DOI:
10.1039/C4RA12490A
(Paper)
RSC Adv., 2015,
5, 5015-5023
Iron(II) bromide-catalyzed oxidative coupling of benzylamines with ortho-substituted anilines: synthesis of 1,3-benzazoles†
Received
16th October 2014
, Accepted 9th December 2014
First published on 9th December 2014
Abstract
An iron(II) bromide-catalyzed oxidative coupling of benzylamines with 2-amino/hydroxy/mercapto-anilines has been developed, allowing the synthesis of a diversity of substituted 1,3-benzazoles in good to excellent yields. This transformation is compatible with a wide range of functional groups. The method is practical, economical and employs molecular oxygen as an oxidant.
Introduction
Nitrogen-containing ring systems are considered to be privileged structures because of their occurrence in many natural products and synthetic compounds with interesting biological activity.1 Specifically, benzimidazoles and their structural isosteres; benzoxazoles and benzothiazoles (1,3-benzazoles) represent a medicinally and pharmaceutically important class of heterocyclic motifs that are found as the core structural skeletons in a variety of drug molecules such as pantoprazole,2 tafamidis,3 and riluzole4 (Fig. 1). They possess a wide range of biological and pharmacological activities including antitumor,5 anticancer,6 antiparasitic,7 antiparkinson,8 and antimicrobial9 properties. Therefore, the development of different synthetic methods for the preparation of 1,3-benzazoles has received much attention. The conventional methods for the synthesis of these important heteroaromatic compounds typically involve two approaches. One consists of the condensation of ortho-substituted anilines having the cyclizable groups (NH2, OH, SH) with carboxylic acids under harsh dehydrating conditions10 or oxidative couplings with aldehydes11 (Scheme 1a). The other consists of the transition metal-catalyzed intramolecular cross-coupling of ortho-haloanilides/analogues (Scheme 1b).12 Our literature survey revealed that 1,3-benzazoles can also be synthesized from benzylamines/aliphatic amines13 or benzylalcohols14 by the reaction of ortho-substituted anilines having the amine/hydroxyl/mercaptan groups. However, there are only a few reports for these approaches. With respect to the amines as substrates, the first oxidative coupling between primary amines and 2-hydroxyanilines to synthesize benzoxazoles using Shvo catalyst {[(η5-Ph4C4CO)]2HRu2(CO)4(μ-H)} and 2,6-dimethoxybenzoquinone (DMBQ) as terminal oxidant was reported by Marsden and co-workers (Scheme 1c).13a Endo and Bäckvall employed Shvo catalyst, Co–salen complex and DMBQ to prepare benzoxazoles from primary amines and 2-hydroxyanilines.13b Taddei and co-workers reported microwave assisted Pd/C-catalyzed reaction between primary amines and 2-amino/hydroxyanilines in the presence of acetic acid and large amount of crotonitrile as hydrogen acceptor to obtain benzimidazoles and benzoxazoles.13c Subsequently, Liu et al. modified the above microwave procedure to the conventional heating and carried out the reaction with Pd/C catalyst in the absence of oxidants and hydrogen acceptors.13d Narender et al.15a and Nguyen et al.15b,c independently reported a few metal-free procedures for synthesis of benzimidazoles and benzothiazoles from benzylamines and 2-amino/mercaptoanilines. Many of the above methods have one or more shortcomings such as need of microwave radiation, precious metal catalysts, complex ligands, stoichiometric amounts of reagents, additives and non-renewable oxidants which adversely affect the economics as well as the ecofriendly nature of the reaction.
 |
| Fig. 1 Representative drugs belonging to benzimidazole, benzoxazole and benzothiazole skeletons. | |
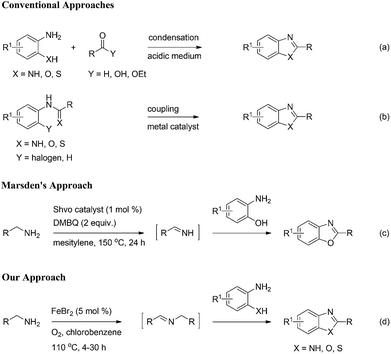 |
| Scheme 1 | |
In recent years, great advances have been made in the use of noble metals and their compounds in organic synthesis. In view of their high cost, limited availability and toxicity, researches were directed to find alternatives to the noble metal catalysts.16 In this context, iron compounds are receiving full attention as ideal candidates on several counts.17 Iron shows variable valences, cheap, environmentally benign, and suitable for safe and large scale applications. Herein, we report a practical and efficient iron(II) bromide catalyzed oxidative coupling of benzylamines with 2-amino/hydroxy/mercaptoanilines to give synthetically valuable 1,3-benzazole compounds using molecular oxygen as a sole oxidant (Scheme 1d).
Results and discussion
In our initial studies, the reaction of benzylamine (1a) with 2-aminoaniline (2a) was performed in the presence of 10 mol% of iron catalyst in chlorobenzene at 110 °C for 18 h under an air atmosphere. Firstly, various iron(II) and iron(III) salts were evaluated for their ability to catalyze the reaction (Table 1). We found that FeBr2 was the best and affording 2-phenylbenzimidazole (3a) in 78% yield (Table 1, entry 7), whereas other iron salts led to low yields (Table 1, entries 1–6). Encouraged by this promising result, we further tested the catalytic activity under the atmosphere of molecular oxygen. Gratifyingly, the reaction was accelerated and the yield of 3a was significantly increased to 92% (Table 1, entry 8). The same yield of the product 3a was also obtained when the iron catalyst loading was decreased from 10 to 5 mol% (Table 1, entry 9). However, a further decrease of iron catalyst loading resulted in relatively low yield of 3a (Table 1, entry 10). No product was observed in the absence of catalyst (Table 1, entry 11). When the reaction performed in an inert atmosphere, only trace amount of product was noticed (Table 1, entry 12). We also examined different solvents in addition to chlorobenzene for the reaction (Table 1, entries 13–15). When toluene, N,N-dimethylformamide (DMF), and N-methyl-2-pyrrolidone (NMP) were used as solvents, the yields of 3a were 68%, 83%, and 79%, respectively. Performing the reaction without solvent (neat) resulted in low yield (Table 1, entry 16). Finally, a lower reaction temperature resulted in low yield of the desired product due to incomplete reaction (Table 1, entry 17). Thus, the optimal catalytic conditions consist of 5 mol% FeBr2, chlorobenzene as the solvent, and the reaction was carried out at 110 °C under the atmosphere of molecular oxygen.
Table 1 Optimization of reaction conditions for the synthesis of benzimidazolesa

|
Entry |
Catalyst |
Solvent |
Time (h) |
Yieldb (%) |
Reaction conditions: 2a (2.0 mmol), 1a (2.4 mmol), catalyst (10 mol%), solvent (2 mL), 110 °C, air atmosphere unless otherwise noted. Isolated yield. Reaction was performed under molecular oxygen (O2 balloon). Using 5 mol% of catalyst. Using 3 mol% of catalyst. Reaction was performed under argon atmosphere. Reaction was performed at 90 °C. |
1 |
FeSO4·7H2O |
Chlorobenzene |
18 |
17 |
2 |
Fe(OAc)2 |
Chlorobenzene |
18 |
31 |
3 |
Fe(acac)3 |
Chlorobenzene |
18 |
12 |
4 |
FeCl3 |
Chlorobenzene |
18 |
43 |
5 |
FeCl2 |
Chlorobenzene |
18 |
56 |
6 |
FeBr3 |
Chlorobenzene |
18 |
70 |
7 |
FeBr2 |
Chlorobenzene |
18 |
78 |
8c |
FeBr2 |
Chlorobenzene |
4 |
92 |
9c,d |
FeBr2 |
Chlorobenzene |
4 |
92 |
10c,e |
FeBr2 |
Chlorobenzene |
4 |
80 |
11c |
— |
Chlorobenzene |
4 |
0 |
12d,f |
FeBr2 |
Chlorobenzene |
18 |
Trace |
13c,d |
FeBr2 |
Toluene |
4 |
68 |
14c,d |
FeBr2 |
DMF |
4 |
83 |
15c,d |
FeBr2 |
NMP |
4 |
79 |
16c,d |
FeBr2 |
— |
4 |
80 |
17c,d,g |
FeBr2 |
Chlorobenzene |
18 |
45 |
With the optimized reaction conditions in hand, we next explored the scope of the oxidative coupling reaction using various benzylamines (1) and 2-aminoanilines (2) (Table 2). First, the reaction between various amines (1b–1n) and 2-aminoaniline (2a) was investigated under standard conditions. The results disclosed that the reactions proceeded smoothly and tolerated a wide range of functional groups, including methoxy, fluoro, chloro, trifluoromethyl, acetal, thienyl, pyridyl and nitro groups. The electronic properties of the substituents on the aryl ring of the benzylamines affected the yields of the reaction to some extent. In general, the benzylamines bearing an electron-donating substituent (e.g., –Me and –OR) (Table 2, entries 2, 3, 8 and 9) produced a slightly higher yield of cyclization products than those analogues bearing an electron-withdrawing substituent (e.g., –F and –Cl) (Table 2, entries 4–6). However, benzylamine bearing a strong electron-withdrawing substituent such as trifluoromethyl group at the meta positions afforded slightly lower yield of product 3g (Table 2, entry 7). The steric hindrance of ortho substituent had some influence on the oxidative coupling reaction. For example, the reaction of 2a with p- and o-methoxybenzylamines resulted in the formation of the corresponding 2-p-anisylbenzimidazole (3c) and 2-o-anisylbenzimidazole (3i) in 96% and 87% yields, respectively (Table 2, entries 3 and 9). Similarly, other sterically hindered substrates such as o-chlorobenzylamine and 1-naphthylmethylamine gave the corresponding 2-(o-chlorophenyl)benzimidazole (3e) and 2-(1-naphthyl)benzimidazole (3j) in 81% and 83% yields, respectively (Table 2, entries 5 and 10). In addition, heteroarylmethylamines such as 2-thiophenemethylamine (1k) and 4-picolylamine (1l) could also be used as substrates, leading to formation of the corresponding 2-(thiophen-2-yl)benzimidazole (3k) and 2-(pyridin-4-yl)benzimidazole (3l) in 81% and 65% yields, respectively (Table 2, entries 11 and 12). Gratifyingly, non-benzylic amine 1m and secondary amine 1n were also good coupling partners for this oxidative coupling reaction, giving the corresponding products in good yields (3m, 3a, 73%, 88%, respectively, Table 2, entries 13 and 14).
Table 2 Synthesis of benzimidazolesa
Next, we studied the reactions between 4-methylbenzylamine (1b) and various substituted 2-aminoanilines (2b–2e) under standard conditions (Table 2, entries 15–18). High yields (76–82%) of the desired products were obtained with both electron-rich and electron-deficient 2-aminoanilines. Even the heteroaryl substrate 2,3-diaminopyridine produced the corresponding cyclized product without any difficulty (Table 2, entry 18). Evidently, electronic effect of the substituents on the aryl ring in 2-aminoanilines has very little influence.
Furthermore, this methodology could be extended to the preparation of benzoxazole derivatives by using 2-hydroxy-5-methylaniline (4). When the substrate 4 was treated with benzylamine (1a) under the optimized conditions used for benzimidazoles, the desired product 2-phenyl-5-methylbenzoxazole (5a) was obtained in 58% yield and the small amount of starting materials were remained in the reaction mixture after 30 h (Table 3, entry 1). However, performing the reaction at 120 °C the starting materials were completely consumed in 30 h and produced the desired product in 71% yield (Table 3, entry 2). We then applied these reaction conditions to explore the generality of the reaction. A variety of benzylamines that were used previously for the benzimidazoles syntheses, were reacted with 2-hydroxy-5-methylaniline (4) in the presence of FeBr2 in chlorobenzene at 120 °C under the atmosphere of molecular oxygen to provide benzoxazoles 5b–5k in moderate to good yields (Table 3, entries 3–12). Unfortunately, 4-picolylamine, n-octylamine and dibenzylamine failed to give the benzoxazole products under the same reaction conditions, and starting materials were recovered.
Table 3 Synthesis of benzoxazolesa
Finally, oxidative coupling of benzylamines with 2-mercaptoaniline was conducted to prepare 2-substituted benzothiazoles. The effective conditions for the reaction of 2-aminoaniline (2a) were found to be not suitable in this case. Treatment of 2-mercaptoaniline (6) with benzylamine (1a) in the presence of FeBr2 (5 mol%) in chlorobenzene under the atmosphere of molecular oxygen gave 2-phenylbenzothiazole (7a) in 53% yield (Table 4, entry 1). However, a good yield of benzothiazole 7a was obtained under solvent-free condition (Table 4, entry 2). In this way, 2-mercaptoaniline (6) was reacted with various benzylamines 1b–1l under solvent-free condition to afford benzothiazoles 7b–7l in good to high yields (Table 4, entries 3–13). A secondary amine 1n was also underwent the oxidative coupling with 2-mercaptoaniline (6) to give the 2-phenylbenzothiazole (7a) in 59% yield (Table 4, entry 14).
Table 4 Synthesis of benzothiazolesa
The synthetic utility of the iron-catalyzed oxidative coupling reaction has been demonstrated by the synthesis of some important biologically active molecules namely 2-(thiophen-2-yl)-1H-benzo[d]imidazole (3k)18 (Table 2, entry 11), and 5-methyl-2-(p-substituted phenyl)benzoxazoles 5b–d (ref. 19) (Table 3, entries 3–5), which exhibits antileishmanial and antibacterial activities, respectively.
To gain some understanding of the mechanism of these oxidative coupling reactions, a few control experiments were performed under standard conditions as shown in Scheme 2. Treatment of benzylamine (1a) with iron(II) bromide under standard conditions but in the absence of 2-amino/hydroxy/mercaptoanilines (2a, 4 and 6) furnished the homo-coupling product N-benzylbenzaldimine (10) in 90% yield (Scheme 2a). Gratifyingly, the desired products 3a, 5a and 7a were obtained smoothly in 94, 67 and 75% yields respectively, when the imine 10 treated with 2-amino/hydroxy/mercaptoanilines separately under standard conditions (Scheme 2b). Next, we carried out a reaction between benzylamine (1a) and 2-aminoaniline (2a) under standard conditions, and analyzed the reaction mixture by GC-MS at different time intervals to identify the possible intermediates (cf. ESI†). We detected the formation of N-benzylbenzaldimine (10) and N-benzylidenebenzene-1,2-diamine (11) intermediates in the reaction. In a separate experiment, the intermediate 11 prepared from 2-aminoaniline and benzaldehyde, was treated with iron(II) bromide in chlorobenzene at 110 °C under the atmosphere of molecular oxygen, furnished the 2-phenylbenzimidazole (3a) in 95% yield (Scheme 2c).
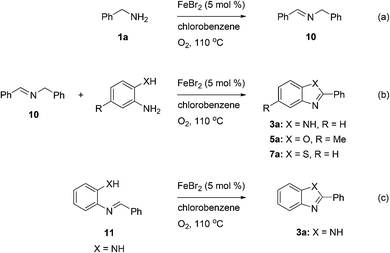 |
| Scheme 2 Control experiments. | |
On the basis of the above experimental results and previous reports in the literature,13a,16b,20 we propose a tentative mechanism for the formation of 1,3-benzazoles as outlined in Scheme 3. The first step may involve the oxidative addition of iron to the benzylamine forming the complex 8. Benzylimine (9) and iron(II) bromide are generated by further oxidation of complex 8. The reaction of imine 9 with another molecule of benzylamine generates N-benzylbenzaldimine (10) with the liberation of ammonia. The imine 10 could be easily transformed into 2-(benzylideneamino)aniline/phenol/thiophenol (11) by the transimination with 2-amino/hydroxy/mercaptoanilines. In parallel, the imine 11 could be formed directly from benzylimine (9) by transimination with 2-amino/hydroxy/mercaptoanilines. Afterwards, the imine 11 may undergo intramolecular cyclization gives the 2-phenyl-2,3-dihydro-1H-benzo[d]imidazole/oxazole/thiazole (12). Oxidation of resulting intermediates 12 in the presence of iron(II) bromide and molecular oxygen gives the 2-substituted benzimidazoles, benzoxazoles and benzothiazoles, through the formation of iron complex 13.
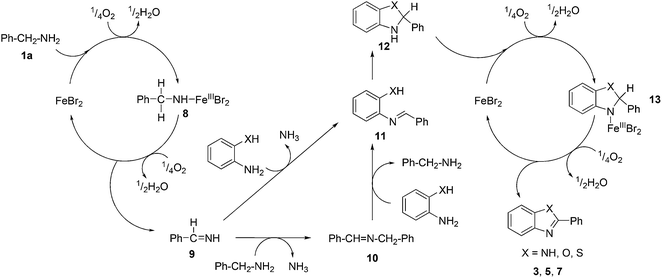 |
| Scheme 3 Proposed reaction mechanism. | |
Conclusions
We have developed a simple, novel, and efficient method for the synthesis of 2-substituted 1,3-benzazoles from benzylamines and 2-amino/hydroxy/mercaptoanilines in a one-pot process. The inexpensive and non-toxic iron(II) bromide as catalyst, and environmentally friendly molecular oxygen as oxidant were used in this procedure, which makes the transformation very practical and economical. Further studies on the scope of the reaction using benzylamines are currently underway in our laboratory.
Experimental section
General information
Melting points were determined on a Büchi melting point apparatus and were uncorrected. NMR spectra were recorded on a 400 MHz spectrometer (1H at 400 MHz, 13C at 100 MHz), using DMSO-d6 or CDCl3 or CD3OD as the solvent with tetramethylsilane (TMS) as the internal standard at room temperature. High-resolution mass spectra (HRMS) were obtained using an electrospray quadrupole time-of-flight (ESI-Q-TOF) mass spectrometer. IR spectra were recorded on a FT-IR spectrometer. GC-MS analysis were performed on a gas chromatograph mass spectrometer operating at 70 eV, connected to a gas chromatograph fitted with a HP-5 column. Standard GC-MS programme: split mode (split ratio 400
:
1), injector temperature 300 °C, MS source 230 °C, MS quadrupole 150 °C, column flow 1 mL min−1, total flow 404 mL min−1. All commercially obtained materials and solvents were used directly without further purification unless otherwise noted. Chlorobenzene was dried over P2O5 and distilled before use. Reactions were carried out in oven-dried Schlenk tube or round-bottom flask.
General procedure for iron-catalyzed synthesis of benzimidazoles 3
To a Schlenk tube were added diamine 2 (1 equiv., 2.0 mmol), benzylamine 1 (1.2 equiv., 2.4 mmol), FeBr2 (5 mol%), and chlorobenzene (2 mL). Next the tube was equipped with O2 balloon, and the reaction mixture was stirred constantly at 110 °C until complete consumption of diamine as monitored by TLC. Upon completion of the reaction, the mixture was cooled to room temperature, diluted with EtOAc, and filtered over neutral alumina. The solvent was evaporated under reduced pressure and the residue was purified by column chromatography over silica gel (100–200 mesh) using hexane/ethyl acetate mixture as eluent to afford the desired products 3.
General procedure for iron-catalyzed synthesis of benzoxazoles 5
To a Schlenk tube were added 2-hydroxy-5-methylaniline (4, 1 equiv., 2.0 mmol), benzylamine 1 (1.2 equiv., 2.4 mmol), FeBr2 (5 mol%), and chlorobenzene (1 mL). Next the tube was equipped with O2 balloon, and the reaction mixture was stirred constantly at 120 °C until complete consumption of 2-hydroxy-5-methylaniline as monitored by TLC. Upon completion of the reaction, the mixture was cooled to room temperature, diluted with CH2Cl2, and filtered over neutral alumina. The solvent was removed under reduced pressure and the residue was purified by column chromatography over silica gel (100–200 mesh) using hexane/ethyl acetate mixture as eluent to furnish the benzoxazoles 5.
General procedure for iron-catalyzed synthesis of benzothiazoles 7
To a Schlenk tube were added 2-mercaptoaniline (6, 1 equiv., 2.0 mmol), benzylamine 1 (1.2 equiv., 2.4 mmol), and FeBr2 (5 mol%). Next the tube was equipped with O2 balloon, and the reaction mixture was stirred constantly at 110 °C until complete consumption of 2-mercaptoaniline as monitored by TLC. Upon completion of the reaction, the mixture was cooled to room temperature, diluted with CH2Cl2, and filtered over neutral alumina. The solvent was evaporated under reduced pressure and the residue was purified by column chromatography over silica gel (100–200 mesh) using hexane/ethyl acetate mixture as eluent to afford the benzothiazoles 7.
Synthesis of N-benzylbenzaldimine (10)
To a Schlenk tube were added benzylamine (1a, 258 mg, 2.4 mmol, 0.5 equiv.), FeBr2 (13 mg, 5 mol%), and chlorobenzene (1 mL). Next the tube was equipped with O2 balloon, and the reaction mixture was stirred constantly at 110 °C for 2.5 h. The mixture was cooled to room temperature, adsorbed on basic alumina and purified by column chromatography over basic alumina using hexane/ethyl acetate mixture as eluent to afford the N-benzylbenzaldimine 10 (212 mg, 90% yield) as a clear, colorless oil; 1H NMR (400 MHz, CDCl3) δ 8.40 (s, 1H), 7.80–7.78 (m, 2H), 7.43–7.40 (m, 3H), 7.35–7.34 (m, 4H), 7.29–7.25 (m, 1H), 4.83 (s, 2H); 13C NMR (100 MHz, CDCl3) δ 161.9, 139.2, 136.1, 130.7, 128.6, 128.5, 128.2, 127.9, 126.9, 65.0.
Synthesis of N-benzylidenebenzene-1,2-diamine (11)
To a stirred solution of 2-aminoaniline (2a, 400 mg, 3.70 mmol) in chlorobenzene (2 mL) was added a solution of benzaldehyde (197 mg, 1.85 mmol) in chlorobenzene (1 mL) by dropwise at 0 °C under nitrogen atmosphere. The reaction mixture was stirred for 30 minutes at 0 °C and then warmed to room temperature. The crude mixture was purified by column chromatography over basic alumina using hexane/ethyl acetate mixture as eluent. Concentration of the relevant fractions (Rf = 0.43 in 0.2/9.8 v/v ethyl acetate/hexane) afforded compound 11 (127 mg, 35%) as a clear, yellow oil; 1H NMR (400 MHz, CDCl3) δ 8.46 (s, 1H), 7.85–7.82 (m, 2H), 7.40–7.38 (m, 3H), 7.02–6.98 (m, 2H), 6.71–6.66 (m, 2H), 4.15 (br, s, 2H); 13C NMR (100 MHz, CDCl3) δ 157.5, 142.2, 137.1, 136.4, 131.1, 128.9, 128.7, 128.6, 127.7, 118.4, 117.1, 115.4; HRMS (ESI) m/z calcd for C13H12N2 [M + H]+ 197.1073, found 197.1069.
Acknowledgements
The authors sincerely thank and acknowledge DU-R&D Grant and University of Delhi for financial support to carry out this work, and DU-DST mass spectrometry facility. S.N.C thanks the Council of Scientific and Industrial Research, New Delhi, India, for a research fellowship. We are grateful to Prof. M. L. N. Rao, Indian Institute of Technology Kanpur, for helping the GC-MS analysis of samples.
References
-
(a) R. D. Carpenter and M. J. Kurth, Nat. Protoc., 2010, 5, 1731 CrossRef CAS PubMed;
(b) M. A. Siracusa, L. Salerno, M. N. Modica, V. Pittalà, G. Romeo, M. E. Amato, M. Nowak, A. J. Bojarski, I. Mereghetti, A. Cagnotto and T. Mennini, J. Med. Chem., 2008, 51, 4529 CrossRef CAS PubMed;
(c) C. G. Mortimer, G. Wells, J.-P. Crochard, E. L. Stone, T. D. Bradshaw, M. F. G. Stevens and A. D. Westwell, J. Med. Chem., 2006, 49, 179 CrossRef CAS PubMed.
- S. M. Cheer, A. Prakash, D. Faulds and H. M. Lamb, Drugs, 2003, 63, 101 CrossRef CAS PubMed.
- C. E. Bulawa, S. Connelly, M. DeVit, L. Wang, C. Weigel, J. Fleming, J. Packman, E. T. Powers, R. L. Wiseman, T. R. Foss, I. A. Wilson, J. W. Kelly and R. Labaudiniere, Proc. Natl. Acad. Sci. U. S. A., 2012, 109, 9629 CrossRef CAS PubMed.
- M. C. Bellingham, CNS Neurosci. Ther., 2011, 17, 4 CrossRef CAS PubMed.
-
(a) M.-S. Chua, D.-F. Shi, S. Wrigley, T. D. Bradshaw, I. Hutchinson, P. N. Shaw, D. A. Barrett, L. A. Stanley and M. F. G. Stevens, J. Med. Chem., 1999, 42, 381 CrossRef CAS PubMed;
(b) S. Aiello, G. Wells, E. L. Stone, H. Kadri, R. Bazzi, D. R. Bell, M. F. G. Stevens, C. S. Matthews, T. D. Bradshaw and A. D. Westwell, J. Med. Chem., 2008, 51, 5135 CrossRef CAS PubMed.
- D. Kumar, M. R. Jacob, M. B. Reynolds and S. M. Kerwin, Bioorg. Med. Chem., 2002, 10, 3997 CrossRef CAS.
-
(a) R. D. Haugwitz, R. G. Angel, G. A. Jacobs, B. V. Maurer, V. L. Narayanan, L. R. Cruthers and J. Szanto, J. Med. Chem., 1982, 25, 969 CrossRef CAS;
(b) G. L. Dunn, P. Actor and V. J. DiPasquo, J. Med. Chem., 1966, 9, 751 CrossRef CAS.
- A. Benazzouz, T. Boraud, P. Dubédat, A. Boireau, J.-M. Stutzmann and C. Gross, Eur. J. Pharmacol., 1995, 284, 299 CrossRef CAS.
- I. Yildiz-Oren, I. Yalcin, E. Aki-Sener and N. Carturk, Eur. J. Med. Chem., 2004, 39, 291 CrossRef CAS PubMed.
- For representative articles related to carboxylic acids, see:
(a) G. Nieddu and G. Giacomelli, Tetrahedron, 2013, 69, 791 CrossRef CAS PubMed;
(b) X. Wen, J. El Bakali, R. Deprez-Poulain and B. Deprez, Tetrahedron Lett., 2012, 53, 2440 CrossRef CAS PubMed;
(c) H. Sharghi and O. Asemani, Synth. Commun., 2009, 39, 860 CrossRef CAS;
(d) A. Hasaninejad, K. Niknam, A. Zare, E. Farsimadan and M. Shekouhy, Phosphorus, Sulfur Silicon Relat. Elem., 2009, 184, 147 CrossRef CAS;
(e) Y. Wang, K. Sarris, D. R. Sauer and S. W. Djuric, Tetrahedron Lett., 2006, 47, 4823 CrossRef CAS PubMed , and references therein.
- For representative articles related to aldehydes, see:
(a) G. A. Molander and K. Ajayi, Org. Lett., 2012, 14, 4242 CrossRef CAS PubMed;
(b) M. A. Chari, D. Shobha and T. Sasaki, Tetrahedron Lett., 2011, 52, 5575 CrossRef PubMed;
(c) K. Bahrami, M. M. Khodaei and F. Naali, J. Org. Chem., 2008, 73, 6835 CrossRef CAS PubMed;
(d) Y.-X. Chen, L.-F. Qian, W. Zhang and B. Han, Angew. Chem., Int. Ed., 2008, 47, 9330 CrossRef CAS PubMed , and references therein.
-
(a) S. K. Alla, R. K. Kumar, P. Sadhu and T. Punniyamurthy, Org. Lett., 2013, 15, 1334 CrossRef CAS PubMed;
(b) K. Liubchak, K. Nazarenko and A. Tolmachev, Tetrahedron, 2012, 68, 2993 CrossRef CAS PubMed;
(c) J. Peng, M. Ye, C. Zong, F. Hu, L. Feng, X. Wang, Y. Wang and C. Chen, J. Org. Chem., 2011, 76, 716 CrossRef CAS PubMed;
(d) E. A. Jaseer, D. J. C. Prasad, A. Dandapat and G. Sekar, Tetrahedron Lett., 2010, 51, 5009 CrossRef CAS PubMed;
(e) P. Saha, T. Ramana, N. Purkait, A. M. Ashif, R. Paul and T. Punniyamurthy, J. Org. Chem., 2009, 74, 8719 CrossRef CAS PubMed;
(f) D. Ma, S. Xie, P. Xue, X. Zhang, J. Dong and Y. Jiang, Angew. Chem., Int. Ed., 2009, 48, 4222 CrossRef CAS PubMed;
(g) J. Bonnamour and C. Bolm, Org. Lett., 2008, 10, 2665 CrossRef CAS PubMed;
(h) T. Itoh and T. Mase, Org. Lett., 2007, 9, 3687 CrossRef CAS PubMed;
(i) G. Evindar and R. A. Batey, J. Org. Chem., 2006, 71, 1802 CrossRef CAS PubMed.
- For synthesis of benzimidazoles and its analogs from amines, please see:
(a) A. J. Blacker, M. M. Farah, S. P. Marsden, O. Saidi and J. M. J. Williams, Tetrahedron Lett., 2009, 50, 6106 CrossRef CAS PubMed;
(b) Y. Endo and J.-E. Bäckvall, Chem.–Eur. J., 2012, 18, 13609 CrossRef CAS PubMed;
(c) M. Pizzetti, E. D. Luca, E. Petricci, A. Porcheddu and M. Taddei, Adv. Synth. Catal., 2012, 354, 2453 CAS;
(d) X. Jin, Y. Liu, Q. Lu, D. Yang, J. Sun, S. Qin, J. Zhang, J. Shen, C. Chu and R. Liu, Org. Biomol. Chem., 2013, 11, 3776 RSC.
- For synthesis of benzimidazoles and its analogs from alcohols, please see:
(a) T. Kondo, S. Yang, K.-T. Huh, M. Kobayashi, S. Kotachi and Y. Watanabe, Chem. Lett., 1991, 20, 1275 CrossRef;
(b) A. J. Blacker, M. M. Farah, M. I. Hall, S. P. Marsden, O. Saidi and J. M. J. Williams, Org. Lett., 2009, 11, 2039 CrossRef CAS PubMed;
(c) Y. Shiraishi, Y. Sugano, S. Tanaka and T. Hirai, Angew. Chem., Int. Ed., 2010, 49, 1656 CrossRef CAS PubMed;
(d) V. R. Ruiz, A. Corma and M. J. Sabater, Tetrahedron, 2010, 66, 730 CrossRef CAS PubMed;
(e) K. D. Parghi and R. V. Jayaram, Catal. Commun., 2010, 11, 1205 CrossRef CAS PubMed;
(f) G. M. Raghavendra, A. B. Ramesh, C. N. Revanna, K. N. Nandeesh, K. Mantelingu and K. S. Rangappa, Tetrahedron Lett., 2011, 52, 5571 CrossRef CAS PubMed.
-
(a) G. Naresh, R. Kant and T. Narender, J. Org. Chem., 2014, 79, 3821 CrossRef CAS PubMed;
(b) T. B. Nguyen, L. Ermolenko and A. Al-Mourabit, Green Chem., 2013, 15, 2713 RSC;
(c) T. B. Nguyen, L. Ermolenko, W. A. Dean and A. Al-Mourabit, Org. Lett., 2012, 14, 5948 CrossRef CAS PubMed.
-
(a) Catalysis Without Precious Metals, ed. R. M. Bullock, Wiley-VCH, Weinheim, 2010 Search PubMed;
(b) C. Bolm, O. Beckmann and O. A. G. Dabard, Angew. Chem., Int. Ed., 1999, 38, 907 CrossRef CAS;
(c) R. A. Sheldon, CHEMTECH, 1994, 24, 38 CAS.
- For representative reviews on iron catalysis, see:
(a) K. Gopalaiah, Chem. Rev., 2013, 113, 3248 CrossRef CAS PubMed;
(b) M. Darwish and M. Wills, Catal. Sci. Technol., 2012, 2, 243 RSC;
(c) K. Junge, K. Schröder and M. Beller, Chem. Commun., 2011, 47, 4849 RSC;
(d) C. Bolm, Nat. Chem., 2009, 1, 420 CrossRef CAS PubMed;
(e) R. H. Morris, Chem. Soc. Rev., 2009, 38, 2282 RSC;
(f) E. B. Bauer, Curr. Org. Chem., 2008, 12, 1341 CrossRef CAS;
(g) A. Correa, O. García Mancheño and C. Bolm, Chem. Soc. Rev., 2008, 37, 1108 RSC;
(h) C. Bolm, J. Legros, J. Le Paih and L. Zani, Chem. Rev., 2004, 104, 6217 CrossRef CAS PubMed.
- A. Shaukat, H. M. Mirza, A. H. Ansari, M. Yasinzai, S. Z. Zaidi, S. Dilshad and F. L. Ansari, Med. Chem. Res., 2013, 22, 3606 CrossRef CAS.
- İ. Yalçin, E. Şener, T. Özden, S. Özden and A. Akin, Eur. J. Med. Chem., 1990, 25, 705 CrossRef.
-
(a) M. Largeron and M.-B. Fleury, Angew. Chem., Int. Ed., 2012, 51, 5409 CrossRef CAS PubMed;
(b) R. D. Patil and S. Adimurthy, Adv. Synth. Catal., 2011, 353, 1695 CrossRef CAS.
Footnote |
† Electronic supplementary information (ESI) available. See DOI: 10.1039/c4ra12490a |
|
This journal is © The Royal Society of Chemistry 2015 |
Click here to see how this site uses Cookies. View our privacy policy here.