DOI:
10.1039/C7RA07992C
(Paper)
RSC Adv., 2017,
7, 47547-47551
Mercaptooxazole–phenazine based blue fluorescent sensor for the ultra-sensitive detection of mercury(II) ions in aqueous solution†
Received
20th July 2017
, Accepted 25th September 2017
First published on 10th October 2017
Abstract
Herein, a mercury(II) ion fluorescent sensor (Z-3) with high sensitivity and immediate response is designed and synthesized. The sensor uses the phenazine group as a luminophore and sulfhydryl as a recognition moiety. The sensor is easily synthesized and it exhibits a remarkable blue shift with Hg2+. Correspondingly, its fluorescence color changes from yellow to blue. In addition, the low naked eye detection limit (10−5) of the sensor allows the identification of concentration limits. Moreover, the sensor could detect mercury(II) ions over a wide pH range (from 2 to 8), which indicates that the detection can be carried out in aqueous systems. In addition, test strips are fabricated, which could act as a convenient pathway for the recognition of Hg2+.
Introduction
Mercury plays an important role in electrical and chemical applications.1–3 Its electrical applications include thermometers, barometers, sphygmomanometers and other related instruments. In the chemical industry, mercury is used to electrolyze a salt for the preparation of high purity chlorine and caustic soda.4–11 However, excessive consumption of mercury can lead to irreversible damage to the environment and human body.12–15 As a toxic metal, mercury and its compound could cause vital organ dysfunction such as DNA damage,16 mitosis impairment,17 and nervous system defects.18 The emission of mercury ions has been increasing due to the full speed development of the mercury industry.19,20 Mercury ions enter the body through the food chain and accumulate gradually, which directly affects the safety of human life. To date, many sensors have been reported for the detection of Hg2+. However, some sensors are made by complex synthesis processes and expensive raw materials or are difficult to synthesize,21–24 and thus their practical applications are limited in certain aspects. Due to their rapid testing, strong practicality and easy operation, colorimetric and fluorescent sensors are the best choice for the detection of mercury(II) ions in physiological and environmental samples.25–29
Among the reported sensors, phenazine and its derivatives have been widely employed in the design of fluorescent sensors due to their stable optical properties, easy synthesis, cheap raw materials and large conjugate systems.30–32 They act as an important skeleton in fluorescence sensors used to identify amino acids, citrate and other ions.33–35
According to these superior properties and our goals,36 we designed and synthesized a phenazine derivative Z-3 (Scheme 1), which could rapidly select Hg2+ in aqueous solutions. Based on its deprotonation mechanism, the fluorescent sensor could detect Hg2+ with blue fluorescence in a wide pH range (from 2–8). It also exhibits high selectivity (2.14 × 10−9 M) and other common metal ions have nearly no influence on its sensing behavior. Moreover, its naked eye minimum detection limits are 10−5 and 10−4 for solution and test strips, respectively, which greatly improve the practicality of this sensor. Moreover, the test strips also can act as a reliable means for the detection of Hg2+ in real-time.
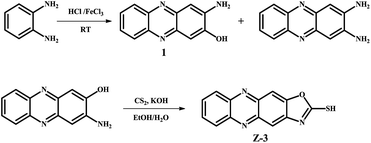 |
| Scheme 1 Synthesis of compound Z-3. | |
Fluorescence spectra were measured with different metal ions in DMSO/H2O (v/v, 3
:
2, buffered with HEPES, pH = 7.20). As shown in Fig. 1a, the addition of Hg2+ induced a distinct fluorescence enhancement with an obvious blue-shift (from 544 to 496 nm). Moreover, the fluorescence color of the solutions changed from yellow to blue under illumination with a 365 nm UV lamp (Fig. 1b). We speculated that the sensor complexed with Hg2+, which resulted in the fluorescence colour change. All these facts indicate that the sensor could be used for the selective identification of Hg2+ among other cations.
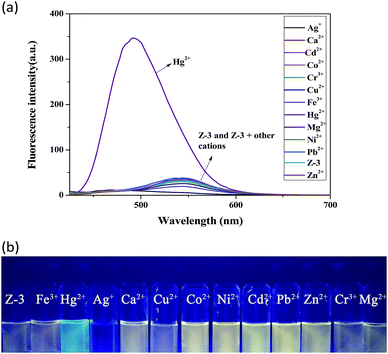 |
| Fig. 1 (a) Fluorescence spectra (λex = 400 nm) of Z-3 (2 × 10–5 M) with different metal ions: Fe3+, Hg2+, Ag+, Ca2+, Cu2+, Co2+, Ni2+, Cd2+, Pb2+, Zn2+, Cr3+ and Mg2+ (10 equiv., respectively) in DMSO/H2O (v/v, 3 : 2; buffered with HEPES, pH = 7.20). (b) Visual fluorescence emission of the Z-3 sensor upon the addition of different metal ions. | |
The most important aspect of selective recognition is that the target analyte is detected over other possible interfering metal ions, and thus a competition experiment was carried out. In Fig. 2a and b, in the presence of other metal ions, such as Fe3+, Ag+, Ca2+, Cu2+, Co2+, Ni2+, Cd2+, Pb2+, Zn2+, Cr3+ and Mg2+, there was no evident fluorescence emission peak shift and color change. Therefore, it is clear that the interference of other ions is negligible during the detection of Hg2+. These results further suggest that Z-3 has a strong ability to complex with Hg2+ over other metal ions and could be used as a sensor for Hg2+ over a wide range of cations.
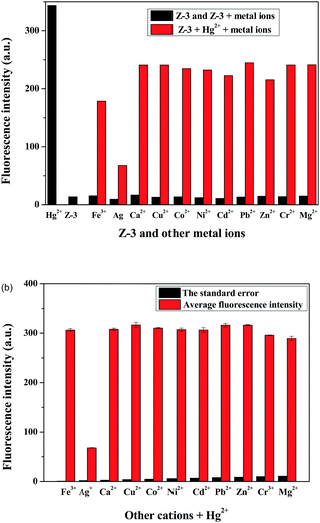 |
| Fig. 2 (a) Fluorescence spectra of Z-3 and Z-3 + Hg2+ in the presence of 10 equiv. various metal ions in DMSO/H2O (v/v, 3 : 2; buffered with HEPES, pH = 7.20) solutions; (b) error bar of Z-3 + Hg2+ in the presence of various cations. | |
We also performed a fluorescence titration to verify the recognition behavior sensitivity (Fig. 3). In the fluorescence spectrum, upon the addition of Hg2+ to Z-3, the emission peak gradually blue shifted from 544 nm to 496 nm with a fluorescence color change from yellow to blue. The detection of Z-3 for Hg2+ calculated on the basis of 3σ/m for the fluorescence spectra (Fig. S5†) is 2.14 × 10−9 M,37 which is lower than the EPA guideline of 10 nM.38 This result also shows that Z-3 has higher sensitivity for Hg2+ compared with other reported Hg2+ sensors (Table 1).
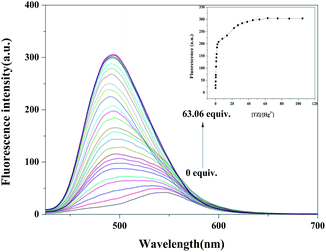 |
| Fig. 3 Fluorescence spectra of Z-3 with different concentrations of Hg2+ in DMSO/H2O (v/v, 3 : 2; buffered with HEPES, pH = 7.20) solutions. Insert: plot of fluorescence intensity vs. concentration of Hg2+ in the range of 0 to 63.06 equiv. (λex = 400 nm). | |
Table 1 A comparison of the detection limits for Hg2+
Method |
Analyte |
Solvent |
Detection limit |
Ref. 39
|
Hg2+ |
1-Propanol-cyclohexane |
1.7 × 10−8 M |
Ref. 40
|
Hg2+ |
MeOH–H2O |
2.1 × 10−7 M |
Ref. 41
|
Hg2+ |
THF-HEPES |
8 × 10−8 M |
Ref. 42
|
Hg2+ |
CH3CN–H2O |
9 × 10−8 M |
Our work |
Hg2+ |
DMSO–HEPES |
2.14 × 10−8 M |
In order to improve the actual operating performance of the detection behavior, we studied the pH scope of Z-3 + Hg2+ and fabricated comparison test strips. As shown in Fig. 4, Z-3 with Hg2+ exhibits strong fluorescence intensity in the pH range of 2–8, while the fluorescence intensity increases obviously at pH > 8, which may result from the fact that too high pH will result in a reaction between Hg2+ and –OH to form the corresponding metal hydroxides. This fact indicates that the sensor could be used in a wide pH range from 2 to 8 for the detection of Hg2+. Test strips were prepared by immersing filter paper into a DMSO solution of Z-3 (2 × 10−4 M) and then drying them in air. The test strips containing Z-3 were utilized to sense Hg2+. As shown in Fig. 5, with a decrease in the Hg2+ concentration, the test paper fluorescence color gradually becomes yellow. The minimum identification limit of the test strips is 10−4 M. Moreover, the test strips could act as a convenient method for the detection of Hg2+ in an aqueous solution. We also conducted the experiments with the minimum detection limit with respect to naked eyes. As shown in Fig. 6, when the concentration of Hg2+ is 10−6 M, the fluorescence color of the solution is consistent with the sensor (Z-3). We thought that the lowest detection limit of Hg2+ was 10−5 M.
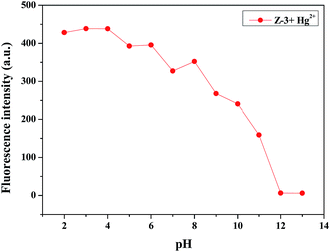 |
| Fig. 4 Fluorescence intensity (λex = 400 nm and λem = 496 nm) of the Z-3 + Hg2+ complex at various pH values in DMSO/H2O (v/v, 3 : 2; buffered with HEPES, pH = 7.20) solution. | |
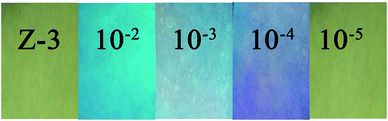 |
| Fig. 5 Photographs of test strips (the concentration of Hg2+ is 0 M, 10−1 M, 10−2 M, 10−3 M, 10−4 M, 10−5 M, both under an UV lamp at 365 nm). | |
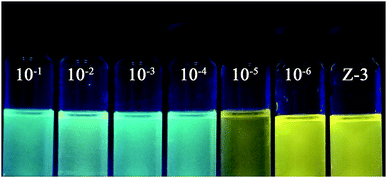 |
| Fig. 6 Naked-eyes detection limit under UV lamp at 365 nm.from left to right, the concentration of Hg2+ is 10−1 M, 10−2 M, 10−3 M, 10−4 M, 10−5 M, 10−6 and 0 M. | |
In order to study the combination of mercury ions(II) with Z-3, we first studied the isomerization of Z-3. As shown in Fig. S1,† the peak at 14.41 ppm is ascribed to the –NH proton in the 1H NMR spectrum. Moreover, we also observed a peak at 2675 cm−1 in the IR spectrum (Fig. S2†), which is the characteristic peak of –SH. Based on these facts, we believe that Z-3 has two tautomers of thioketone (
S) and thiols (–SH), and the structure of thione (
S) is the main form in solution. From the 1H NMR titration (Fig. 7), the peak at 14.41 ppm disappeared with the addition of Hg2+, which suggests that the complexation occurred after the –NH proton was fully deprotonated. Amazingly, the solution precipitated solid on the addition of 0.2 equivalents of mercury ions. We speculate that it may be from the perchlorate, and its molecular formula may be [Z-3 + Hg]+ClO4−. In addition, we also carried out an IR experiment on the solid of Z-3 and Z-3 + Hg2+. As shown in Fig. S3,† the peak at 2675 cm−1, which was recognized as the characteristic peak of –SH, disappeared upon the addition of Hg2+. All these results indicate that the mercury ions coordinated with the sulfur atom and nitrogen atom on the oxazole ring after the protonation of the sensor.
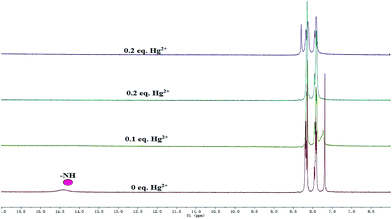 |
| Fig. 7 Partial 1H NMR spectra of Z-3 in DMSO-d6 with different concentrations of Hg2+. | |
Moreover, in order to clarify the stoichiometric relationship between Hg2+ and Z-3, the Job plot was obtained. As shown in Fig. 8, the fluorescence intensity at 496 nm was plotted against molar fraction of Z-3 sensor. The maximum emission intensity was reached when the molar fraction was 0.4. This result manifests a 1
:
1 ratio for the Z-3 + Hg2+ complex.
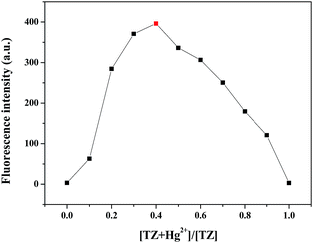 |
| Fig. 8 Job plot examining the relationship between Z-3 and Hg2+. | |
Based on all these facts, we propose a reasonable mechanism for the detection of Hg2+ by the sensor in an aqueous solution (Fig. 9). We hypothesize that the thiocarbonyl and secondary amine nitrogen of the oxazole motif coordinate with Hg2+, and then the complex and perchloric acid form a salt and precipitate.
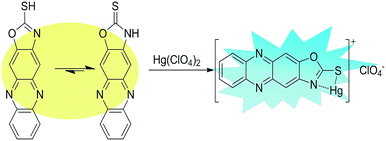 |
| Fig. 9 Possible binding mode of Z-3 with Hg2+. | |
In conclusion, we obtained a fluorescent sensor Z-3via a simple method for the detection of Hg2+, which displays high selectivity and sensitivity in the recognition process. The sensor exhibits an obvious fluorescence colour change from yellow to blue, and its detection behaviour operates in a wide pH range. Moreover, its test strips and naked-eyes minimum detection limit greatly improve the practicality of Z-3. Hence, this study also implies that phenazine and its derivatives are potential candidates for the detection of ions.
Conflicts of interest
There are no conflicts to declare.
Acknowledgements
This study was supported by the National Natural Science Foundation of China (NSFC) (No. 21662031; 21661028; 21574104; 21262032), the Natural Science Foundation of Gansu Province (1506RJZA273) and the Program for Changjiang Scholars and Innovative Research Team in University of Ministry of Education of China (IRT 15R56).
Notes and references
- C. Caltagirone and P. A. Gale, Chem. Soc. Rev., 2009, 38, 520 RSC.
- R. Zare-Dorabei, R. Rahimi, A. Koohi and S. Zargari, RSC Adv., 2015, 5, 93310 RSC.
- D. G. Streets, M. K. Devane, Z. Lu, T. C. Bond, E. M. Sunderland and D. J. Jacob, Environ. Sci. Technol., 2011, 45, 10485 CrossRef CAS.
- L. Wang, J. Jin, L. Zhao, H. Shen, C. Shen and P. Zhang, Carbohydrate Research, 2016, 433, 41 CrossRef CAS.
- K. P. Carter, A. M. Young and A. E. Palmer, Chem. Rev., 2014, 114, 4564 CrossRef CAS.
- J. Kuchlyan, S. Basak, D. Dutta, A. K. Das and D. Ma, Chem. Phys. Lett., 2017, 673, 84 CrossRef CAS.
- T. Yu, T. T. Zhang, W. Zhao, J. J. Xu and H. Y. Chen, Talanta, 2017, 165, 570 CrossRef CAS.
- N. Busschaert, C. Caltagirone, W. V. Rossom and P. A. Gale, Chem. Rev., 2015, 115, 8038 CrossRef CAS PubMed.
- C. T. Driscoll, R. P. Mason, H. M. Chan, D. J. Jacob and N. Pirrone, Environ. Sci. Technol., 2013, 47, 4967 CrossRef CAS PubMed.
- H. H. Wang, L. Xue, C. L. Yu, Y. Y. Qian and H. Jiang, Dyes Pigm., 2011, 91, 350 CrossRef CAS.
-
(a) L. Campbell, D. G. Dixon and R. E. Hecky, J. Toxicol. Environ. Health, Part B, 2003, 6, 325 CrossRef CAS;
(b) Q. Ye, F. Yan, Y. Luo, Y. Wang, X. Zhou and L. Chen, Spectrochim. Acta, Part A, 2017, 173, 854 CrossRef CAS;
(c) C. Jiang, Z. Guan, S. Y. Rachel Lim, L. Polavarapu and Q. H. Xu, Nanoscale, 2011, 3, 3316 RSC;
(d) Y. Chen, L. Wu, Y. Chen, N. Bi, X. Zheng, H. Qi and M. Qin, Microchim. Acta, 2012, 177, 341 CrossRef CAS.
-
(a) Y. Li, H. Huang, Y. Li, X. Su, Y. Li, H. Huang, Y. Li and X. Su, Sens. Actuators, B, 2013, 188, 772 CrossRef CAS;
(b) W. Ren, C. Zhu and E. Wang, Nanoscale, 2012, 4, 5902 RSC;
(c) E. O. Ganbold, J. H. Park, K. S. Ock and S. W. Joo, Bull. Korean Chem. Soc., 2011, 32, 519 CrossRef CAS.
- C. Chen, R. Wang, L. Guo, N. Fu, H. Dong and Y. Yuan, Org. Lett., 2011, 13, 1162 CrossRef CAS.
- H. H. Harris, I. J. Pickering and G. N. George, Science, 2003, 301 Search PubMed.
- P. B. Tchounwou, W. K. Ayensu, N. Ninashvili and D. Sutton, Environ. Toxicol., 2003, 18, 149 CrossRef CAS.
- C. Wu, J. Wang, J. Shen, C. Bi and H. Zhou, Sens. Actuators, B, 2017, 243, 678 CrossRef CAS.
- I. Samb, J. Bell, P. Y. Toullec, V. Michelet and I. Leray, Org. Lett., 2011, 13, 1182 CrossRef CAS.
- Y. Ding, W. Zhu, Y. Xu and X. Qian, Sens. Actuators, B, 2015, 220, 762 CrossRef CAS.
- X. Ma, J. Wang, Q. L. Shan, Z. W. Tan, G. H. Wei, D. B. Wei and Y. G. A. Du, Org. Lett., 2012, 14, 820 CrossRef CAS PubMed.
-
(a) Z. S. Qian, X. Y. Shan, L. J. Chai, J. J. Ma, J. R. Chen and H. Feng, ACS Appl. Mater. Interfaces, 2014, 6, 6797 CrossRef CAS;
(b) P. F. Wei, D. B. Li, B. B. Shi, Q. Wang and F. Huang, Chem. Commun., 2015, 51, 15169 RSC;
(c) C. H. Yao, Y. H. Cao, Q. Wang, Y. Pan, J. L. Jiang and L. Y. Wang, Chem. Commun., 2016, 52, 8715 RSC;
(d) C. Jin, M. Zhang, L. Wu, Y. F. Guan, Y. Pan, J. L. Jiang, C. Lin and L. Y. Wang, Chem. Commun., 2013, 49, 2015 Search PubMed.
- X. F. Wu, Q. J. Ma, X. J. Wei, Y. M. Hou and X. Zhu, Sens. Actuators, B, 2013, 183, 565 CrossRef CAS.
- Z. Chen, T. Lou, Q. Wu, K. Li, L. Tan and J. Sun, Sens. Actuators, B, 2015, 221, 36 Search PubMed.
- P. Jarujamrus, M. Amatatongchai, A. Thima, T. Khongrangdee and C. Mongkontong, Spectrochim. Acta, Part A, 2015, 142, 86 CrossRef CAS.
- M. Taki, K. Akaoka, S. Iyoshi and Y. Yamamoto, Inorg. Chem., 2012, 51, 13075 CrossRef CAS.
-
(a) L. Y. Wang, L. H. Zhu and D. Cao, New J. Chem., 2015, 39, 7211 RSC;
(b) X. Y. Hu, X. Wu, Q. Duan, T. Xiao, C. Lin and L. Wang, Org. Lett., 2012, 14, 4826 CrossRef CAS;
(c) M. Xue, Y. Yang, X. Chi, Z. Zhang and F. Huang, Acc. Chem. Res., 2012, 45, 1294 CrossRef CAS PubMed;
(d) L. Y. Wang, X. G. Chen and D. Cao, RSC Adv., 2016, 6, 96676 RSC.
-
(a) W. Ren, Y. Zhang, H. G. Chen, H. Z. F. Gao, N. B. Li and H. Q. Luo, Anal. Chem., 2016, 88, 1385 CrossRef CAS;
(b) K. Johari, N. Saman, S. T. Song, S. C. Cheu, H. Kong and H. Mat, Chemosphere, 2016, 156, 56 CrossRef CAS;
(c) S. Sun, X. Y. Hu, D. Chen, J. Shi, Y. Dong, C. Lin, Y. Pan and L. Wang, Polym. Chem., 2013, 4, 2224 RSC;
(d) Y. Chen, M. He, B. Li, L. Wang, H. Meier and D. Cao, RSC Adv., 2013, 3, 21405 RSC.
- E. M. Nolan and S. J. Lippard, Chem. Rev., 2008, 108, 3443 CrossRef CAS.
- D. T. Quang and J. S. Kim, Chem. Rev., 2010, 110, 6280 CrossRef CAS.
- C. Zhu, L. Li, F. Fang, J. Chen and Y. Wu, Chem. Lett., 2005, 34, 898 CrossRef CAS.
-
(a) D. C. Lee, B. Cao, K. Jang and P. M. Forster, J. Mater. Chem., 2010, 20, 867 RSC;
(b) V. S. S. Mosali, G. A. Bowmaker, M. Gerard, P. A. Kilmartin, J. T. Sejdicb and Z. D. Zujovicb, Polym. Int., 2015, 64, 1135 CrossRef CAS;
(c) S. Biradar, Y. Shigemitsu, Y. Kubota, K. Funabiki, H. Satoc and M. Matsui, RSC Adv., 2014, 4, 59387 RSC;
(d) J. F. Zhao, Y. Liu, J. B. Soh, Y. X. Li, R. Ganguly, K. Q. Ye, F. Huo, L. Huang, A. I. Y. Tok, J. S. C. Loo and Q. Zhang, Tetrahedron Lett., 2012, 53, 6044 CrossRef CAS;
(e) J. Zhao, G. Li, C. Wang, W. Chen, S. C. J. Loo and Q. Zhang, RSC Adv., 2013, 3, 9653 RSC.
- C. J. Tonzola, M. M. Alam, W. Kaminsky and S. A. Jenekhe, J. Am. Chem. Soc., 2003, 125, 13548 CrossRef CAS.
-
(a) F. J. Huo, J. Su, Y. Q. Sun, C. X. Yin, J and B. Chao, Chem. Lett., 2010, 39, 738 CrossRef CAS;
(b) Y. A. Son, Y. M. Park, S. Y. Park, C. J. Shin and S. H. Kim, Dyes Pigm., 2007, 73, 76 CrossRef CAS;
(c) J. Xu, S. B. Sun, Q. Li, Y. Yue, Y. D. Li and S. J. Shao, Anal. Chim. Acta, 2014, 849, 36 CrossRef CAS;
(d) F. J. Huo, C. X. Yin, Y. T. Yang, J. Su, J. B. Chao and D. S. Liu, Anal. Chem., 2012, 84, 2219 CrossRef CAS;
(e) S. Erdemir and O. Kocyigit, Sens. Actuators, B, 2015, 221, 900 CrossRef CAS;
(f) C. J. Gao, X. J. Jin, X. H. Yan, P. An, Y. Zhang, L. L. Liu, H. Tian, W. S. Liu, X. J. Yao and Y. Tang, Sens. Actuators, B, 2013, 176, 775 CrossRef CAS.
- X. Zhang, Y. Yan, Y. Hang, J. Wang, J. Hua and H. Tian, Chem. Commun., 2017, 53, 5760 RSC.
- Y. Gao, G. Huang, Z. Ou, Z. Wang, B. Ju, Y. Li, X. Wang and S. Yin, New J. Chem., 2015, 39, 8948 RSC.
-
(a) P. Y. Gu, Z. Wang and Q. Zhang, J. Mater. Chem., 2016, 4, 7060 RSC;
(b) J. Li, J. Gao, W. W. Xiong, P. Z. Li, H. Zhang, Y. Zhao and Q. Zhang, Chem.–Asian J., 2014, 9, 121 CrossRef CAS;
(c) J. F. Zhao, Y. Liu, J. B. Soh, Y. X. Li, R. Ganguly, K. Q. Ye, F. Huo, L. Huang, A. I. Y. Tok, J. S. C. Loo and Q. Zhang, Tetrahedron Lett., 2012, 53, 6044 CrossRef CAS.
-
(a) W. T. Li, G. Y. Wu, W. J. Qu, Q. Li, J. C. Lou, Q. Lin, H. Yao, Y. M. Zhang and T. B. Wei, Sens. Actuators, B, 2017, 239, 671 CrossRef CAS;
(b) H. L. Zhang, T. B. Wei, W. T. Li, W. J. Qu, Y. L. Leng, J. H. Zhang, Q. Lin, Y. M. Zhang and H. Yao, Spectrochim. Acta, Part A, 2017, 175, 117 CrossRef CAS PubMed.
-
Analytical Methods Committee, Analyst 1987, 112, 199 Search PubMed.
- H. F. Wang and S. P. Wu, Tetrahedron, 2013, 69, 1965 CrossRef CAS.
- N. A. Bumagina, E. V. Antina and D. I. Sozonov, J. Lumin., 2017, 183, 315 CrossRef CAS.
- Y. Lei, H. Li, X. Huang, J. Chen, M. Liu, W. Gao, J. Ding, D. Lin and H. Wu, Tetrahedron, 2015, 71, 3453 CrossRef CAS.
- M. Kaur, M. J. Cho and D. H. Choi, Dyes Pigm., 2016, 125, 1 CrossRef CAS.
- I. Samb, J. Bell, P. Y. Toullec, V. Michelet and I. Leray, Org. Lett., 2011, 13, 1182 CrossRef CAS.
Footnote |
† Electronic supplementary information (ESI) available: Experimental details, synthesis of M2, NMR spectra, and other materials. See DOI: 10.1039/c7ra07992c |
|
This journal is © The Royal Society of Chemistry 2017 |