DOI:
10.1039/C6QO00060F
(Research Article)
Org. Chem. Front., 2016,
3, 574-578
Generation of N-aminosulfonamides via a photo-induced fixation of sulfur dioxide into aryl/alkyl halides†
Received
6th February 2016
, Accepted 3rd March 2016
First published on 3rd March 2016
Abstract
A catalyst-free aminosulfonylation through insertion of sulfur dioxide with aryl/alkyl halides enabled by photoenergy is presented. Under ultraviolet irradiation, a three-component reaction of aryl/alkyl halides, sulfur dioxide, and hydrazines proceeds smoothly under mild conditions without any metals or photo-redox catalysts. The corresponding N-aminosulfonamides are afforded in good to excellent yields. A broad reaction scope is demonstrated with good functional group tolerance. Not only aryl bromide but also aryl chloride is suitable under the conditions. Additionally, alkyl halides are good substrates as well during the insertion of sulfur dioxide. A possible mechanism involving a radical process is proposed, supported by theoretical calculations.
Introduction
In the last decade, visible-light photocatalysis has attracted continuous interest with the expectation of designing novel transformations proceeding under mild reaction conditions through reactive radical or ionic species.1 For example, MacMillan and co-workers described an efficient carbon–oxygen coupling reaction of alcohols and aryl bromides in the presence of photoredox and nickel catalysis, and further discovered that alcohols could act as mild alkylating reagents in heteroarene C–H bond functionalization.2 Xiao and co-workers reported an efficient route to 4,5-dihydropyrazoles, and the photocatalytic generation of the N-centered hydrazonyl radical was demonstrated as the key step.3 During the reaction, photocatalysis was crucial for the successful conversion. On the other hand, transition-metal-free reactions enabled by photoenergy have been discovered recently. For instance, Murakami developed a clean method for carboxylation of o-alkylphenyl ketones with CO2 under UV light or even solar light.4 Li described a transition-metal-free coupling between aryl alkyne and alkyl iodide in water under ultraviolet irradiation.5a In the meantime, a photo-induced halogen exchange in aryl or vinyl halides was observed under metal-free conditions.5b Very recently, an efficient process for the arylation of pyrimidine derivatives under moderate energetic irradiation conditions was reported by Goddard.6 During the reaction processes, the generation of alkyl or aryl radicals from alkyl/aryl halides enabled by photoenergy was believed to be a key point. These results are interesting and promising, since photocatalysis is not necessary and other photo-induced couplings of aryl/alkyl halides under metal-free conditions would be expected.
Recently, much attention has been paid to the insertion of sulfur dioxide, which could easily introduce the sulfonyl group into small organic molecules.7–10 Most of the procedures involved the transition-metal-catalyzed couplings of aryl iodides or bromides with the insertion of sulfur dioxide (Scheme 1, eqn (1)). For example, Willis and co-workers reported a palladium-catalyzed coupling reaction of aryl iodides, sulfur dioxide, and hydrazines.7a Since aryl halides are easily available and the sulfonamide or sulfone is an important group due to its widespread presence in top-selling pharmaceuticals,11 the metal-free reaction of aryl halides with sulfur dioxide would provide a green and more efficient pathway to produce sulfonyl compounds. In 2014, we developed a three-component reaction of sulfur dioxide, aryldiazonium salt and hydrazines.9c The transformation proceeded through a radical process. Although the conversion was efficient, the aryldiazonium salts which had to be prepared from the corresponding anilines were unstable and unsuitable for storage.
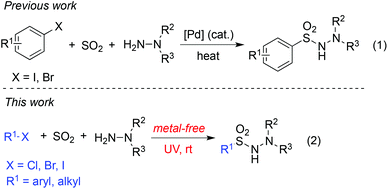 |
| Scheme 1 Aminosulfonylation of aryl/alkyl halides, sulfur dioxide, and hydrazines. | |
Inspired by the photo-induced metal-free couplings of abundant aryl/alkyl halides,5 we envisioned that an aryl/alkyl radical could be generated as well in the metal-free aminosulfonylation through insertion of sulfur dioxide with aryl/alkyl halides under ultraviolet irradiation (Scheme 1, eqn (2)). It would be anticipated that not only aryl bromide but also aryl chloride would be suitable under the conditions. Additionally, alkyl halides could be expected as good substrates as well during the insertion of sulfur dioxide. Thus, β-H elimination would be evitable compared with the transition-metal-catalyzed process. We therefore, initiated a program to explore the photo-induced metal-free aminosulfonylation of aryl/alkyl halides, sulfur dioxide, and hydrazines under mild conditions.
Results and discussion
The reaction of 1-bromo-4-methoxybenzene 1a, sulfur dioxide, and morpholin-4-amine 2a was selected as the model (Table 1). The reaction was performed at 25 °C under ultraviolet irradiation (mercury lamp) at an intensity of 0.67 W cm−2 by using a standard photo-reactor (see the ESI†). Initially, the reaction was evaluated in different solvents. To our delight, the desired product 3a was generated in 32% yield when the reaction occurred in MeCN (Table 1, entry 1). Only a trace amount of the product was detected when 1,4-dioxane was used as the solvent (Table 1, entry 2). The yield was lower (19%) when the reaction was performed in methanol (Table 1, entry 3). From the results, it seemed that the solvent was critical for this transformation, which was similar to Li's report.5 We further examined the effect of additives. No better result was obtained when DABCO, potassium carbonate, or sodium carbonate was added to the reaction (Table 1, entries 4–6). Interestingly, the yield was improved when TBAB (tetrabutylammonium bromide) was present in the reaction (Table 1, entry 7). However, the reaction failed to afford the desired product when TBAC (tetrabutylammonium chloride) was used as a replacement (Table 1, entry 8). The result was dramatically increased in the presence of TBAI (tetrabutylammonium iodide), giving rise to the corresponding product 3a in 73% yield (Table 1, entry 9).
Table 1 Initial studies for the photo-induced metal-free aminosulfonylation of 1-bromo-4-methoxybenzene 1a, sulfur dioxide, and morpholin-4-amine 2a
a

|
Entry |
Additive |
Solvent |
Yieldb (%) |
Reaction conditions: 1-bromo-4-methoxybenzene 1a (0.2 mmol), morpholin-4-amine (1.5 equiv.), DABSO (0.8 equiv.), solvent (4.0 mL), additive (1.5 equiv.).
Isolated yield based on 1-bromo-4-methoxybenzene 1a.
|
1 |
— |
MeCN |
32 |
2 |
— |
1,4-Dioxane |
Trace |
3 |
— |
MeOH |
19 |
4 |
DABCO |
MeCN |
32 |
5 |
K2CO3 |
MeCN |
25 |
6 |
Na2CO3 |
MeCN |
28 |
7 |
TBAB |
MeCN |
48 |
8 |
TBAC |
MeCN |
Trace |
9 |
TBAI |
MeCN |
73 |
With the above optimal reaction conditions in hand, we then explored the reaction scope of this photo-induced metal-free aminosulfonylation of aryl halides, sulfur dioxide, and hydrazines (Table 2). It was found that all reactions of aryl bromides proceeded smoothly, leading to the desired products 3 in moderate to good yields. Different functional groups including fluoro, cyano, and hydroxy were tolerated under the conditions. For example, the reaction of 1-bromo-4-hydroxybenzene, sulfur dioxide, and morpholin-4-amine 2a furnished the corresponding product 3l in 67% yield. It was noteworthy that the reaction of 2-bromo-1,3,5-trimethylbenzene worked efficiently as well, which produced the desired product 3h in 54% yield. It seemed that steric hindrance did not retard the reaction. Other hydrazines were examined in the meantime, and the products obtained as expected. Further exploration revealed that not only aryl iodide was effective in the photo-induced metal-free aminosulfonylation, but also aryl chloride was a good substrate under the standard conditions. Chlorobenzene 5 reacted with sulfur dioxide and morpholin-4-amine 2a delivered the desired product 3f in 51% yield.
Table 2 Photo-induced metal-free aminosulfonylation of aryl halides, sulfur dioxide, and hydrazinesa
Isolated yield based on aryl halide.
|
|
The photo-induced metal-free aminosulfonylation of alkyl halides, sulfur dioxide, and hydrazines was further investigated (Table 3). As expected, the insertion of sulfur dioxide with alkyl halides worked well, giving rise to the desired products 7 in good to excellent yields. So far, there are no reports for transition-metal-catalyzed insertion of sulfur dioxide with alkyl halides. This photo-induced procedure could open a new avenue for the aminosulfonylation of alkyl halides.
Table 3 Photo-induced metal-free aminosulfonylation of alkyl halides, sulfur dioxide, and hydrazinesa
Isolated yield based on alkyl halide.
|
|
Preliminary mechanistic investigation was carried out to understand the process of this transformation. As mentioned above, the generation of alkyl or aryl radicals from alkyl/aryl halides enabled by photoenergy has been demonstrated.5 Additionally, we have disclosed a radical process for the insertion of sulfur dioxide with aryldiazonium salt.9c Inspired by these results, we believed that the photo-induced metal-free aminosulfonylation of aryl/alkyl halides, sulfur dioxide, and hydrazines would proceed through a radical process as well. As anticipated, when 2,2,6,6-tetramethyl-1-piperidinyloxy (TEMPO) was added to the photo-induced metal-free aminosulfonylation of bromobenzene 1f, sulfur dioxide, and morpholin-4-amine 2a, no desired product (3f) was detected (Scheme 2, eqn (a)). When substrate 8 was used in the reaction of sulfur dioxide and morpholin-4-amine 2a (Scheme 2, eqn (b)), compound 9 was obtained in 76% yield, which indicated a possible radical process.
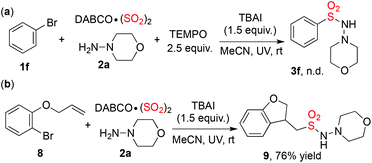 |
| Scheme 2 Investigation of the mechanism. | |
On the basis of the experimental results and previous reports,5,9c we did the theoretical calculations (Fig. 1) and proposed a possible route for the photo-induced metal-free aminosulfonylation reaction (Scheme 3). We reasoned that the hydrazine–SO2 complex A would be generated by a barrierless exchange of sulfur dioxide between DABCO·(SO2)2 and morpholin-4-amine 2a. Subsequently, the phenyl radical generated via ultraviolet irradiation from bromobenzene would attack the hydrazine–SO2 complex A, leading to intermediate Bvia the transition state TS1 with a free-energy barrier of 9.7 kcal mol−1. Subsequent formation of radical C would occur with the release of morpholin-4-amine 2a. Another possible pathway affording the radical intermediate C could not be excluded, which proceeded through the addition of the phenyl radical to sulfur dioxide directly. However, according to theoretical calculations, the Gibbs free-energy barrier of this route to form radical C is 13.4 kcal mol−1 (higher than the above pathway of 9.7 kcal mol−1, see the ESI†), which indicated that this process was less favored compared to the above pathway. Subsequently, the bromine radical produced along with the formation of the aryl radical would integrate with morpholin-4-amine 2a to form the intermediate D. Deprotonation via the transition state TS3 would produce radical E, which was found to be the rate-determining step and proceeded with a free-energy barrier of 21.3 kcal mol−1. Finally, radical E would combine with intermediate C to afford the desired product 3f rapidly. Additionally, theoretical calculations showed that the Gibbs free-energy barrier of the rate-determining step was 39.8 kcal mol−1 when hydrazine was replaced by aniline as a representative of simple amines (the Gibbs free-energy profiles were provided in the ESI†). This theoretical calculation result might explain why only hydrazines were efficient and amines were not suitable in this transformation.
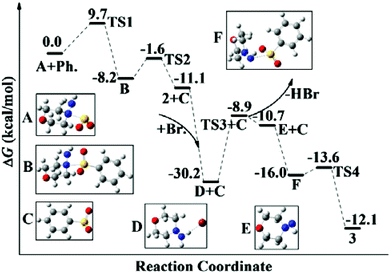 |
| Fig. 1 Free-energy profiles in acetonitrile for the preferred reaction pathway at 298 K calculated by the M06-2x/6-31+G(d,p) method. | |
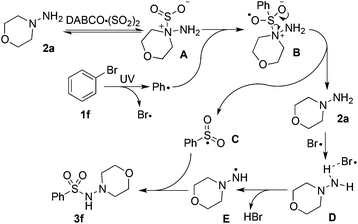 |
| Scheme 3 A possible mechanism for the photo-induced metal-free aminosulfonylation of aryl/alkyl halides, sulfur dioxide, and hydrazines. | |
Conclusions
In summary, we have developed a catalyst-free aminosulfonylation through insertion of sulfur dioxide with aryl/alkyl halides enabled by photoenergy for the first time. Under ultraviolet irradiation, a three-component reaction of aryl/alkyl halides, sulfur dioxide, and hydrazines proceeds smoothly under mild conditions without any metals or photo-redox catalysts. The broad reaction scope is demonstrated with good functional group tolerance. Not only aryl bromide but also aryl chloride is suitable under the conditions. Additionally, alkyl halides are good substrates as well during the insertion of sulfur dioxide. A possible mechanism involving a radical process is proposed, supported by theoretical calculations.
Acknowledgements
Financial support from the National Natural Science Foundation of China (no. 21372046, 21532001, 21273042, and 21573044) is gratefully acknowledged. We thank the Super Computer Center of Fudan University for computer time.
Notes and references
- For reviews, see:
(a) T. P. Yoon, M. A. Ischay and J. Du, Nat. Chem., 2010, 2, 527 CrossRef CAS PubMed;
(b) F. Teplý, Collect. Czech. Chem. Commun., 2011, 76, 859 CrossRef;
(c) J. M. R. Narayanam and C. R. J. Stephenson, Chem. Soc. Rev., 2011, 40, 102 RSC;
(d) J. Xuan and W.-J. Xiao, Angew. Chem., Int. Ed., 2012, 51, 6828 CrossRef CAS PubMed;
(e) L. Shi and W.-J. Xia, Chem. Soc. Rev., 2012, 41, 7687 RSC;
(f) Y.-M. Xi, H. Yi and A.-W. Lei, Org. Biomol. Chem., 2013, 11, 2387 RSC;
(g) C. K. Prier, D. A. Rankic and D. W. C. MacMillan, Chem. Rev., 2013, 113, 5322 CrossRef CAS PubMed;
(h) D. M. Schultz and T. P. Yoon, Science, 2014, 343, 985 CrossRef CAS PubMed;
(i) M. N. Hopkinson, B. Sahoo, J.-L. Li and F. Glorius, Chem. – Eur. J., 2014, 20, 3874 CrossRef CAS PubMed;
(j) J. Xuan, Z.-G. Zhang and W.-J. Xiao, Angew. Chem., Int. Ed., 2015, 54, 15632 CrossRef CAS PubMed;
(k) J.-R. Chen, X.-Q. Hu, L.-Q. Lu and W.-J. Xiao, Chem. Soc. Rev., 2016 10.1039/C5CS00655D.
-
(a) J. A. Terrett, J. D. Cuthbertson, V. W. Shurtleff and D. W. C. MacMillan, Nature, 2015, 524, 330 CrossRef CAS PubMed;
(b) C. C. Nawrat, C. R. Jamison, Y. Slutskyy, D. W. C. MacMillan and L. E. Overman, J. Am. Chem. Soc., 2015, 137, 11270 CrossRef CAS PubMed;
(c) J. Jin and D. W. C. MacMillan, Nature, 2015, 525, 87 CrossRef CAS PubMed.
- X.-Q. Hu, J.-R. Chen, Q. Wei, F.-L. Liu, Q.-H. Deng, A. M. Beauchemin and W.-J. Xiao, Angew. Chem., Int. Ed., 2014, 53, 12163 CrossRef CAS.
- Y. Masuda, N. Ishida and M. Murakami, J. Am. Chem. Soc., 2015, 137, 14063 CrossRef CAS PubMed.
-
(a) W. Liu, L. Li and C.-J. Li, Nat. Commun., 2015, 6, 6526 CrossRef CAS PubMed;
(b) L. Li, W. Liu, H. Zeng, X. Mu, G. Cosa, Z. Mi and C.-J. Li, J. Am. Chem. Soc., 2015, 137, 8328 CrossRef CAS PubMed.
- J. Ruch, A. Aubin, G. Erbland, A. Fortunato and J.-P. Goddard, Chem. Commun., 2016, 52, 2326 RSC.
-
(a) B. Nguyen, E. J. Emmet and M. C. Willis, J. Am. Chem. Soc., 2010, 132, 16372 CrossRef CAS PubMed;
(b) A. J. Emmet, C. S. Richards-Taylor, B. Nguyen, A. B. Garcia-Rubia, R. Hayter and M. C. Willis, Org. Biomol. Chem., 2012, 10, 4007 RSC;
(c) E. J. Emmett, B. R. Hayter and M. C. Willis, Angew. Chem., Int. Ed., 2013, 52, 12679 CrossRef CAS PubMed;
(d) E. J. Emmett, B. R. Hayter and M. C. Willis, Angew. Chem., Int. Ed., 2014, 53, 10204 CrossRef CAS PubMed;
(e) A. S. Deeming, C. J. Rusell, A. J. Hennessy and M. C. Willis, Org. Lett., 2014, 16, 150 CrossRef CAS PubMed;
(f) B. N. Rocke, K. B. Bahnck, M. Herr, S. Lavergne, V. Mascitti, C. Perreault, J. Polivkova and A. Shavnya, Org. Lett., 2014, 16, 154 CrossRef CAS PubMed;
(g) M. W. Johnson, S. W. Bagley, N. P. Mankad, R. G. Bergman, V. Mascitti and F. D. Toste, Angew. Chem., Int. Ed., 2014, 53, 4404 CrossRef CAS PubMed;
(h) P. Bisseret and N. Blanchard, Org. Biomol. Chem., 2013, 11, 5393 RSC;
(i) L. Martial, Synlett, 2013, 1595 Search PubMed;
(j) W. Li, H. Li, P. Langer, M. Beller and X.-F. Wu, Eur. J. Org. Chem., 2014, 3101 CrossRef;
(k) W. Li, M. Beller and X.-F. Wu, Chem. Commun., 2014, 50, 9513 RSC;
(l) X. Wang, L. Xue and Z. Wang, Org. Lett., 2014, 16, 4056 CrossRef CAS PubMed;
(m) A. S. Deeming, C. J. Russell and M. C. Willis, Angew. Chem., Int. Ed., 2015, 54, 1168 CrossRef CAS PubMed.
-
(a) A. Shavnya, K. D. Hesp, V. Mascitti and A. C. Smith, Angew. Chem., Int. Ed., 2015, 54, 13571 CrossRef CAS PubMed;
(b) A. S. Deeming, C. J. Russell and M. C. Willis, Angew. Chem., Int. Ed., 2016, 55, 747 CrossRef CAS PubMed;
(c) D. Zheng, R. Mao, Z. Li and J. Wu, Org. Chem. Front., 2016, 3, 359 RSC;
(d) A. S. Tsai, J. M. Curto, B. N. Rocke, A. R. Dechert-Schmitt, G. K. Ingle and V. Mascitti, Org. Lett., 2016, 18, 508 CrossRef CAS PubMed.
-
(a) S. Ye and J. Wu, Chem. Commun., 2012, 48, 7753 RSC;
(b) S. Ye and J. Wu, Chem. Commun., 2012, 48, 10037 RSC;
(c) D. Zheng, Y. An, Z. Li and J. Wu, Angew. Chem., Int. Ed., 2014, 53, 2451 CrossRef CAS PubMed;
(d) S. Ye, H. Wang, Q. Xiao, Q. Ding and J. Wu, Adv. Synth. Catal., 2014, 356, 3225 CrossRef CAS;
(e) Y. An, D. Zheng and J. Wu, Chem. Commun., 2014, 50, 11746 RSC;
(f) Y. Luo, X. Pan, C. Chen, L. Yao and J. Wu, Chem. Commun., 2015, 51, 180 RSC;
(g) D. Zheng, Y. Li, Y. An and J. Wu, Chem. Commun., 2014, 50, 8886 RSC;
(h) X. Liu, W. Wang, D. Zheng, X. Fan and J. Wu, Tetrahedron, 2015, 71, 3359 CrossRef CAS.
-
(a) G. Liu, C. Fan and J. Wu, Org. Biomol. Chem., 2015, 13, 1592 RSC;
(b) C. S. Richards-Taylor, D. C. Blakemore and M. C. Willis, Chem. Sci., 2014, 5, 222 RSC;
(c) W. E. Truce and A. M. Murphy, Chem. Rev., 1951, 48, 69 CrossRef CAS PubMed;
(d) A. S. Deeming, E. J. Emmett, C. S. Richards-Taylor and M. C. Willis, Synthesis, 2014, 2701 Search PubMed.
-
(a)
M. Bartholow, Top 200 drugs of 2011. Pharmacy times. http://www.pharmacytimes.com/publications/issue/2012/July2012/Top-200-Drugs-of-2011, accessed on Jan 9, 2013;
(b) For a list of top drugs by year, see: http://cbc.arizona.edu/njardarson/group/toppharmaceuticals-poster, accessed on Jan 9, 2013;
(c) J. Drews, Science, 2000, 287, 1960 CrossRef CAS PubMed.
Footnote |
† Electronic supplementary information (ESI) available. See DOI: 10.1039/c6qo00060f |
|
This journal is © the Partner Organisations 2016 |