DOI:
10.1039/C8RA06178E
(Paper)
RSC Adv., 2018,
8, 31231-31236
AIE-active polyanetholesulfonic acid sodium salts with room-temperature phosphorescence characteristics for Fe3+ detection†
Received
21st July 2018
, Accepted 23rd August 2018
First published on 4th September 2018
Abstract
Room-temperature phosphorescent materials have been a major focus of research and development during the past decades, due to their applications in OLEDs, photovoltaic cells, chemical sensors, and bioimaging. However, achieving polymeric phosphorescent materials without heavy-metal atoms and halogens under ambient conditions remains a major challenge. Here, we report a polymeric phosphor, namely polyanetholesulfonic acid sodium salt, which not only has room temperature phosphorescence characteristic but also aggregation-induced emission and dependence on the excitation wavelength characteristics. Moreover, it can recognize Fe3+ effectively.
1. Introduction
Polymeric luminophores are promising materials for applications in various areas such as light-emitting diodes, plastic lasers, solar cells, chemosensors and bioprobes.1–7 In response to the growing demand, a large number of polymeric luminescent materials have been synthesized. However, many of them are highly radiative in dilute solutions while being weakly luminescent or quenched in concentrated solutions or in the solid state, exhibiting concentration quenching and aggregation-caused quenching (ACQ) problems.8 The ACQ effect has limited the scope of technological applications because luminescent materials have to be used in the solid state (e.g. as thin films), where the luminophores tend to form aggregates.9 To alleviate the ACQ effect in the condensed phase, various chemical, physical and engineering approaches have been developed. For example, branched chains, spiro kinks, and dendritic wedges have been covalently attached to aromatic rings to impede aggregate formation. However, those attempts have met with limited success.9
Aggregation-induced emission (AIE) characteristic, which is opposite to the ACQ effect was discovered by Tang et al. in 2001. Since then, many organic and polymeric AIE-active materials have been synthesized and applied in various fields.10 However, in the field of polymeric AIE-active materials, most studies focus on fluorescent polymeric materials,11–13 few literature examples deal with phosphorescent polymers at ambient or cryogenic temperatures.14–17
Polymeric room temperature phosphorescent (RTP) materials have attracted a great deal of interest due to their potential applications in organic light-emitting diodes (OLED),18 photovoltaics,19 photocatalytic reactions,20 and bioimaging.21 Phosphorescent compounds have a large Stokes shift and long lifetimes.22 Therefore, for applications in bio-probes, phosphorescent probes can distinguish the background signals from each other by using time-resolved techniques, thus improving the signal-to-noise and sensitivity of detection.23 Large numbers of polymeric RTP materials that have been developed so far are rare metal complexes involving Ir and Pt. However, these compounds have potential toxicity and instability in aqueous environments. And these noble metals are not only costly but also resource-limited, which have hindered the development of phosphorescent materials. Also, most organic RTP materials that have been reported are compounds containing halogen atoms, which are essential units to facilitate intersystem crossing.24–27 However, aromatic halogen-containing compounds may have adverse effects on the environment and human health.28–30 Therefore, polymeric luminophores without noble metals and halogens might be better choices.
Herein, a noble metal- and halogen-free polymer material (Scheme 1) based on commercial polyanetholesulfonic acid sodium salts (PASAS, CAS number: 52993-95-0) is purified and further studied. The results show that PASAS is AIE active, and the photoluminescence quantum yield (PLQY) of its solid samples (Φsolid) is 7.57%. More importantly, PASAS also exhibits RTP, and the phosphorescence lifetimes at 460 and 525 nm are 0.24 ms and 0.77 ms, respectively. In addition, it can also recognize Fe3+ effectively in aqueous solution.
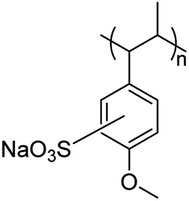 |
| Scheme 1 Chemical structure of polyanetholesulfonic acid sodium salts. | |
2. Experimental
2.1 Materials
PASAS was obtained from J&K Scientific., Ltd. Metal salts of LiCl, CuCl, AgNO3, CaCl2, MgSO4, AlCl3·6H2O, CuNO3·3H2O, FeCl2·4H2O, FeCl3·6H2O, ZnNO3·6H2O were obtained from Xilong Chemical Co., Ltd. CoCl2·6H2O, K2CO3, CdCl2·5/2H2O and NiCl2·6H2O were obtained from Adamas Reagent Co., Ltd. Distilled water was prepared by Milli-Q Pure Water instrument. The commercially available reagents were used without further purification.
2.2 Characterization
UV-vis absorption spectra were obtained using a UV3600 UV-vis spectrophotometer under ambient conditions. Fluorescence spectra of the solutions were obtained from a Cary Eclipse fluorescence spectrophotometer. The mean diameter (Dm) and polydispersity (PD) of PASAS solution were determined on a Malvern Zetasizer nano ZS90 instrument equipped with a He–Ne laser (λ = 633 nm) at a scattering angle of 90° at 25 °C. X-ray diffraction (XRD) measurements were performed on a X′ Pert PRO X-ray diffractometer. The fluorescence lifetimes were measured with an FLS-980 fluorescence spectrophotometer. The emission spectra, quantum yields and phosphorescence lifetime of solid samples were recorded on a FluoroMax-4 fluorescence spectrophotometer. Quantum yields (Φ) of PASAS in water as solvent were estimated using 2-aminopyridine (Φ = 60% in 0.1 N H2SO4) as standard.31 Centrifugation was carried out with CT14D high-speed table centrifuge.
2.3 Purification of PASAS
First, a PASAS solution was obtained by dissolving it in a small amount of water. Then, a straw-stuffed with a small amount of cotton at the tip was used for sucking the solution into a beaker filled with a large amount of methanol, so that the solid samples of PASAS could slowly precipitate. At last, the pure solid samples of PASAS were obtained by centrifugation and drying.
2.4 Preparation of selectivity towards Fe3+
Solutions of the following metal ions (Li+, K+, Ag+, Cu+, Ca2+, Mg2+, Zn2+, Cd2+, Co2+, Ni2+, Cu2+, Fe2+, Fe3+, and Al3+, 2.0 × 10−3 mol L−1) were prepared in distilled water. A stock solution of PASAS (5.0 mg mL−1) was prepared with distilled water. The resulting solutions were obtained by mixing the metal ion solutions (2 mL) with the polymer solutions (5 mL), respectively. Then the absorption and fluorescence measurements of the mixed solutions were conducted.
2.5 Preparation of fluorescence assay for detecting Fe3+
Solutions of Fe3+ with different concentrations (0, 0.02 × 10−3, 0.04 × 10−3, 0.06 × 10−3, 0.08 × 10−3, 0.1 × 10−3, 0.2 × 10−3, 0.4 × 10−3, 0.8 × 10−3, 1.2 × 10−3, 1.6 × 10−3, 2.0 × 10−3 mol L−1) were prepared with distilled water. The stock solution of PASAS (5.0 mg mL−1) was prepared with distilled water at room temperature. The resulting solutions were obtained by mixing the Fe3+ solutions (2 mL) with polymer solutions (5 mL). Then the absorption and fluorescence measurements of the mixed solutions were conducted.
3. Results and discussion
3.1 Excitation dependent fluorescence emission
Photoluminescence properties of many polymer materials have a dependence on the excitation wavelengths because of the dispersity of their molecular weight.32–34 To verify whether it had a dependence on excitation wavelengths, the fluorescence emission spectra of PASAS aqueous solution (10 mg mL−1) were measured at different excitation wavelengths. As shown in Fig. 1a, when excited at 310 nm, it showed a maximal emission at 372 nm. With increasing excitation wavelengths, the emission intensity tended to increase first and then decrease, meanwhile the emission wavelength gradually redshifted. When excited at 320 nm, the fluorescence intensity was the strongest. At the same time, we could also observe that the illuminant colors were deep blue and sky blue under 312 nm and 365 nm UV radiation, respectively. To compare the optical behavior of different emissions, we measured the time-resolved photoluminescent (PL) decay curves, as shown in Fig. 1b. The fluorescence lifetimes at 372 nm and 420 nm were 27.97 ns and 4.31 ns, respectively. These results indicated PASAS had versatile optical properties.
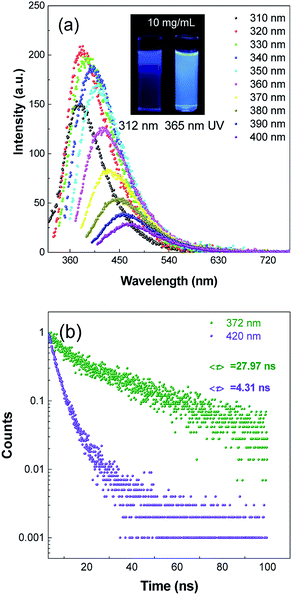 |
| Fig. 1 (a) Fluorescence spectra of PASAS aqueous solution (10 mg mL−1) with different excitation wavelengths. (b) The effect of excitation wavelength on fluorescence lifetime of PASAS aqueous solution (10 mg mL−1). | |
3.2 Aggregation-induced emission (AIE) characteristics
In order to find out whether it had AIE characteristics, fluorescence spectra and UV absorption spectra of PASAS aqueous solutions with different concentrations were measured. As shown in Fig. S1a (ESI†), the absorption intensity gradually increased and the absorption wavelength gradually red-shifted as the concentration gradually increased. Meanwhile, the absorption spectra also showed the end tailing phenomenon. These phenomena could be due to the scattering effect caused by the accumulation of aggregated particles in the system. As shown in Fig. 2b, when the concentration was 0.1 mg mL−1, the emission intensity was very weak. The emission intensity continuously strengthened with the increase in concentration. When increased to 10 mg mL−1 (Dm = 185 nm, PD = 0.48, Fig. S1b, ESI†), the emission intensity rapidly increased due to molecular aggregation, giving a strong blue emission at about 380 nm. Meanwhile, it could also be seen that the luminescence intensity increased with the increase in concentration under 365 nm UV radiation (Fig. 2a), which indicated aggregation-induced emission (AIE) characteristics.
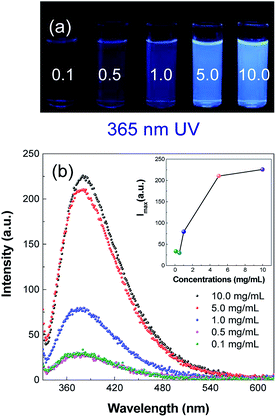 |
| Fig. 2 (a) Photographs taken under 365 nm UV light and (b) fluorescence spectra of PASAS aqueous solutions with different concentrations. | |
To gain more information on the AIE process, emission spectra of PASAS aqueous solution and ACN/water mixtures were measured (Fig. S2a†). ACN was adopted because it was a nonsolvent for PASAS, the polymer chains may be aggregated in the mixture with high ACN fractions. When the ACN fraction (fACN) was 0%, only a weak signal was recorded. However, when fACN was increased to 99% (Dm = 116.2 nm, PD = 0.37, Fig. S2b, ESI†), the emission intensity rapidly increased. This also indicated that aggregates formed with the increase in the nonsolvent content. Besides, the Φsolution values of PASAS aqueous solution were as low as 1.70%. However, the Φsolid values of PASAS solids increased to 7.57%, and those results also suggest that PASAS is AIE active.
3.3 Room temperature phosphorescence (RTP) characteristics
Preliminary studies found PASAS solids could emit light blue luminescence with excitation by a UV lamp at 365 nm under ambient conditions (Fig. 3a). To gain deeper insight into the nature of the light blue emission, the prompt and steady-state photoluminescence spectra of solid samples were collected under ambient conditions. As shown in Fig. 3b, the fluorescence emission wavelength gradually red-shifted with an increase in the excitation wavelength, which also indicated a dependence on the excitation wavelength. Time-resolved (delayed) PL spectra were obtained 0.5 ms after excitation (Fig. 3c). The delayed spectra were found to exhibit dual emission bands peaking at around 460 and 525 nm. Furthermore, the difference between the peak positions of the prompt spectrum and the delayed spectrum was nearly 80 nm under excitation of 320 nm, which indicated that it was room temperature phosphorescence rather than delayed fluorescence. To further confirm PASAS solids had room temperature phosphorescence characteristics, time-resolved decay curves at 460 and 525 nm were recorded (Fig. 3d). The results showed that the lifetimes of both were in milliseconds, which accorded with the phosphorescence characteristic of the long lifetime. Therefore, the above results showed that PASAS solids had RTP characteristics.35 RTP was ascribed to restricted molecular motions (RMM)36 from the strong ionic bond and abundant hydrogen bonding in the crystalline state (Fig. S3, ESI†). Such intermolecular and intramolecular interactions greatly rigidified the molecular conformation and drastically decreased the nonradiative deactivation channels of the triplet excitons, thus giving rise to phosphorescent emission at room temperature.36,37
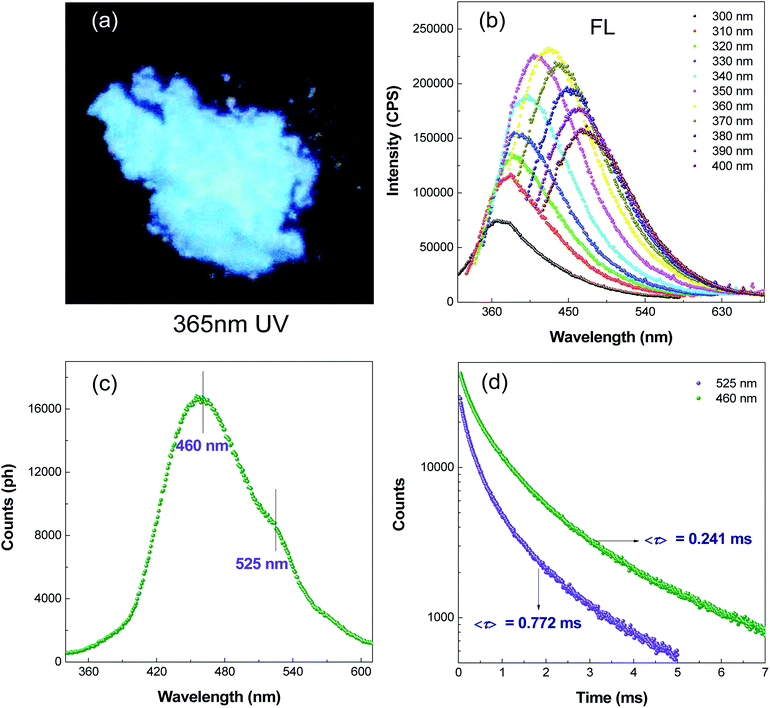 |
| Fig. 3 (a) The photograph of PASAS solids taken under 365 nm UV radiation. (b) and (c) are the fluorescence and phosphorescence spectra of PASAS solids, respectively. (d) Time-resolved decay curves of PASAS solids at 460 and 525 nm. Excitation wavelength and delay time of phosphorescence spectra are 320 nm and 0.5 ms, respectively. | |
3.4 Selectivity of polymer chemosensor towards Fe3+
The UV absorption and fluorescence response of PASAS (5 mg mL−1) to various metal ions including Li+, K+, Ag+, Mg2+, Ca2+, Zn2+, Cd2+, Cu+, Cu2+, Co2+, Ni2+, Fe2+, Fe3+ and Al3+ (2 × 10−3 mol L−1) were studied in aqueous solution (5
:
2, v/v). 365 nm UV photographs had preliminarily indicated that only Fe3+ could quench the luminescence of the solution (Fig. 4a). Meanwhile, only Fe3+ could enhance the absorption intensity, which changed a little after addition of other metal ions (Fig. S4, ESI†). Interestingly, when 2 mL of different metal ions were added, only the addition of Fe3+ significantly quenched the fluorescence intensity of PASAS at 380 nm (Fig. 4b). Other metal ions could not induce a significant change in fluorescence intensity of PASAS. To gain a deeper insight into the mechanism of fluorescence quenching, the fluorescence lifetimes before and after the addition of Fe3+ (0.2 × 10−3 mol L−1) were measured. Here, the lifetime values of PASAS solution before and after the addition of Fe3+ ion are determined as 11.2 and 8.6 ns (Fig. S5, ESI†), respectively. The results show that the lifetime becomes shorter after the addition of Fe3+. Thus, the reason of fluorescence emission quenching was the stronger coordination ability to the polymer sensor and photoinduced electron transfer (PET) process38,39 from sodium benzene sulfonic acid moiety to the coordinated metal ions (Fe3+). Therefore, Fe3+ could be rapidly distinguished from other metal ions by comparing the luminescence intensity of the solution. It also indicated that PASAS was a highly selective fluorescence probe for Fe3+ among various metal ions.
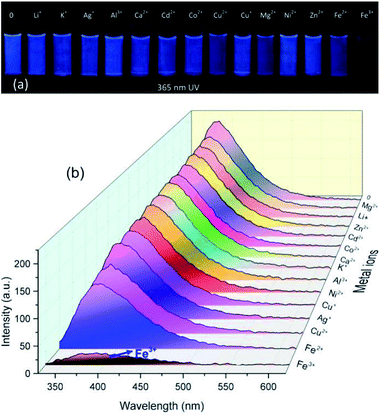 |
| Fig. 4 (a) Photographs taken under 365 nm UV light and (b) fluorescence spectra of PASAS aqueous solution (5 mg mL−1) in the presence of various metal ions (2 × 10−3 mol L−1). | |
3.5 Fluorescence assay for detecting Fe3+
To evaluate the Fe3+ sensing property of PASAS, the UV absorption and fluorescence response of PASAS (5 mg mL−1) in aqueous solution towards various concentrations of Fe3+ were investigated. As shown in Fig. S6a (ESI†), the UV absorption intensity increased with the increase in Fe3+ concentration due to the absorption of FeCl3. Upon the addition of Fe3+, significant fluorescence quenching could be observed visually in the aqueous solution under 365 nm UV lamp irradiation. Remarkably, the emission intensity (at 380 nm) was quenched by about 5.9-fold when the concentration of Fe3+ increased to 2 × 10−3 mol L−1 (Fig. 5). The fluorescence intensity against the Fe3+ concentration exhibited a good linear relationship (R2 = 0.9997) in the range of 0.1 × 10−3 to 0.8 × 10−3 mol L−1 (Fig. S6b, ESI†). Based on the above results, it can be confirmed that this polymer sensor could quantitatively detect Fe3+ in aqueous solution.40
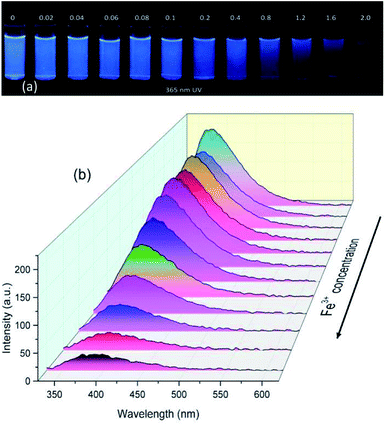 |
| Fig. 5 (a) Photographs taken under 365 nm UV light and (b) fluorescence spectra of PASAS aqueous solution (5 mg mL−1) on addition of different concentrations of Fe3+. | |
4. Conclusions
In summary, an AIE-active room temperature phosphorescence polymer without heavy-metal atom and halogen was investigated. This polymer is weakly emissive in solution, but could be induced to emit intensely in highly concentrated solutions or in the solid state. The PLQY of the solid sample is 7.57%. Moreover, PASAS also emits RTP under ambient conditions, the phosphorescence emission peak and lifetime at 460 and 525 nm were 0.24 ms and 0.77 ms, respectively. Furthermore, the potential application of this polymer as a fluorescence sensor has been demonstrated by using it as a Fe3+ probe. All these advantages make it a very promising material for developing luminescent devices for a large number of applications.
Conflicts of interest
There are no conflicts to declare.
Acknowledgements
This work was financially supported by the National Natural Science Foundation of China (51603050, 51863006), the Natural Science Foundation of Guangxi (2016GXNSFBA380196), Guangxi University Young and Middle-aged Teachers Basic Ability Promotion Project (KY2016YB316).
Notes and references
- Y. Sagara and T. Kato, Nat. Chem., 2009, 1, 605 CrossRef CAS.
- N. Sang, C. Zhan and D. Cao, J. Mater. Chem. A, 2015, 3, 92–96 RSC.
- W. Zhang Yuan and Y. Zhang, J. Polym. Sci., Part A: Polym. Chem., 2017, 55, 560–574 CrossRef.
- F. Bella, G. Griffini, J.-P. Correa-Baena, G. Saracco, M. Grätzel, A. Hagfeldt, S. Turri and C. Gerbaldi, Science, 2016, 354, 203–206 CrossRef CAS.
- S. Y. Lee, T. Yasuda, H. Komiyama, J. Lee and C. Adachi, Adv. Mater., 2016, 28, 4019–4024 CrossRef CAS.
- S. T. Duong and M. Fujiki, Polym. Chem., 2017, 8, 4673–4679 RSC.
- G. D. Gutierrez, I. Coropceanu, M. G. Bawendi and T. M. Swager, Adv. Mater., 2016, 28, 497–501 CrossRef CAS.
- R. Hu, N. L. Leung and B. Z. Tang, Chem. Soc. Rev., 2014, 43, 4494–4562 RSC.
- J. Mei, N. L. Leung, R. T. Kwok, J. W. Lam and B. Z. Tang, Chem. Rev., 2015, 115, 11718–11940 CrossRef CAS.
- J. Luo, Z. Xie, J. W. Lam, L. Cheng, H. Chen, C. Qiu, H. S. Kwok, X. Zhan, Y. Liu and D. Zhu, Chem. Commun., 2001, 1740–1741 RSC.
- Y. Hong, J. W. Lam and B. Z. Tang, Chem. Commun., 2009, 4332–4353 RSC.
- Z. Sheng, B. Guo, D. Hu, S. Xu, W. Wu, W. H. Liew, K. Yao, J. Jiang, C. Liu and H. Zheng, Adv. Mater., 2018, 30, 1870214 CrossRef.
- M. Chen, R. Chen, Y. Shi, J. Wang, Y. Cheng, Y. Li, X. Gao, Y. Yan, J. Z. Sun and A. Qin, Adv. Funct. Mater., 2018, 28, 1704689 CrossRef.
- C.-R. Wang, Y.-Y. Gong, W.-Z. Yuan and Y.-M. Zhang, Chin. Chem. Lett., 2016, 27, 1184–1192 CrossRef CAS.
- S. Mukherjee and P. Thilagar, Chem. Commun., 2015, 51, 10988–11003 RSC.
- C. Li, X. Tang, L. Zhang, C. Li, Z. Liu, Z. Bo, Y. Q. Dong, Y. H. Tian, Y. Dong and B. Z. Tang, Adv. Opt. Mater., 2015, 3, 1184–1190 CrossRef CAS.
- Q. Zhou, B. Cao, C. Zhu, S. Xu, Y. Gong, W. Z. Yuan and Y. Zhang, Small, 2016, 12, 6586–6592 CrossRef CAS.
- X. Yang, G. Zhou and W.-Y. Wong, J. Mater. Chem. C, 2014, 2, 1760–1778 RSC.
- C. C. Chen, W. H. Chang, K. Yoshimura, K. Ohya, J. You, J. Gao, Z. Hong and Y. Yang, Adv. Mater., 2014, 26, 5670–5677 CrossRef CAS.
- L. Pan, M.-Y. Xu, L.-J. Feng, Q. Chen, Y.-J. He and B.-H. Han, Polym. Chem., 2016, 7, 2299–2307 RSC.
- Z. Chen, K. Y. Zhang, X. Tong, Y. Liu, C. Hu, S. Liu, Q. Yu, Q. Zhao and W. Huang, Adv. Funct. Mater., 2016, 26, 4386–4396 CrossRef CAS.
- Q. Zhao, C. Huang and F. Li, Chem. Soc. Rev., 2011, 40, 2508–2524 RSC.
- G. Marriott, R. M. Clegg, D. J. Arndt-Jovin and T. M. Jovin, Biophys. J., 1991, 60, 1374–1387 CrossRef CAS.
- S. Cai, H. Shi, D. Tian, H. Ma, Z. Cheng, Q. Wu, M. Gu, L. Huang, Z. An and Q. Peng, Adv. Funct. Mater., 2018, 28, 1705045 CrossRef.
- S. K. Min, Y. Yu, C. Coburn, A. W. Phillips, K. Chung, A. Shanker, J. Jung, G. Kim, K. Pipe and S. R. Forrest, Nat. Commun., 2015, 6, 8947 CrossRef.
- B. Xu, H. Wu, J. Chen, Z. Yang, Z. Yang, Y. C. Wu, Y. Zhang, C. Jin, P. Y. Lu and Z. Chi, Chem. Sci., 2017, 8, 1909 RSC.
- S. Hirata, Adv. Opt. Mater., 2017, 5, 1700116 CrossRef.
- P. O. Darnerud, Environ. Int., 2003, 29, 841–853 CrossRef CAS.
- B. J. Finlayson-Pitts and J. N. Pitts, Science, 1997, 276, 1045–1051 CrossRef CAS PubMed.
- A. Poland and J. C. Knutson, Annu. Rev. Pharmacol. Toxicol., 1982, 22, 517–554 CrossRef CAS.
- R. Rusakowicz and A. C. Testa, J. Phys. Chem., 1968, 72, 2680–2681 CrossRef CAS.
- L. Pan, S. Sun, A. Zhang, K. Jiang, L. Zhang, C. Dong, Q. Huang, A. Wu and H. Lin, Adv. Mater., 2015, 27, 7782–7787 CrossRef CAS.
- H. Wang, C. Sun, X. Chen, Y. Zhang, V. L. Colvin, Q. Rice, J. Seo, S. Feng, S. Wang and W. Y. William, Nanoscale, 2017, 9, 1909–1915 RSC.
- A. Sharma, T. Gadly, A. Gupta, A. Ballal, S. K. Ghosh and M. Kumbhakar, J. Phys. Chem. Lett., 2016, 7, 3695–3702 CrossRef CAS.
- Y. Gong, L. Zhao, Q. Peng, D. Fan, W. Z. Yuan, Y. Zhang and B. Z. Tang, Chem. Sci., 2015, 6, 4438–4444 RSC.
- L. Du, G. He, Y. Gong, W. Z. Yuan, S. Wang, C. Yu, Y. Liu and C. Wei, Compos. Commun., 2018, 8, 106–110 CrossRef.
- X. Dou, Q. Zhou, X. Chen, Y. Tan, X. He, P. Lu, K. Sui, B. Z. Tang, Y. Zhang and W. Z. Yuan, Biomacromolecules, 2018, 19, 2014–2022 CrossRef CAS.
- J. Liu and Y. Qian, Dyes Pigm., 2017, 136, 782–790 CrossRef CAS.
- R. Krämer, Angew. Chem., Int. Ed., 1998, 37, 772–773 CrossRef.
- L. Lan, Q. Niu, Z. Guo, H. Liu and T. Li, Sens. Actuators, B, 2017, 244, 500–508 CrossRef CAS.
Footnote |
† Electronic supplementary information (ESI) available: UV spectra, XRD and the mean diameter. See DOI: 10.1039/c8ra06178e |
|
This journal is © The Royal Society of Chemistry 2018 |
Click here to see how this site uses Cookies. View our privacy policy here.