PubMed.
- Q. Liu, Q. M. Yang, H. J. Hu, L. Yang, Y. B. Yang, G. X. Chou and Z. T. Wang, J. Nat. Prod., 2014, 77(7), 1594–1600 CrossRef CAS PubMed.
- K. I. Sinan, K. Bene, G. Zengin, A. Diuzheva, J. Jeko, Z. Cziaky, C. M. N. Picot-Allain, A. Mollica, K. R. engasamy and M. F. Mahomoodally, Int. J. Environ. Health Res., 2019, 31(3), 285–297 CrossRef PubMed , Ahead of Print..
- H. Alkhalidy, W. Moore, Y. Wang, J. Luo, R. P. McMillan, W. Zhen, K. Zhou and D. Liu, Molecules, 2018, 23(9), 2338 CrossRef PubMed.
- X. Chen, X. Cai, R. Le, M. Zhang, X. Gu, F. Shen, G. Hong and Z. Chen, Biochem. Biophys. Res. Commun., 2018, 496(2), 245–252 CrossRef CAS PubMed.
- H. M. Eid and P. S. Haddad, Curr. Med. Chem., 2017, 24(4), 355–364 CrossRef PubMed.
- A. Ghorbani, Biomed Pharmacother., 2017, PMID: 29017142 Search PubMed.
- T. Hibi, Y. Imai, A. Senoo, K. Ohta and Y. Ukyo, J. Gastroenterol., 2017, 52, 1101–1111 CrossRef CAS PubMed.
- S. Raja Kumar, E. S. Mohd Ramli, N. A. Abdul Nasir, N. H. M. Ismail and N. A. Mohd Fahami, Evid Based Complement Alternat Med, 2019, 9752826 Search PubMed.
- P. M. Giang, P. T. Son, M. Katsuyoshi and O. Hideaki, Chem. Pharm. Bull., 2006, 54(4), 546–549 CrossRef PubMed.
- S. Asano, M. Mizutani, T. Hayashi, N. Morita and N. Takeguchi, J. Biol. Chem., 1990, 265(36), 22167–22173 CrossRef CAS.
- C. Y. Zhang, L. Z. Chen, Y. Y. Li, N. Wei, L. Zhang, L. Dong, Y. Zhang and X. P. Zhang, Rec. Nat. Prod., 2020, 14(5), 383–386 CrossRef CAS.
- W. Sun, Y. Zhang, Y. Li, L. Chen, L. Zhang, C. Zhang and X. Zhang, Phytochem. Lett., 2020, 38, 25–27 CrossRef CAS.
- R. Saikia, M. D. Choudhury, A. D. Talukdar and P. Chetia, Asian J. Pharmaceut. Clin. Res., 2012, 5(Suppl. 2), 153–158 CAS.
- T. Hayashi, K. Okamura, M. Kakemi, S. Asano, M. Mizutani, N. Takeguchi, M. Kawasaki, Y. Tezuka, T. Kikuchi and N. Morita, Chem. Pharm. Bull., 1990, 38(10), 2740–2745 CrossRef CAS PubMed.
- M. Ahsan, S. K. N. Islam, I. Gray Alexander and H. Stimson William, J. Nat. Prod., 2003, 66(7), 958–961 CrossRef CAS PubMed.
- M. S. Islam, M. K. Ahmed, M. Raknuzzaman, M. Habibullah-Al-Mamun and S. Masunaga, Arch. Environ. Contam. Toxicol., 2015, 68(1), 92–106 CrossRef CAS PubMed.
- S. B. Mahato, M. C. Das and N. P. Sahu, Phytochemistry, 1981, 20(1), 171–173 CrossRef CAS.
- R. Sharma Khaga, A. Adhikari, M. I. Choudhary, R. Sharma Khaga, K. Kalauni Surya, M. Hafizur Rahman, A. Hameed, A. Raza Sayed, J.-I. Miyazaki and M. I. Choudhary, Phytotherapy research, 2015, 29(10), 1672–1675 CrossRef PubMed.
- J. L. Ríos and S. Manez, Plantamedica, 2018, 84(01), 8–19 CrossRef PubMed.
- W. T. Wan, Y. Y. Ma, L. J. Xu and P. G. Xiao, Zhongcaoyao, 2015, 46(16), 2492–2498 CAS.
- J. Larsson, J. Gottfries, L. Bohlin and A. Backlund, J. Nat. Prod., 2005, 68(7), 985–991 CrossRef CAS PubMed.
- R. Khanra, S. Dewanjee, T. K. Dua and N. Bhattacharjee, Biomed. Pharmacother., 2017, 88, 918–923 CrossRef CAS PubMed.
- R. Khanra, N. Bhattacharjee, T. K. Dua, A. Nandy, A. Saha and J. Kalita, Biomed. Pharmacother., 2017, 94(2017), 726–741 CrossRef CAS PubMed.
- Q. Liang, J. Yang, J. He, X. Chen, H. Zhang, M. Jia, K. Liu, C. Jia, Y. Pan and J. Wei, Biosci. Rep., 2020, 40(4), BSR20192133 CrossRef CAS PubMed.
- S. Babu and S. Jayaraman, Biomed. Pharmacother., 2020, 131, 110702 CrossRef CAS PubMed.
- S. Datta, B. K. Sinha, S. Bhattacharjee and T. Seal, J. Pharmacogn. Phytochem., 2018, 7(2), 3963–3970 CAS.
- M. Naveed, V. Hejazi, M. Abbas, A. A. Kamboh, G. J. Khan, M. Shumzaid, F. Ahmad, D. Babazadeh, X. FangFang, F. Modarresi-Ghazani, L. WenHua and Z. XiaoHui, Biomed. Pharmacother., 2018, 97, 67–74 CrossRef CAS PubMed.
- B. M. Bocco, R. A. Louzada, D. H. Silvestre, M. C. Santos, E. Anne-Palmer, I. F. Rangel, S. Abdalla, A. C. Ferreira, M. O. Ribeiro, B. Gereben, D. P. Carvalho, A. C. Bianco and J. P. Werneck-de-Castro, J. Physiol., 2016, 594(18), 5255–5269 CrossRef CAS PubMed.
- A. Pandi and V. M. Kalappan, Mol. Biol. Rep., 2021, 2021, PMID: 33988797 Search PubMed.
- J. Qiao, Z. Luo, S. Cui, H. Zhao, Q. Tang, C. Mo, X. Ma and Z. Ding, J. Ind. Microbiol. Biotechnol., 2019, 46(2), 147–157 CrossRef CAS PubMed.
- J. E. Beh, L. T. Khoo, J. Latip, M. P. Abdullah, N. B. M. Alitheen, Z. Adam, A. Ismail and M. Hamid, J. Ethnopharmacol., 2013, 150(1), 339–352 CrossRef CAS PubMed.
- O. M. Zabad, Y. A. Samra and A. E. Laila, Life Sci., 2019, 238, 116965 CrossRef CAS PubMed.
- A. Farshad, B. M. Razavi and H. Hosseinzadeh, Phytother Res., 2020, 34(4), 729–741 CrossRef PubMed.
- K. Wangsa, I. Sarma, P. Saikia, D. Ananthakrishnan, H. N. Sarma and D. Velmurugan, J. Reproduction Infertil., 2020, 21(4), 247–258 Search PubMed.
- K. M. Nkembo, J. B. Lee and T. Hayashi, Chem. Pharm. Bull., 2005, 53(1), 780–782 CrossRef CAS PubMed.
- L. Pari and M. Latha, BMC Compl. Alternative Med., 2004, 4, 16 CrossRef PubMed.
- M. Latha, L. Pari, S. Sitasawad and R. Bhonde, J. Biochem. Mol. Toxicol., 2004, 18(5), 261–272 CrossRef CAS PubMed.
- N. H. Parikh, P. K. Parikh and C. Kothari, Chin. J. Nat. Med., 2014, 12(5), 335–344 CAS.
- R. Saikia, M. D. Choudhury, A. D. Talukdar and P. Chetia, Asian J. Pharmaceut. Clin. Res., 2012, 5(Suppl. 2), 153–158 CAS.
- M. Latha and L. Pari, Braz. J. Med. Biol. Res., 2004, 37(4), 577–586 CrossRef CAS PubMed.
- M. Latha, L. Pari, S. B. Sandhya and R. Bhonde, Life Sci., 2004, 75(16), 2003–2014 CrossRef CAS PubMed.
- L. Pari and M. Latha, Gen. Physiol. Biophys., 2005, 24(1), 13–26 CAS.
- A. Hameed, M. Hafizur Rahman, M. I. Khan, A. Jawed, S. Siddiqui, F. Khan, H. Wang, M. Zhao, K. Matsunaga, T. Izumi, A. Adhikari and R. Sharma Khaga, Eur. J. Pharmacol., 2019, 858, 172514 CrossRef CAS PubMed.
- R. Sharma Khaga, A. Adhikari, M. I. Choudhary, R. Sharma Khaga, K. Kalauni Surya, M. Hafizur Rahman, A. Hameed, A. Raza Sayed, J.-I. Miyazaki and M. I. Choudhary, Phytotherapy researchPTR, 2015, 29(10), 1672–1675 CrossRef PubMed.
- V. J. Sharma and U. D. Shah, Int. J. ChemTech Res., 2010, 2(1), 214–218 CAS.
- P. Geethi and K. D. Nedra, Evid.-Based Complementary Altern. Med., 2016, 2016, 8243215 Search PubMed.
- J. E. Beh, J. Latip, M. P. Abdullah, A. Ismail and M. Hamid, J. Ethnopharmacol., 2010, 129(1), 23–33 CrossRef CAS PubMed.
- P. R. D. Perera, S. Ekanayake and K. Ranaweera, Int. J. Appl. Chem., 2015, 2015, 519–524 Search PubMed.
- L. Pari and M. Latha, J. Med. Food, 2006, 9(1), 102–107 CrossRef PubMed.
- S. P. A. Senadheera, S. Ekanayake and C. Wanigatunge, BMC Compl. Alternative Med., 2015, 15, 410 CrossRef PubMed.
- S. M. Freire, L. M. Torres, N. F. Roque, C. Souccar and A. J. Lapa, Mem. Inst. Oswaldo Cruz, 1991, 86(Suppl 2), 149–151 CrossRef PubMed.
- S. M. Freire, J. A. da Silva Emim, A. J. Lapa, C. Souccar and S. M. d. F. Freire, Phytother Res., 1993, 7(6), 408–414 CrossRef.
- J. C. Tsai, W. H. Peng, T. H. Chiu, S. C. Lai and C. Y. Lee, Am. J. Chin. Med., 2011, 39(5), 943–956 CrossRef CAS PubMed.
- J. Ofori-Amoah and G. A. Koffuor, Pharmacologia, 2015, 6(8), 337–346 CrossRef.
- P. Libby, P. M. Ridker and G. K. Hansson, Nature, 2011, 473(7347), 317–325 CrossRef CAS PubMed.
- N. E. J. Orhue and E. A. C Nwanze, Afr. J. Biotechnol., 2006, 5(10), 883–887 Search PubMed.
- S. S. Nambiar, N. P. Shetty, P. Bhatt and B. Neelwarne, Phcog. Mag., 2014, 10(Suppl 2), S240–S248 Search PubMed.
- M. V. V. Lima, A. d. O. Freire, E. L. F. Sousa, A. A. M. Vale, A. J. O. Lopes, C. C. Vasconcelos, M. V. V. Lima-Aragao, H. O. Serra, R. N. M. G. Liberio, A. P. Silva de Azevedo dos Santos, G. E. B. Silva, C. Quintino da Rocha, F. C. V. M. Lima, M. d. S. d. S. Cartagenes and J. B. S. Garcia, Molecules, 2019, 24(19), 3474 CrossRef CAS.
- The 168th Hospital of PLA, Infection Department of 168 Hospital of Chinese people's Liberation Army, 16th hospital of the Chinese People’s Liberation, Army, 73, 1976, in Chinese Search PubMed.
- J. C. Tsai, W. H. Peng, T. H. Chiu, S. C. Huang, T. H. Huang, S. C. Lai, Z. R. Lai and C. Y. Lee, Am. J. Chin. Med., 2010, 38(4), 761–775 CrossRef PubMed.
- J. Paysant, P. Sansilvestri-Morel, E. Bouskela and T. J. Verbeuren, Int. Angiol., 2008, 27(1), 81–85 CAS.
- T. K. Praveen, S. Dharmaraj, J. Bajaj, S. P. Dhanabal, S. Manimaran, M. J. Nanjan and R. Razdan, Indian J. Pharmacol., 2009, 41(3), 110–114 CrossRef CAS PubMed.
- I. Bulama, H. T. Kabara and A. Zarami, Comp. Clin. Pathol., 2020, 29, 807–813 CrossRef CAS.
- M. A. Adaikpoh, N. E. J. Orhue and I. Igbe, Afr. J. Biotechnol., 2007, 6(10), 1192–1196 CAS.
- W. Wankhar, S. Srinivasan, R. Rajan and R. Sheeladevi, J. Biomed. Res., 2017, 31(2), 143–153 Search PubMed.
- W. D. Ratnasooriya, J. Jayakody, G. A. S. Premakumara and E. Ediriweera, Fitoterapia, 2005, 76(2), 220–222 CrossRef CAS PubMed.
- Y. Coulibaly Ahmed, M. Kiendrebeogo, G. Kehoe Patrick, A. E. D. Sombie Pierre, E. Lamien Charles, F. Millogo Jeanne and G. Nacoulma Odile, J. Med. Food, 2011, 14(12), 1576–1582 CrossRef CAS PubMed.
- A. Elayaraja and S. Abdul Rahaman, J. Pharmaceut. Sci. Res., 2012, 4(2), 1724–1727 CAS.
- N. Chana, P. Supaphon and A. Phongdara, Songklanakarin J. Sci. Technol., 2019, 41(1), 246–253 CAS.
- M. M. A. R. Moniruzzaman and A. Ferdous, J. Evidence-Based Complementary Altern. Med., 2015, 2015, 873954 Search PubMed.
- T. A. Abere, C. J. Okoye and F. O. Ahoeryo, et al., BMC Complementary Altern. Med., 2015, 15, 414 CrossRef PubMed.
- B. Madakkannu and R. Ravichandran, Toxicol. Rep., 2017, 4, 484–493 CrossRef PubMed.
- R. Ahemd, H. D. Nuhu and H. Ibrahim, et al., Trop. J. Nat. Prod. Res., 2019, 3(3), 64–70 CrossRef.
- C. O. Esume, A. O. Opajobi, A. Osasuyi, O. O. Ebong and A. K. Osakwe, Biomed. Pharmacol. J., 2011, 4(1), 11–19 CrossRef CAS.
- F. A. van de Laar, P. L. Lucassen, R. P. Akkermans, E. H. van de Lisdonk, G. E. Rutten and C. van Weel, Diabetes Care, 2005, 28(1), 154–163 CrossRef CAS.
- M. C. Thomas, K. A. Jandeleit-Dham and C. Tikellis, PPAR Res., 2012, 2012(2), 456529 CAS.
- S. Y. Chow, S. M. Chen, C. M. Yang and H. Hsu, J. Formosan Med. Assoc., 1974, 73, 729–739 CAS.
- L. Pari, M. Latha and C. A. Rao, J. Basic Clin. Physiol. Pharmacol., 2004, 15(3–4), 223–240 CAS.
- M. Latha, L. Pari, K. M. Ramkumar, P. Rajaguru, T. Suresh, T. Dhanabal, S. Sitasawad and R. Bhonde, Nat. Prod. Res., 2009, 23(16), 1528–1540 CrossRef CAS PubMed.
- R. Rajan, M. Vedi, B. Sridharan, M. Himaja, E.P. Sabina and N.A.N. Raj, Int. J. Phytomed., 2014, 6(4), 617–624 CAS.
|
This journal is © The Royal Society of Chemistry 2021 |
Click here to see how this site uses Cookies. View our privacy policy here.